- 1Laboratory of Aquatic Animal Nutrition and Feed, Fisheries College, Guangdong Ocean University, Zhanjiang, China
- 2Guangdong Provincial Key Laboratory of Improved Variety Reproduction in Aquatic Economic Animals, Institute of Aquatic Economic Animals, School of Life Sciences, Sun Yat-sen University, Guangzhou, China
The aim of the present experiment was to evaluate the effects of Schizochytrium limacinum supplementation on the immune response, gut microbiota, and health of Penaeus monodon fed a low fish-meal (FM) diet. A diet containing 25% FM was used as a control (Diet A), and three other diets were formulated to contain 15% FM and supplemented with 0, 0.75, and 1.5% S. limacinum (Diet B, C, and D, respectively). The experiment was carried out in quadruplicates (30 shrimp per replicate, average weight 1.01 ± 0.01 g), and the shrimps were fed the test diets to apparent satiation three times daily for 8 weeks. Shrimp fed diet B and D showed lower weight gain than those fed diet A. Supplementation of 0.75% S. limacinum enhanced expression of antioxidative genes (superoxide dismutase and catalase) and immune-response-related genes in hepatopancreas but could not affect the gene expression of immune deficiency in hepatopancreas and Tube in the intestine. A low FM diet induced endoplasmic reticulum swelling of the intestinal epithelial cells, which was alleviated by S. limacinum supplementation. Ultra-performance liquid chromatography coupled with quadrupole time of flight mass spectrometry was employed to analyze the changes of hemolymph metabolomics, 49 significantly different metabolites were identified, and lysoPCs, deoxyinosine, inosine, and highly unsaturated fatty acids were lower in fish fed with low FM diets. Intestinal microbial diversity was lower in shrimp fed Diet B than those fed the control diet. Dietary supplementation of 0.75% S. limacinum increased intestinal microbial diversity of shrimp and decreased the ratio of pathogenic bacterium (Thalassotalea and Tenacibaculum). These results indicated that supplementing S. limacinum into a low FM diet improves the growth performance, immune response, and intestinal health of P. monodon. The optimum inclusion level of seems to be 0.75% of diet.
Introduction
Fish-meal (FM) is the most important dietary protein source for shrimp and carnivorous fish (Bendiksen et al., 2011; Olsen and Hasan, 2012; Xie et al., 2014). Due to the global expansion of aquaculture, the limited production of FM has become a restrictive factor for the speedy and sustainable development of aquaculture.
Penaeus monodon has been one of the most abundantly cultured shrimp species in the world, and it has an annual yield of over 700,000 tons (FAO, 2018). Despite this, research into FM replacement in P. monodon is much less than those in Litopenaeus vannamei (Amaya et al., 2007; Ye et al., 2011; Yue et al., 2012; Kuhn et al., 2016). In China, the country with the biggest shrimp production, FM levels in commercial shrimp feed are still very high (>15%) (Rahimnejad et al., 2018). Our previous research showed that supplementation of essential nutrients in low FM diets can prevent suppression of the immune response (Wang et al., 2009; Xie et al., 2016, 2018; Xie S. et al., 2019).
Schizochytrium limacinum is a microalgae, Xie J. et al. (2019) has reported that dietary supplementation of S. limacinum could improve the growth performance of golden pompano. Since S. limacinum is rich in docosahexaenoic acid (DHA), it has been tested as an alternative to fish oil in fish and shrimp feed (García-Ortega et al., 2016; Wang et al., 2017a, b). Docosahexaenoic acid has been shown to reduce oxidative stress, inhibite inflammation, and modulate apoptosis and autophagy in many studies (Vincentini et al., 2011; Kim et al., 2014; Jeromson et al., 2015). Due to the high content of DHA, S. limacinum is also reported to enhance the immune function of white shrimp and golden pompano (Non-wachai et al., 2010; Xie J. et al., 2019). To date, there is no report on the application of S. limacinum for P. monodon. Accordingly, this study was designed to evaluate whether supplementation of S. limacinum in a low FM diet could improve immune function and intestinal health of P. monodon.
Recently, increasing attention is being paid to the relationship between diet, gut microbiota, and host health (Shapiro et al., 2014; Rooks and Garrett, 2016). In this study, ultra-performance liquid chromatography coupled with quadrupole time of flight mass spectrometry (UPLC-QTOF-MS) was employed to evaluate the effects of dietary supplementation with S. limacinum on hemolymph metabolites profile of shrimp, and 16S rRNA was employed to explore the changes of intestinal microbiota induced by S. limacinum. UPLC-QTOF-MS and 16S rRNA were combined to find out the potential mechanism through which S. limacinum enhances the immune response in shrimp.
Materials and Methods
Ethics Statement
This study was carried out in accordance with the recommendations of Care and Use of Laboratory Animals in China, Animal Ethical and Welfare Committee of China Experimental Animal Society. The protocol was approved by the Animal Ethical and Welfare Committee of Sun Yat-sen University (Guangzhou, China).
Diet Preparation
Four practical diets were formulated, a 25% FM diet was used as a control (diet A), and three other diets were prepared by replacing 10% of FM with soy protein concentrate. Low FM diets were supplemented with 0, 0.75, and 1.5% S. limacinum (diet B, C and D, respectively). S. limacinum was provided by Kingdomway (Xiamen, China), which contained 19.4% DHA. Formulation and proximate composition of the diets are presented in Table 1, and fatty acid compositions of the diets are showed in Supplementary Table 1. Crystalline amino acids were mixed and precoated with 1% carboxymethyl cellulose to prevent leaching loss. The extra supplemented vitamins and minerals were dissolved in 200 mL water and then mixed with the dry ingredients. The 1.2 mm diameter pellets were cold-extruded using a pelletizer (Institute of Chemical Engineering, South China University of Technology, Guangdong, China), heated in an electric oven at 90°C for 60 min, then air-dried to approximately 10% moisture, and stored at -20°C until used.
Shrimp and Experimental Conditions
Juvenile P. monodon were obtained from the Shenzhen experimental base of South China Sea Fisheries Research Institute (Shenzhen, China). Prior to the experiment, shrimp were acclimated for 3 weeks and fed the commercial diet (25% FM). At the beginning of the experiment, 480 shrimp with average weight 1.01 ± 0.01 g were distributed randomly into 16 cylindrical fiberglass tanks (500 L), at a density of 30 shrimp in each tank. Each diet was randomly assigned to four tanks. All shrimp were fed to apparent satiation three times daily at 8:00, 13:00, and 18:00 for 8 weeks. Any uneaten feed was collected by siphoning and used to calculate feed intake.
During the experimental period, water temperatures ranged from 26 to 30°C, salinity was approximately 30‰, pH 7.7–8.0, ammonia nitrogen was lower than 0.05 mg L–1, and dissolved oxygen was higher than 6.5 mg L–1. Water quality parameters were measure by following the instructions of the detection kit (Sangpu, Beijing, China). Natural light-dark cycle was used during the trial.
Sample Collection and Chemical Analyses
After the 8 weeks feeding trial, shrimp from each tank were weighed, counted, and sampled 24 h after the last feeding. Eight shrimp per tank were used to collect hemolymph samples, and hemolymph were taken from the pericardial cavity using 1-ml syringe and then centrifuged (8,000 rpm) at 4°C for 10 min. Supernatant was pooled and stored at −80°C until analysis of the enzymatic activity and metabolomics. Hepatopancreas and intestine from two shrimp per tank were immediately flash-frozen in liquid nitrogen and stored at −80°C prior to RNA extraction.
Moisture, crude protein, and crude lipid contents in the diets were determined using standard methods (AOAC, 1995). Moisture was determined by drying at 105°C until constant weight. Crude protein was measured by the Kjeldahl method after acid digestion using an Auto Kjeldahl System (1030-Auto-analyzer; Tecator, Hoganos, Sweden). Crude lipid was determined by the ether-extraction method using a Soxtec System HT (Soxtec System HT6; Tecator, Sweden). Fatty acid compositions in diets were measured by China National Analytical Center (Guangzhou, China). Lipids were extracted by a mixture of chloroform and methanol (2:1, v/v), the lipid phase was evaporated, and fatty acids were then saponificated by potassium hydrate. Fatty acids methyl esters were separated and quanitified by a gas chromatograph (GC 7820A, Agilent, United States) equipmented with a HP-88 column (30 cm × 0.25 mm).
Hemolymph Biochemical Indices
Lipid-related metabolits and immune-related parameters in hemolymph were determined in this study. Total cholesterol (TC) contents were determined by cholesterol oxidase-peroxidase method (Allain et al., 1974). The contents of glutathione (GSH) were measured by its ability to react with DTNB (dithiobis-2-nitrobenzoic acid), and malondialdehyde (MDA) was measured by thiobarbituric acid method (Janero, 1990). The activities of superoxide dismutase (SOD) were determined by a hydroxylamine method (Masayasu and Hiroshi, 1979). All these parameters were determined following the instructions of the detection kit (Nanjing Jiancheng Bioengineering Institute, China), and they were measured by monitoring the absorbance changes in a microplate reader (TECAN infinite M200, Switzerland).
Intestinal Histological Examination
Hematoxylin/eosin (H&E) staining and transmission eletron microscopy (TEM) were employed to evaluate the intestinal damage of shrimp. The same section of middle intestine from two shrimp per tank were fixed in Bouin’s solution for 12 h and then fixed in 70% ethanol. All fixed samples were dehydrated in a graded series of ethyl alcohol and embedded in paraffin. Sections (4 μm thickness) were stained with H&E and observed under a light microscope (Nikon Ni-U, Japan).
Another two intestinal samples per tank were used for TEM examination. As described by Zhang et al. (2012), samples were firstly fixed in 2.5% glutaraldehyde solution and then fixed in 1% OsO4 for 1 h, dehydrated in a graded series of ethyl alcohol, and embedded with resin. Resin blocks were sectioned using a diamond knife. Ultrathin sections (∼90 nm) from each sample were cut and placed on copper grids. Sections were stained with saturated uranyl acetate solution for 30 min, rinsed with distilled water and poststained with lead citrate for 30 min. Ultrathin sections were then screened with a TEM (JEOL JEM-1400, Japan) at 150 kV.
Quantitative Real-Time PCR Analysis
Total RNA from hepatopancreas and intestine was extracted with RNAiso Plus reagent according to the manufacturer’s instructions (TaKaRa, Japan). Agarose gel electrophoresis and spectrophotometric analysis (A260:A280 nm) were used to assess RNA quality and concentration. cDNA was synthesized using a PrimeScriptTM RT reagent kit with gDNA Eraser (Takara, Japan), according to the manufacturer’s instructions.
Real-time PCR for the target genes were performed using a SYBR® Premix Ex TaqTM II (Takara, Japan) and quantified on the LightCycler 480 (Roche Applied Science, Basel Switzerland). As described by manufacturer’s introduction, 400 nM of forward- and reverse-specific primers, 10 ng of cDNA template, and nuclease-free water to a final volume of 10 μL were used. Then we used the following program: denaturation step at 95°C for 1 min, 40 amplification cycles of 5 s denaturation at 95°C, 15 s annealing at 60°C, 20 s extension at 72°C, a melt-curve analysis, and, finally, cooling to 4°C. The efficiency of primers for each gene was previously evaluated by serial dilutions to ensure that it was close to 100% (Supplementary Table 2).
Normalization of real-time qPCR data was done by geometric averaging of multiple internal reference genes (elongation factor 1α and β-actin) (Vandesompele et al., 2002). Three independent duplicates were conducted for each of the data.
UPLC-Q/TOF-MS Analysis
In total, 48 shrimp hemolymph samples were analyzed by UPLC-QTOF-MS in this research to investigate the impact of dietary S. limacinum on the lipid profiles of shrimp with 12 shrimp for each group. Briefly, 400 μL acetonitrile/methanol (9:1) was added to 100 μL hemolymph, and the mixture was vortexed for 30 s. The mixture was centrifuged at 13,000 g for 10 min at 4°C, and 200 μL supernatant was collected for UPLC-QTOF-MS analysis. Equal amounts of all samples were pooled as a QC sample for LC-MS system conditioning and quality control.
Ultra-performance liquid chromatography coupled with quadrupole time of flight mass spectrometry was performed on an ACQUITY UPLC system (Waters, Manchester, United Kingdom) coupled with a G2-Si HDMS QTOF mass spectrometer (Waters, Manchester, United Kingdom). Chromatographic separation was carried out at 40°C on an ACQUITY HSS T3 1-class column (2.1 mm × 100 mm, 1.8 μm, Waters). Mobile phase A consisted of 10 nM ammonium formate in water and 0.1% formic acid, and mobile phase B consisted of 10 nM ammonium formate in IPA/ACN (70/30) and 0.1% formic acid. The flow rate was 0.4 mL+/min. From the start to 0.5 min, B was held at 2%, linearly increased to 40% during the next 1 min, increased to 45% during the next 3.5 min, increased to 55% during the next 3 min, increased to 85% during the next 6 min, increased to 99% during the next 6 min, kept constant for 3 min, decreased to 2% in 0.1 min, and then kept constant for 2.9 min. Both positive and negative modes were performed and operated in continuum mode with an acquisition time of 0.3 s per scan. The scan range was set at 50–1500 m/z. The capillary was set at 2.5and 2 kV in positive ion mode and negative ion mode, respectively. Sampling cone voltages were set at 40 V in both modes. The desolvation temperature and gas flow were 350°C and 700 L/h, the source temperature was set at 120°C. Before analyzing the sample sequence, five QC samples were run, and, during the analysis of the sample sequence, one QC sample was run after every six injections.
The acquired mass data were imported to Waters Masslynx v4.1 software for peak detection and alignment. All of the data were normalized to the summed total ion intensity per chromatogram. We detected 4025 and 3233 features in positive and negative modes, respectively. All the features with CVs >30% were removed. The data were imported to SIMCA-P (Version 13.0, Umetrics, Sweden), where multivariate analysis were performe, mainly included principal component analysis (PCA) and orthogonal partial least squares discriminant analysis (OPLS-DA). The quality of the models is described by the R2 and Q2 values. R2 is defined as the proportion of variance in the data explained by the models and indicates goodness of fit. Q2 is defined as the proportion of variance in the data predicted by the model and indicates predictability, calculated by a cross-validation procedure. A default seven-round cross-validation in SIMCA-P was performed throughout to determine the optimal number of principal components and to avoid model overfitting.
Significantly different metabolites were selected on the basis of the combination of a statistically significant threshold of variable influence on projection (VIP) values obtained from the OPLS-DA model, P values from one way ANOVA analysis on the normalized peak areas, and the maximum fold change, where metabolites with VIP values larger than 1.0, P values less than 0.05, and fold change larger than 1.5 were included, respectively.
Metabolite identification was performed using Progenesis QI (Waters, Non-linear Dynamics, Newcastle, United Kingdom). LipidMaps, Chemspider and Human Metabolome databased (HMDB) were used for MS1 identification, and theoretical fragments were used for MS/MS identification. A score (total 60) of identified metabolites obtained from QI larger than 40 was regarded as acceptable, and anything else was regarded as uncertain.
Heatmap and cluster analyses were conducted using the pheatmap package based on R software (version 3.7.2).
16S rRNA Analysis
Total bacterial DNA in the intestine was extracted with a PowerFecal® DNA Isolation kit. DNA samples were amplificated (Sutton et al., 2013) and then quantified using Quant-iTTM dsDNA HS Reagent. High-throughput sequencing analysis of bacterial rRNA was performed by Illumina HiSeq 2500 at Biomarker Tchnologies Corporation (Beijing, China). The splicing and filtering of the raw data were performed using FLASH v1.2.7 and Trimmornatic v0.33, and cleaning of chimera were performed using UCHIME v4.2. OTUs were obtained by QIIME (version 1.8.0), Alpha diversity was evaluated by Mothur (version v.1.30). We used binary jaccard to estimate β diversity (QIIME).
Calculations and Statistical Analysis
The parameters were calculated as follows:
Percentage weight gain (WG, %) = 100 × (final body weight - initial body weight)/initial body weight
Feed efficiency (FE) = (final body weight - initial body weight)/feed consumed
Survival (%) = 100 × (final amount of shrimp)/(initial amount of shrimp)
The results are presented as the means ± SEM (standard error of the mean). All the data were statistically analyzed by SPSS 19.0 (SPSS, Chicago, IL, United States). The data were first tested for homogeneity; if the data had similar variances, then a one-way ANOVA was used to test the main effect of dietary treatment. When there were significant differences (P < 0.05), the group means were further compared using Duncan’s multiple range test. When data did not have a homogeneous viaration, the non-parameter Kruskal–Wallis test was applied and followed by all pairwise multiple comparisons if the results of Kruskal–Wallis test shown significant difference (P < 0.05).
Results
Growth Performance
The final body weight (FBW), WG, and FE were higher in shrimp fed diet A than those fed diet B and D (P < 0.05; Figure 1), and there were no differences between group A and C. Survival was similar between all the groups (P > 0.05).
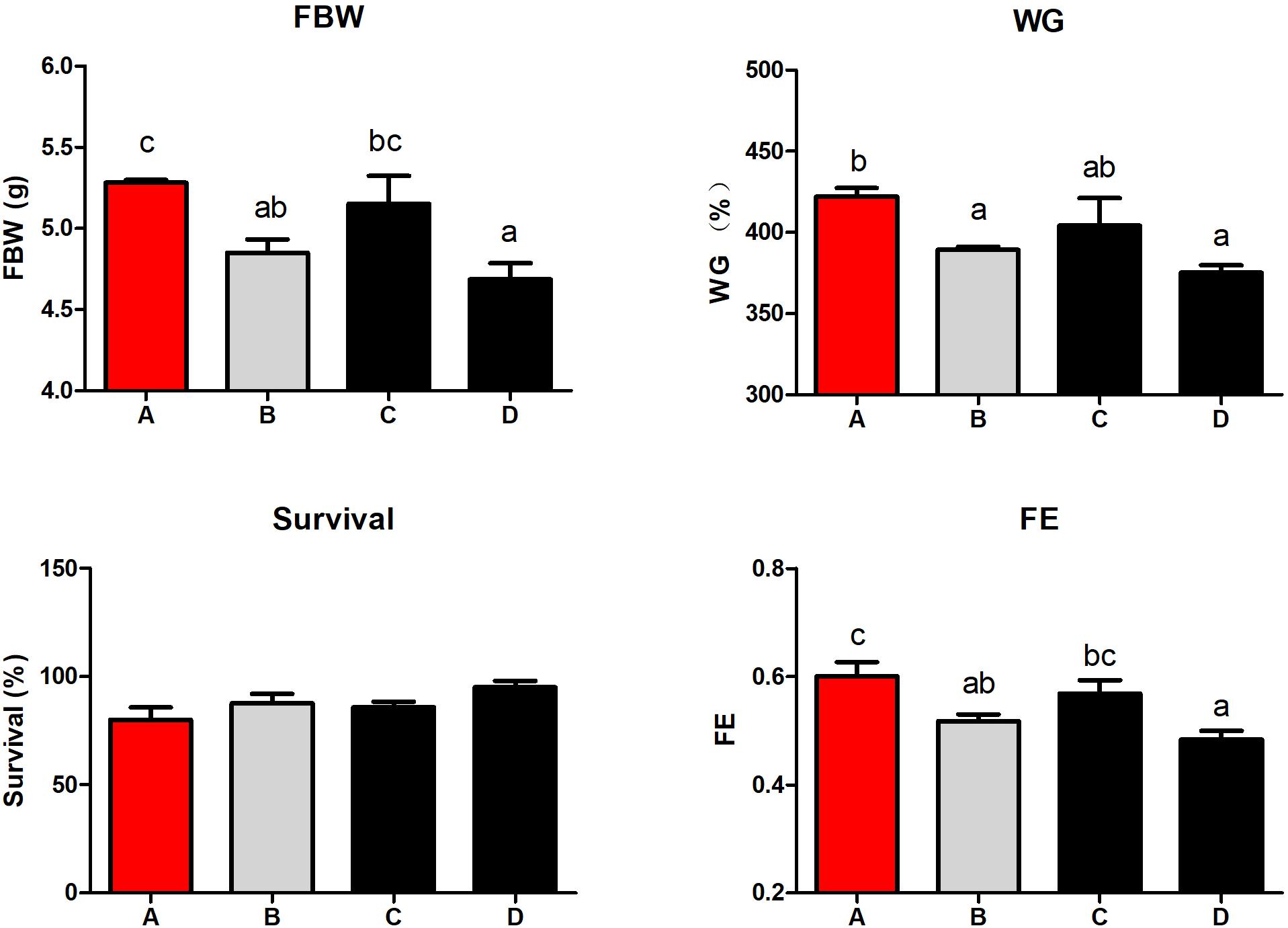
Figure 1. The effect of different diets on growth performance and feed utilization of shrimp. Values are means, with standard error represented by vertical bars. Mean values with different letters are significantly different (P < 0.05). Diet A, 25% fish meal; diet B, 15% fish meal; diet C, 15% fish meal + 0.75% Schizochytrium Limacinum; diet D, 15% fish meal + 1.5% Schizochytrium Limacinum. FBW, final body weight; WG, percentage weight gain; FE, feed efficiency.
Biochemical Parameters of Hemolymph and Hepatopancreas
Hemolymph TC content in shrimp fed diet B was higher than those fed diets containing S. limacinum (P < 0.05; Figure 2). S. limacinum supplementation significantly increased hemolymph MDA content of shrimp (P < 0.05). The GSH content in hepatopancreas was higher in group C than the other groups (P < 0.05). The SOD activities in hemolymph and hepatopancreas showed no significant differences between the four groups, the MDA contents in hepatopancreas showed a similar trend (P > 0.05).
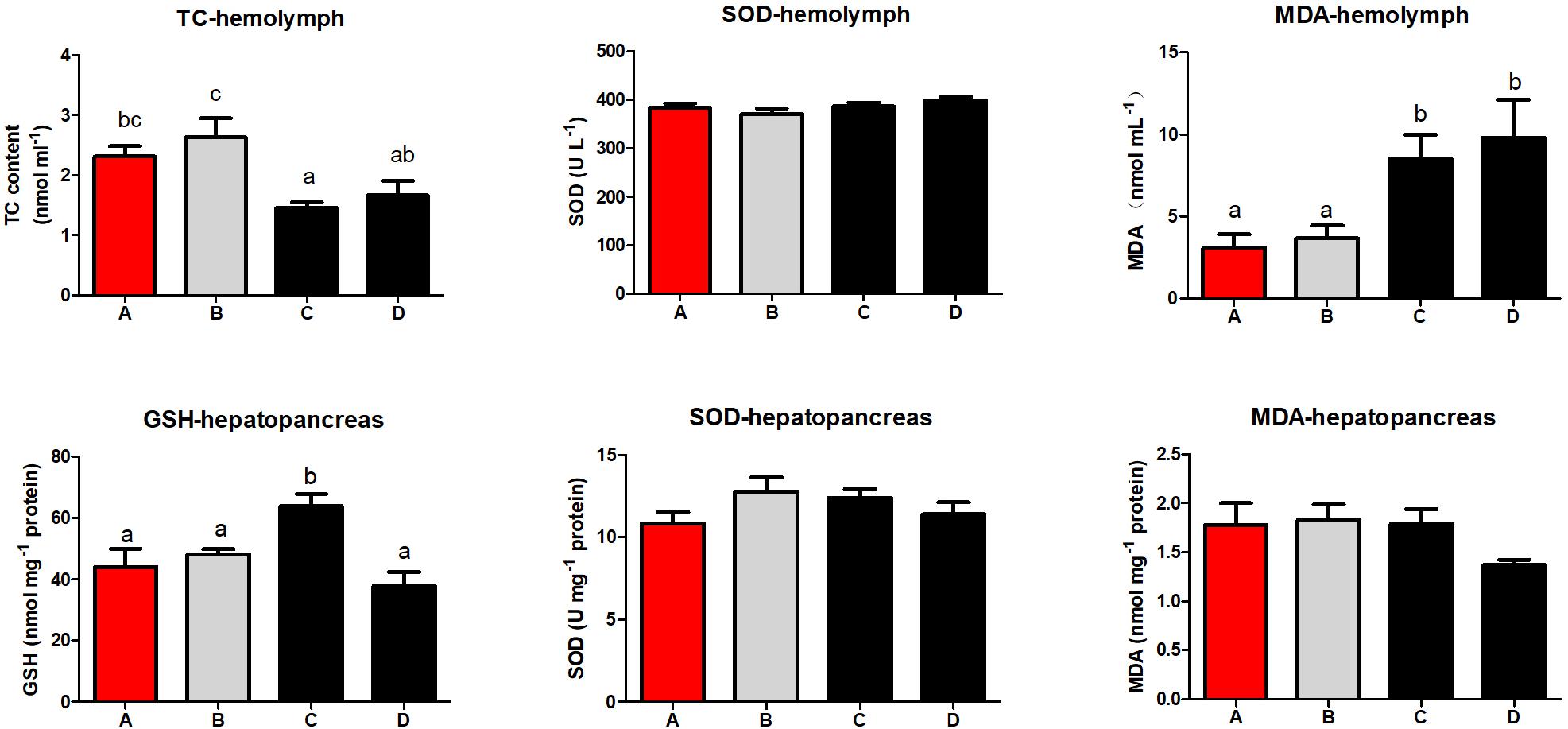
Figure 2. Effect of different diets on hemolymph and hepatopancreas physiologique index of Penaeus monodon. TC, total cholesterol; SOD, superoxide dismutase; MDA, malonaldehyde; GSH, glutathione.
Hepatopancreatic Immune Response
Low FM diet suppressed mRNA expression of immune deficiency (IMD) and endoplasmic reticulum protein57 (ERP57) in the hepatopancreas (P < 0.05), and supplementation of S. limacinum had no effect on their expression (Figure 3). However, S. limacinum supplementation alleviated the low expression of SOD and inhibitor of apoptosis proteins (IAP) induced by a low FM diet (P < 0.05). Shrimp fed diets containing S. limacinum obtained higher catalase (CAT), myeloid differentiation primary response gene 88 (MyD88), and autophagy-related protein 8 (ATG8) mRNA expression compared to those fed other diets (P < 0.05). Shrimp fed diet C obtained the highest mRNA expression of heat shock protein 70 (HSP70), Relish, X-box binding protein 1 (XBP1), defender against cell death 1 (DAD1), and ubiquitin conjugated enzyme 2 (UCE2) between all the groups. The mRNA level of Tube in group D was higher than that of other groups.
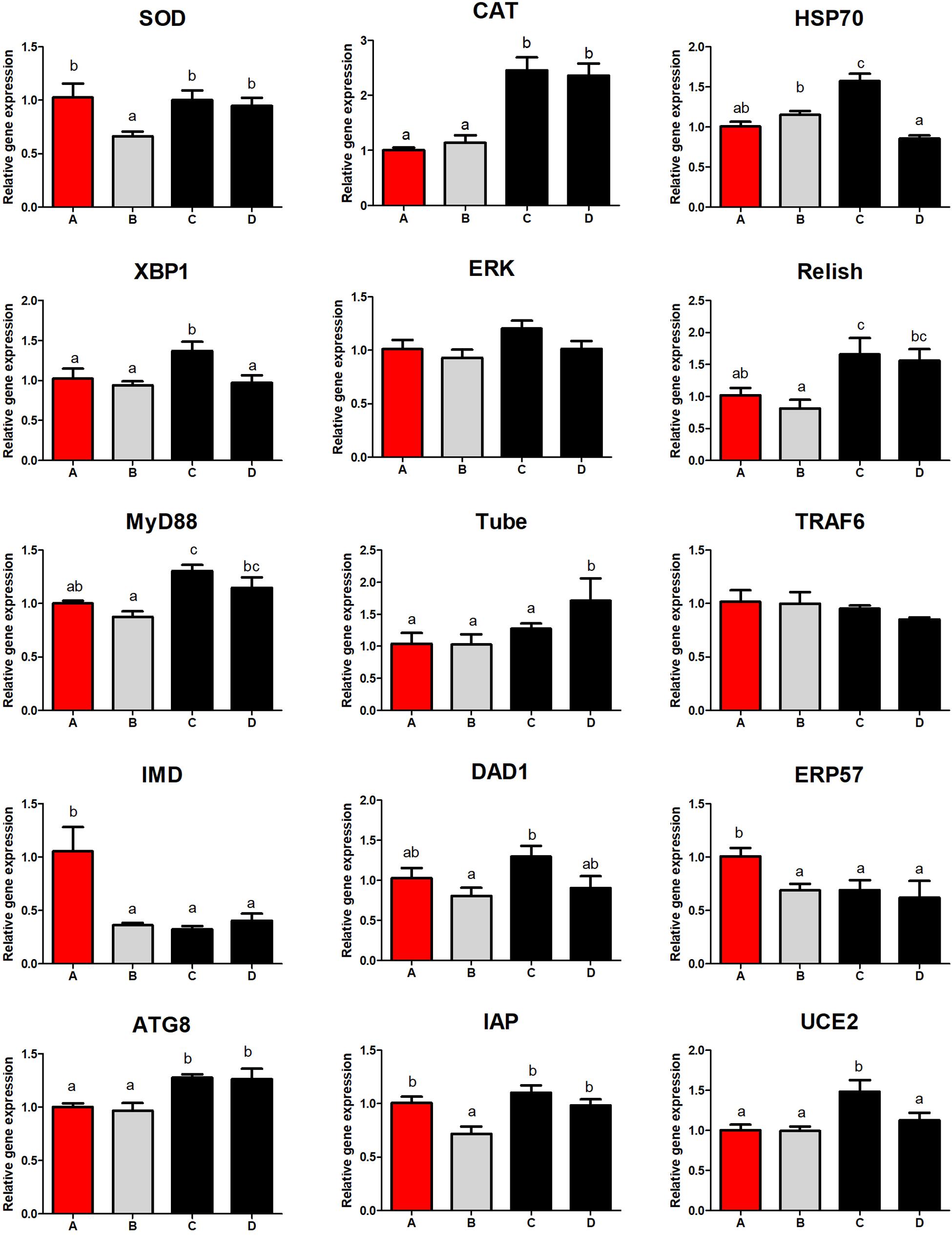
Figure 3. Effect of different diets on hepatopancreas immune response of Penaeus monodon. SOD, superoxide dismutase; IAP, inhibitor of apoptosis proteins; IMD, immune deficiency; ERP57, endoplasmic reticulum protein57; CAT, catalase; MyD88, myeloid differentiation primary response gene 88; ATG8, autophagy-related protein 8; ERK, extracellular signal-regulated kinase; HSP70, heat shock protein 70; XBP1, X-box binding protein 1; DAD1, defender against cell death 1; UCE2, ubiquitin conjugated enzyme 2.
Intestinal Immune Response
Shrimp fed diet B obtained lower Relish and higher XBP1 expression than those fed other diets (P < 0.05; Figure 4). Superoxide dismutase, extracellular signal-regulated kinase (ERK), Toll, and IAP mRNA levels in the intestine were lowest in shrimp fed diet D (P < 0.05). Low dietary FM downregulated the mRNA expression of Tube (P < 0.05). There were no differences in the expression of HSP70 and tumor necrosis factor receptor associated factor 6 (TRAF6) among all the treatment groups (P > 0.05).
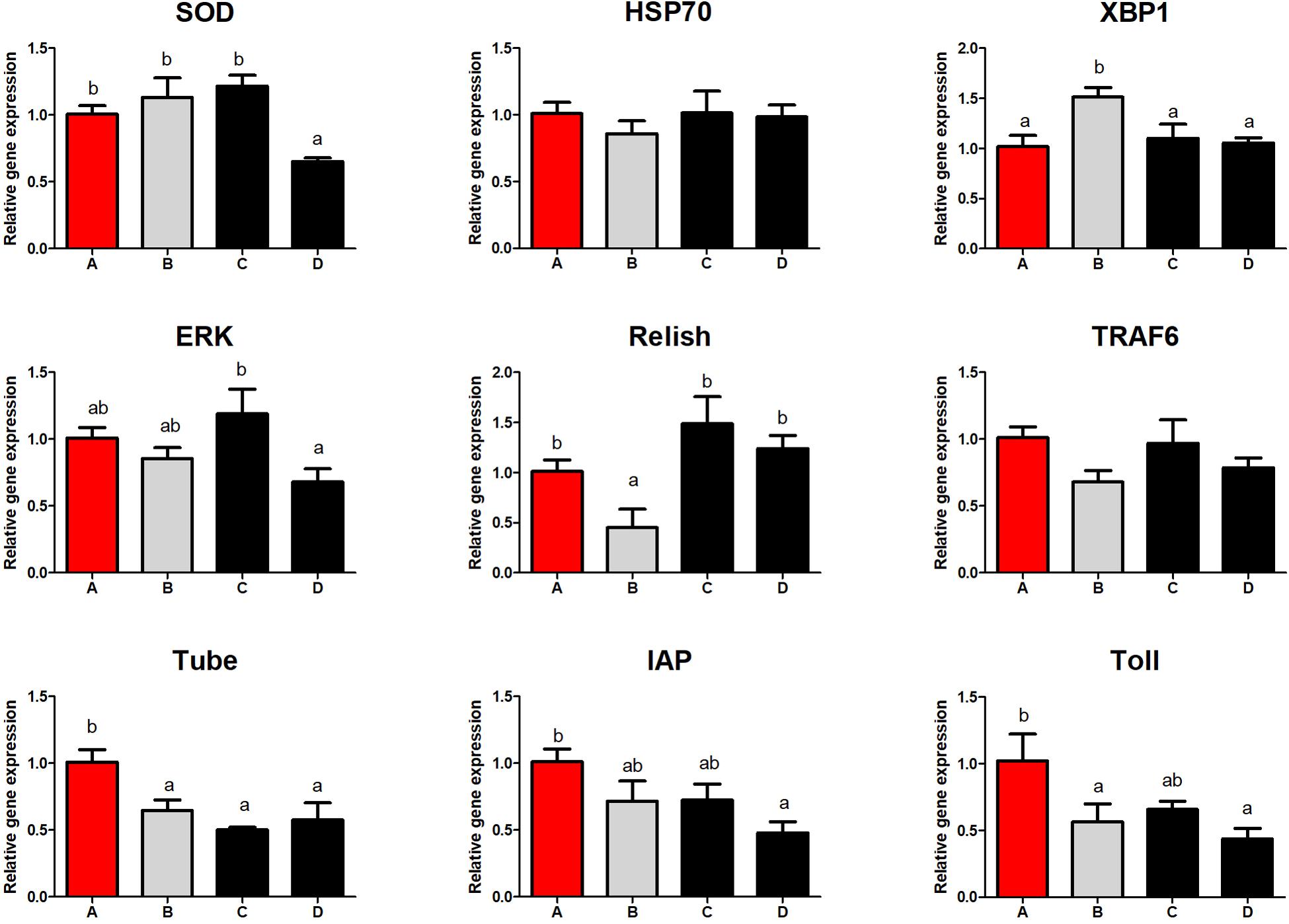
Figure 4. Effect of different diets on intestinal immune response of Penaeus monodon. SOD, superoxide dismutase; IAP, inhibitor of apoptosis proteins; ERK, extracellular signal-regulated kinase; HSP70, heat shock protein 70; XBP1, X-box binding protein 1; TRAF6, tumor necrosis factor receptor associated factor 6.
Intestinal Histology
Intestinal H&E staining showed that intestinal morphology were adversely affected by diet B (Figure 5), the intestinal fold height was lower in shrimp fed diet B compared with those fed diets A and C (Figure 6). TEM analysis also demonstrated that the intestine suffered more serious damage in shrimp fed diet B (Figure 7), especially the endoplasmic reticulum (ER), which showed obvious swelling in group B. The ER back to normal after dietary supplementation with S. limacinum, and S. limacinum supplementation increased the microvilli length of shrimp (P < 0.05).
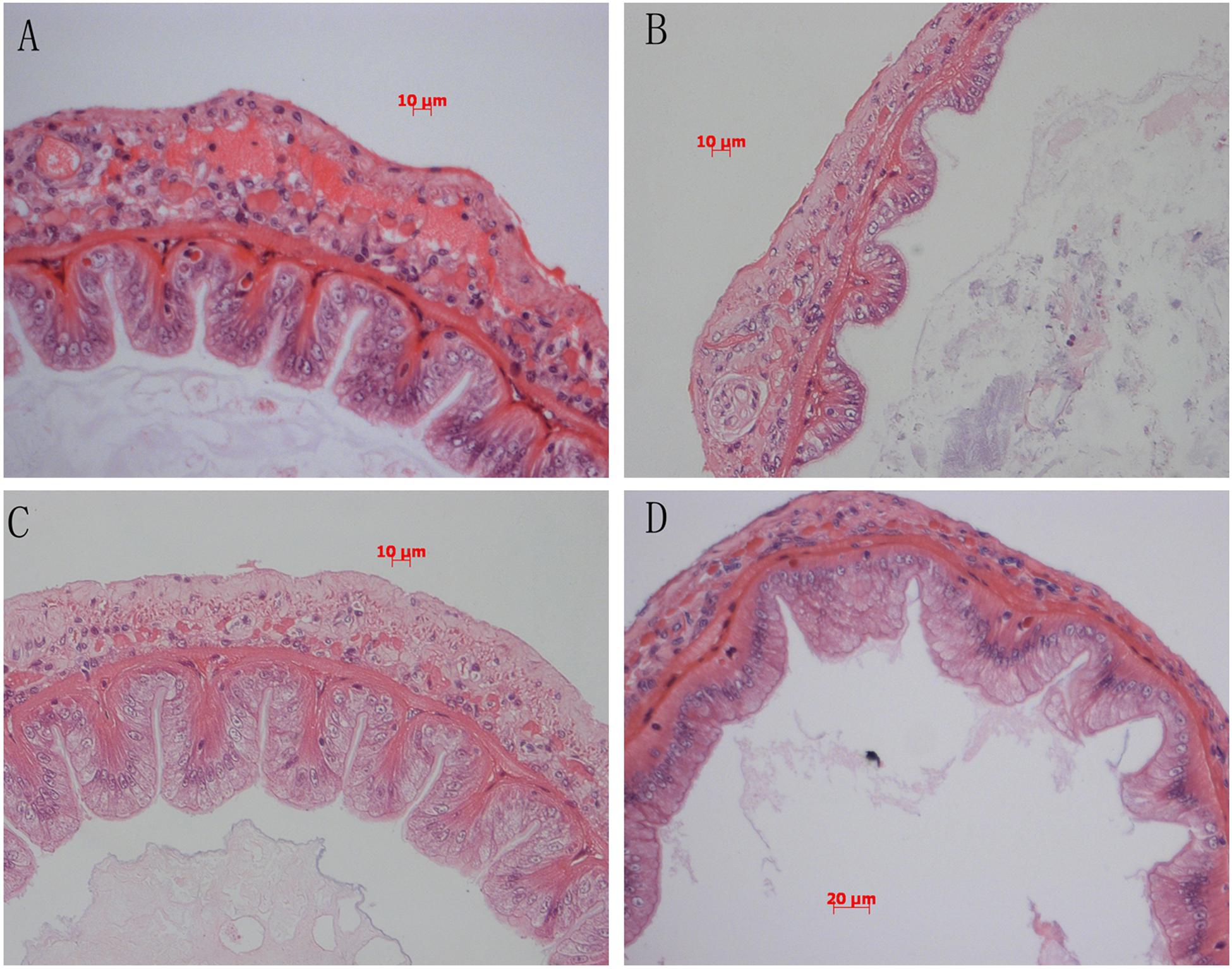
Figure 5. Effect of different diets on intestinal histology of Penaeus monodon. (A–D) Represent the intestinal histology of different groups.
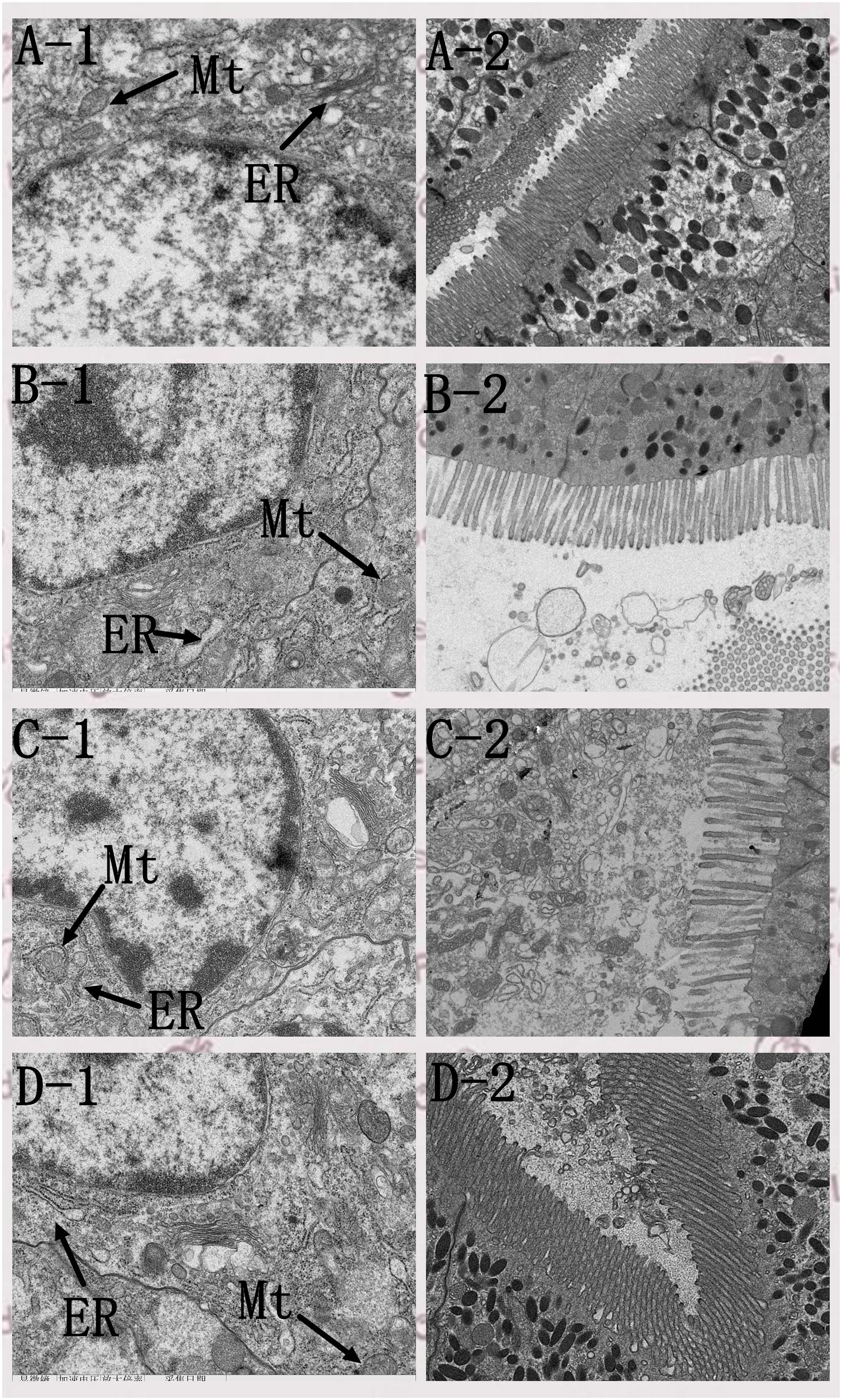
Figure 7. Effect of different diets on intestinal transmission electron microscope ultramicrotomy of Penaeus monodon. Mt, mitochondria; ER, endoplasmic reticulum. (A–D) Represent the intestinal histology of different groups. The number 1 represent the visual field of cell nucleus and organelle, the number 2 represent the visual field of microvilli.
Multivariate Analysis and Biomarker Identification
In this study, a non-targeted metabolomics strategy was applied. PCA was applied to evaluate the separation between the four groups, Figures 8A,B show a clear cluster in ESI+ (R2X = 0.982, Q2 = 0.911) and ESI- (R2X = 0.775, Q2 = 0.654) modes. A supervised multivariate data analysis method, OPLS-DA, was established for testing differences between features that clearly distinguished the different groups in ESI+ (R2X = 0.906, R2Y = 0.62, Q2 = 0.497) and ESI- (R2X = 0.791, R2Y = 0.97, Q2 = 0.923) modes (Figures 8C,D), showed a goodness of fit (R2) and predictive ability (Q2) in this model.
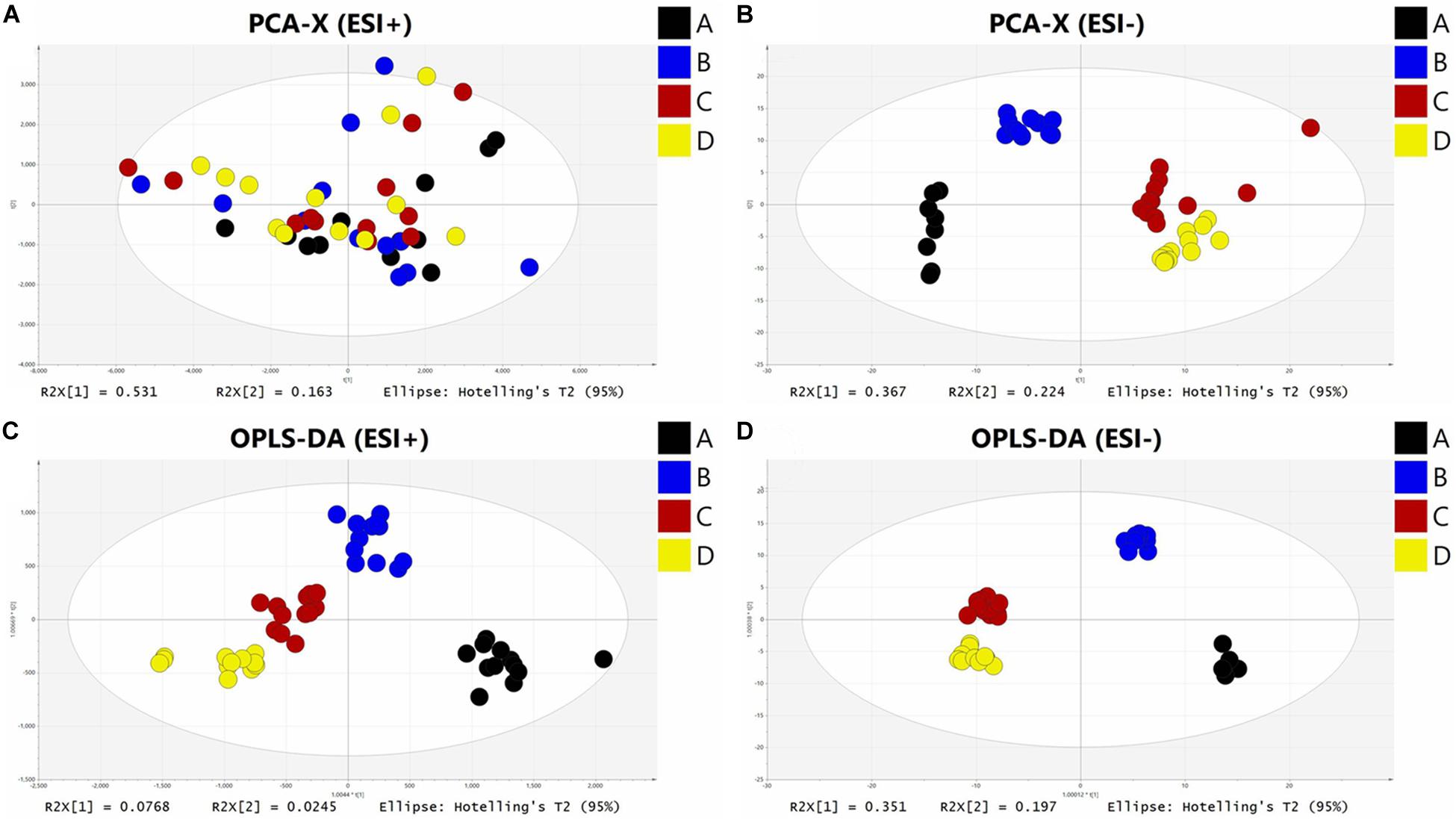
Figure 8. The PCA and OPLS-DA analysis of hemolymph samples in ESI + mode (A,C) and ESI- mode (B,D).
Features with a VIP value larger than 1.0, P value less than 0.05 and fold change larger than 1.2 were regarded as significantly different features. In total, 218 significant features were found to satisfy the criterion (119 in ESI+ and 99 in ESI-). Among them, 41 in ESI+ and 54 in ESI-were identified, 20 in ESI+ and 39 in ESI- were regarded as reliable. Therefore, 59 significantly different metabolites were identified in this experiment and are presented in Table 2.
Significantly Different Metabolites
A heatmap based on significantly different metabolites showed clear differences between shrimp fed diet A and other diets (Figure 9). Most of these metabolites were lipids, especially phospholipids, including phosphatidylcholine (PC), phosphatidyl ethanolamine (PE), Phosphatidyl serine (PS), sphingolipid and fatty acids. Shrimp fed high FM showed higher levels of lowly unsaturated LysoPCs (LPC) and highly unsaturated fatty acids [HUFAs; DHA, eicosapentaenoic acid (EPA), and arachidonic acid (ARA)] in the hemolymph, than other groups (P < 0.05). Meanwhile, S. limacinum supplementation in low FM diets could not alleviate this situation. The proportion of 2′-deoxyinosine and inosine were lower in shrimp fed low FM diets than those fed a high FM diet, and a similar tendency was found in the proportions of L-tryptophan and cholic acid (P < 0.05). S. limacinum supplementation increased the content of lysoPE (LPE; 22:6) and 6′-hydroxysiphonaxanthin in hemolymph. There were no clear trends found in the effects of different diets on unsaturated degree of phospholipids.
Intestinal Microbiota
A total of 3,027,778 high quality sequences were produced in this study, with an average of 189,236 sequences per sample. The coverage of each group exceeded 99%, which indicated that the 16S rRNA sequences identified in each group represented the majority of bacteria in the samples analyzed in this study. As shown in Table 3, the Shannon index was higher in shrimp fed a high FM diet compared to those fed diet B and D, which indicated the higher bacterial diversity in group A. There were no differences in OTUs numbers and community richness (Chao1 and ACE) between all the treatments. The analysis of PCoA showed that first two principal components explained 35.12% of the variations (Supplementary Figure 1).
The relative abundance of gut microbiota in each group at phylum level is shown in Supplementary Figure 2. The dominant phyla in the four groups were Proteobacteria, Bacteroidetes, Actinobacteria, Cyanobacteria, and Tenericutes. The relative abundance of Proteobacteria were exceeded 90% in all the groups. The relative abundance of Bacteroidetes, Actinobacteria, and Tenericutes were highest in shrimp fed diet A, while the relative abundance of Proteobacteria showed an opposite trend. The relative abundance of Cyanobacteria were highest in shrimp fed diet B and lowest in shrimp fed diet C.
The relative abundance of gut microbiota in each group at genus level is shown in Supplementary Figure 3. The dominant genera in four groups were Vibrio, Pseudoalteromonas, Photobacterium, Halocynthiibacter, and Shimia. The relative abundance of Vibrio was lower in shrimp fed the high FM diet, compared with those fed low FM diets (P < 0.05). The relative abundance of Silicimonas, Hahella, and Erythrobacter were higher in shrimp fed the high FM diet compared to those fed low FM diets (P < 0.05; Figure 10). The relative abundance of Thalassotalea and Tenacibaculum were higher in group B compared with that of the other groups (P < 0.05), while the relative abundance of Maribacter showed an opposite tendency. S. limacinum supplementation increased the relative abundance of Sphingomonas in the intestine.
Discussion
The results of this study indicated that weight gain of P. monodon decreased when dietary FM decreased from 25 to 15%, even though fish oil, amino acids, and micro-nutrients were supplemented into the low FM diet to balance the nutrition profile. It was earlier reported that dietary FM in P. monodon could be reduced from 35 to 17.5% by soy protein concentrate without negative impact on growth performance (Paripatananont et al., 2001). The different results may due to the differences in environment and prawn strain. Interestingly, in this study suitable S. limacinum supplementation in low FM diet improved growth of shrimp. Previous research mainly focused on fish oil replacement with S. limacinum in feed of L. vannamei (Wang et al., 2017c; Perez-Velazquez et al., 2018; Araújo et al., 2019), and similar research also were carried out in Epinephelus lanceolatus, Seriola rivoliana, and Sciaenops ocellatus (García-Ortega et al., 2016; Kissinger et al., 2016; Perez-Velazquez et al., 2018). Xie J. et al. (2019) reported 3% S. limacinum could improve the growth of Trachinotus ovatus. This is the first study to reveal the direct growth promoting effect of S. limacinum in shrimp. The high content of DHA, EPA, and micronutrients such as carotenoid may contribute to the growth promoting effect of S. limacinum (Xie J. et al., 2019). Although 0.75% S. limacinum enhanced the growth performance of P. monodon, 1.5% S. limacinum did not produce a similar effect. Previous research also reported that high levels of S. limacinum impaired the growth of white shrimp and blunt snout bream (Wang et al., 2017b, 2020). High levels of S. limacinum may induce an imbalance of dietary fatty acids composition, and this consequently results in suppression of shrimp growth.
Schizochytrium limacinum supplementation showed a large impact on antioxidative ability and immune response of P. monodon in this study. Superoxide dismutase and CAT are two important antioxidative related genes (Lu et al., 2017), supplementation of S. limacinum in low FM diet upregulated their expression, also increased the andioxidant (GSH) content in hepatopancreas. Similar result has also been observed in L. vannamei (Non-wachai et al., 2010). The antioxidative role of S. limacinum may due to the high content of DHA, previous research indicated that DHA can decrease oxidative stress of animals by changing the composition of cell membrane (Ye et al., 2010). Docosahexaenoic acid is also able to inhibit inflammation, its derivatives resolvins, protectins and maresins are involved in inflammatory processes such as cytokines production and lymphocyte activation (Ye et al., 2010; Zhao et al., 2015). ER stress and NF-κB have been well documented to be the main signaling pathways which DHA modulates the immune response (Begum et al., 2012; Crnkovic et al., 2012). Compared with group B, suitable S. limacinum supplementation upregulated the gene expression of XBP1 in hepatopancrease and downregulated its expression in intestine. XBP1 is the marker of ER stress (Sano and Reed, 2013), the results indicated that S. limacinum supplementation may alleviate the ER stress in shrimp. Relish, MyD88 and Tube are involved in NF-κB signaling pathway (Qiu et al., 2014), the present gene expression data indicated that NF-κB signaling pathway was modulated by S. limacinum. This study also found that autophagy (ATG8) and apoptosis (IAP and UCE2) related genes expression were affected by S. limacinum, which may be a downstream response of the NF-κB signaling pathway (Calder, 2015). However, the low expression of two critical innate immunity gene (IMD and Toll) induced by low FM diet could not be alleviated by S. limacinum supplementation, which revealed the limited benifical effects of S. limacinum. These results indicated that suitable supplementation of S. limacinum in a low FM diet could enhance the antioxidative ability of shrimp and improve the immune response of shrimp mainly through modulating the NF-κB related signaling pathways.
The intestine is a very important digestive organ, and intestinal health is closely related to the immune response of shrimp (Rooks and Garrett, 2016). In this study, the intestinal fold height and microvilli length were lower in shrimp fed diet B, which indicated that intestinal histology and epithelial cell ultrastructure of shrimp were damaged by high level of SPC. The damage of the intestine would have affected the digestion and absorption of nutrients, which may be the main cause of the compromised growth performance and immune response. Suitable supplementation of S. limacinum could alleviate endoplasmic reticulum swelling, and this result was consistent with the expression of ER stress and NF-κB signaling pathway related genes. In addition, suitable supplementation of S. limacinum also increased microvilli length and improved the overall intestinal health of shrimp, similar results were found in golden pompano (Xie J. et al., 2019).
The present results obtained through UPLC-QTOF-MS indicated that shrimp fed on a low FM diet showed fewer HUFAs in hemolymph than those fed a high FM diet, and it was unfortunate that S. limacinum supplementation could not increase hemolymph HUFAs content. Since the HUFAs play a critical role in immune response, the low hemolymph HUFAs in low FM groups may need to be improved in future studies. LysoPCs and LPE are derived from PC and PE, respectively, and have been considered as inflammatory lipids involved in several immune-mediated disease (Rindlisbacher et al., 2018), they also participate in cell signaling, energy metabolism and storage and other biological processes (Bai et al., 2014). In some studies, LPC and LPE have been found to aggravate inflammation associated diseases (Xie et al., 2020), and they were found to exert their effects through different signaling pathways, such as NF-κB, PKC, and ERK (Zeng et al., 2017). However, in other studies, LPC and LPE were found to decrease in inflammatory condition (Bai et al., 2014; Zhou et al., 2014; Qian et al., 2017; Wang et al., 2017a). These inconsistencies results of various research have indicated that the relationship between inflammation and levels of LPC and LPE may depend on species and inflammation stage. In this study, decreased LPCs in low FM diet groups may be associated with inflammation, and S. limacinum supplementation had no benificial effect on these changes. Additionally, S. limacinum supplementation also had little influence on the low deoxyinosine, inosine, and cholic acid content induced by low FM diet. These results from UPLC-QTOF-MS revealed that S. limacinum supplementation enhancing the immune response may not through changing the metabolomics profile of shrimp.
There have been numerous studies that have evaluated the effects of different protein sources on the gut microbiota composition of aquatic animal (Zhou et al., 2018; Niu et al., 2019), and previous studies examining P. monodon evaluated the effect of marine invertebrate meals on gut microbiota (Simon et al., 2019). In this study, low dietary FM levels decreased the gut microbial diversity of shrimp and increased the ratio of harmful genera such as Vibrio, Pseudoalteromonas, and Photobacterium, which may be associated with the impaired intestinal health in shrimp. Similar to our results, a study in Atlantic salmon indicated that high content of soybean meal decreased the ratio of lactic acid, and increased the ratio of Aeromonas (Bakke-McKellep et al., 2007). Desai et al. (2012) also reported that plant ingredients changed the gut microbial composition, and the changes in gut microbiota may contribute to negative health outcomes. Thalassotalea and Tenacibaculum are two pathogenic bacterium found in aquatic animals (Costantini et al., 2017). S. limacinum supplementation in low FM diet significantly decreased their ratios in the intestine of shrimp, while S. limacinum had no effect on the ratios of Vibrio, Pseudoalteromonas, and Photobacterium. These results indicated that low dietary FM levels increased the ratio of harmful bacterial genera, S. limacinum supplementation could decrease some of these harmful bacterial genera (Thalassotalea and Tenacibaculum). Dietary supplementation of S. limacinum enhancing the immune response and intestinal health may through changing intestinal microbiota of shrimp.
In conclusion, a low FM diet decreased the weight gain of P. monodon, impaired the immune response, induced intestinal damage, and increased the ratio of harmful bacterial genera in shrimp. Dietary supplementation with 0.75% S. limacinum promoted growth performance of and improved the immune response and intestinal health of shrimp.
Data Availability Statement
The sequencing data has been deposited into the Sequence Read Archive (accession: PRJNA632684).
Ethics Statement
This study was carried out in accordance with the recommendations of Care and Use of Laboratory Animals in China, Animal Ethical and Welfare Committee of China Experimental Animal Society. The protocol was approved by the Animal Ethical and Welfare Committee of Sun Yat-sen University (Guangzhou, China).
Author Contributions
SX, JN, YL, and LT designed the experiments. SX and DW carried out the experiments. SX wrote the manuscript. SX and BT revised the manuscript. All authors read and approved the final version of the manuscript.
Funding
This work was supported by the fund of The National Key R&D Progrem of China (2019YFD0900200), Guangdong Basic and Applied Basic Research Foundation (2019A1515011970), and China Agriculture Research Systerm-48 (CARS 48).
Conflict of Interest
The authors declare that the research was conducted in the absence of any commercial or financial relationships that could be construed as a potential conflict of interest.
Acknowledgments
We would like to thank the participants for their time and commitment to this study.
Supplementary Material
The Supplementary Material for this article can be found online at: https://www.frontiersin.org/articles/10.3389/fphys.2020.00613/full#supplementary-material
References
Allain, C. C., Poon, L. S., Chan, C. S. G., Richmond, W. Y., and Fu, P. C. (1974). Enzymatic determination of total serum cholesterol. Clin. Chem. 20, 470–475.
Amaya, E. A., Davis, D. A., and Rouse, D. B. (2007). Replacement of fish meal in practical diets for the pacific white shrimp (litopenaeus vannamei) reared under pond conditions. Aquaculture 262, 393–401. doi: 10.1016/j.aquaculture.2006.11.015
AOAC (1995). Official Methods of Analysis of Official Analytical Chemists International, 16th Edn. Arlington, VA: Association of official analytical chemists.
Araújo, B. C., Mata-Sotres, J. A., Viana, M. T., Tinajero, A., and Braga, A. (2019). Fish oil-free diets for pacific white shrimp litopenaeus vannamei: the effects of dha-epa supplementation on juvenile growth performance and muscle fatty acid profile. Aquaculture 511:734276. doi: 10.1016/j.aquaculture.2019.734276
Bai, Y., Zhang, H., Sun, X., Sun, C., and Ren, L. (2014). Biomarker identification and pathway analysis by serum metabolomics of childhood acute lymphoblastic leukemia. Clin. Chim. Acta 436, 207–216. doi: 10.1016/j.cca.2014.05.022
Bakke-McKellep, A. M., Penn, M. H., Salas, P. M., Refstie, S., Sperstad, S., Landsverk, T., et al. (2007). Effects of dietary soyabean meal, inulin and oxytetracycline on intestinal microbiota and epithelial cell stress, apoptosis and proliferation in the teleost atlantic salmon (Salmo salar l.). Brit J Nutr. 97, 699–713. doi: 10.1017/S0007114507381397
Begum, G., Kintner, D., Liu, Y., Cramer, S. W., and Sun, D. (2012). Dha inhibits er ca2+ release and er stress in astrocytes following in vitro ischemia. J. Neurochem. 120, 622–630. doi: 10.1111/j.1471-4159.2011.07606.x
Bendiksen, E. A., Johnsen, C. A., Olsen, H. J., and Jobling, M. (2011). Sustainable aquafeeds: progress towards reduced reliance upon marine ingredients in diets for farmed atlantic salmon (Salmo salar l.). Aquaculture 314, 132–139.
Calder, P. C. (2015). Marine omega-3 fatty acids and inflammatory processes: effects, mechanisms and clinical relevance. Biochim. Biophys. Acta 1851, 469–484. doi: 10.1016/j.bbalip.2014.08.010
Costantini, L., Molinari, R., Farinon, B., and Merendino, N. (2017). Impact of omega-3 fatty acids on the gut microbiota. Int. J. Mol. Sci. 18:2645.
Crnkovic, S., Riederer, M., Lechleitner, M., Hallström, S., Malli, R., Graier, W. F., et al. (2012). Docosahexaenoic acid-induced unfolded protein response, cell cycle arrest, and apoptosis in vascular smooth muscle cells are triggered by ca2+-dependent induction of oxidative stress. Free Radical Bio Med. 52, 1786–1795. doi: 10.1016/j.freeradbiomed.2012.02.036
Desai, A. R., Links, M. G., Collins, S. A., Mansfield, G. S., Drew, M. D., Van Kessel, A. G., et al. (2012). Effects of plant-based diets on the distal gut microbiome of rainbow trout (Oncorhynchus mykiss). Aquaculture 35, 134–142. doi: 10.1016/j.aquaculture.2012.04.005
García-Ortega, A., Kissinger, K. R., and Trushenski, J. T. (2016). Evaluation of fish meal and fish oil replacement by soybean protein and algal meal from Schizochytrium limacinum in diets for giant grouper epinephelus lanceolatus. Aquaculture 452, 1–8. doi: 10.1016/j.aquaculture.2015.10.020
Janero, D. R. (1990). Malondialdehyde and thiobarbituric acid-reactivity as diagnostic indices of lipid peroxidation and peroxidative tissue injury. Free Radical Bio Med. 9, 515–540. doi: 10.1016/0891-5849(90)90131-2
Jeromson, S., Gallagher, J. I., Galloway, D. S., and Hamilton, L. D. (2015). Omega-3 fatty acids and skeletal muscle health. Mar. Drugs 13, 6977–7004. doi: 10.3390/md13116977
Kim, W., Barhoumi, R., McMurray, D. N., and Chapkin, R. S. (2014). Dietary fish oil and dha down-regulate antigen-activated cd4+ t-cells while promoting the formation of liquid-ordered mesodomains. Br. J. Nutr. 111, 254–260.
Kissinger, K. R., García-Ortega, A., and Trushenski, J. T. (2016). Partial fish meal replacement by soy protein concentrate, squid and algal meals in low fish-oil diets containing schizochytrium limacinum for longfin yellowtail seriola rivoliana. Aquaculture 452, 37–44. doi: 10.1016/j.aquaculture.2015.10.022
Kuhn, D. D., Lawrence, A. L., Crockett, J., and Taylor, D. (2016). Evaluation of bioflocs derived from confectionary food effluent water as a replacement feed ingredient for fishmeal or soy meal for shrimp. Aquaculture 454, 66–71.
Lu, K., Wang, L., Zhang, D., Liu, W., and Xu, W. (2017). Berberine attenuates oxidative stress and hepatocytes apoptosis via protecting mitochondria in blunt snout bream megalobrama amblycephala fed high-fat diets. Fish Physiol Biochem. 43, 65–76. doi: 10.1007/s10695-016-0268-5
Masayasu, M., and Hiroshi, Y. (1979). A simplified assay method of superoxide dismutase activity for clinical use. Clin. Chim. Acta 92, 337–342. doi: 10.1016/0009-8981(79)90211-0
Niu, J., Xie, J., Guo, T., Fang, H., Zhang, Y., Liao, S. Y., et al. (2019). Comparison and evaluation of four species of macro-algaes as dietary ingredients in litopenaeus vannamei under normal rearing and wssv challenge conditions: effect on growth, immune response, and intestinal microbiota. Front. Physiol. 9:1880. doi: 10.3389/fphys.2018.01880
Non-wachai, T., Purivirojkul, W., Limsuwan, C., Chuchird, N., Velasco, M., Dhar, A. K., et al. (2010). Growth, nonspecific immune characteristics, and survival upon challenge with vibrio harveyi in pacific white shrimp (Litopenaeus vannamei) raised on diets containing algal meal. Fish Shellfish Immun. 29, 298–304. doi: 10.1016/j.fsi.2010.04.009
Olsen, R. L., and Hasan, M. R. (2012). A limited supply of fishmeal: impact on future increases in global aquaculture production. Trends Food Sci. Tech. 27, 120–128.
Paripatananont, T., Boonyaratpalin, M., Pengseng, P., and Chotipuntu, P. (2001). Substitution of soy protein concentrate for fishmeal in diets of tiger shrimp penaeus monodon. Aquac. Res. 32, 369–374. doi: 10.1046/j.1355-557x.2001.00045.x
Perez-Velazquez, M., Gatlin, D. M., González-Félix, M. L., and García-Ortega, A. (2018). Partial replacement of fishmeal and fish oil by algal meals in diets of red drum sciaenops ocellatus. Aquaculture 487, 41–50. doi: 10.1016/j.aquaculture.2018.01.001
Qian, Y., Peng, Y., Shang, E., Zhao, M., Yan, L., Zhu, Z., et al. (2017). Metabolic profiling of the hepatotoxicity and nephrotoxicity of ginkgolic acids in rats using ultra-performance liquid chromatography-high-definition mass spectrometry. Chem. Biol. Interact. 273, 11–17. doi: 10.1016/j.cbi.2017.05.020
Qiu, W., Zhang, S., Chen, Y., Wang, P., Xu, X., Li, C. Z., et al. (2014). Litopenaeus vannamei NF-κB is required for wssv replication. Dev. Comp. Immunol. 45, 156–162. doi: 10.1016/j.dci.2014.02.016
Rahimnejad, S., Yuan, X., Wang, L., Lu, K., Song, K., Zhang, C., et al. (2018). Chitooligosaccharide supplementation in low-fish meal diets for pacific white shrimp (Litopenaeus vannamei): effects on growth, innate immunity, gut histology, and immune-related genes expression. Fish Shellfish Immun. 80, 405–415. doi: 10.1016/j.fsi.2018.06.025
Rindlisbacher, B., Schmid, C., Geiser, T., Bovet, C., and Funke-Chambour, M. (2018). Serum metabolic profiling identified a distinct metabolic signature in patients with idiopathic pulmonary fibrosis–a potential biomarker role for lysopc. Resp. Res. 19:7. doi: 10.1186/s12931-018-0714-2
Rooks, M. G., and Garrett, W. S. (2016). Gut microbiota, metabolites and host immunity. Nat. Rev. Immunol.16, 341–352.
Sano, R., and Reed, J. C. (2013). Er stress-induced cell death mechanisms. Biochim. Biophys. Acta Mol. Cell Res. 1833, 3460–3470. doi: 10.1016/j.bbamcr.2013.06.028
Shapiro, H., Thaiss, C. A., Levy, M., and Elinav, E. (2014). The cross talk between microbiota and the immune system: metabolites take center stage. Curr. Opin. Immunol 30, 54–62. doi: 10.1016/j.coi.2014.07.003
Simon, C. J., Truong, H. H., Noble, T. H., Osborne, S. A., Wynne, J. W., Wade, N. M., et al. (2019). Microbial biomass, marine invertebrate meals and feed restriction influence the biological and gut microbiota response of shrimp penaeus monodon. Aquaculture 2019:734679. doi: 10.1016/j.aquaculture.2019.734679
Sutton, N. B., Maphosa, F., Morillo, J. A., Abu Al-Soud, W., Langenhoff, A. A. M., Grotenhuis, T., et al. (2013). Impact of long-term diesel contamination on soil microbial community structure. Appl. Environ. Microb. 79:619. doi: 10.1128/AEM.02747-12
Vandesompele, J., De Preter, K., Pattyn, F., Poppe, B., Van Roy, N., De Paepe, A., et al. (2002). Accurate normalization of real-time quantitative rt-pcr data by geometric averaging of multiple internal control genes. Genome Biol. 3, h31–h34. doi: 10.1186/gb-2002-3-7-research0034
Vincentini, O., Quaranta, M. G., Viora, M., Agostoni, C., and Silano, M. (2011). Docosahexaenoic acid modulates in vitro the inflammation of celiac disease in intestinal epithelial cells via the inhibition of cpla2. Clin. Nutr. 30, 541–546. doi: 10.1016/j.clnu.2011.02.007
Wang, C., Liu, W., Huang, Y., Wang, X., Li, X., Zhang, D. D., et al. (2020). Dietary dha affects muscle fiber development by activating ampk/sirt1 pathway in blunt snout bream (Megalobrama amblycephala). Aquaculture 518:734835. doi: 10.1016/j.aquaculture.2019.734835
Wang, D., Yu, Y., and Zhao, B. (2009). Effects of cotton seed meal and rapeseed meal on growth performance, non-specific immune indexes and body compositions of Litopenaeus vannamei. China Feed. 23:12.
Wang, H., Zhou, J., Liu, Q. Z., Wang, L. L., and Shang, J. (2017a). Simvastatin and bezafibrate ameliorate emotional disorder induced by high fat diet in c57bl/6 mice. Sci. Rep. 7:2335. doi: 10.1038/s41598-017-02576-5
Wang, Y., Li, M., Filer, K., Xue, Y., Ai, Q., Mai, K., et al. (2017b). Evaluation of schizochytrium meal in microdiets of pacific white shrimp (Litopenaeus vannamei) larvae. Aquac. Res. 48, 2328–2336. doi: 10.1111/are.13068
Wang, Y., Li, M., Filer, K., Xue, Y., Ai, Q., Mai, K., et al. (2017c). Replacement of fish oil with a dha-rich schizochytrium meal on growth performance, activities of digestive enzyme and fatty acid profile of pacific white shrimp (Litopenaeus vannamei) larvae. Aquacult. Nutr. 23, 1113–1120. doi: 10.1111/anu.12479
Xie, J., Fang, H., Liao, S., Guo, T., Yin, P., Liu, Y., et al. (2019). Study on Schizochytrium sp. Improving the growth performance and non-specific immunity of golden pompano (Trachinotus ovatus) while not affecting the antioxidant capacity. Fish Shellfish Immun. 95, 617–623. doi: 10.1016/j.fsi.2019.10.028
Xie, S., Wei, D., Yin, P., Zheng, L., Guo, T., Liu, Y., et al. (2019). Dietary replacement of fish-meal impaired protein synthesis and immune response of juvenile pacific white shrimp, Litopenaeus vannamei at low salinity. Comp. Biochem. Physiol. Part B 228, 26–33. doi: 10.1016/j.cbpb.2018.11.002
Xie, S., Liu, Y., Zeng, S., Niu, J., and Tian, L. (2016). Partial replacement of fish-meal by soy protein concentrate and soybean meal based protein blend for juvenile pacific white shrimp, Litopenaeus vannamei. Aquaculture 464, 296–302. doi: 10.1016/j.aquaculture.2016.07.002
Xie, S., Niu, J., Zhou, W., Liu, Y., and Tian, L. (2018). Developing a low fishmeal diet for juvenile pacific white shrimp, Litopenaeus vannamei, using the nutritional value of fm as the reference profile. Aquacult. Nutr. 24, 1184–1197. doi: 10.1111/anu.12657
Xie, S., Tian, L., Jin, Y., Yang, H., Liang, G., Liu, Y. J., et al. (2014). Effect of glycine supplementation on growth performance, body composition and salinity stress of juvenile pacific white shrimp, Litopenaeus vannamei fed low fishmeal diet. Aquaculture 418, 159–164.
Xie, S., Yin, P., Tian, L., Liu, Y., and Niu, J. (2020). Lipid metabolism and plasma metabolomics of juvenile largemouth bass Micropterus Salmoides were affected by dietary oxidized fish oil. Aquaculture 522:735158. doi: 10.1016/j.aquaculture.2020.735158
Ye, J. D., Wang, K., Li, F. D., Sun, Y. Z., and Liu, X. H. (2011). Incorporation of a mixture of meat and bone meal, poultry by-product meal, blood meal and corn gluten meal as a replacement for fish meal in practical diets of pacific white shrimp Litopenaeus vannamei at two dietary protein levels. Aquacult. Nutr. 17, e337–e347.
Ye, S., Tan, L., Ma, J., Shi, Q., and Li, J. (2010). Polyunsaturated docosahexaenoic acid suppresses oxidative stress induced endothelial cell calcium influx by altering lipid composition in membrane caveolar rafts. Prostaglandins Leukotrienes Essential Fatty Acids 83, 37–43. doi: 10.1016/j.plefa.2010.02.002
Yue, Y. R., Liu, Y. J., Tian, L. X., Gan, L., Yang, H. J., Liang, G. Y., et al. (2012). Effects of replacing fish meal with soybean meal and peanut meal on growth, feed utilization and haemolymph indexes for juvenile white shrimp Litopenaeus vannamei, boone. Aquac. Res. 43, 1687–1696. doi: 10.1111/j.1365-2109.2011.02976.x
Zeng, C., Wen, B., Hou, G., Lei, L., Mei, Z., Jia, X., et al. (2017). Lipidomics profiling reveals the role of glycerophospholipid metabolism in psoriasis. Gigascience 6, 1–11.
Zhang, J., Liu, Y., Tian, L., Yang, H., Liang, G., Xu, D., et al. (2012). Effects of dietary mannan oligosaccharide on growth performance, gut morphology and stress tolerance of juvenile pacific white shrimp, Litopenaeus vannamei. Fish Shellfish Immun. 33, 1027–1032.
Zhao, J., Shi, P., Sun, Y., Sun, J., Dong, J., Wang, H. G., et al. (2015). Dha protects against experimental colitis in il-10-deficient mice associated with the modulation of intestinal epithelial barrier function. Br. J. Nutr. 114, 181–188. doi: 10.1017/S0007114515001294
Zhou, L., Liao, Y., Yin, P., Zeng, Z., Li, J., Lu, X., et al. (2014). Metabolic profiling study of early and late recurrence of hepatocellular carcinoma based on liquid chromatography-mass spectrometry. J. Chromatogr. B 966, 163–170. doi: 10.1016/j.jchromb.2014.01.057
Keywords: fish-meal, Schizochytrium limacinum, DHA, metabolomics, intestinal health
Citation: Xie S, Wei D, Tan B, Liu Y, Tian L and Niu J (2020) Schizochytrium limacinum Supplementation in a Low Fish-Meal Diet Improved Immune Response and Intestinal Health of Juvenile Penaeus monodon. Front. Physiol. 11:613. doi: 10.3389/fphys.2020.00613
Received: 10 February 2020; Accepted: 15 May 2020;
Published: 30 June 2020.
Edited by:
Samad Rahimnejad, University of South Bohemia in České Budějovice, CzechiaReviewed by:
Arash Akbarzadeh, Hormozgan University of Medical Sciences, IranKang-le Lu, Jimei University, China
Yanjiao Zhang, Ocean University of China, China
Copyright © 2020 Xie, Wei, Tan, Liu, Tian and Niu. This is an open-access article distributed under the terms of the Creative Commons Attribution License (CC BY). The use, distribution or reproduction in other forums is permitted, provided the original author(s) and the copyright owner(s) are credited and that the original publication in this journal is cited, in accordance with accepted academic practice. No use, distribution or reproduction is permitted which does not comply with these terms.
*Correspondence: Shiwei Xie, eHN3enNkeEAxNjMuY29t; Jin Niu, Z3puaXVqaW4yMDAzQDE2My5jb20=