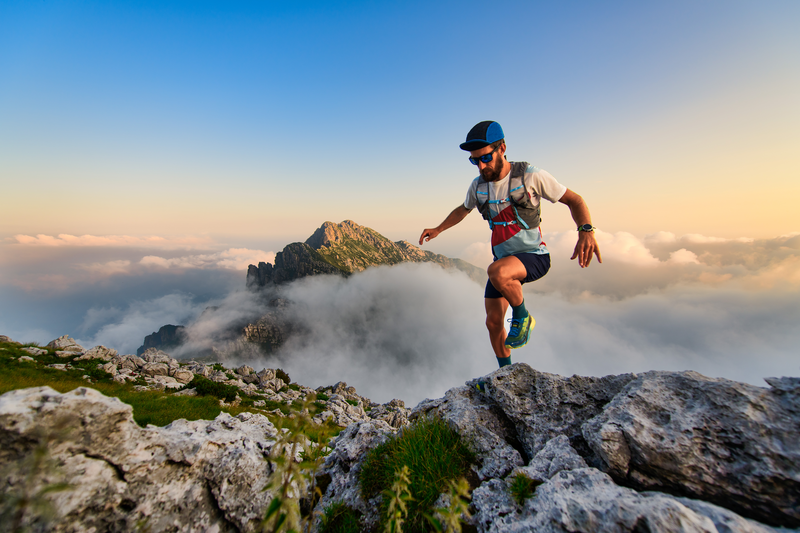
94% of researchers rate our articles as excellent or good
Learn more about the work of our research integrity team to safeguard the quality of each article we publish.
Find out more
SYSTEMATIC REVIEW article
Front. Physiol. , 23 June 2020
Sec. Exercise Physiology
Volume 11 - 2020 | https://doi.org/10.3389/fphys.2020.00652
This article is part of the Research Topic Optimal Mobility and Function across the Lifespan View all 15 articles
Osteoporosis is a major health problem in post-menopausal women (PMW). Exercise training is considered a cost-effective strategy to prevent osteoporosis in middle aged-older people. The purpose of this study is to summarize the effect of exercise on BMD among PMW. A comprehensive search of electronic databases was conducted through PubMed, Scopus, Web of Science, Cochrane, Science Direct, Eric, ProQuest, and Primo. BMD changes (standardized mean differences: SMD) of the lumbar spine (LS) femoral neck (FN) and/or total hip were considered as outcome measures. After subgroup categorization, statistical methods were used to combine data and compare subgroups. Seventy-five studies were included. The pooled number of participants was 5,300 (intervention group: n = 2,901, control group: n = 2,399). The pooled estimate of random effect analysis was SMD = 0.37, 95%-CI: 0.25–0.50, SMD = 0.33, 95%-CI: 0.23–0.43, and SMD = 0.40, 95%-CI: 0.28–0.51 for LS, FN, and total Hip-BMD, respectively. In the present meta-analysis, there was a significant (p < 0.001), but rather low effect (SMD = 0.33–0.40) of exercise on BMD at LS and proximal femur. A large variation among the single study findings was observed, with highly effective studies but also studies that trigger significant negative results. These findings can be largely attributed to differences among the exercise protocols of the studies. Findings suggest that the true effect of exercise on BMD is diluted by a considerable amount of studies with inadequate exercise protocols.
Osteoporosis is a disease characterized by low bone mass, microarchitectural deterioration of bone tissue, leading to enhanced bone fragility, and a consequent increase in fracture risk (1991). The disease is an important global public health problem (Compston et al., 2019). Due to the menopausal transition, and the corresponding decline of estrogen, post-menopausal women (PMW) in particular, are at high risk of osteoporosis (Christenson et al., 2012). Exercise training is considered to be a low cost and safe non-pharmaceutical treatment strategy for the protection of musculoskeletal health and fracture prevention (Kemmler et al., 2015; Beck et al., 2017; Daly et al., 2019), thus, many studies have focused on the effects of exercise on bone mineral density (BMD) in PMW (Bonaiuti et al., 2002; Howe et al., 2011; Marques et al., 2011a; Zhao et al., 2017). However, their effects on BMD, as the most frequently assessed parameter for bone strength, vary widely. Some studies even report a negative effect (vs. control) on BMD (Bassey and Ramsdale, 1995; Nichols et al., 1995; Choquette et al., 2011). Considering the large variety of intervention protocols that can be created when combining different types of exercise, exercise-parameters, and training-principles, there is no doubt that some loading protocols demonstrate favorable, while others trigger negative effects, on BMD. Additionally, participant characteristics vary considerably for parameters (e.g., menopausal status, bone status, training status) that might modulate the effect of exercise on BMD and thus may contribute to the low effect size of exercise reported by most meta-analyses (Kelley, 1998a,b; Martyn-St James and Carroll, 2011; Marques et al., 2011a; Zhao et al., 2017).
In the present systematic review and meta-analysis, we aimed to; (1) quantify the general effect of exercise on BMD at lumbar spine (LS) and proximal femur (PF) regions of interest (ROI) by meta-analytic techniques, (2) identify participants and exercise characteristics that explain the effect of exercise on BMD and (3) propose exercise recommendations to favorably affect BMD at the LS, femoral neck (FN) and total hip (tHip) ROI in PMW.
This review and meta-analysis follows the Preferred Reporting Items for Systematic Reviews and Meta-Analyses (PRISMA) statement (Moher et al., 2015) and was registered in advance in the International prospective register of systematic reviews (PROSPERO) (ID: CRD42018095097). A comprehensive search of electronic databases was conducted through PubMed, Scopus, Web of Science, Cochrane, Science Direct, Eric, ProQuest, and Primo for all articles published up to March 01, 2019, with no language restrictions. The search strategy utilized the population, intervention and outcome approach. The literature search was constructed around search terms for “bone mineral density,” exercise,” and “post-menopausal.”
A standard protocol for this search was developed and controlled vocabulary (Mesh term for MEDLINE) was used. Key words and their synonymous were used by applying the following queries, (“Bone” or “Bone mass” or “Bone status” or “Bone structure” or “Bone turnover” or “Bone metabolism” or “Bone mineral content” or “Skeleton” or “Bone Mineral Density” or “BMD” or “Bone Density” or “Osteoporoses” or “Osteoporosis” or “Osteopenia”) AND (“Postmenopause” or “Post-Menopause” or “Post-menopausal”) AND (“Exercise” or “Training” or “Athletic” or “Sport” or” “physical activity”) AND (“Clinical trial” or “Randomized clinical trial”). Furthermore, reference lists of the included articles were searched manually to locate additional relevant studies. Unpublished reports or articles for which only abstracts were available were not considered. Duplicate publications were identified by comparing author names, treatment comparisons, publication dates, sample sizes, intervention, and outcomes. In the case of unclear eligibility criteria or when the confirmation of any data or additional information was needed, the authors were contacted by e-mail.
Studies were included if they met the following criteria: (a) randomized or non-randomized controlled trials with at least one exercise group as an intervention vs. one control group with habitual (sedentary) lifestyle or sham exercises; (b) participants were post-menopausal at study onset; (c) the training program lasted a minimum of 6 months; (d) BMD of the LS or/and the proximal femur regions “total hip” and/or “FN” were used as outcome measures; (e) baseline and final BMD assessment reported at least for one desired regions; (f) BMD measurement assessed by dual-energy X-ray absorptiometry (DXA) or dual-photon absorptiometry (DPA); (g) studies with ≤ 10% of participants on hormone replacement therapy (HRT), hormone therapy (HT), adjuvant endocrine therapy, antiresorptive, or osteoanabolic pharmaceutic agents (e.g., Bisphosphonate, Denosumab, Strontiumranelate) or drugs with a dedicated osteo-catabolic effect on bone metabolism, (glucocorticoids), albeit only if the number of users was similar between exercise and control.
Studies addressing (a) interventions applying novel exercise technologies (e.g., whole-body vibration) (b) mixed gender or mixed pre- and post-menopausal cohorts without separate BMD analysis for PMW; (c) PMW under chemo- and/or radiotherapy; (d) PMW with diseases that affect bone metabolism; (e) the synergistic/additive effect of exercise and pharmaceutic therapy, or (f) duplicate studies or preliminary data from the subsequently published study and review articles, case reports, editorials, conference abstracts, and letters were excluded from the analysis.
Titles and abstracts were screened by an independent reviewer (MS) to exclude irrelevant studies. Two reviewers (SV and MS) separately and independently evaluated full-text articles and extracted data from the included studies. Disagreement was resolved by discussion between the two reviewers; if they could not reach a consensus a third reviewer was consulted (WK). An extraction form was designed to record the relevant data regarding publication details (i.e., the first author's name, title, country and publication year), details of the study (i.e., design, objectives, sample size for each group), participants' characteristics (i.e., age, weight, BMI, years since menopause), description of intervention (i.e., type of exercise, intervention period, frequency, intensity, duration, sets and repetition), compliance (including number of withdrawals), risk assessment, BMD assessment tool and evaluated region, BMD values at baseline and study completion.
Outcomes of interest were BMD at the LS and the proximal femur (FN and/or tHip) as assessed by Dual Energy X-Ray Absorptiometry (DXA) or Dual Photon Absorptiometry (DPA) at least at baseline and study end.
Included articles were independently assessed for risk of bias using the Physiotherapy Evidence Database (PEDro) scale risk of bias tool (Sherrington et al., 2000; de Morton, 2009). This was completed by two reviewers from Germany (MS, SvS). Partners from Finland (MM, MJ, TR), Italy (LB, LD, SM, GB) or Northern Ireland (MHM, AS) acted as a third reviewer. Potential biases in studies were selection bias, performance bias, detection bias, attrition bias, and reporting bias using 11 criteria, however, the scale scores 10 items. The categories assessed were randomization, allocation concealment, similarity at baseline, blinding of participants and staff, assessor blinding, incomplete outcome data, intention-to-treat analysis, between groups comparison, and measure of variability. Scores ranged from 0 to 10 and points were awarded when a criterion was clearly explained; otherwise, a point was not awarded. Discrepancies were discussed with a review author from Germany (WK) until a consensus was reached. The methodological quality of the included studies was classified as follows: ≥7, high; 5–6, moderate; <5, low (Ribeiro de Avila et al., 2018).
For sub-analyses, the intervention period was stratified as ≤ 8, 9–18, and >18 months by considering the remodeling cycle for cancellous and cortical bone (Eriksen, 2010). Post-menopausal status was categorized as early (≤ 8 years) and late (>9 years) (Harlow et al., 2012). We also classified the type of exercise into seven sub-groups including weight-bearing aerobic exercise (WB-AE), dynamic resistance training (DRT), Jumping+[resistance training (RT) and/or WB], WB+RT, Jumping, non-WB+RT and Tai Chi. Type of mechanical forces was categorized as joint reaction force (JRF), ground reaction force (GRF), and mix of JRF+GRF (Daly et al., 2019; Kemmler and von Stengel, 2019).
If the studies presented a confidence interval (CI) or standard errors (SE), they were converted to standard deviation (SD) by using standardized formulae (Higgins and Green, 2008). Where standard deviation was not given, authors were contacted to provide the missing data. When no reply was received or data were not available, the exact p-value of the absolute change of BMD was obtained to compute the SD of the change. In the case of unreported p-value, we calculated the SDs using pre and post SDs, and correlation coefficients with the following formula:
where “corr” is the correlation coefficient which was imputed using the mean of the correlations available for some included studies. SDpre and SDpost are the baseline and final standard deviation, respectively (Higgins and Green, 2008). This resulted in using a within-participant correlation of r = 0.95 and r = 0.94 in exercise and control groups at LS, respectively. At FN, the mean correlation was computed r = 0.82 among exercise groups and r = 0.85 for control groups. Finally, at the total hip, r = 0.97 and r = 0.98 were considered for intervention and control groups, respectively. When the absolute mean difference was not available, it was imputed by calculation of the difference between post- and pre-intervention. For those studies which measured BMD at multiple times, only the baseline and final values were included in the analysis.
The meta-analyses were performed using the package metaphor in the statistical software R (R Development Core Team, 2019). Effect size (ES) values were considered as the standardized mean differences (SMDs) combined with the 95% confidence interval (CI).
Random-effects meta-analysis was conducted by using the meta for package (Viechtbauer, 2010). Heterogeneity for between-study variability was implemented using the Cochran Q test and considered statistically significant if p-value < 0.05. The extent of heterogeneity was examined with the I2 statistics. I2 0 to 40% is considered as low heterogeneity, 30 to 60%, and 50 to 90% represent moderate and substantial heterogeneity, respectively (Higgins and Green, 2008). For those studies with two different intervention groups, the control group was split into 2 smaller groups for comparison against each intervention group (Higgins and Green, 2008).
To explore potential publication biases, a funnel plot with regression test and the rank correlation between effect estimates and their standard errors (SEs), using the t-test and Kendall's τ statistic were conducted, respectively. The p-value < 0.05 was defined as the significant level for all tests.
Subgroup analyses were performed for menopausal status, intervention duration, type of exercise, and type of mechanical forces. Sensitivity analysis was conducted to try different values of the correlation coefficient (minimum, mean or maximum) to determine whether the overall result of the analysis is robust to the use of the imputed correlation coefficient.
Of 1,757 articles initially retrieved, 1,743 studies were found from all included databases and other resources. Duplicate articles were removed and the title and abstract of the remaining articles were screened and checked based on the eligibility criteria. The full-text of 153 potentially relevant articles were then checked, and 78 of them were found not to meet the inclusion criteria. A total of 75 articles were thus included in this study, published from 1989 to 2019 (Figure 1). Three included studies contained English abstracts but with Italian (Tolomio et al., 2009), Portuguese (Orsatti et al., 2013), and German (Kemmler, 1999) full texts, which were translated by native speakers.
Seventy-five studies were included in this systematic review and meta-analysis, comprising 88 individual training groups based on our eligibility criteria (Sinaki et al., 1989; Nelson et al., 1991, 1994; Grove and Londeree, 1992; Lau et al., 1992; Pruitt et al., 1992, 1995; Bloomfield et al., 1993; Caplan et al., 1993; Hatori et al., 1993; Martin and Notelovitz, 1993; Bassey and Ramsdale, 1995; Kohrt et al., 1995, 1997; Nichols et al., 1995; Prince et al., 1995; Hartard et al., 1996; Kerr et al., 1996, 2001; Lord et al., 1996; Brooke-Wavell et al., 1997, 2001; Ebrahim et al., 1997; Bassey et al., 1998; Ryan et al., 1998; Adami et al., 1999; Kemmler, 1999; Bemben et al., 2000, 2010; Rhodes et al., 2000; Iwamoto et al., 2001; Chilibeck et al., 2002, 2013; Hans et al., 2002; Sugiyama et al., 2002; Going et al., 2003; Jessup et al., 2003; Milliken et al., 2003; Chan et al., 2004; Kemmler et al., 2004, 2010, 2013; Verschueren et al., 2004; Yamazaki et al., 2004; Englund et al., 2005; Korpelainen et al., 2006; Wu et al., 2006; Evans et al., 2007; Maddalozzo et al., 2007; Woo et al., 2007; Bergstrom et al., 2008; Kwon et al., 2008; Park et al., 2008; Bocalini et al., 2009; Chuin et al., 2009; de Matos et al., 2009; Deng, 2009; Silverman et al., 2009; Tolomio et al., 2009; Sakai et al., 2010; Choquette et al., 2011; Marques et al., 2011b,c; Tartibian et al., 2011; Bolton et al., 2012; Karakiriou et al., 2012; Basat et al., 2013; Orsatti et al., 2013; Bello et al., 2014; Moreira et al., 2014; Liu et al., 2015; Nicholson et al., 2015; Wang et al., 2015; Duff et al., 2016; de Oliveira et al., 2019). The pooled number of participants was 5,300 (intervention group: n = 2,901, control group: n = 2,399) and sample size in individual studies ranged from five (Grove and Londeree, 1992) to 125 (Adami et al., 1999) participants per group. Table 1 presents a summary of included study characteristics. The mean menopausal age ranged from at least 0.5 (according to eligibility criteria) (Sinaki et al., 1989; Wang et al., 2015) to 24 years (Jessup et al., 2003), and the range of mean ages was between 50 (Bemben et al., 2000) and 79 (Lau et al., 1992; Tella and Gallagher, 2014) years. The mean body mass index (BMI, kg/m2) of individual studies varied from 19.7 (Iwamoto et al., 2001) to 32.6 kg/m2 (Silverman et al., 2009) (Table 1).
Twenty-seven studies recruited participants with sedentary life style (Nelson et al., 1991, 1994; Grove and Londeree, 1992; Bloomfield et al., 1993; Kohrt et al., 1995, 1997; Brooke-Wavell et al., 1997, 2001; Ryan et al., 1998; Adami et al., 1999; Rhodes et al., 2000; Iwamoto et al., 2001; Jessup et al., 2003; Yamazaki et al., 2004; Wu et al., 2006; Woo et al., 2007; Bocalini et al., 2009; Kemmler et al., 2010; Choquette et al., 2011; Marques et al., 2011b,c; Tartibian et al., 2011; Karakiriou et al., 2012; Orsatti et al., 2013; Bello et al., 2014; Moreira et al., 2014; de Oliveira et al., 2019), 33 trials involved participants with some kinds of exercises activities (Pruitt et al., 1992, 1995; Martin and Notelovitz, 1993; Bassey and Ramsdale, 1995; Nichols et al., 1995; Prince et al., 1995; Hartard et al., 1996; Kerr et al., 1996, 2001; Lord et al., 1996; Ebrahim et al., 1997; Bassey et al., 1998; Kemmler, 1999; Bemben et al., 2000, 2010; Chilibeck et al., 2002, 2013; Going et al., 2003; Milliken et al., 2003; Chan et al., 2004; Kemmler et al., 2004, 2013; Bergstrom et al., 2008; Kwon et al., 2008; Park et al., 2008; Deng, 2009; Silverman et al., 2009; Sakai et al., 2010; Bolton et al., 2012; Basat et al., 2013; Nicholson et al., 2015; Wang et al., 2015; Duff et al., 2016), while the remaining studies did not provide any information with respect to the life style status of participants (Sinaki et al., 1989; Lau et al., 1992; Caplan et al., 1993; Hatori et al., 1993; Hans et al., 2002; Sugiyama et al., 2002; Verschueren et al., 2004; Englund et al., 2005; Korpelainen et al., 2006; Evans et al., 2007; Maddalozzo et al., 2007; Chuin et al., 2009; de Matos et al., 2009; Tolomio et al., 2009; Liu et al., 2015).
Sixty-one studies comprised healthy participants (Sinaki et al., 1989; Nelson et al., 1991; Grove and Londeree, 1992; Lau et al., 1992; Pruitt et al., 1992, 1995; Bloomfield et al., 1993; Caplan et al., 1993; Hatori et al., 1993; Martin and Notelovitz, 1993; Bassey and Ramsdale, 1995; Kohrt et al., 1995, 1997; Nichols et al., 1995; Prince et al., 1995; Kerr et al., 1996, 2001; Lord et al., 1996; Brooke-Wavell et al., 1997, 2001; Ebrahim et al., 1997; Bassey et al., 1998; Ryan et al., 1998; Adami et al., 1999; Kemmler, 1999; Bemben et al., 2000, 2010; Rhodes et al., 2000; Chilibeck et al., 2002, 2013; Sugiyama et al., 2002; Going et al., 2003; Jessup et al., 2003; Milliken et al., 2003; Chan et al., 2004; Verschueren et al., 2004; Englund et al., 2005; Wu et al., 2006; Evans et al., 2007; Maddalozzo et al., 2007; Woo et al., 2007; Kwon et al., 2008; Park et al., 2008; Bocalini et al., 2009; Chuin et al., 2009; Deng, 2009; Silverman et al., 2009; Kemmler et al., 2010, 2013; Sakai et al., 2010; Choquette et al., 2011; Marques et al., 2011b,c; Tartibian et al., 2011; Orsatti et al., 2013; Bello et al., 2014; Moreira et al., 2014; Nicholson et al., 2015; Wang et al., 2015; Duff et al., 2016; de Oliveira et al., 2019), and the remaining studies recruited participants with osteopenia, osteoporosis, or with a history of spinal fracture(s) (Nelson et al., 1994; Hartard et al., 1996; Iwamoto et al., 2001; Hans et al., 2002; Kemmler et al., 2004; Yamazaki et al., 2004; Korpelainen et al., 2006; Bergstrom et al., 2008; de Matos et al., 2009; Tolomio et al., 2009; Bolton et al., 2012; Karakiriou et al., 2012; Basat et al., 2013; Liu et al., 2015) (Table 2).
Table 2 outlines the exercise prescription characteristics. The program duration ranged from six (Hartard et al., 1996; Ryan et al., 1998; Adami et al., 1999; Bemben et al., 2000; Sugiyama et al., 2002; Verschueren et al., 2004; Kwon et al., 2008; Bocalini et al., 2009; Chuin et al., 2009; Silverman et al., 2009; Sakai et al., 2010; Choquette et al., 2011; Tartibian et al., 2011; Karakiriou et al., 2012; Basat et al., 2013; Moreira et al., 2014; Nicholson et al., 2015; de Oliveira et al., 2019) to 30 months (Korpelainen et al., 2006).
Eleven studies applied an intervention period of ≥18 months (Sinaki et al., 1989; Caplan et al., 1993; Prince et al., 1995; Ebrahim et al., 1997; Iwamoto et al., 2001; Kerr et al., 2001; Hans et al., 2002; Kemmler et al., 2004, 2010; Korpelainen et al., 2006; Chilibeck et al., 2013), 39 trials used an intervention period between 9 and 18 months (Nelson et al., 1991, 1994; Grove and Londeree, 1992; Lau et al., 1992; Pruitt et al., 1992, 1995; Martin and Notelovitz, 1993; Bassey and Ramsdale, 1995; Kohrt et al., 1995, 1997; Nichols et al., 1995; Kerr et al., 1996; Lord et al., 1996; Brooke-Wavell et al., 1997, 2001; Bassey et al., 1998; Kemmler, 1999; Rhodes et al., 2000; Chilibeck et al., 2002; Going et al., 2003; Milliken et al., 2003; Chan et al., 2004; Yamazaki et al., 2004; Englund et al., 2005; Wu et al., 2006; Evans et al., 2007; Maddalozzo et al., 2007; Woo et al., 2007; Bergstrom et al., 2008; Park et al., 2008; de Matos et al., 2009; Deng, 2009; Tolomio et al., 2009; Bolton et al., 2012; Kemmler et al., 2013; Orsatti et al., 2013; Liu et al., 2015; Wang et al., 2015; Duff et al., 2016), and 25 scheduled a short intervention period of ≤ 8 months (Bloomfield et al., 1993; Hatori et al., 1993; Hartard et al., 1996; Ryan et al., 1998; Adami et al., 1999; Bemben et al., 2000, 2010; Sugiyama et al., 2002; Jessup et al., 2003; Verschueren et al., 2004; Kwon et al., 2008; Bocalini et al., 2009; Chuin et al., 2009; Silverman et al., 2009; Sakai et al., 2010; Choquette et al., 2011; Marques et al., 2011b,c; Tartibian et al., 2011; Karakiriou et al., 2012; Basat et al., 2013; Bello et al., 2014; Moreira et al., 2014; Nicholson et al., 2015; de Oliveira et al., 2019). Of importance, no study reported a delay between the end of the intervention and the control assessments.
Of all 75 included studies, 13 had two intervention groups (based on our eligibility criteria). Five of them assigned various types of exercises between the intervention groups (Grove and Londeree, 1992; Kohrt et al., 1997; Woo et al., 2007; Marques et al., 2011c; Basat et al., 2013), the other 5 trials compared two different training intensities (Hatori et al., 1993; Pruitt et al., 1995; Kerr et al., 1996, 2001; Bemben et al., 2000) whereas, Martin and Notelovitz (1993) categorized intervention groups according to the training duration (Martin and Notelovitz, 1993). Moreover, one study considered two intervention groups with different Tai Chi styles (Wang et al., 2015). Kemmler (1999) classified participants based on the menopausal status, and they were included in the analysis as individual intervention groups.
The majority of the 88 intervention groups employed aerobic exercise as the main component of their intervention, with walking and/or jogging the most common types (Nelson et al., 1991; Grove and Londeree, 1992; Lau et al., 1992; Bloomfield et al., 1993; Hatori et al., 1993; Martin and Notelovitz, 1993; Bassey and Ramsdale, 1995; Kohrt et al., 1995, 1997; Prince et al., 1995; Brooke-Wavell et al., 1997, 2001; Ebrahim et al., 1997; Bassey et al., 1998; Ryan et al., 1998; Hans et al., 2002; Sugiyama et al., 2002; Yamazaki et al., 2004; Korpelainen et al., 2006; Wu et al., 2006; Evans et al., 2007; Silverman et al., 2009; Sakai et al., 2010; Marques et al., 2011c; Tartibian et al., 2011; Basat et al., 2013). Twenty-six training protocols combined aerobic and resistance exercise (Caplan et al., 1993; Lord et al., 1996; Kohrt et al., 1997; Adami et al., 1999; Kemmler, 1999; Iwamoto et al., 2001; Kerr et al., 2001; Going et al., 2003; Jessup et al., 2003; Milliken et al., 2003; Kemmler et al., 2004, 2010, 2013; Englund et al., 2005; Bergstrom et al., 2008; Kwon et al., 2008; Park et al., 2008; de Matos et al., 2009; Deng, 2009; Choquette et al., 2011; Marques et al., 2011b; Bolton et al., 2012; Karakiriou et al., 2012; Chilibeck et al., 2013; Bello et al., 2014; Moreira et al., 2014). Resistance exercise as the predominant component was prescribed by 27 intervention groups (Sinaki et al., 1989; Pruitt et al., 1992, 1995; Nelson et al., 1994; Nichols et al., 1995; Hartard et al., 1996; Kerr et al., 1996, 2001; Kohrt et al., 1997; Bemben et al., 2000, 2010; Rhodes et al., 2000; Chilibeck et al., 2002; Verschueren et al., 2004; Maddalozzo et al., 2007; Woo et al., 2007; Bocalini et al., 2009; Chuin et al., 2009; Marques et al., 2011c; Basat et al., 2013; Orsatti et al., 2013; Nicholson et al., 2015; Duff et al., 2016; de Oliveira et al., 2019), Tai Chi was utilized in 5 training groups (Chan et al., 2004; Woo et al., 2007; Liu et al., 2015; Wang et al., 2015).
Exercise intensities varied considerably between the exercise protocols (very low to high; Garber et al., 2011). With respect to resistance training, most of the studies prescribed a training intensity of 70–80% of one repetition maximum (1-RM). Aerobic exercise was predominately performed in the range between 60 and 80% of the maximum heart rate maximum (HRmax). In 54 intervention groups, the exercise intensity was progressively increased during the intervention period (Sinaki et al., 1989; Pruitt et al., 1992, 1995; Bloomfield et al., 1993; Martin and Notelovitz, 1993; Nelson et al., 1994; Kohrt et al., 1995, 1997; Nichols et al., 1995; Hartard et al., 1996; Kerr et al., 1996, 2001; Ryan et al., 1998; Kemmler, 1999; Bemben et al., 2000; Rhodes et al., 2000; Iwamoto et al., 2001; Chilibeck et al., 2002, 2013; Hans et al., 2002; Going et al., 2003; Jessup et al., 2003; Milliken et al., 2003; Kemmler et al., 2004, 2010, 2013; Verschueren et al., 2004; Englund et al., 2005; Korpelainen et al., 2006; Evans et al., 2007; Maddalozzo et al., 2007; Bergstrom et al., 2008; Kwon et al., 2008; Bocalini et al., 2009; Chuin et al., 2009; de Matos et al., 2009; Deng, 2009; Choquette et al., 2011; Marques et al., 2011b,c; Tartibian et al., 2011; Bolton et al., 2012; Orsatti et al., 2013; Moreira et al., 2014; Nicholson et al., 2015; Duff et al., 2016; de Oliveira et al., 2019).
Fifty-one intervention groups adequately addressed their endpoints LS and/or FN BMD by their exercise protocol (site specificity) (Lau et al., 1992; Pruitt et al., 1992, 1995; Nelson et al., 1994; Nichols et al., 1995; Hartard et al., 1996; Kerr et al., 1996, 2001; Lord et al., 1996; Kohrt et al., 1997; Kemmler, 1999; Bemben et al., 2000, 2010; Rhodes et al., 2000; Iwamoto et al., 2001; Chilibeck et al., 2002, 2013; Going et al., 2003; Jessup et al., 2003; Milliken et al., 2003; Kemmler et al., 2004, 2010, 2013; Englund et al., 2005; Korpelainen et al., 2006; Evans et al., 2007; Maddalozzo et al., 2007; Woo et al., 2007; Bergstrom et al., 2008; Kwon et al., 2008; Bocalini et al., 2009; Chuin et al., 2009; de Matos et al., 2009; Deng, 2009; Choquette et al., 2011; Marques et al., 2011b,c; Karakiriou et al., 2012; Basat et al., 2013; Orsatti et al., 2013; Bello et al., 2014; Moreira et al., 2014; Nicholson et al., 2015; Duff et al., 2016; de Oliveira et al., 2019). Some studies defined BMD at LS and/or FN as a study endpoint—however, the corresponding bone regions were not (or at least not adequately) addressed by their training protocol (Table 2).
The majority of studies prescribed an exercise frequency of three times per week (range 2–9 sessions/week) (Nelson et al., 1994; Hartard et al., 1996; Lord et al., 1996; Adami et al., 1999; Iwamoto et al., 2001; Englund et al., 2005; Maddalozzo et al., 2007; Marques et al., 2011b; Nicholson et al., 2015). Exercise session duration ranged from ≈2 to 110 min (Adami et al., 1999; Sakai et al., 2010). During resistance training sessions 1–21 exercises (Sinaki et al., 1989; Nicholson et al., 2015; de Oliveira et al., 2019), with up to 108 repetitions (Nicholson et al., 2015) structured in 1–5 sets (Sinaki et al., 1989; Pruitt et al., 1992; Deng, 2009; Basat et al., 2013; de Oliveira et al., 2019), were applied per session. Sixteen RT studies (Nelson et al., 1994; Nichols et al., 1995; Hartard et al., 1996; Kerr et al., 1996; Kemmler et al., 2004, 2010, 2013; Maddalozzo et al., 2007; Bocalini et al., 2009; Chuin et al., 2009; de Matos et al., 2009; Marques et al., 2011c; Karakiriou et al., 2012; Orsatti et al., 2013; Moreira et al., 2014; de Oliveira et al., 2019) additionally listed rest period between sets and/or exercises (range: 15–180 s). Time under tension (TUT) was reported in nine studies only (Nelson et al., 1994; Hartard et al., 1996; Rhodes et al., 2000; Kemmler et al., 2004, 2010, 2013; Maddalozzo et al., 2007; de Matos et al., 2009; Marques et al., 2011c) and ranged between 3 and 9 s per repetition, with two studies using fast or explosive movements in the concentric part of the exercise.
Exercise sessions were supervised in 59 studies (Nelson et al., 1991, 1994; Grove and Londeree, 1992; Lau et al., 1992; Pruitt et al., 1992, 1995; Bloomfield et al., 1993; Caplan et al., 1993; Martin and Notelovitz, 1993; Bassey and Ramsdale, 1995; Nichols et al., 1995; Prince et al., 1995; Hartard et al., 1996; Kerr et al., 1996, 2001; Lord et al., 1996; Bassey et al., 1998; Ryan et al., 1998; Adami et al., 1999; Kemmler, 1999; Bemben et al., 2000, 2010; Rhodes et al., 2000; Chilibeck et al., 2002, 2013; Going et al., 2003; Jessup et al., 2003; Milliken et al., 2003; Chan et al., 2004; Kemmler et al., 2004, 2010, 2013; Englund et al., 2005; Korpelainen et al., 2006; Wu et al., 2006; Evans et al., 2007; Maddalozzo et al., 2007; Woo et al., 2007; Bergstrom et al., 2008; Kwon et al., 2008; Bocalini et al., 2009; Chuin et al., 2009; Deng, 2009; Silverman et al., 2009; Tolomio et al., 2009; Choquette et al., 2011; Marques et al., 2011b,c; Tartibian et al., 2011; Bolton et al., 2012; Karakiriou et al., 2012; Basat et al., 2013; Orsatti et al., 2013; Bello et al., 2014; Moreira et al., 2014; Nicholson et al., 2015; Wang et al., 2015; Duff et al., 2016; de Oliveira et al., 2019). Ten trials used non-supervised home-exercise protocols (Sinaki et al., 1989; Kohrt et al., 1995; Brooke-Wavell et al., 1997, 2001; Ebrahim et al., 1997; Iwamoto et al., 2001; Hans et al., 2002; Sugiyama et al., 2002; Sakai et al., 2010; Liu et al., 2015). The remaining studies did not state the corresponding setting comprehensively (Hatori et al., 1993; Kohrt et al., 1997; Verschueren et al., 2004; Yamazaki et al., 2004; Park et al., 2008; de Matos et al., 2009).
The majority of studies reported attendance rates of more than 70% [minimum: 39% (Prince et al., 1995), maximum: 100% (Brooke-Wavell et al., 1997; Ebrahim et al., 1997; Yamazaki et al., 2004)]. However, 15 studies did not provide any information regarding the attendance rate (Sinaki et al., 1989; Lau et al., 1992; Hatori et al., 1993; Iwamoto et al., 2001; Jessup et al., 2003; Milliken et al., 2003; Verschueren et al., 2004; Wu et al., 2006; Evans et al., 2007; Kwon et al., 2008; Park et al., 2008; de Matos et al., 2009; Tolomio et al., 2009; Orsatti et al., 2013; Wang et al., 2015).
PEDro scores are listed in Table 3. The methodological quality of 14 trials can be considered as high (Ebrahim et al., 1997; Chilibeck et al., 2002, 2013; Jessup et al., 2003; Korpelainen et al., 2006; Woo et al., 2007; Park et al., 2008; Kemmler et al., 2010, 2013; Bolton et al., 2012; Orsatti et al., 2013; Nicholson et al., 2015; Duff et al., 2016; de Oliveira et al., 2019), 44 studies demonstrated moderate (Sinaki et al., 1989; Nelson et al., 1991, 1994; Grove and Londeree, 1992; Lau et al., 1992; Pruitt et al., 1992, 1995; Caplan et al., 1993; Hatori et al., 1993; Martin and Notelovitz, 1993; Nichols et al., 1995; Prince et al., 1995; Hartard et al., 1996; Kerr et al., 1996, 2001; Brooke-Wavell et al., 1997, 2001; Kemmler, 1999; Rhodes et al., 2000; Iwamoto et al., 2001; Hans et al., 2002; Going et al., 2003; Milliken et al., 2003; Chan et al., 2004; Verschueren et al., 2004; Wu et al., 2006; Evans et al., 2007; Maddalozzo et al., 2007; Bergstrom et al., 2008; Bocalini et al., 2009; Chuin et al., 2009; Tolomio et al., 2009; Bemben et al., 2010; Sakai et al., 2010; Choquette et al., 2011; Marques et al., 2011b,c; Tartibian et al., 2011; Basat et al., 2013; Bello et al., 2014; Moreira et al., 2014; Liu et al., 2015; Wang et al., 2015), while the remaining studies (n = 17) were classified as being of low quality (Table 3).
Fourteen of the 75 trials assessed BMD at LS and proximal femur (Prince et al., 1995; Pruitt et al., 1995; Bemben et al., 2000, 2010; Chilibeck et al., 2002, 2013; Sugiyama et al., 2002; Kemmler et al., 2004; Wu et al., 2006; Maddalozzo et al., 2007; Choquette et al., 2011; Nicholson et al., 2015; Duff et al., 2016; de Oliveira et al., 2019), 9 studies measured BMD only at LS (Sinaki et al., 1989; Grove and Londeree, 1992; Hatori et al., 1993; Martin and Notelovitz, 1993; Iwamoto et al., 2001; Verschueren et al., 2004; Yamazaki et al., 2004; Evans et al., 2007; Karakiriou et al., 2012), while seven studies focused only on the BMD of at least one proximal femur ROI (Kerr et al., 1996; Hans et al., 2002; Korpelainen et al., 2006; Tolomio et al., 2009; Sakai et al., 2010; Marques et al., 2011c; Bello et al., 2014).
Seventy-nine trials evaluated the effect of exercise on BMD at the LS. In summary, the exercise intervention resulted in significant positive effects (P < 0.001). The pooled estimate of random effect analysis was 0.37, 95%-CI: 0.25–0.50 with a substantial level of heterogeneity between trials [I2 = 73.2%, Q = 262.43, degrees of freedom (df) = 78, P < 0.001; Figure 2A]. Sensitivity analysis revealed the most similar effect, when the mean correlation coefficient (max correlation: SMD = 0.65, 95%-CI: 0.43–0.86; min correlation: SMD = 0.26, 95%-CI: 0.17–0.36) was utilized to impute SD of the absolute change for those studies with missing SDs, and when the analysis was computed among studies with available SDs of the change (25 groups) (SMD = 0.32, 95%-CI: 0.10–0.53, P = 0.004). The funnel plot suggested positive evidence of publication bias (Figure 2B). The rank correlation test for funnel plot asymmetry further confirmed the significant asymmetry (P = 0.002).
Figure 2. (A) Forest plot of meta-analysis results at the LS. The data are shown as pooled standard mean difference (SMD) with 95% CI for changes in exercise and control groups. (B) Funnel plot of LS BMD with Trim and Fill. SE, standard error of standardized mean difference; SMD, standardized mean difference.
Sixty-eight intervention groups evaluated the effect of exercise on BMD of the FN. The random-effect analysis demonstrated a significant pooled difference between the exercise and control groups (P < 0.0001). The pooled estimate of random effect analysis was 0.33, 95%-CI: 0.23–0.43. There was a moderate level of heterogeneity in estimates of the exercise effect [I2 = 59.8%, Q = 166.35, degrees of freedom (df) = 67, P < 0.001; Figure 3A]. Sensitivity analysis indicated the most similar effect when the mean correlation coefficient (max correlation: SMD = 0.74, 95%-CI: 0.49–1.00; min correlation: SMD = 0.24, 95%-CI: 0.16–0.32) was used to impute SD of the absolute change for those trials with missing SDs, and when the analysis was conducted among studies with available SDs of the change (25 groups) (SMD = 0.36, 95%-CI: 0.19–0.52, P = 0.0001). The funnel plot suggested positive evidence of publication bias (Figure 3B). The regression test for funnel plot asymmetry presented the significant asymmetry (P = 0.03).
Figure 3. (A) Forest plot of meta-analysis results at the FN. The data are shown as pooled standard mean difference (SMD) with 95% CI for changes in exercise and control groups. (B) Funnel plot of FN BMD with Trim and Fill. SE, standard error of standardized mean diffterence; SMD, standardized mean difference.
Twenty-nine intervention groups addressed the effect of exercise on BMD of the total Hip. Our result demonstrated a significant exercise-induced improvement in total Hip BMD (P < 0.0001). The pooled estimate of random effect analysis, favoring exercise intervention over the control group, was 0.40, 95%-CI: 0.28–0.51. There was a low level of heterogeneity in estimates of the exercise effect [I2 = 21.8%, Q = 34.79, degrees of freedom (df) = 28, P = 0.176; Figure 4A). Sensitivity analysis revealed the most similar effect when the mean correlation coefficient (max correlation: SMD = 0.51, 95%-CI: 0.36–0.66; min correlation: SMD = 0.32, 95%-CI: 0.21–0.42) was used to impute SD of the absolute change for those studies with missing SDs, and when the analysis was computed among studies with available SDs of the change (11 groups) (SMD = 0.39, 95%-CI: 0.19–0.58, P < 0.0001). The funnel plot provided no evidence of publication bias (Figure 4B) which was confirmed by the rank correlation test for funnel plot asymmetry (P = 0.42).
Figure 4. (A) Forest plot of meta-analysis results at the total hip. The data are shown as pooled standard mean difference (SMD) with 95% CI for changes in exercise and control groups. (B) Funnel plot of total hip BMD with Trim and Fill. SE, standard error of standardized mean difference; SMD, standardized mean difference.
LS-BMD: To estimate the effect of menopausal status on LS BMD, we only included studies that listed information concerning the menopausal status (early vs. late) of their cohorts. In summary, forty-nine groups were analyzed and a mixed-effects analysis found no significant difference between the early (≤ 8 years, 14 groups) and late (> 8 years, 35 groups) (P = 0.24) post-menopausal groups. A subgroup analysis that compared the early (Grove and Londeree, 1992; Pruitt et al., 1992; Kemmler, 1999; Bemben et al., 2000; Sugiyama et al., 2002; Chan et al., 2004; Kemmler et al., 2004, 2013; Wu et al., 2006; Maddalozzo et al., 2007; Deng, 2009; Karakiriou et al., 2012) and late-post-menopausal (Nelson et al., 1991; Lau et al., 1992; Bloomfield et al., 1993; Caplan et al., 1993; Kohrt et al., 1995, 1997; Nichols et al., 1995; Prince et al., 1995; Pruitt et al., 1995; Lord et al., 1996; Brooke-Wavell et al., 1997, 2001; Adami et al., 1999; Kemmler, 1999; Rhodes et al., 2000; Iwamoto et al., 2001; Jessup et al., 2003; Verschueren et al., 2004; Yamazaki et al., 2004; Englund et al., 2005; Woo et al., 2007; Kwon et al., 2008; Park et al., 2008; Bocalini et al., 2009; Chuin et al., 2009; de Matos et al., 2009; Bemben et al., 2010; Kemmler et al., 2010; Marques et al., 2011b; Tartibian et al., 2011; Nicholson et al., 2015; Duff et al., 2016) group with their corresponding control-groups indicate comparable effects on LS-BMD (early: SMD = 0.64, 95%-CI: 0.33–0.95 vs. late post-menopausal: 0.39, 0.19–0.59).
FN-BMD: Of 68 groups that addressed FN-BMD, 44 exercise groups comprised early or late post-menopausal participants. A mixed-effects analysis found no significant difference between early (≤ 8 years, 10 groups) and late (>8 years, 34 groups) (P = 0.65) PMW. The subgroup analysis that compared the early (Pruitt et al., 1992; Kemmler, 1999; Bemben et al., 2000; Sugiyama et al., 2002; Chan et al., 2004; Kemmler et al., 2004; Wu et al., 2006; Maddalozzo et al., 2007; Deng, 2009) vs. the late-post-menopausal exercise-groups (Nelson et al., 1991; Lau et al., 1992; Bloomfield et al., 1993; Caplan et al., 1993; Kohrt et al., 1995, 1997; Nichols et al., 1995; Prince et al., 1995; Pruitt et al., 1995; Lord et al., 1996; Brooke-Wavell et al., 1997, 2001; Adami et al., 1999; Kemmler, 1999; Rhodes et al., 2000; Hans et al., 2002; Jessup et al., 2003; Englund et al., 2005; Korpelainen et al., 2006; Kwon et al., 2008; Park et al., 2008; Bocalini et al., 2009; Chuin et al., 2009; Bemben et al., 2010; Kemmler et al., 2010; Sakai et al., 2010; Marques et al., 2011b,c; Tartibian et al., 2011; Nicholson et al., 2015; Duff et al., 2016) with their corresponding control-groups did not detect different effects of menopausal status on FN-BMD (early: SMD = 0.31; 95%-CI: 0.09–0.52 vs. late-post-menopausal: 0.39, 0.17–0.60).
Total Hip-BMD: Twenty studies with tHip-BMD assessment reported the menopausal status of their cohorts. A mixed-effects analysis indicated no statistically significant difference between the early (≤ 8 years, 7 groups) and late (> 8 years, 13 groups) post-menopausal group (P = 0.37).
The sub-group analysis did not indicate a different effect of varying menopausal status on BMD at the tHip-ROI [early- (Bemben et al., 2000; Sugiyama et al., 2002; Kemmler et al., 2004, 2013; Wu et al., 2006; Maddalozzo et al., 2007): SMD = 0.51, 95%-CI: 0.27–0.75 vs. late post-menopausal (Prince et al., 1995; Pruitt et al., 1995; Hans et al., 2002; Woo et al., 2007; de Matos et al., 2009; Bemben et al., 2010; Sakai et al., 2010; Marques et al., 2011c; Nicholson et al., 2015; Duff et al., 2016): 0.38, 0.20–0.56].
LS-BMD: Of 79 groups, 25 training groups were included in the short-term intervention (≤ 8 months) group (Bloomfield et al., 1993; Hatori et al., 1993; Hartard et al., 1996; Ryan et al., 1998; Adami et al., 1999; Bemben et al., 2000, 2010; Sugiyama et al., 2002; Jessup et al., 2003; Verschueren et al., 2004; Kwon et al., 2008; Bocalini et al., 2009; Chuin et al., 2009; Silverman et al., 2009; Choquette et al., 2011; Marques et al., 2011b; Tartibian et al., 2011; Karakiriou et al., 2012; Basat et al., 2013; Moreira et al., 2014; Nicholson et al., 2015; de Oliveira et al., 2019), 44 groups were classified as applying a moderate duration (9–18 months) intervention (Nelson et al., 1991, 1994; Grove and Londeree, 1992; Lau et al., 1992; Pruitt et al., 1992, 1995; Martin and Notelovitz, 1993; Bassey and Ramsdale, 1995; Kohrt et al., 1995, 1997; Nichols et al., 1995; Lord et al., 1996; Brooke-Wavell et al., 1997, 2001; Bassey et al., 1998; Kemmler, 1999; Rhodes et al., 2000; Chilibeck et al., 2002; Going et al., 2003; Milliken et al., 2003; Chan et al., 2004; Yamazaki et al., 2004; Englund et al., 2005; Wu et al., 2006; Evans et al., 2007; Maddalozzo et al., 2007; Woo et al., 2007; Bergstrom et al., 2008; Park et al., 2008; de Matos et al., 2009; Deng, 2009; Bolton et al., 2012; Kemmler et al., 2013; Orsatti et al., 2013; Liu et al., 2015; Wang et al., 2015; Duff et al., 2016), and 10 training groups applied a long intervention (≥18 months) (Sinaki et al., 1989; Caplan et al., 1993; Prince et al., 1995; Ebrahim et al., 1997; Iwamoto et al., 2001; Kerr et al., 2001; Kemmler et al., 2004, 2010; Chilibeck et al., 2013). According to a mixed-effects analysis, no significant difference was observed between the sub-groups (P = 0.26). However, the short intervention period demonstrated a slightly higher effect (exercise vs. control, SMD = 0.59, 95%-CI: 0.29–0.9) than the moderate (0.30, 0.15–0.45) or the long intervention duration (0.28, −0.15–0.58) that did not significantly differ from control (P = 0.06).
FN-BMD: Of 68 groups, 25 studies applied a short (Bloomfield et al., 1993; Hartard et al., 1996; Ryan et al., 1998; Bemben et al., 2000, 2010; Sugiyama et al., 2002; Jessup et al., 2003; Kwon et al., 2008; Bocalini et al., 2009; Chuin et al., 2009; Silverman et al., 2009; Sakai et al., 2010; Choquette et al., 2011; Marques et al., 2011b,c; Tartibian et al., 2011; Basat et al., 2013; Bello et al., 2014; Moreira et al., 2014; Nicholson et al., 2015; de Oliveira et al., 2019), 35 groups scheduled a moderate (Nelson et al., 1991, 1994; Lau et al., 1992; Pruitt et al., 1992, 1995; Bassey and Ramsdale, 1995; Kohrt et al., 1995, 1997; Nichols et al., 1995; Kerr et al., 1996; Lord et al., 1996; Brooke-Wavell et al., 1997, 2001; Bassey et al., 1998; Kemmler, 1999; Rhodes et al., 2000; Chilibeck et al., 2002; Going et al., 2003; Milliken et al., 2003; Chan et al., 2004; Englund et al., 2005; Wu et al., 2006; Maddalozzo et al., 2007; Park et al., 2008; Deng, 2009; Tolomio et al., 2009; Orsatti et al., 2013; Liu et al., 2015; Wang et al., 2015; Duff et al., 2016), and 8 groups conducted a long duration of the exercise intervention (Caplan et al., 1993; Prince et al., 1995; Ebrahim et al., 1997; Hans et al., 2002; Kemmler et al., 2004, 2010; Korpelainen et al., 2006; Chilibeck et al., 2013). A mixed-effects analysis did not observe significant differences between the sub-groups (P = 0.83). The subgroups analysis demonstrated that the short intervention period triggered the highest effects (exercise vs. control, SMD = 0.38, 95%-CI: 0.20–0.56) followed by moderate (0.32, 0.15–0.49), and long intervention duration (0.30, 0.13–0.47).
Total Hip-BMD: Of 29 groups, 11 training groups were classified as short-term (Bemben et al., 2000, 2010; Sugiyama et al., 2002; Sakai et al., 2010; Choquette et al., 2011; Marques et al., 2011c; Bello et al., 2014; Nicholson et al., 2015; de Oliveira et al., 2019), 12 groups were classified as moderate (Pruitt et al., 1995; Chilibeck et al., 2002; Wu et al., 2006; Maddalozzo et al., 2007; Woo et al., 2007; Bergstrom et al., 2008; de Matos et al., 2009; Bolton et al., 2012; Kemmler et al., 2013; Duff et al., 2016), and six training groups were categorized as long-term interventions (Prince et al., 1995; Kerr et al., 2001; Hans et al., 2002; Kemmler et al., 2004; Chilibeck et al., 2013). A mixed-effects analysis indicated no significant difference between the subgroups (P = 0.50). In contrast to LS and FN, the subgroup analysis indicated that long-term intervention demonstrated a tendentially more favorable effect on tHip-BMD (exercise vs. control, SMD = 0.48, 95%-CI: 0.27–0.7) than moderate (0.39, 0.23–0.55) or short intervention duration (0.31, 0.06–0.55).
LS-BMD: Of 79 groups, 18 training groups were classified as WB-AE (Nelson et al., 1991; Lau et al., 1992; Hatori et al., 1993; Martin and Notelovitz, 1993; Kohrt et al., 1995, 1997; Prince et al., 1995; Brooke-Wavell et al., 1997, 2001; Ebrahim et al., 1997; Ryan et al., 1998; Yamazaki et al., 2004; Wu et al., 2006; Evans et al., 2007; Silverman et al., 2009; Tartibian et al., 2011), 15 as DRT (Pruitt et al., 1992, 1995; Nelson et al., 1994; Hartard et al., 1996; Bemben et al., 2000; Chilibeck et al., 2002; Maddalozzo et al., 2007; Woo et al., 2007; Orsatti et al., 2013; Nicholson et al., 2015; Duff et al., 2016; de Oliveira et al., 2019), 11 as Jumping+RT+WB (Grove and Londeree, 1992; Bassey and Ramsdale, 1995; Kemmler, 1999; Milliken et al., 2003; Kemmler et al., 2004, 2013; Deng, 2009; Bolton et al., 2012; Karakiriou et al., 2012; Basat et al., 2013), 24 as WB+RT (Grove and Londeree, 1992; Caplan et al., 1993; Nichols et al., 1995; Lord et al., 1996; Adami et al., 1999; Iwamoto et al., 2001; Kerr et al., 2001; Going et al., 2003; Jessup et al., 2003; Verschueren et al., 2004; Englund et al., 2005; Bergstrom et al., 2008; Kwon et al., 2008; Park et al., 2008; Bocalini et al., 2009; Chuin et al., 2009; de Matos et al., 2009; Bemben et al., 2010; Kemmler et al., 2010; Choquette et al., 2011; Marques et al., 2011b; Basat et al., 2013; Chilibeck et al., 2013), two groups as jumping (Bassey et al., 1998; Sugiyama et al., 2002), 4 groups as non-WB+RT (Bloomfield et al., 1993; Kohrt et al., 1997; Rhodes et al., 2000; Moreira et al., 2014), and five training groups as Tai Chi intervention (Chan et al., 2004; Woo et al., 2007; Liu et al., 2015; Wang et al., 2015). A mixed-effects analysis did not reveal significant differences between the subgroups (P = 0.36). According to the subgroup analysis, Jumping+RT+WB triggered the most favorable (and reliable) effects on LS-BMD (exercise vs. control, SMD = 0.71, 95%-CI: 0.33–1.10), followed by dynamic RT (0.40, 0.13–0.67) and the WB+RT intervention (0.30, 0.10–0.50). There was a considerable variation of study effects in the WB-AE (18 groups, 0.24, −0.03 −0.52), Tai Chi (5 groups, 0.37, −0.08 to 0.83), Non-WB+RT (4 groups, 1.05, −0.31 to 2.50) -groups with no significant differences to control in the three latter groups. Of note, the (two) jumping only studies revealed a slight trend to negative effects on BMD (−0.07, −0.46 to 0.32).
FN-BMD: Of 68 training groups, 15 were classified as WB-AE (Nelson et al., 1991; Lau et al., 1992; Kohrt et al., 1995, 1997; Prince et al., 1995; Brooke-Wavell et al., 1997, 2001; Ebrahim et al., 1997; Ryan et al., 1998; Hans et al., 2002; Wu et al., 2006; Silverman et al., 2009; Sakai et al., 2010; Marques et al., 2011c; Tartibian et al., 2011), 15 as DRT (Pruitt et al., 1992, 1995; Nelson et al., 1994; Hartard et al., 1996; Kerr et al., 1996; Bemben et al., 2000; Chilibeck et al., 2002; Maddalozzo et al., 2007; Orsatti et al., 2013; Nicholson et al., 2015; Duff et al., 2016; de Oliveira et al., 2019), 8 as Jumping+RT+WB (Bassey and Ramsdale, 1995; Kemmler, 1999; Milliken et al., 2003; Kemmler et al., 2004; Korpelainen et al., 2006; Deng, 2009; Basat et al., 2013), 20 as WB+RT (Caplan et al., 1993; Nichols et al., 1995; Lord et al., 1996; Adami et al., 1999; Going et al., 2003; Jessup et al., 2003; Englund et al., 2005; Kwon et al., 2008; Park et al., 2008; Bocalini et al., 2009; Chuin et al., 2009; Tolomio et al., 2009; Bemben et al., 2010; Kemmler et al., 2010; Choquette et al., 2011; Marques et al., 2011b,c; Basat et al., 2013; Chilibeck et al., 2013; Bello et al., 2014), 2 as jumping (Bassey and Ramsdale, 1995; Sugiyama et al., 2002), 4 as non-WB+RT (Bloomfield et al., 1993; Kohrt et al., 1997; Rhodes et al., 2000; Moreira et al., 2014), and 4 as Tai Chi exercise type (Chan et al., 2004; Liu et al., 2015; Wang et al., 2015). A mixed-effects analysis did not result in significant differences between the subgroups (P = 0.43). According to the subgroup analysis, the Non-WB+RT (4 groups, SMD = 0.68, 95%-CI: 0.16–1.19) and the Tai Chi (4 groups, 0.64, 0.21–1.05) demonstrated the most favorable effects (vs. corresponding control), followed by WB-AE (0.42, 0.03–0.81), Jumping+RT+WB (0.39, 0.17–0.62), WB+RT (0.30, 0.12–0.48) and DRT (0.21, 0.04–0.38). A tangentially negative effect was observed for the Jumping subgroup (2 studies, −0.12, −0.62 to 0.37).
Total Hip-BMD: Of 29 groups, five training groups were considered as WB-AE (Prince et al., 1995; Hans et al., 2002; Wu et al., 2006; Sakai et al., 2010; Marques et al., 2011c), 10 groups as DRT (Prince et al., 1995; Bemben et al., 2000; Chilibeck et al., 2002; Maddalozzo et al., 2007; Woo et al., 2007; Nicholson et al., 2015; Duff et al., 2016; de Oliveira et al., 2019), three groups as Jumping+RT+WB (Kemmler et al., 2004, 2013; Bolton et al., 2012), and 9 groups as WB+RT (Kerr et al., 2001; Bergstrom et al., 2008; de Matos et al., 2009; Bemben et al., 2010; Choquette et al., 2011; Marques et al., 2011c; Chilibeck et al., 2013; Bello et al., 2014). The Jumping (Sugiyama et al., 2002) and Tai Chi (Woo et al., 2007) groups comprised only one intervention group, thus they were excluded from the analysis. Based on the mixed-effects analysis, no significant differences were seen between the subgroups (P = 0.08). According to the subgroup analysis, Jumping+RT+WB showed the largest effect (exercise vs. control, SMD = 0.65, 95%-CI: 0.30–1.00) followed by the DRT (0.51, 0.28–0.74), the WB-AE (0.36, 0.16–0.56), and the WB+RT group (0.24, 0.08–0.41).
Finally, study interventions were categorized in GRF, JRF or mixed (GRF and JRF) mechanical forces.
LS-BMD: Of 79 groups, 19 training groups applied JRF exercise (Sinaki et al., 1989; Pruitt et al., 1992, 1995; Bloomfield et al., 1993; Nelson et al., 1994; Hartard et al., 1996; Kohrt et al., 1997; Bemben et al., 2000; Rhodes et al., 2000; Chilibeck et al., 2002; Maddalozzo et al., 2007; Woo et al., 2007; Orsatti et al., 2013; Moreira et al., 2014; Nicholson et al., 2015; Duff et al., 2016; de Oliveira et al., 2019), 20 applied GRF exercise (Nelson et al., 1991; Lau et al., 1992; Hatori et al., 1993; Martin and Notelovitz, 1993; Bassey and Ramsdale, 1995; Kohrt et al., 1995, 1997; Prince et al., 1995; Brooke-Wavell et al., 1997, 2001; Ebrahim et al., 1997; Bassey et al., 1998; Sugiyama et al., 2002; Yamazaki et al., 2004; Wu et al., 2006; Silverman et al., 2009; Tartibian et al., 2011; Basat et al., 2013), and 35 studies prescribed mixed mechanical forces protocols (Grove and Londeree, 1992; Caplan et al., 1993; Nichols et al., 1995; Lord et al., 1996; Ryan et al., 1998; Adami et al., 1999; Kemmler, 1999; Iwamoto et al., 2001; Kerr et al., 2001; Going et al., 2003; Jessup et al., 2003; Milliken et al., 2003; Kemmler et al., 2004, 2010, 2013; Verschueren et al., 2004; Englund et al., 2005; Evans et al., 2007; Bergstrom et al., 2008; Kwon et al., 2008; Park et al., 2008; Bocalini et al., 2009; Chuin et al., 2009; de Matos et al., 2009; Deng, 2009; Bemben et al., 2010; Choquette et al., 2011; Marques et al., 2011b; Bolton et al., 2012; Karakiriou et al., 2012; Basat et al., 2013; Chilibeck et al., 2013). A further of 5 training groups (Chan et al., 2004; Woo et al., 2007; Liu et al., 2015; Wang et al., 2015), could not be reliably classified within one of the categories therefore we excluded them from the subgroup analysis. A mixed-effects analysis found no significant differences between the categories (P = 0.46). According to the subgroup analysis, JRF exercise triggered the highest effect on LS-BMD (exercise vs. control, SMD = 0.46, 95%-CI: 0.21–0.70), followed by the mixed JRF and GRF (0.41, 0.22–0.59). GRF exercise however, did not significantly (P = 0.09) differ from corresponding control (0.24, −0.04 to 0.53).
FN-BMD: Of 78 groups, 19 training groups were classified as JRF type exercise (Pruitt et al., 1992; Bloomfield et al., 1993; Nelson et al., 1994; Prince et al., 1995; Hartard et al., 1996; Kerr et al., 1996; Kohrt et al., 1997; Bemben et al., 2000; Rhodes et al., 2000; Chilibeck et al., 2002; Maddalozzo et al., 2007; Orsatti et al., 2013; Moreira et al., 2014; Nicholson et al., 2015; Duff et al., 2016; de Oliveira et al., 2019), 18 as GRF (Nelson et al., 1991; Lau et al., 1992; Bassey and Ramsdale, 1995; Kohrt et al., 1995, 1997; Prince et al., 1995; Brooke-Wavell et al., 1997, 2001; Ebrahim et al., 1997; Bassey et al., 1998; Hans et al., 2002; Sugiyama et al., 2002; Korpelainen et al., 2006; Wu et al., 2006; Silverman et al., 2009; Marques et al., 2011c; Tartibian et al., 2011; Basat et al., 2013) and 26 groups as mixed JRF and GRF protocols (Caplan et al., 1993; Nichols et al., 1995; Lord et al., 1996; Ryan et al., 1998; Adami et al., 1999; Kemmler, 1999; Going et al., 2003; Jessup et al., 2003; Milliken et al., 2003; Kemmler et al., 2004, 2010; Englund et al., 2005; Kwon et al., 2008; Park et al., 2008; Bocalini et al., 2009; Chuin et al., 2009; Deng, 2009; Tolomio et al., 2009; Bemben et al., 2010; Choquette et al., 2011; Marques et al., 2011b,c; Basat et al., 2013; Chilibeck et al., 2013; Bello et al., 2014). Five training groups cannot be reliably classified (Chan et al., 2004; Sakai et al., 2010; Liu et al., 2015; Wang et al., 2015), therefore they were excluded from the sub-group analysis. A mixed-effects analysis demonstrated no significant differences between the subgroups (P = 0.89). All the groups demonstrated comparable significant effects on FN-BMD (JRF: SMD = 0.29, 95%-CI: 0.14–0.44 vs. GRF: 0.35, 0.03–0.66 vs. JRF and GRF: 0.34, 0.19–0.49).
Total Hip-BMD: Of 29 groups, 10 training groups were included in the JRF group (Pruitt et al., 1995; Bemben et al., 2000; Chilibeck et al., 2002; Maddalozzo et al., 2007; Woo et al., 2007; Nicholson et al., 2015; Duff et al., 2016; de Oliveira et al., 2019). Five intervention groups were classified as GRF (Prince et al., 1995; Hans et al., 2002; Sugiyama et al., 2002; Wu et al., 2006; Marques et al., 2011c) and 12 groups as mixed intervention (Kerr et al., 2001; Kemmler et al., 2004, 2013; Bergstrom et al., 2008; de Matos et al., 2009; Bemben et al., 2010; Choquette et al., 2011; Marques et al., 2011c; Bolton et al., 2012; Chilibeck et al., 2013; Bello et al., 2014). Two training groups (Woo et al., 2007; Sakai et al., 2010) that could not be reliably classified were excluded. A mixed-effects analysis found no significant differences between the subgroups (P = 0.57). According to the subgroup analysis, effect size in the JRF-group was largest (SMD = 0.51, 95%-CI: 0.28–0.74), followed by the GRF (0.44, 0.22–0.66) and the mixed JRF and GRF subgroup (0.34, 0.14–0.53) obtained a positive significant difference in comparison with control groups.
A considerable number of systematic reviews and meta-analyses focus on the effect of exercise on BMD at the LS and/or proximal femur. With few exceptions (for LS; Howe et al., 2011) most studies reported low effect sizes (SMD = 0.2–0.5) on average (e.g., Kelley, 1998a,b; Martyn-St. James and Caroll, 2006; Howe et al., 2011; Marques et al., 2011a; Zhao et al., 2017). Due to continued research in the area, we have been able to include more exercise studies in our analysis than previous works (e.g., Howe et al., 2011; Marques et al., 2011a; Zhao et al., 2017). Nevertheless, our finding (SMD-LS = 0.37, SMD-FN = 0.33, SMD-tHip = 0.40) confirmed the results of a significant, but rather small effect of exercise on BMD, at the LS or a relevant proximal femur-ROIs. We largely attribute this finding of limited increase in BMD to the widely diverging effect sizes (e.g., Figures 2A, 3B) across the exercise trials included. Apart from participants' characteristics, considerable differences in exercise characteristics might explain these striking variations among the included trials. We sought to identify parameters that affect the impact of exercise on BMD. Therefore, studies were classified according to (1) menopausal status (Kemmler, 1999; Beck and Snow, 2003), (2) type of exercise (Giangregorio et al., 2014; Beck et al., 2017; Daly et al., 2019), (3) type of mechanical forces (JRF, GRF, JRF and GRF) (Martyn-St James and Carroll, 2011; Daly et al., 2019), and (4) duration of the intervention. Menopausal status might be an important predictor of exercise effects on BMD (Kemmler, 1999), due to the high bone-turnover during the early-menopausal years (Tella and Gallagher, 2014). However, the corresponding subgroup analysis did not determine significant differences or a consistent trend for all BMD-regions (LS, FN, tHip). Type of exercise and mechanical forces were included since mechanistically, they might be the most crucial predictors for the effect of exercise on bone (Giangregorio et al., 2014; Beck et al., 2017; Daly et al., 2019), while longer exposure to exercise (i.e., intervention duration) should result in higher effects on bone, at least when strain was regularly adjusted (“progression”) (Kemmler et al., 2015). Accepting the viewpoint that exercise-induced BMD changes were predominately generated by remodeling (Eriksen, 2010), and considering the length of a remodeling cycle in (older) adults (Eriksen, 2010; Bonucci and Ballanti, 2014), interventions ≤ 8 months might be too short to determine the full extent of bones mineralization1. In contrast, although non-significant, the subgroup analysis demonstrated considerably higher effects on LS-BMD among studies with short compared with moderate or long durations (SMD = 0.59 vs. 0.30 vs. 0.28). Based on bone physiology (Eriksen, 2010), it is rather unlikely that exercise interventions ≤ 8 months resulted in higher increases in BMD-LS compared with interventions 18 months and longer. We attribute this dubious finding to the complex interaction of exercise parameters that might have confounded the interaction between training frequency and BMD-LS.
Significant differences in BMD changes within the corresponding subgroups was not detected. Tendentially negative effects of jumping exercise on LS- and FN-BMD2 or the trend (p = 0.06) to higher effects of short exercise duration on LS and FN-BMD was observed.
We did not address exercise intensity (Rubin and Lanyon, 1985; Frost, 2003) or -frequency (Kemmler and von Stengel, 2013; Kemmler et al., 2016), which is a key modulator of effective exercise protocols (Weineck, 2019). It was planned to include “exercise intensity” in the subgroups analysis; however, it was not possible to present a meaningful and comprehensive rating of all the studies3. Since 15 studies did not report attendance rate and therefore the factual training frequency remained vague, exercise frequency was not evaluated.
Due to the results of the (exercise) group comparisons and subgroup analysis, we are unable to give validated exercise recommendations for optimized bone-strengthening protocols for PMW. In this context, Gentil et al. (2017) questioned whether “there is any practical application of meta-analytic results in strength training.” This might be overstating the issue; however trying to derive exercise recommendations and, to a lesser degree, the proper effect size estimation will fail when addressing varying exercise interventions “en bloc.” Several aspects support this view. First, exercise is a very complex intervention. The type of exercise alone ranges from HIT-RT or depth jumps, for example, to brisk walking, chair exercises and balance training. Additionally, exercise parameters (intensity, duration, cycle number, frequency etc.; Toigo and Boutellier, 2006; Weineck, 2019) and training principles (e.g., progression, periodization etc.; Weineck, 2019), fundamentally modify the effect of the exercise type on a given study endpoint. Even minor variations in single exercise parameters can result in considerable differences in BMD changes (e.g., Kemmler et al., 2016). In parallel, the present analysis indicates that a lack of consistent progression might prevent further BMD changes after initial adaptations4, according to non-compliance with the overload principle (Weineck, 2019). At this point, a frequent limitation of exercise research arises: Unlike in pharmaceutical trials, the general effectiveness of the exercise protocol was rarely evaluated before the initiation of the clinical trial (phase III) (Umscheid et al., 2011). Further, in some cases, there is an impression that some older studies (Bloomfield et al., 1993; Brooke-Wavell et al., 1997, 2001) evaluate the least significant effect of exercise on bone. This further contributes to the considerable “apple-oranges problem” (Esteves et al., 2017; Milojevic et al., 2018) of meta-analysis in the area of “exercise.” In summary thus, we conclude that uncritical acceptance of the acquired meta-analytic data (particularly) of exercise studies is certainly unwarranted.
Some study limitations may decrease the validity of our study. The lack of information related to participant and exercise characteristics and in the case of missing responses after contacting the authors meant that we estimated some variables. For example, in studies that did not provide the menopausal status of their participants, we consider the age of 51 years as the menopausal transition age to estimate the post-menopausal age (Palacios et al., 2010). Further, we excluded studies that included participants with pharmaceutic agents or diseases, known to relevantly affect BMD, in order to prevent a confounding, synergistic/additive/permissive effect on our study endpoints. However, due to the lack of information in most individual studies, we were unable to adjust for changes of medication, diet or emerging diseases.
Another predominately biometrical issue was that SDs of the absolute change in BMD were not consistently available and have thus to be imputed, which may have reduced the accuracy of the data. Further, there is considerable evidence for a publication bias with respect to exercise-induced BMD changes at the LS and tHip. Considering the aspect that most authors tend to reported positive effects the true effect size of exercise on BMD might be slightly lower compared to the results presented here (Sterne et al., 2011).
The main limitation was the extensive approach of including all types of exercise in the main analysis, which resulted in large variations in effects sizes. Moreover, our inability to categorize adequately relevant exercise characteristics hinders the proper comparison of homogeneous and widely independent subgroups and thus prevents validated exercise recommendations. Hence, upcoming meta-analysis in the area of exercise on bone should focus on dedicated areas of exercise. However, we conclude that well-designed randomized controlled trials which allow adjusting for one single parameter while keeping all others constant might be the better option for evaluating the contribution of participants and exercise parameters on exercise effect on bone and deriving sophisticated recommendations for exercise.
In summary, our approach of (1) including heterogeneous exercise studies, (2) categorizing them according to relevant modulators and exercise parameters, and (3) comparing the corresponding subgroups to identify modulators of exercise effects on bone and (more important) the most favorable exercise protocol on bone by means of enhanced statistics ultimately failed. This result can be largely attributed to fundamental and complex differences among the exercise protocols of the large amount of exercise studies included, which in effect prevent a meaningful categorization of exercise parameters.
The datasets generated during and/or analyzed during the current study are available from the first author (bWFoZGllaC5zaG9qYWFAaW1wLnVuaS1lcmxhbmdlbi5kZQ==) at the Institute of Medical Physics of Friedrich-Alexander University Erlangen-Nürnberg upon reasonable request.
MS and WK initiated the Meta-analysis. The literature search was done by MS. MS, SV, MK, DS, and WK performed data analysis, interpretation, and drafted the manuscript. MS, WK, SV, MK, DS, GB, LB, LD, SM, MHM, AS, MM, MJ, and TR contributed to quality assessment and revised the manuscript. WK accepted responsibility for the integrity of the data sampling, analysis and interpretation. All authors contributed to the article and approved the submitted version.
This study is one of the intellectual outputs of the project ACTLIFE-Physical activity the tool to improve the quality of life in osteoporosis people and had grant support from the European Union's Erasmus Plus Sport program under grant agreement No. 2017-2128/001-001.
The authors declare that the research was conducted in the absence of any commercial or financial relationships that could be construed as a potential conflict of interest.
The present work was performed in (partial) fulfillment of the requirements for obtaining the degree Dr. rer. biol. hum for the MS.
1. ^Taking into account that DXA only determines the mineralized bone matrix (i.e., BMD).
2. ^Most recommendations (e.g., Beck et al., 2017; Daly et al., 2019; Kemmler and von Stengel, 2019), however, consider Jumping as a favorable of type of exercise for PMW.
3. ^The classification of exercise intensity in the area of bone research is not trivial. WK and SV failed to generate a reliable classification of exercise intensity/strain magnitude across the (endurance and resistance type) studies of the present review.
4. ^We speculate that lack of progression contribute to the result of the subgroup analysis that address intervention-duration.
(1991). Consensus development conference: prophylaxis and treatment of osteoporosis. Am. J. Med. 90, 107–110. doi: 10.1016/0002-9343(91)90512-V
Adami, S., Gatti, D., Braga, V., Bianchini, D., and Rossini, M. (1999). Site-specific effects of strength training on bone structure and geometry of ultradistal radius in postmenopausal women. J. Bone Miner. Res. 14, 120–124. doi: 10.1359/jbmr.1999.14.1.120
Basat, H., Esmaeilzadeh, S., and Eskiyurt, N. (2013). The effects of strengthening and high-impact exercises on bone metabolism and quality of life in postmenopausal women: a randomized controlled trial. J. Back Musculoskelet. Rehabil. 26, 427–435. doi: 10.3233/BMR-130402
Bassey, E. J., and Ramsdale, S. J. (1995). Weight-bearing exercise and ground reaction forces: a 12-month randomized controlled trial of effects on bone mineral density in healthy postmenopausal women. Bone 16, 469–476. doi: 10.1016/8756-3282(95)90193-0
Bassey, E. J., Rothwell, M. C., Littlewood, J. J., and Pye, D. W. (1998). Pre- and postmenopausal women have different bone mineral density responses to the same high-impact exercise. J. Bone Miner. Res. 13, 1805–1813. doi: 10.1359/jbmr.1998.13.12.1805
Beck, B. R., Daly, R. M., Singh, M. A., and Taaffe, D. R. (2017). Exercise and sports science Australia (ESSA) position statement on exercise prescription for the prevention and management of osteoporosis. J. Sci. Med. Sport 20, 438–445. doi: 10.1016/j.jsams.2016.10.001
Beck, B. R., and Snow, C. M. (2003). Bone health across the lifespan–exercising our options. Exerc. Sport Sci. Rev. 31, 117–122. doi: 10.1097/00003677-200307000-00003
Bello, M., Sousa, M. C., Neto, G., Oliveira, L., Guerras, I., Mendes, R., et al. (2014). The effect of a long-term, community-based exercise program on bone mineral density in postmenopausal women with pre-diabetes and type 2 diabetes. J. Hum. Kinet. 43, 43–48. doi: 10.2478/hukin-2014-0088
Bemben, D. A., Fetters, N. L., Bemben, M. G., Nabavi, N., and Koh, E. T. (2000). Musculoskeletal responses to high- and low-intensity resistance training in early postmenopausal women. Med. Sci. Sports Exerc. 32, 1949–1957. doi: 10.1097/00005768-200011000-00020
Bemben, D. A., Palmer, I. J., Bemben, M. G., and Knehans, A. W. (2010). Effects of combined whole-body vibration and resistance training on muscular strength and bone metabolism in postmenopausal women. Bone 47, 650–656. doi: 10.1016/j.bone.2010.06.019
Bergstrom, I., Landgren, B., Brinck, J., and Freyschuss, B. (2008). Physical training preserves bone mineral density in postmenopausal women with forearm fractures and low bone mineral density. Osteoporos Int. 19, 177–183. doi: 10.1007/s00198-007-0445-6
Bloomfield, S. A., Williams, N. I., Lamb, D. R., and Jackson, R. D. (1993). Non-weightbearing exercise may increase lumbar spine bone mineral density in healthy postmenopausal women. Am. J. Phys. Med. Rehabil. 72, 204–209. doi: 10.1097/00002060-199308000-00006
Bocalini, D. S., Serra, A. J., dos Santos, L., Murad, N., and Levy, R. F. (2009). Strength training preserves the bone mineral density of postmenopausal women without hormone replacement therapy. J. Aging Health 21, 519–527. doi: 10.1177/0898264309332839
Bolton, K. L., Egerton, T., Wark, J., Wee, E., Matthews, B., Kelly, A., et al. (2012). Effects of exercise on bone density and falls risk factors in post-menopausal women with osteopenia: a randomised controlled trial. J. Sci. Med. Sport 15, 102–109. doi: 10.1016/j.jsams.2011.08.007
Bonaiuti, D., Shea, B., Iovine, R., Negrini, S., Robinson, V., Kemper, H. C., et al. (2002). Exercise for preventing and treating osteoporosis in postmenopausal women. Cochrane Database Syst. Rev. 3:CD000333. doi: 10.1002/14651858.CD000333
Bonucci, E., and Ballanti, P. (2014). Osteoporosis-bone remodeling and animal models. Toxicol. Pathol. 42, 957–969. doi: 10.1177/0192623313512428
Brooke-Wavell, K., Jones, P. R., and Hardman, A. E. (1997). Brisk walking reduces calcaneal bone loss in post-menopausal women. Clin. Sci. 92, 75–80. doi: 10.1042/cs0920075
Brooke-Wavell, K., Jones, P. R., Hardman, A. E., and Tsuritan Yamada, Y. (2001). Commencing, continuing and stopping brisk walking: effects on bone mineral density, quantitative ultrasound of bone and markers of bone metabolism in postmenopausal women. Osteoporos. Int. 12, 581–587. doi: 10.1007/s001980170081
Caplan, G. A., Ward, J. A., and Lord, S. R. (1993). The benefits of exercise in postmenopausal women. Aust. J. Public Health 17, 23–26. doi: 10.1111/j.1753-6405.1993.tb00099.x
Chan, K., Qin, L., Lau, M., Woo, J., Au, S., Choy, W., et al. (2004). A randomized, prospective study of the effects of Tai Chi Chun exercise on bone mineral density in postmenopausal women. Arch. Phys. Med. Rehabil. 85, 717–722. doi: 10.1016/j.apmr.2003.08.091
Chilibeck, P. D., Davison, K. S., Whiting, S. J., Suzuki, Y., Janzen, C. L., and Peloso, P. (2002). The effect of strength training combined with bisphosphonate (etidronate) therapy on bone mineral, lean tissue, and fat mass in postmenopausal women. Can. J. Physiol. Pharmacol. 80, 941–950. doi: 10.1139/y02-126
Chilibeck, P. D., Vatanparast, H., Pierson, R., Case, A., Olatunbosun, O., Whiting, S. J., et al. (2013). Effect of exercise training combined with isoflavone supplementation on bone and lipids in postmenopausal women: a randomized clinical trial. J. Bone Miner. Res. 28, 780–793. doi: 10.1002/jbmr.1815
Choquette, S., Riesco, E., Cormier, E., Dion, T., Aubertin-Leheudre, M., and Dionne, I. J. (2011). Effects of soya isoflavones and exercise on body composition and clinical risk factors of cardiovascular diseases in overweight postmenopausal women: a 6-month double-blind controlled trial. Br. J. Nutr. 105, 1199–1209. doi: 10.1017/S0007114510004897
Christenson, E. S., Jiang, X., Kagan, R., and Schnatz, P. (2012). Osteoporosis management in post-menopausal women. Minerva. Ginecol. 64, 181–194.
Chuin, A., Labonte, M., Tessier, D., Khalil, A., Bobeuf, F., Doyon, C. Y., et al. (2009). Effect of antioxidants combined to resistance training on BMD in elderly women: a pilot study. Osteoporos. Int. 20, 1253–1258. doi: 10.1007/s00198-008-0798-5
Compston, J. E., McClung, M. R., and Leslie, W. D. (2019). Osteoporosis. Lancet 393, 364–376. doi: 10.1016/S0140-6736(18)32112-3
Daly, R. M., Dalla Via, J., Duckham, R. L., Fraser, S. F., and Helge, E. W. (2019). Exercise for the prevention of osteoporosis in postmenopausal women: an evidence-based guide to the optimal prescription. Braz. J. Phys. Ther. 23, 170–180. doi: 10.1016/j.bjpt.2018.11.011
de Matos, O. D. J., Lopes da Silva, J., de Oliveira, M., and Castelo-Branco, C. (2009). Effect of specific exercise training on bone mineral density in women with postmenopausal osteopenia or osteoporosis. Gynecol. Endocrinol. 25, 616–620. doi: 10.1080/09513590903015593
de Morton, N. A. (2009). The PEDro scale is a valid measure of the methodological quality of clinical trials: a demographic study. Aust. J. Physiother. 55, 129–133. doi: 10.1016/S0004-9514(09)70043-1
de Oliveira, L. C., de Oliveira, R. G., and de Almeida Pires-Oliveira, D. A. (2019). Effects of whole-body vibration versus pilates exercise on bone mineral density in postmenopausal women: a randomized and controlled clinical trial. J. Geriatr. Phys. Ther. 42, E23–E31. doi: 10.1519/JPT.0000000000000184
Deng, S. (2009). Effects of exercise therapy on bone mineral density in early postmenopausal women: a controlled trial. Front. Med. China 3, 323–329. doi: 10.1007/s11684-009-0061-2
Duff, W. R., Kontulainen, S. A., Candow, D. G., Gordon, J. J., Mason, R. S., Taylor-Gjevre, R., et al. (2016). Effects of low-dose ibuprofen supplementation and resistance training on bone and muscle in postmenopausal women: a randomized controlled trial. Bone Rep. 5, 96–103. doi: 10.1016/j.bonr.2016.04.004
Ebrahim, S., Thompson, P. W., Baskaran, V., and Evans, K. (1997). Randomized placebo-controlled trial of brisk walking in the prevention of postmenopausal osteoporosis. Age Ageing 26, 253–260. doi: 10.1093/ageing/26.4.253
Englund, U., Littbrand, H., Sondell, A., Pettersson, U., and Bucht, G. (2005). A 1-year combined weight-bearing training program is beneficial for bone mineral density and neuromuscular function in older women. Osteoporos. Int. 16, 1117–1123. doi: 10.1007/s00198-004-1821-0
Eriksen, E. F. (2010). Cellular mechanisms of bone remodeling. Rev. Endocr. Metab. Disord. 11, 219–227. doi: 10.1007/s11154-010-9153-1
Esteves, S. C., Majzoub, A., and Agarwal, A. (2017). The problem of mixing 'apples and oranges' in meta-analytic studies. Transl. Androl. Urol. 6(Suppl. 4), S412–S413. doi: 10.21037/tau.2017.03.23
Evans, E. M., Racette, S. B., Van Pelt, R. E., Peterson, L. R., and Villareal, D. T. (2007). Effects of soy protein isolate and moderate exercise on bone turnover and bone mineral density in postmenopausal women. Menopause 14(3 Pt 1), 481–488. doi: 10.1097/01.gme.0000243570.78570.f7
Frost, H. M. (2003). Bone's mechanostat. A 2003 update. Anat Rec. 275A, 1081–1101. doi: 10.1002/ar.a.10119
Garber, C. E., Blissmer, B., Deschenes, M. R., Franklin, B. A., Lamonte, M. J., Lee, I. M., et al. (2011). American College of Sports Medicine Position stand. Quantity and quality of exercise for developing and maintaining cardiorespiratory, musculoskeletal, and neuromotor fitness in apparently healthy adults: guidance for prescribing exercise. Med. Sci. Sports Exerc. 43, 1334–1359. doi: 10.1249/MSS.0b013e318213fefb
Gentil, P., Arruda, A., Souza, D., Giessing, J., Paoli, A., Fisher, J., et al. (2017). Is there any practical application of meta-analytical results in strength training? Front. Physiol. 8:1. doi: 10.3389/fphys.2017.00001
Giangregorio, L. M., Papaioannou, A., Macintyre, N. J., Ashe, M. C., Heinonen, A., Shipp, K., et al. (2014). Too fit to fracture: exercise recommendations for individuals with osteoporosis or osteoporotic vertebral fracture. Osteoporos. Int. 25, 821–835. doi: 10.1007/s00198-013-2523-2
Going, S., Lohman, T., Houtkooper, L., Metcalfe, L., Flint-Wagner, H., Blew, R., et al. (2003). Effects of exercise on bone mineral density in calcium-replete postmenopausal women with and without hormone replacement therapy. Osteoporos. Int. 14, 637–643. doi: 10.1007/s00198-003-1436-x
Grove, K. A., and Londeree, B. R. (1992). Bone density in postmenopausal women: high impact vs low impact exercise. Med. Sci. Sports Exerc. 24, 1190–1194. doi: 10.1249/00005768-199211000-00002
Hans, D., Genton, L., Drezner, M. K., Schott, A. M., Pacifici, R., Avioli, L., et al. (2002). Monitored impact loading of the hip: initial testing of a home-use device. Calcif. Tissue Int. 71, 112–120. doi: 10.1007/s00223-001-2063-1
Harlow, S. D., Gass, M., Hall, J. E., Lobo, R., Maki, P., Rebar, R. W., et al. (2012). Executive summary of the Stages of reproductive aging workshop + 10: addressing the unfinished agenda of staging reproductive aging. J. Clin. Endocrinol. Metab. 97, 1159–1168. doi: 10.1210/jc.2011-3362
Hartard, M., Haber, P., Ilieva, D., Preisinger, E., Seidl, G., and Huber, J. (1996). Systematic strength training as a model of therapeutic intervention. A controlled trial in postmenopausal women with osteopenia. Am. J. Phys. Med. Rehabil. 75, 21–28. doi: 10.1097/00002060-199601000-00006
Hatori, M., Hasegawa, A., Adachi, H., Shinozaki, A., Hayashi, R., Okano, H., et al. (1993). The effects of walking at the anaerobic threshold level on vertebral bone loss in postmenopausal women. Calcif. Tissue Int. 52, 411–414. doi: 10.1007/BF00571327
Higgins, J. P. T., and Green, S. (2008). Cochrane Handbook for Systematic Reviews of Interventions. Chichester; West Sussex: The Cochrane Collaboration; John Wiley & Sons. doi: 10.1002/9780470712184
Howe, T. E., Shea, B., Dawson, L. J., Downie, F., Murray, A., Ross, C., et al. (2011). Exercise for preventing and treating osteoporosis in postmenopausal women. Cochrane Database Syst. Rev. 6:Cd000333. doi: 10.1002/14651858.CD000333.pub2
Iwamoto, J., Takeda, T., and Ichimura, S. (2001). Effect of exercise training and detraining on bone mineral density in postmenopausal women with osteoporosis. J. Orthop. Sci. 6, 128–132. doi: 10.1007/s007760100059
Jessup, J. V., Horne, C., Vishen, R. K., and Wheeler, D. (2003). Effects of exercise on bone density, balance, and self-efficacy in older women. Biol. Res. Nurs. 4, 171–180. doi: 10.1177/1099800402239628
Karakiriou, S., Douda, H., Smilios, I., Volaklis, K. A., and Tokmakidis, S. P. (2012). Effects of vibration and exercise training on bone mineral density and muscle strength in post-menopausal women. Eur. J. Sport Sci. 12, 81–88. doi: 10.1080/17461391.2010.536581
Kelley, G. A. (1998a). Aerobic exercise and bone density at the hip in postmenopausal women: a meta-analysis. Prev. Med. 27, 798–807. doi: 10.1006/pmed.1998.0360
Kelley, G. A. (1998b). Exercise and regional bone mineral density in postmenopausal women: a meta-analytic review of randomized trials. Am. J. Phys. Med. Rehabil. 77, 76–87. doi: 10.1097/00002060-199801000-00015
Kemmler, W. (1999). Einfluß unterschiedlicher lebensabschnitte auf die belastungsabhängige reaktion ossärer risikofaktoren einer osteoporose. Dtsch. Z Sportmed. 50, 114–119.
Kemmler, W., Bebenek, M., Kohl, M., and Von Stengel, S. (2015). Exercise and fractures in postmenopausal women. Final results of the controlled erlangen fitness and osteoporosis prevention study (EFOPS). Osteoporos. Int. 26, 2491–2499. doi: 10.1007/s00198-015-3165-3
Kemmler, W., Bebenek, M., von Stengel, S., Engelke, K., and Kalender, W. A. (2013). Effect of block-periodized exercise training on bone and coronary heart disease risk factors in early post-menopausal women: a randomized controlled study. Scand. J. Med. Sci. Sports 23, 121–129. doi: 10.1111/j.1600-0838.2011.01335.x
Kemmler, W., Lauber, D., Weineck, J., Hensen, J., Kalender, W., and Engelke, K. (2004). Benefits of 2 years of intense exercise on bone density, physical fitness, and blood lipids in early postmenopausal osteopenic women: results of the erlangen fitness osteoporosis prevention study (EFOPS). Arch. Intern. Med. 164, 1084–1091. doi: 10.1001/archinte.164.10.1084
Kemmler, W., and von Stengel, S. (2013). Exercise frequency, health risk factors, and diseases of the elderly. Arch. Phys. Med. Rehabil. 94, 2046–2053. doi: 10.1016/j.apmr.2013.05.013
Kemmler, W., and von Stengel, S. (2019). “Chapter 20 - the role of exercise on fracture reduction and bone strengthening,” in Muscle and Exercise Physiology, ed J. A. Zoladz (Academic Press), 433–455. doi: 10.1016/B978-0-12-814593-7.00020-7
Kemmler, W., von Stengel, S., Engelke, K., Haberle, L., and Kalender, W. A. (2010). Exercise effects on bone mineral density, falls, coronary risk factors, and health care costs in older women: the randomized controlled senior fitness and prevention (SEFIP) study. Arch. Intern. Med. 170, 179–185. doi: 10.1001/archinternmed.2009.499
Kemmler, W., von Stengel, S., and Kohl, M. (2016). Exercise frequency and bone mineral density development in exercising postmenopausal osteopenic women. Is there a critical dose of exercise for affecting bone? Results of the erlangen fitness and osteoporosis prevention study. Bone 89, 1–6. doi: 10.1016/j.bone.2016.04.019
Kerr, D., Ackland, T., Maslen, B., Morton, A., and Prince, R. (2001). Resistance training over 2 years increases bone mass in calcium-replete postmenopausal women. J. Bone Miner. Res. 16, 175–181. doi: 10.1359/jbmr.2001.16.1.175
Kerr, D., Morton, A., Dick, I., and Prince, R. (1996). Exercise effects on bone mass in postmenopausal women are site-specific and load-dependent. J. Bone. Miner. Res. 11, 218–225. doi: 10.1002/jbmr.5650110211
Kohrt, W. M., Ehsani, A. A., and Birge, S. J. Jr. (1997). Effects of exercise involving predominantly either joint-reaction or ground-reaction forces on bone mineral density in older women. J. Bone Miner. Res. 12, 1253–1261. doi: 10.1359/jbmr.1997.12.8.1253
Kohrt, W. M., Snead, D. B., Slatopolsky, E., and Birge, S. J. Jr. (1995). Additive effects of weight-bearing exercise and estrogen on bone mineral density in older women. J. Bone Miner. Res. 10, 1303–1311. doi: 10.1002/jbmr.5650100906
Korpelainen, R., Keinanen-Kiukaanniemi, S., Heikkinen, J., Vaananen, K., and Korpelainen, J. (2006). Effect of impact exercise on bone mineral density in elderly women with low BMD: a population-based randomized controlled 30-month intervention. Osteoporos. Int. 17, 109–118. doi: 10.1007/s00198-005-1924-2
Kwon, Y., Park, S., Kim, E., and Park, J. (2008). The effects of multi-component exercise training on VO2max, muscle mass, whole bone mineral density and fall risk in community-dwelling elderly women Jpn. J. Phys. Fitness Sport Med. 57, 339–348. doi: 10.7600/jspfsm.57.339
Lau, E. M., Woo, J., Leung, P. C., Swaminathan, R., and Leung, D. (1992). The effects of calcium supplementation and exercise on bone density in elderly Chinese women. Osteoporos. Int. 2, 168–173. doi: 10.1007/BF01623922
Liu, B. X., Chen, S. P., Li, Y. D., Wang, J., Zhang, B., Lin, Y., et al. (2015). The effect of the modified eighth section of eight-section brocade on osteoporosis in postmenopausal women: a prospective randomized trial. Medicine 94:e991. doi: 10.1097/MD.0000000000000991
Lord, S. R., Ward, J. A., Williams, P., and Zivanovic, E. (1996). The effects of a community exercise program on fracture risk factors in older women. Osteoporos. Int. 6, 361–367. doi: 10.1007/BF01623009
Maddalozzo, G. F., Widrick, J. J., Cardinal, B. J., Winters-Stone, K. M., Hoffman, M. A., and Snow, C. M. (2007). The effects of hormone replacement therapy and resistance training on spine bone mineral density in early postmenopausal women. Bone 40, 1244–1251. doi: 10.1016/j.bone.2006.12.059
Marques, E. A., Mota, J., and Carvalho, J. (2011a). Exercise effects on bone mineral density in older adults: a meta-analysis of randomized controlled trials. Age 34, 1493–1515. doi: 10.1007/s11357-011-9311-8
Marques, E. A., Mota, J., Machado, L., Sousa, F., Coelho, M., Moreira, P., et al. (2011b). Multicomponent training program with weight-bearing exercises elicits favorable bone density, muscle strength, and balance adaptations in older women. Calcif. Tissue Int. 88, 117–129. doi: 10.1007/s00223-010-9437-1
Marques, E. A., Wanderley, F., Machado, L., Sousa, F., Viana, J. L., Moreira-Goncalves, D., et al. (2011c). Effects of resistance and aerobic exercise on physical function, bone mineral density, OPG and RANKL in older women. Exp. Gerontol. 46, 524–532. doi: 10.1016/j.exger.2011.02.005
Martin, D., and Notelovitz, M. (1993). Effects of aerobic training on bone mineral density of postmenopausal women. J. Bone Miner. Res. 8, 931–936. doi: 10.1002/jbmr.5650080805
Martyn-St James, M., and Carroll, S. (2011). Effects of different impact exercise modalities on bone mineral density in premenopausal women: a meta-analysis. J. Bone Miner. Metab. 28, 251–267. doi: 10.1007/s00774-009-0139-6
Martyn-St. James, M., and Caroll, S. (2006). High intensity exercise training and postmenopausal bone loss: a meta-analysis. Osteoporos. Int. 17, 1225–1240. doi: 10.1007/s00198-006-0083-4
Milliken, L. A., Going, S. B., Houtkooper, L. B., Flint-Wagner, H. G., Figueroa, A., Metcalfe, L. L., et al. (2003). Effects of exercise training on bone remodeling, insulin-like growth factors, and bone mineral density in postmenopausal women with and without hormone replacement therapy. Calcif. Tissue Int. 72, 478–484. doi: 10.1007/s00223-001-1128-5
Milojevic, M., Sousa-Uva, M., Durko, A. P., and Head, S. J. (2018). Mixing 'apples and oranges' in meta-analytic studies: dangerous or delicious? Eur. J. Cardiothorac. Surg. 53, 1294–1298. doi: 10.1093/ejcts/ezx471
Moher, D., Shamseer, L., Clarke, M., Ghersi, D., Liberati, A., Petticrew, M., et al. (2015). Preferred reporting items for systematic review and meta-analysis protocols (PRISMA-P) 2015 statement. Syst. Rev. 4:1. doi: 10.1186/2046-4053-4-1
Moreira, L. D., Fronza, F. C., Dos Santos, R. N., Zach, P. L., Kunii, I. S., Hayashi, L. F., et al. (2014). The benefits of a high-intensity aquatic exercise program (HydrOS) for bone metabolism and bone mass of postmenopausal women. J. Bone Miner. Metab. 32, 411–419. doi: 10.1007/s00774-013-0509-y
Nelson, M. E., Fiatarone, M. A., Morganti, C. M., Trice, I., Greenberg, R. A., and Evans, W. J. (1994). Effects of high-intensity strength training on multiple risk factors for osteoporotic fractures. A randomized controlled trial. JAMA 272, 1909–1914. doi: 10.1001/jama.272.24.1909
Nelson, M. E., Fisher, E. C., Dilmanian, F. A., Dallal, G. E., and Evans, W. J. (1991). A 1-y walking program and increased dietary calcium in postmenopausal women: effects on bone. Am. J. Clin. Nutr. 53, 1304–1311. doi: 10.1093/ajcn/53.5.1304
Nichols, J., Nelson, K., Peterson, K., and Sartoris, D. (1995). Bone mineral density responses to high-intensity strength training in active older women. J. Aging Phys. Act. 3, 26–38. doi: 10.1123/japa.3.1.26
Nicholson, V. P., McKean, M. R., Slater, G. J., Kerr, A., and Burkett, B. J. (2015). Low-load very high-repetition resistance training attenuates bone loss at the lumbar spine in active post-menopausal women. Calcif. Tissue Int. 96, 490–499. doi: 10.1007/s00223-015-9976-6
Orsatti, F. N., Nahas-Neto, J., Orsatti, C. L., and Teixeira, A. S. (2013). Effects of isoflavone and counter-resistance training on bone mineral density in postmenopausal women. Rev. Bras. Cineantropom. Desempenho. Hum. 15, 726–736. doi: 10.5007/1980-0037.2013v15n6p726
Palacios, S., Henderson, V. W., Siseles, N., Tan, D., and Villaseca, P. (2010). Age of menopause and impact of climacteric symptoms by geographical region. Climacteric 13, 419–428. doi: 10.3109/13697137.2010.507886
Park, H., Kim, K. J., Komatsu, T., Park, S. K., and Mutoh, Y. (2008). Effect of combined exercise training on bone, body balance, and gait ability: a randomized controlled study in community-dwelling elderly women. J. Bone Miner. Metab. 26, 254–259. doi: 10.1007/s00774-007-0819-z
Prince, R., Devine, A., Dick, I., Criddle, A., Kerr, D., Kent, N., et al. (1995). The effects of calcium supplementation (milk powder or tablets) and exercise on bone density in postmenopausal women. J. Bone Miner. Res. 10, 1068–1075. doi: 10.1002/jbmr.5650100711
Pruitt, L. A., Jackson, R. D., Bartels, R. L., and Lehnhard, H. J. (1992). Weight-training effects on bone mineral density in early postmenopausal women. J. Bone Miner. Res. 7, 179–185. doi: 10.1002/jbmr.5650070209
Pruitt, L. A., Taaffe, D. R., and Marcus, R. (1995). Effects of a one-year high-intensity versus low-intensity resistance training program on bone mineral density in older women. J. Bone Miner. Res. 10, 1788–1795. doi: 10.1002/jbmr.5650101123
R Development Core Team (2019). A Language and Environment for Statistical Computing. Vienna: A Foundation for Statistical Computing.
Rhodes, E. C., Martin, A. D., Taunton, J. E., Donnelly, M., Warren, J., and Elliot, J. (2000). Effects of one year of resistance training on the relation between muscular strength and bone density in elderly women. Br. J. Sports Med. 34, 18–22. doi: 10.1136/bjsm.34.1.18
Ribeiro de Avila, V., Bento, T., Gomes, W., Leitao, J., and de Sousa, N. F. (2018). Functional outcomes and quality of life after ankle fracture surgically treated: a systematic review. J. Sport Rehabil. 27, 274–283. doi: 10.1123/jsr.2016-0199
Rubin, C. T., and Lanyon, L. E. (1985). Regulation of bone mass by mechanical strain magnitude. Calcif. Tissue Int. 37, 411–417. doi: 10.1007/BF02553711
Ryan, A. S., Nicklas, B. J., and Dennis, K. E. (1998). Aerobic exercise maintains regional bone mineral density during weight loss in postmenopausal women. J. Appl. Physiol. (1985) 84, 1305–1310. doi: 10.1152/jappl.1998.84.4.1305
Sakai, A., Oshige, T., Zenke, Y., Yamanaka, Y., Nagaishi, H., and Nakamura, T. (2010). Unipedal standing exercise and hip bone mineral density in postmenopausal women: a randomized controlled trial. J. Bone Miner. Metab. 28, 42–48. doi: 10.1007/s00774-009-0100-8
Sherrington, C., Herbert, R. D., Maher, C. G., and Moseley, A. M. (2000). PEDro. A database of randomized trials and systematic reviews in physiotherapy. Man. Ther. 5, 223–226. doi: 10.1054/math.2000.0372
Silverman, N. E., Nicklas, B. J., and Ryan, A. S. (2009). Addition of aerobic exercise to a weight loss program increases BMD, with an associated reduction in inflammation in overweight postmenopausal women. Calcif. Tissue Int. 84, 257–265. doi: 10.1007/s00223-009-9232-z
Sinaki, M., Wahner, H. W., Offord, K. P., and Hodgson, S. F. (1989). Efficacy of nonloading exercises in prevention of vertebral bone loss in postmenopausal women: a controlled trial. Mayo Clin. Proc. 64, 762–769. doi: 10.1016/S0025-6196(12)61748-0
Sterne, J. A., Sutton, A. J., Ioannidis, J. P., Terrin, N., Jones, D. R., Lau, J., et al. (2011). Recommendations for examining and interpreting funnel plot asymmetry in meta-analyses of randomised controlled trials. BMJ 343:d4002. doi: 10.1136/bmj.d4002
Sugiyama, T., Yamaguchi, A., and Kawai, S. (2002). Effects of skeletal loading on bone mass and compensation mechanism in bone: a new insight into the mechanostat theory. J. Bone Miner. Metab. 20, 196–200. doi: 10.1007/s007740200028
Tartibian, B., Hajizadeh Maleki, B., Kanaley, J., and Sadeghi, K. (2011). Long-term aerobic exercise and omega-3 supplementation modulate osteoporosis through inflammatory mechanisms in post-menopausal women: a randomized, repeated measures study. Nutr. Metab. 8:71. doi: 10.1186/1743-7075-8-71
Tella, S. H., and Gallagher, J. C. (2014). Prevention and treatment of postmenopausal osteoporosis. J. Steroid. Biochem. Mol. Biol. 142, 155–170. doi: 10.1016/j.jsbmb.2013.09.008
Toigo, M., and Boutellier, U. (2006). New fundamental resistance exercise determinants of molecular and cellular muscle adaptations. Eur. J. Appl. Physiol. 97, 643–663. doi: 10.1007/s00421-006-0238-1
Tolomio, S., Lalli, A., Travain, G., and Zaccaria, M. (2009). Effects of a combined weight-bearing and non-weight-bearing (warm water) exercise program on bone mass and quality in postmenopausal women with low bone-mineral density. Clin. Ter. 160, 105–109.
Umscheid, C. A., Margolis, D. J., and Grossman, C. E. (2011). Key concepts of clinical trials: a narrative review. Postgrad. Med. 123, 194–204. doi: 10.3810/pgm.2011.09.2475
Verschueren, S. M., Roelants, M., Delecluse, C., Swinnen, S., Vanderschueren, D., and Boonen, S. (2004). Effect of 6-month whole body vibration training on hip density, muscle strength, and postural control in postmenopausal women: a randomized controlled pilot study. J. Bone Miner. Res. 19, 352–359. doi: 10.1359/JBMR.0301245
Viechtbauer, W. (2010). Conducting meta-analyses in R with the metafor package. J. Stat. Softw. 36, 1–48. doi: 10.18637/jss.v036.i03
Wang, H., Yu, B., Chen, W., Lu, Y., and Yu, D. (2015). Simplified tai chi resistance training versus traditional tai chi in slowing bone loss in postmenopausal women. Evid. Based Complement Alternat. Med. 2015:379451. doi: 10.1155/2015/379451
Woo, J., Hong, A., Lau, E., and Lynn, H. (2007). A randomised controlled trial of tai chi and resistance exercise on bone health, muscle strength and balance in community-living elderly people. Age Ageing 36, 262–268. doi: 10.1093/ageing/afm005
Wu, J., Oka, J., Tabata, I., Higuchi, M., Toda, T., Fuku, N., et al. (2006). Effects of isoflavone and exercise on BMD and fat mass in postmenopausal Japanese women: a 1-year randomized placebo-controlled trial. J. Bone Miner. Res. 21, 780–789. doi: 10.1359/jbmr.060208
Yamazaki, S., Ichimura, S., Iwamoto, J., Takeda, T., and Toyama, Y. (2004). Effect of walking exercise on bone metabolism in postmenopausal women with osteopenia/osteoporosis. J. Bone Miner. Metab. 22, 500–508. doi: 10.1007/s00774-004-0514-2
Keywords: exercise, training, bone mineral density, BMD, post-menopausal women
Citation: Shojaa M, Von Stengel S, Schoene D, Kohl M, Barone G, Bragonzoni L, Dallolio L, Marini S, Murphy MH, Stephenson A, Mänty M, Julin M, Risto T and Kemmler W (2020) Effect of Exercise Training on Bone Mineral Density in Post-menopausal Women: A Systematic Review and Meta-Analysis of Intervention Studies. Front. Physiol. 11:652. doi: 10.3389/fphys.2020.00652
Received: 17 March 2020; Accepted: 22 May 2020;
Published: 23 June 2020.
Edited by:
Ronald F. Zernicke, University of Michigan, United StatesReviewed by:
Antonino Catalano, University of Messina, ItalyCopyright © 2020 Shojaa, Von Stengel, Schoene, Kohl, Barone, Bragonzoni, Dallolio, Marini, Murphy, Stephenson, Mänty, Julin, Risto and Kemmler. This is an open-access article distributed under the terms of the Creative Commons Attribution License (CC BY). The use, distribution or reproduction in other forums is permitted, provided the original author(s) and the copyright owner(s) are credited and that the original publication in this journal is cited, in accordance with accepted academic practice. No use, distribution or reproduction is permitted which does not comply with these terms.
*Correspondence: Mahdieh Shojaa, bWFoZGllaC5zaG9qYWFAaW1wLnVuaS1lcmxhbmdlbi5kZQ==
Disclaimer: All claims expressed in this article are solely those of the authors and do not necessarily represent those of their affiliated organizations, or those of the publisher, the editors and the reviewers. Any product that may be evaluated in this article or claim that may be made by its manufacturer is not guaranteed or endorsed by the publisher.
Research integrity at Frontiers
Learn more about the work of our research integrity team to safeguard the quality of each article we publish.