- 1Biochemistry and Molecular Genetic Department, Centre Hospitalier Universitaire (CHU) Clermont-Ferrand, Clermont-Ferrand, France
- 2Faculty of Medicine, CNRS 6293, INSERM 1103, GReD, Université Clermont Auvergne, Clermont-Ferrand, France
- 3Centre de Recherche du Centre Hospitalier Universitaire (CHU) de Québec-Université Laval, Québec City, QC, Canada
- 4Department of Molecular Biology, Medical Biochemistry and Pathology, Faculty of Medicine, Université Laval, Québec City, QC, Canada
- 5Department of Obstetrics and Gynecology, Faculty of Medicine, Université Laval, Québec City, QC, Canada
- 6Biostatistics Unit Direction de la Recherche Clinique et des Innovations (DRCI), Centre Hospitalier Universitaire (CHU) Clermont-Ferrand, Clermont-Ferrand, France
- 7Department of Obstetrics and Gynecology, Centre Hospitalier Universitaire (CHU) Clermont-Ferrand, Clermont-Ferrand, France
Preterm premature rupture of membranes (PPROM), defined as rupture of fetal membranes prior to 37 weeks of gestation, complicates approximately 2–4% of pregnancies and is responsible for 40% of all spontaneous preterm births. PPROM arises from complex pathophysiological pathways with a key actor: inflammation. Sterile inflammation is a feature of senescence-associated fetal membrane maturity. During specific steps of sterile inflammation, cells also release highly inflammatory damage-associated molecular pattern markers (DAMPs), such as high-mobility group box 1 (HMGB1) or S100A8/A9, known to link and activate the receptor for advanced glycation end products (RAGE). The objective of this study was to measure longitudinally during pregnancy concentrations of the soluble form of RAGE (sRAGE) and its main ligands (AGE, HMGB1, S100A8/A9) in blood specimens. We studied 246 pregnant women (82 with PPROM and 164 matched control pregnant women without complications) from a cohort of 7,866 pregnant women recruited in the first trimester and followed during pregnancy until delivery. sRAGE, AGE, HMGB1, and S100A8/A9 concentrations were measured in plasma and in serum-extracted extracellular vesicles from first trimester (T1), second trimester (T2), and delivery (D). In plasma, we observed, in both PPROM and control groups, (i) a significant increase of HMGB1 concentrations between T1 vs. T2, T1 vs. D, but not between T2 vs. D; (ii) a significant decrease of sRAGE concentrations between T1 and T2 and a significant increase between T2 and D; (iii) a significant decrease of AGE from T1 to D; (iv) no significant variation of S100A8/A9 between trimesters. In intergroup comparisons (PPROM vs. control group), there were no significant differences in time variation taking into account the matching effects. There was a correlation between plasma and serum-extracted extracellular vesicle concentrations of sRAGE, AGE, HMGB1, and S100A8/A9. Our results suggest that the rupture of fetal membranes (physiological or premature) is accompanied by a variation in plasma concentrations of sRAGE, HMGB1, and AGE. The study of RAGE and its main ligands in extracellular vesicles did not give additional insight into the pathophysiological process conducting to PPROM.
Introduction
Preterm premature rupture of membranes (PPROM), defined as rupture of fetal membranes prior to 37 weeks of gestation, complicates approximately 2–4% of all pregnancies and is responsible for 40–50% of all preterm births (Naeye and Peters, 1980; Mercer et al., 2000). PPROM arises from complex, multifaceted pathophysiological pathways where the inflammation axis plays a major role (Menon and Richardson, 2017). Indeed, recent reports indicated that PPROM may be associated with sterile inflammation in the fetal membranes (Romero et al., 2015). In support of this hypothesis, it has been shown that histological chorioamnionitis in the presence of a negative amniotic fluid culture increases the risk of preterm birth (Park et al., 2017). Sterile inflammation is a feature of senescence-associated fetal membranes maturity and is characterized mostly by the presence of inflammatory biomarkers, growth factors, and matrix degrading enzymes (Coppe et al., 2008). During the specific steps of sterile inflammation, senescent, stressed, or necrotic cells release highly inflammatory damage-associated molecular pattern markers (DAMPs) (Menon and Richardson, 2017). High-mobility group box 1 (HMGB1) is one of the DAMPs that have been linked to parturition (Sheller-Miller et al., 2017; D’Angelo et al., 2018). In a mouse model, intra-amniotic administration of HMGB1 induces spontaneous preterm labor and birth (Gomez-Lopez et al., 2016). Moreover, it was observed that HMGB1 induces an inflammatory response, partially mediated by the inflammasome, in the fetal membranes (Plazyo et al., 2016). This intra-amniotic inflammasome activation was highlighted in vivo in human (Gomez-Lopez et al., 2019). HMGB1 is a known ligand of receptor for advanced glycation end products (RAGE), and the RAGE system is associated with pregnancy complications as preeclampsia or PPROM (Naruse et al., 2012; Rzepka et al., 2015). Moreover, AGEs could be implicated in PPROM with blood levels significantly higher in pregnant women complicated with PPROM (Kansu-Celik et al., 2019). Calprotectin (or S100A8/A9) is also a known ligand of RAGE (Pruenster et al., 2016) implicated in some pregnancy pathologies as preeclampsia (Pergialiotis et al., 2016).
Extracellular vesicles are a heterogeneous group of cell-derived membranous structures comprising exosomes (50–150 nm) and microvesicles (50–500 nm up to 1 μm), which originate from the endosomal system or which are shed from the plasma membrane, respectively (van Niel et al., 2018). The study of maternal plasma exosomes determines pathways associated with PPROM including non-specific inflammation or oxidative stress (Menon et al., 2019). The RAGE system (receptor and ligands) has not been specifically studied in maternal blood exosomes. However, some DAMPs, such as HMGB1 have been identified as present in oxidative-stressed amnion epithelial cell-derived exosomes (Sheller-Miller et al., 2017). Furthermore, in vitro, amnion epithelial cell exosomes lead to an increased inflammatory response in maternal uterine cells, suggesting that fetal cell exosomes may act as a signal to parturition in choriodecidua and migrate into the maternal circulation (Hadley et al., 2018). Combining maternal characteristics and environmental and clinical known risk factors (Bouvier et al., 2019) to candidate biomarkers may in the future result in proposing a clinically predictive model identifying asymptomatic women at higher risk of PPROM.
In this context, taking advantage of a large cohort of pregnant women recruited prospectively at the beginning of pregnancy, we investigated the changes in the concentrations of the soluble form of RAGE (sRAGE) and its main ligands (AGE, HMGB1, S100A8/A9) in plasma and in the serum-extracted extracellular vesicles from first trimester to delivery to better understand the potential role of the RAGE system in PPROM.
Materials and Methods
Study Design and Participants
This is a case/control study of sRAGE, HMGB1, AGE, and S100A8/A9 concentrations in plasma samples and serum-extracted exosomes of pregnant women with PPROM from an already constituted prospective biobank for which blood samples were collected [research program funded by the CIHR Institute of Human Development, Child and Youth Health Initiative (Grant Number: NRFHPG-)]. The biobank includes samples from 7,866 pregnant women recruited at the CHU de Québec-Université Laval between April 2005 and March 2010 and followed during pregnancy until delivery (Forest et al., 2014). Participants gave their informed written consent, and the study was approved by the Ethics Committee of the CHU de Québec [initial approval date: November 9, 2004, project 5-04-10-01 [95.05.17], SC12-01-159]. Cases were selected from all pregnant women with PPROM for whom three successive blood samples were collected and then frozen: one during the first trimester (T1), one during the second trimester (T2), and one at delivery (D). In the control group, we selected pregnant women (two control for one case) with delivery at term (after 37 weeks of gestation) and for whom three plasma and serum samples were collected and then frozen (T1, T2, and D). Women in the control group were matched with those in the case group on the following criteria: maternal age (±5 years), gestational age at T1 sample (±1 week), gestational age at T2 sample (±3 weeks), storage time at -80°C (±6 months). A total of 246 pregnant women (82 with PPROM and 164 matched control pregnant women) were selected.
Serum Extracellular Vesicle Extraction
For total extracellular vesicles from 30 to 120 nm isolation from 738 serum samples (three samples T1, T2, and D for 246 women), we used a kit (ref 4478360) from InvitrogenTM (Carlsbad, California, United States) using 450 μl of serum and following the manufacturer’s instructions. Then, for extraction of total proteins from extracellular vesicles, we used InvitrogenTM kit (ref 4478545) following the manufacturer’s instructions. The assay of total proteins in the extracellular vesicle extracts was carried out using a Vista® analyzer (Siemens, Munich, Germany). The assay of apolipoprotein B (Apo B) in 16 extracellular vesicle extracts (eight from the control group and eight from the PPROM group) was carried out using a Vista® analyzer (Siemens, Munich, Germany).
ELISA of Soluble Receptor for Advanced Glycation End Products, High-Mobility Group Box 1, Advanced Glycation End Products, and S100A8/A9
The concentrations of sRAGE, HMGB1, AGE, and S100A8/A9 in 738 plasma samples (three samples T1, T2, and D for 246 women) and in 738 serum-extracted extracellular vesicle samples (three samples T1, T2, and D for 246 women) was measured by the ELISA method using MyBioSource® kits (San Diego, California, United States) following the manufacturer’s instructions (ref MBS2515963, MBS024146, MBS2000151, and MBS7606803, respectively). The concentrations of sRAGE, HMGB1, AGE, and S100A8/A9 of each serum extracellular vesicle sample were normalized against total protein concentrations.
Statistics
Statistical analyses were performed using Stata software, Version 13 (StataCorp, College Station, Texas, United States). All tests were two-sided, with a Type I error set at 0.05. Continuous data were expressed as mean and standard deviation (SD) or median and interquartile range (IQR) according to statistical distribution. The assumption of normality was assessed by using the Shapiro–Wilk test. The comparisons between the PPROM and control groups, for non-repeated data, were performed using Student t-test or Mann–Whitney test when the assumptions of t-test were not met for continuous parameters. Chi-square test or, if applicable, Fisher’s exact test were applied for categorical variables. The relation between continuous variables (AGEs, sRAGE, HMGB1, S100A8/A9 concentrations) in serum-extracted extracellular vesicles and in plasma was analyzed estimating correlation coefficients, Pearson or Spearman according to the statistical distribution and applying a Sidak’s type I error correction to take into account multiple comparisons. These correlations’ results were illustrated with a color-coded heat map.
Random-effects models for repeated data were performed to compare the evolution of AGEs, sRAGE, HMGB1, and S100A8/A9 plasma concentrations and serum-extracted extracellular vesicle concentrations between groups (PPROM and controls). The following fixed effects were measured: time (T1, T2, D), group and time × group interaction, taking into account between- and within-participant variability (subject as random-effect). The normality of residuals from these models was studied using the Shapiro–Wilk test. When appropriate, a logarithmic transformation was proposed to achieve the normality of dependent outcome. A Sidak’s type I error correction was applied to perform multiple comparisons.
Results
Description of the Cohort
Of the 7,866 pregnant women recruited for the biobank, 189 women presented a PPROM (2.4%). Of these, 82 fulfilled the criteria of disposing of three blood samples. Therefore, a total of 246 pregnant women (82 with PPROM and 164 matched control pregnant women, 1:2 ratio) were selected. No significant differences (p = 0.7) were observed between the mean age of mothers in the control group (29.5 years, SD: 4.1) and the PPROM group (29.3 years, SD: 4.2) (Table 1). Some risk factors of PPROM were found significantly higher in the PPROM group as nulliparity, past history of PPROM, gestational diabetes mellitus, smoking during pregnancy (Table 1). A significant difference (p < 0.001) for the gestational age at delivery was expectedly observed between the PPROM group [36 weeks, interquartile range (IQR): 35.1–36.4] and the control group (38.7 weeks, IQR: 38.1–39.3) (Table 1).
Assays in Plasma
A significant decrease of median concentrations of AGEs in both PPROM and control groups was observed between T1 and T2, T2 and D, and T1 and D (see p1 and p2 in Table 2 for the PPROM and control group, respectively; Figure 1A). These variations in concentrations observed during pregnancy were not significantly different between the PPROM and control groups (see p3 in Table 2). Similarly, for each sampling time (T1, T2, D), the medians of plasma concentrations are not significantly different between the PPROM and control groups (see p4 in Table 2).
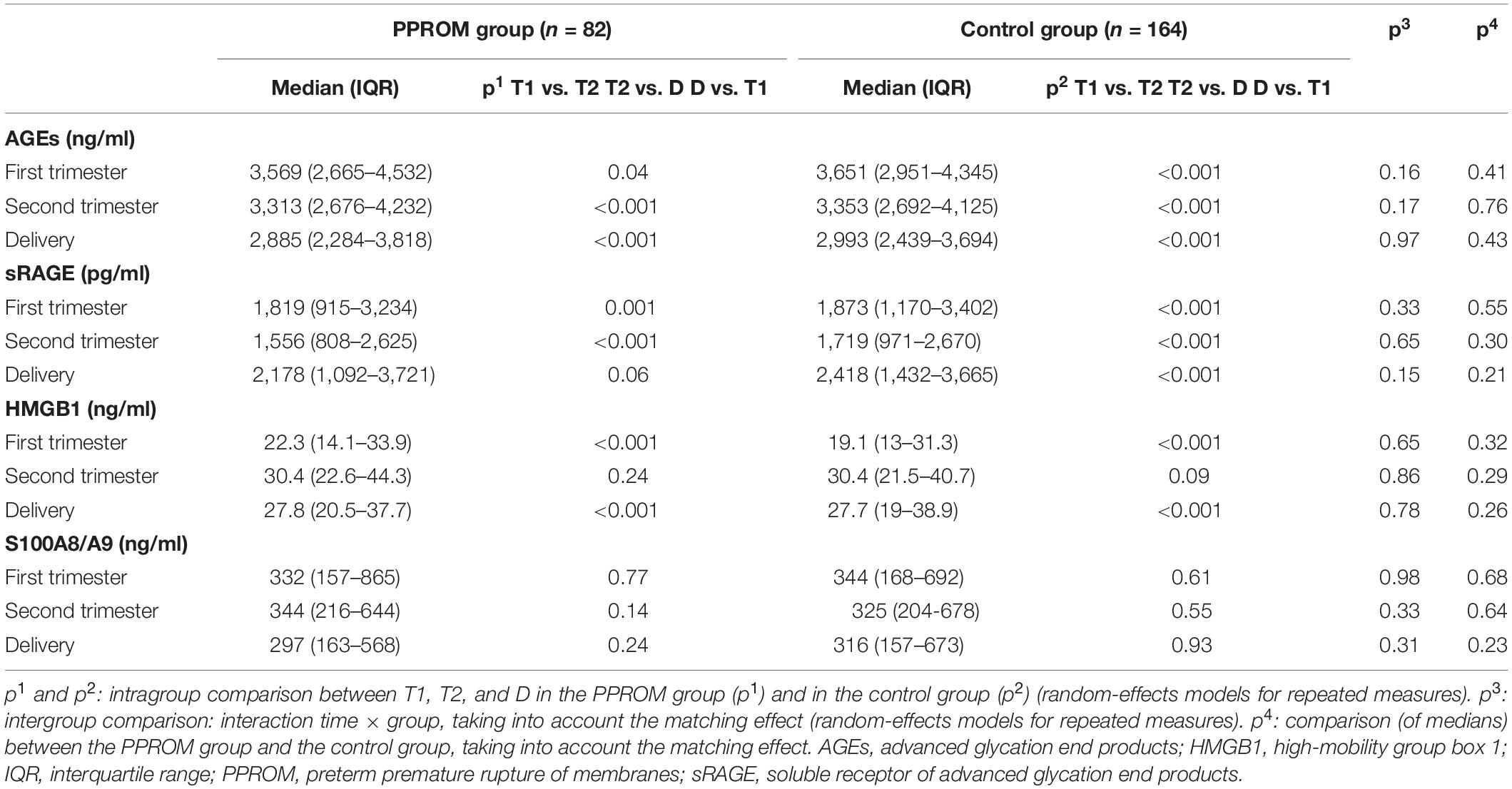
Table 2. Median AGEs, sRAGE, HMGB1, and S100A8/A9 plasma concentrations from 82 women in the PPROM group and 164 women in the control group at three points: first trimester (T1), second trimester (T2), and delivery (D).
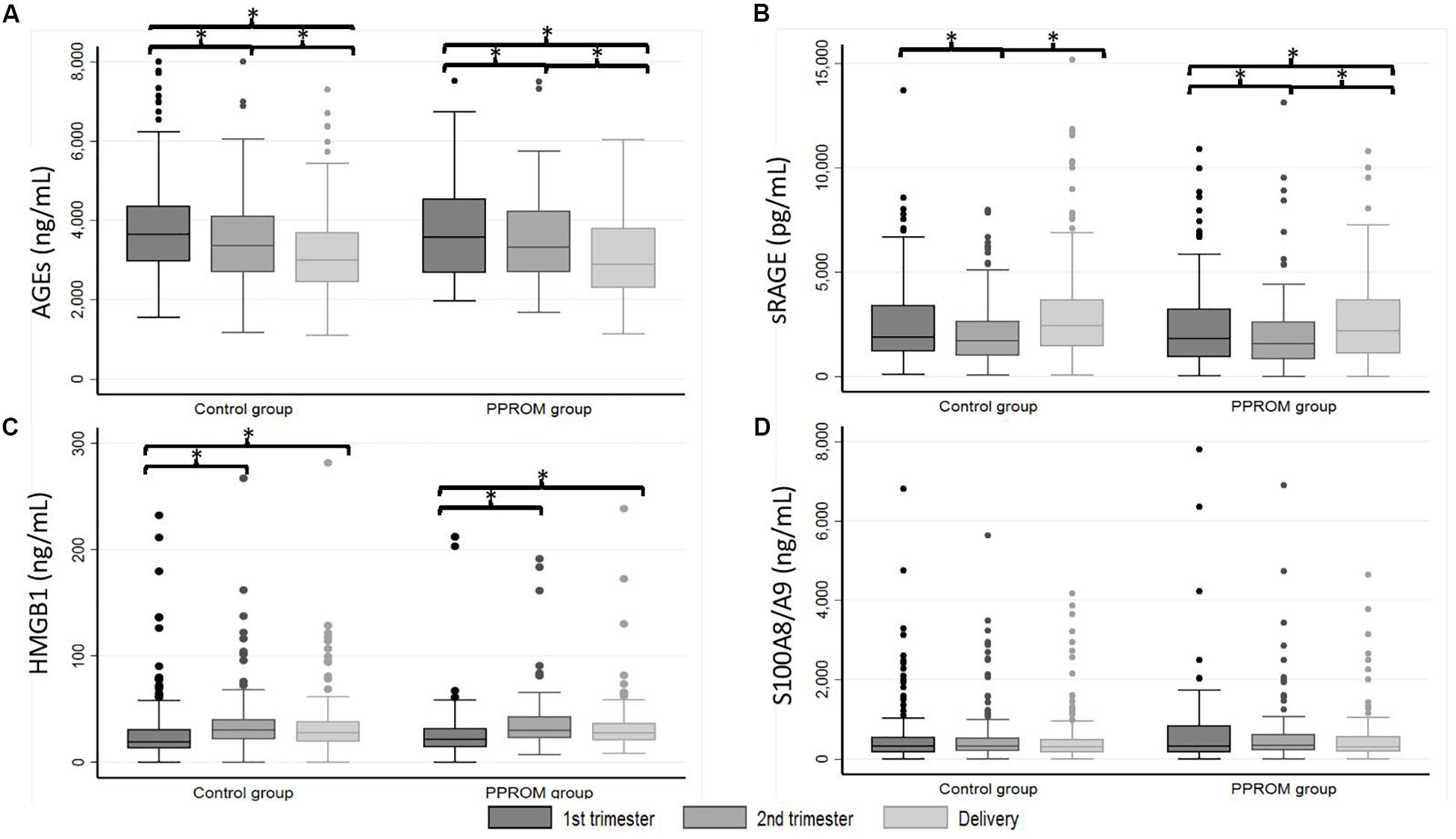
Figure 1. Box plot of AGEs (A), sRAGE (B), HMGB1 (C), and S100A8/A9 (D) plasma median concentrations from 82 women in the PPROM group and 164 women in the control group at three points: first trimester, second trimester, and delivery. *p < 0.05 (random-effects for repeated measures). AGEs, advanced glycation end products; HMGB1, high-mobility group box 1; PPROM, preterm premature rupture of membranes; sRAGE, soluble receptor of advanced glycation end products.
For sRAGE in both PPROM and control groups, a significant decrease of median concentration between T1 and T2 and then a significant increase between T2 and D were observed (see p1 and p2 in Table 2 for the PPROM and control group, respectively; Figure 1B). At delivery, the sRAGE concentration was significantly higher than at T1 in the control group (p < 0.001) but not in PPROM (p = 0.06). These variations in concentrations observed during pregnancy were not significantly different between the PPROM and control groups (see p3 in Table 2). Similarly, for each sampling time (T1, T2, D), the medians of plasma concentrations were not significantly different between the PPROM and control groups (see p4 in Table 2).
For HMGB1 in both PPROM and control groups, a significant increase of median concentration was observed between T1 and T2 (and also T1 and D) followed by a stagnation between T2 and D (see p1 and p2 in Table 2 for the PPROM and control groups, respectively; Figure 1C). These variations in concentrations observed during pregnancy were not significantly different between the PPROM and control groups (see p3 in Table 2). Similarly, for each sampling time (T1, T2, D), the medians of plasma concentrations were not significantly different between the PPROM and control groups (see p4 in Table 2).
For S100A8/A9, in both PPROM and control groups, no significant variations of median concentrations were observed between T1, T2, and D (see p1 and p2 in Table 2 for the PPROM and control groups, respectively, see p3 in Table 2 and Figure 1D). Similarly, for each sampling time (T1, T2, D), the medians of plasma concentrations were not significantly different between the PPROM and control groups (see p4 in Table 2).
Assays in Serum-Extracted Extracellular Vesicles
Similar results as those obtained in plasma are presented in Table 3 were observed. The variations of concentration during pregnancy were similar as in plasma (see p1 and p2 in Table 3 for the PPROM and control groups, respectively; Figure 2). For all four markers (AGEs, sRAGE, HMGB1, and S100A8/A9) measured in serum-extracted extracellular vesicles, the variations in concentration observed during pregnancy were not significantly different between the PPROM group and the control group (see p3 in Table 3). Similarly, for each sampling time (T1, T2, D), the medians of serum-extracted extracellular vesicles concentrations were not significantly different between the two groups (see p4 in Table 3). Moreover, AGEs, sRAGE, HMGB1, and S100A8/A9 concentrations were significantly correlated between the plasma and the serum-extracted extracellular vesicles for both the PPROM group (expect for AGEs at delivery) and the control group (Figure 3).
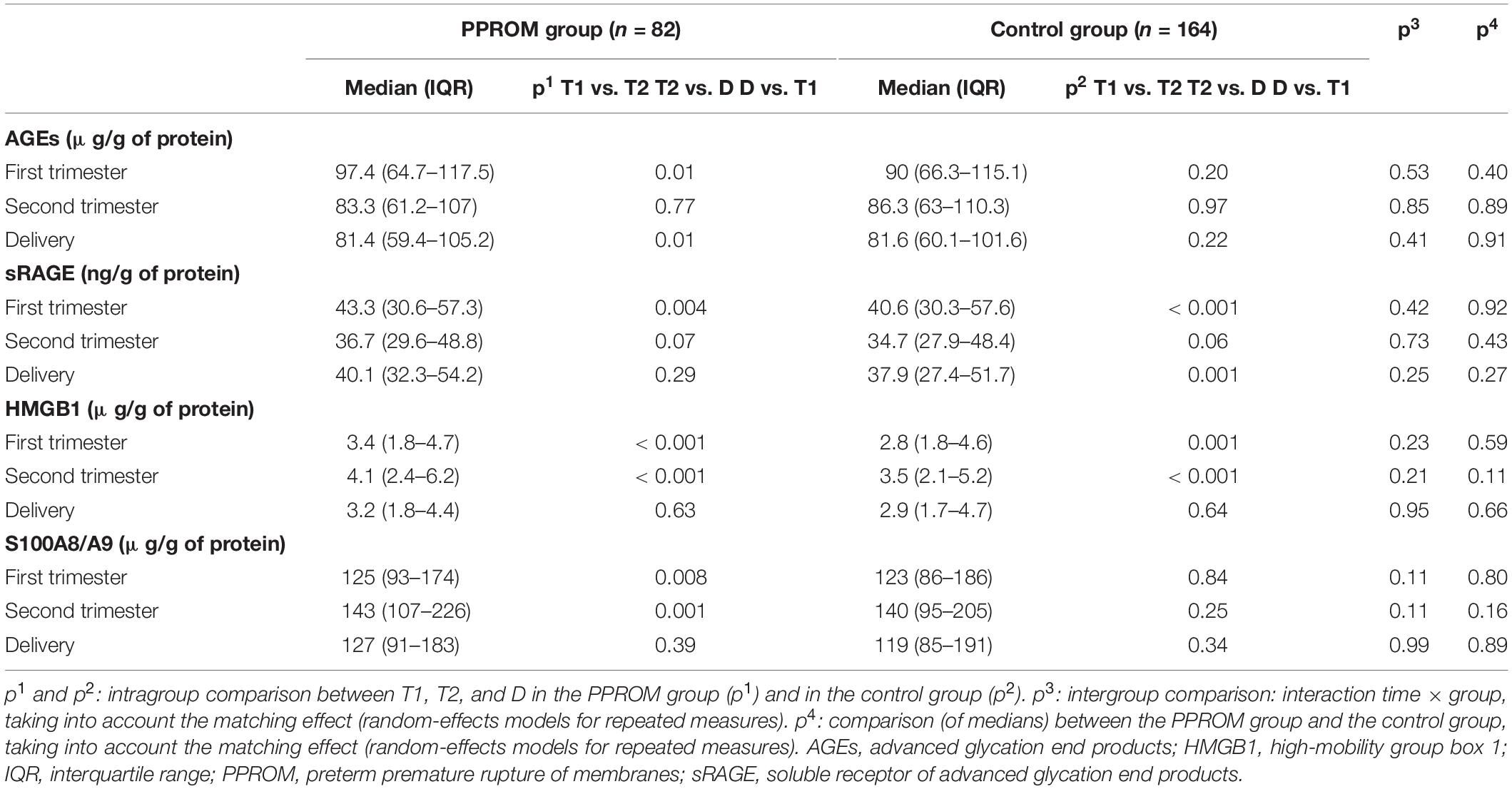
Table 3. Median AGEs, sRAGE, HMGB1, and S100A8/A9 serum-extracted extracellular vesicle concentrations from 82 women in the PPROM group and 164 women in the control group at three points: first trimester (T1), second trimester (T2), and delivery (D).
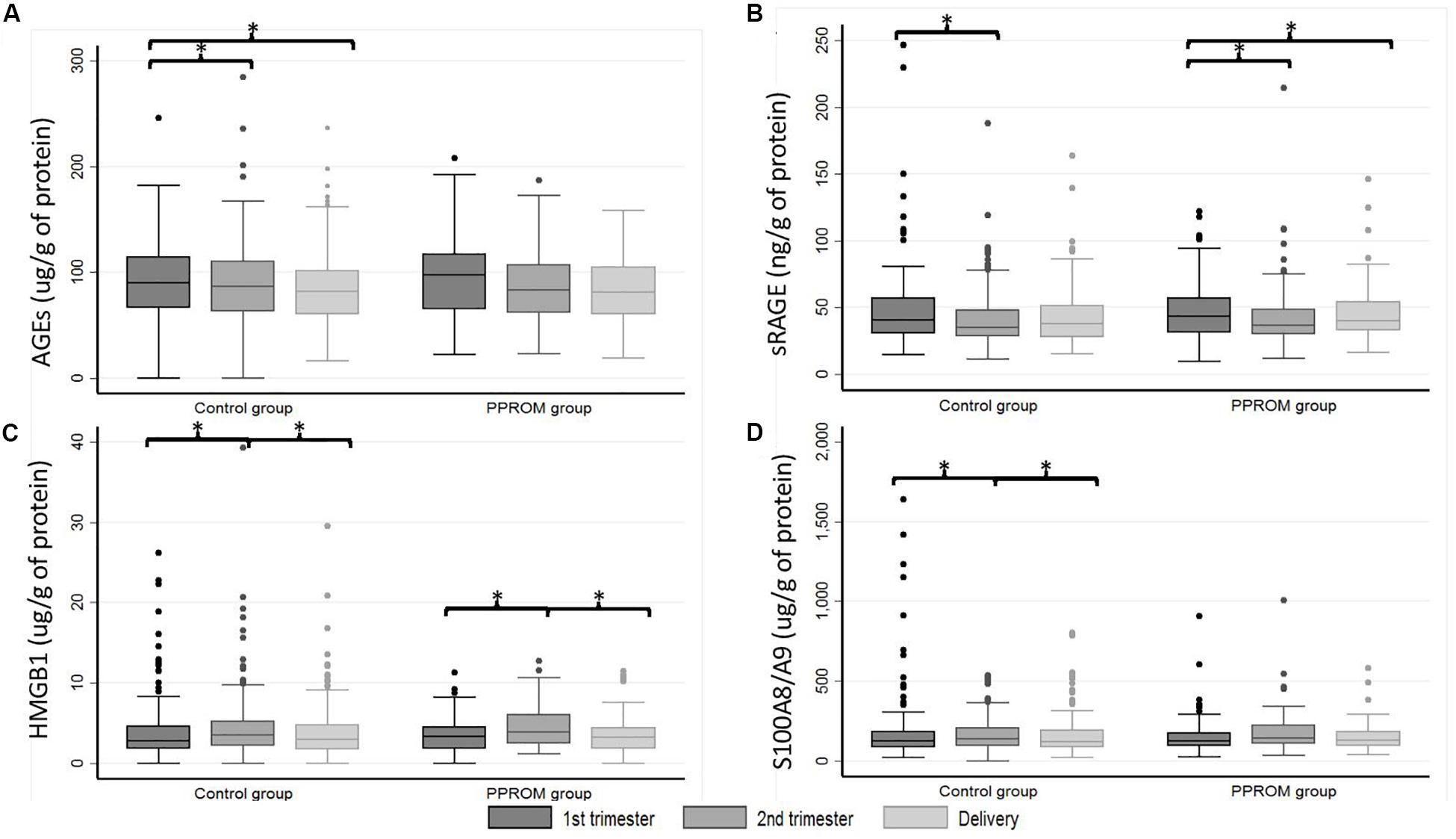
Figure 2. Box plot of AGEs (A), sRAGE (B), HMGB1 (C), and S100A8/A9 (D) serum-extracted extracellular vesicle median concentrations from 82 women in the PPROM group and 164 women in the control group at three points: first trimester, second trimester, and delivery. *p < 0.05 (random-effects for repeated measures). AGEs, advanced glycation end products; HMGB1, high-mobility group box 1; PPROM, preterm premature rupture of membranes; sRAGE, soluble receptor of advanced glycation end products.
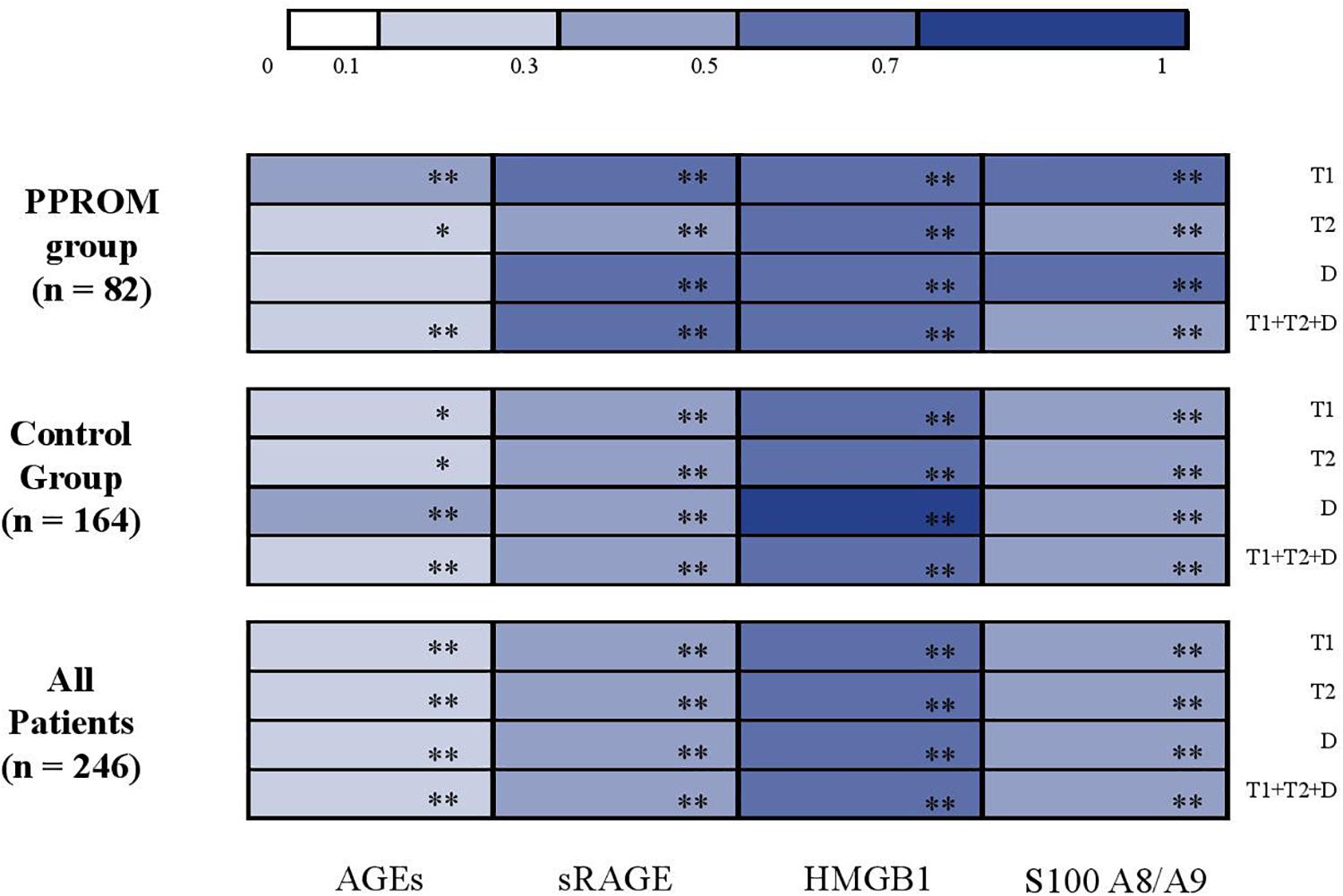
Figure 3. Correlation analysis between AGEs, sRAGE, HMGB1, and S100A8/A9 concentrations in serum-extracted extracellular vesicles and in plasma (color-coded heat map plot). AGEs, advanced glycation end products; D, delivery; HMGB1, high-mobility group box 1; PPROM, preterm premature rupture of membranes; sRAGE, soluble receptor of advanced glycation end products; T1, first trimester; T2, second trimester. *p < 0.05; **p < 0.001.
The assay of Apo B in 16 extracellular vesicles extracts (eight from the control group and eight from the PPROM group) was carried out. The results were found to be below or at the lower limit of linearity (<0.26 g/L).
Discussion
We studied for the first time, on a large number of pregnant women (with and without PPROM), the kinetics from the first trimester to delivery of the plasma concentrations (and serum-extracted exosomes) of four major actors of the RAGE system: sRAGE and three of its ligands, HMGB1, AGEs, and S100A8/9. Among the 189 women with PPROM (2.4% of the cohort), 82 had three blood samples during their pregnancies and were matched to normal controls in a ratio of 1–2. Despite the potential bias linked to the matching criteria, we identified the clinical known risk factors for PPROM as described in a previous study (Bouvier et al., 2019).
There were no significant differences in the serum concentration of total circulating extracellular vesicles between pregnant women with and without PPROM. However, it was proposed that the study of maternal plasma-extracted exosomes could determine pathways associated with PPROM including non-specific inflammation or oxidative stress (Menon et al., 2019). The specific study of biomarkers of the RAGE system in serum-extracted extracellular vesicles of pregnant women was relevant to identify an earlier signal of suffering from the fetal membranes. In this study, we observed that this is not the case and that the concentrations are strongly correlated to those of “total serum,” for the studied actors of RAGE signaling, in contrast to the cellular networks in the work of Menon’s team. Flow cytometry could have been used to determine whether RAGE or studied DAMPs are present on the surface of or inside the extracellular vesicles, but this was not available in our current setting. Another limitation of the study is the absence of characterization of the extracellular vesicles extracted from the serum (van Niel et al., 2018) and of the study of the cellular or tissue origin of these vesicles. Measurement of Apo B below or at the lower limit of linearity (<0.26 g/L) confirmed the absence of contamination of the preparations of extracellular vesicles.
So far, studies on blood levels of sRAGE as PPROM or prematurity risk factor show discordant results (Hajek et al., 2008; Germanova et al., 2010; Bastek et al., 2012; Rzepka et al., 2016). In our study, no differences in plasma sRAGE concentrations were observed between the control and PPROM groups, as already described (Hajek et al., 2008; Rzepka et al., 2016). Indeed, Rzepka et al. (2016) found the same results and found the endogenous secretory RAGE (esRAGE) more interesting as a potential biomarker of PPROM. Bastek et al. (2012) found lower serum concentrations of sRAGE in women who gave birth prematurely including spontaneous premature labor with intact membranes and thus confirmed results of a smaller study (Germanova et al., 2010). Conversely, Hajek et al. (2008) found, in a pilot study, higher values of serum concentrations of sRAGE in women who gave birth prematurely. In our study, an interesting kinetics is observed with a decrease of sRAGE plasma concentration between T1 and T2, and then an increase between T2 and D. Germanova et al. (2010) described exactly the opposite but on a smaller cohort of 79 women with only 25 measurements per trimester. These different conclusions could be due to the presence of different size of cohorts, type of circulating RAGE, and various assays used to measure sRAGE.
Concerning the RAGE ligands in plasma, we observed no differences in S100A8/19 concentrations between the PPROM group and the control group and no variations during pregnancy. Also, we found no differences in plasma AGE concentrations between the PPROM and control groups. These results are in contradiction with those of a recent study on a small cohort of 46 pregnant women (nine with PPROM and 37 without PPROM) where the blood concentration of AGEs at T1 was higher in women with PPROM (Kansu-Celik et al., 2019). Noteworthy, a decrease in the serum concentration of AGEs during pregnancy was also observed in a previous study where the kinetics were studied between the second trimester and delivery (Quintanilla-Garcia et al., 2018). In our study, no differences in plasma HMGB1 concentrations were observed between the PPROM and control groups. However, variations in plasma HMGB1 concentrations during pregnancy were observed with a significant increase between T1 and T2 and then stagnation between T2 and D in both PPROM and control groups. It was previously observed that senescent fetal membranes contribute to sterile inflammation by generation of DAMPs, like HMGB1 (Menon et al., 2016). Also, an increase in the expression of HMGB1, with activation of the RAGE pathway, in the placenta of women with PPROM has been demonstrated (Yan et al., 2018). Our results show the same kinetics in women with PPROM and in control women. HMGB1 has a physiological implication and can be at the “frontiers in physiology.” Based on our blood results, the mechanistic link between the increases in plasma concentrations of HMGB1 and sRAGE and the decrease in the plasma concentration of AGEs should be further investigated. It has been reported that the sRAGE–AGE complex becomes degraded in the spleen or liver (Ramasamy et al., 2008).
Conclusion
In conclusion, our results suggest that the rupture of fetal membranes (physiological or premature) may be related to RAGE activation possibly by the ligand HMGB1. If a more important production of HMGB1 occurring during pregnancy with PPROM could not be detected directly by a higher concentration of HGMB1 in PPROM vs. control group, an indirect proof by the different kinetics of sRAGE between first trimester and delivery could be proposed.
Data Availability Statement
All datasets generated for this study are included in the article/supplementary material.
Ethics Statement
The studies involving human participants were reviewed and approved by the Ethics Committee of the CHU de Québec. The patients/participants provided their written informed consent to participate in this study.
Author Contributions
DB analyzed and interpreted the data and wrote the initial version of the manuscript. J-CF and YG were in charge of the research program on pregnancy complications, designed the study, and assisted with the interpretation of the data and writing of the manuscript. NB and EB supervised the trial and data collection. DB and NB carried out serum exosome extractions and all assays. BP provided statistical advice for the study design and analyzed the data. VS, LB, and DG experts in PPROM, reviewed the manuscript, assisted with the interpretation of the data. All authors substantially contributed to its revision.
Funding
This work was supported by the Canadian Institutes of Health Research (CIHR, Healthy Pregnancy Initiative from the Institute for Human Development, Child and Youth Health, Grant No: NRFHPG-78880). This work was also funded by the Auvergne Rhône-Alpes region as part of the support program for international academic and scientific cooperation between the two French and Canadian teams (SCUSI 2017).
Conflict of Interest
The authors declare that the research was conducted in the absence of any commercial or financial relationships that could be construed as a potential conflict of interest.
Acknowledgments
The authors thank the research nurses for the recruitment of participants and retrieval of data from the medical records. The authors also thank all study participants.
References
Bastek, J. A., Brown, A. G., Foreman, M. N., McShea, M. A., Anglim, L. M., Adamczak, J. E., et al. (2012). The soluble receptor for advanced glycation end products can prospectively identify patients at greatest risk for preterm birth. J. Maern. Fetal Neonatal. Med. 25, 1762–1768. doi: 10.3109/14767058.2012.663825
Bouvier, D., Forest, J. C., Blanchon, L., Bujold, E., Pereira, B., Bernard, N., et al. (2019). Risk factors and outcomes of preterm premature rupture of membranes in a cohort of 6968 pregnant women prospectively recruited. J. Clin. Med. 8:E1987. doi: 10.3390/jcm8111987
Coppe, J. P., Patil, C. K., Rodier, F., Sun, Y., Munoz, D. P., Goldstein, J., et al. (2008). Senescence-associated secretory phenotypes reveal cell-nonautonomous functions of oncogenic RAS and the P53 tumor suppressor. PLoS Biol. 6:2853–2868. doi: 10.1371/journal.pbio.0060301
D’Angelo, G., Marseglia, L., Granese, R., Di Benedetto, A., Giacobbe, P., Impellizzeri, A., et al. (2018). Different concentration of human cord blood HMGB1 according to delivery and labour: a pilot study. Cytokine 108, 53–56. doi: 10.1016/j.cyto.2018.03.019
Forest, J. C., Theriault, S., Masse, J., Bujold, E., and Giguere, Y. (2014). Soluble fms-like tyrosine kinase-1 to placental growth factor ratio in mid-pregnancy as a predictor of preterm preeclampsia in asymptomatic pregnant women. Clin. Chem. Lab. Med. 52, 1169–1178. doi: 10.1515/cclm-2013-0955
Germanova, A., Koucky, M., Hajek, Z., Parizek, A., Zima, T., and Kalousova, M. (2010). Soluble receptor for advanced glycation end products in physiological and pathological pregnancy. Clin. Biochem. 43, 442–446. doi: 10.1016/j.clinbiochem.2009.11.002
Gomez-Lopez, N., Romero, R., Maymon, E., Kusanovic, J. P., Panaitescu, B., Miller, D., et al. (2019). Clinical chorioamnionitis at term IX: in vivo evidence of intra-amniotic inflammasome activation. J. Perinat. Med. 47, 276–287. doi: 10.1515/jpm-2018-0271
Gomez-Lopez, N., Romero, R., Plazyo, O., Panaitescu, B., Furcron, A. E., Miller, D., et al. (2016). Intra-amniotic administration of hmgb1 induces spontaneous preterm labor and birth. Am. J. Reprod. Immunol. 75, 3–7. doi: 10.1111/aji.12443
Hadley, E. E., Sheller-Miller, S., Saade, G., Salomon, C., Mesiano, S., Taylor, R. N., et al. (2018). Amnion epithelial cell-derived exosomes induce inflammatory changes in uterine cells. Am. J. Obstet. Gynecol. 219, 478.e1–478.e21. doi: 10.1016/j.ajog.2018.08.021
Hajek, Z., Germanova, A., Koucky, M., Zima, T., Kopecky, P., Vitkova, M., et al. (2008). Detection of feto-maternal infection/inflammation by the soluble receptor for advanced glycation end products (SRAGE): results of a pilot study. J. Perinat. Med. 36, 399–404. doi: 10.1515/JPM.2008.080
Kansu-Celik, H., Tasci, Y., Karakaya, B. K., Cinar, M., Candar, T., and Caglar, G. S. (2019). Maternal serum advanced glycation end products level as an early marker for predicting preterm labor/PPROM: a prospective preliminary study. J. Matern. Fetal Neonatal. Med.? 32, 2758–2762. doi: 10.1080/14767058.2018.1449202
Menon, R., Behnia, F., Polettini, J., Saade, G. R., Campisi, J., and Velarde, M. (2016). Placental membrane aging and HMGB1 signaling associated with human parturition. Aging 8, 216–230. doi: 10.18632/aging.100891
Menon, R., Dixon, C. L., Sheller-Miller, S., Fortunato, S. J., Saade, G. R., Palma, C., et al. (2019). Quantitative proteomics by SWATH-MS of maternal plasma exosomes determine pathways associated with term and preterm birth. Endocrinology 160, 639–650. doi: 10.1210/en.2018-00820
Menon, R., and Richardson, L. S. (2017). Preterm prelabor rupture of the membranes: a disease of the fetal membranes. Semin. Perinatol. 41, 409–419. doi: 10.1053/j.semperi.2017.07.012
Mercer, B. M., Goldenberg, R. L., Meis, P. J., Moawad, A. H., Shellhaas, C., Das, A., et al. (2000). The preterm prediction study: prediction of preterm premature rupture of membranes through clinical findings and ancillary testing. The National Institute of Child Health and Human Development Maternal-Fetal Medicine Units Network. Am. J. Obstet. Gynecol. 183, 738–745. doi: 10.1067/mob.2000.106766
Naeye, R. L., and Peters, E. C. (1980). Causes and consequences of premature rupture of fetal membranes. Lancet 1, 192–194. doi: 10.1016/s0140-6736(80)90674-1
Naruse, K., Sado, T., Noguchi, T., Tsunemi, T., Yoshida, S., Akasaka, J., et al. (2012). Peripheral RAGE (Receptor for Advanced Glycation Endproducts)-ligands in normal pregnancy and preeclampsia: novel markers of inflammatory response. J. Reprod. Immunol. 93, 69–74. doi: 10.1016/j.jri.2011.12.003
Park, J. W., Park, K. H., and Jung, E. Y. (2017). Clinical significance of histologic chorioamnionitis with a negative amniotic fluid culture in patients with preterm labor and premature membrane rupture. PLoS One 12:e0173312. doi: 10.1371/journal.pone.0173312
Pergialiotis, V., Prodromidou, A., Pappa, E., Vlachos, G. D., Perrea, D. N., and Papantoniou, N. (2016). An Evaluation of calprotectin as serum marker of preeclampsia: a systematic review of observational studies. Inflamm. Res. 65, 95–102. doi: 10.1007/s00011-015-0903-0
Plazyo, O., Romero, R., Unkel, R., Balancio, A., Mial, T. N., Xu, Y., et al. (2016). HMGB1 induces an inflammatory response in the chorioamniotic membranes that is partially mediated by the inflammasome. Biol. Reprod. 95:130. doi: 10.1095/biolreprod.116.144139
Pruenster, M., Vogl, T., Roth, J., and Sperandio, M. (2016). S100A8/A9: from basic science to clinical application. Pharmacol. Ther. 167, 120–131. doi: 10.1016/j.pharmthera.2016.07.015
Quintanilla-Garcia, C. V., Uribarri, J., Fajardo-Araujo, M. E., Barrientos-Romero, J. J., Romero-Gutierrez, G., Reynaga-Ornelas, M. G., et al. (2018). Changes in circulating levels of carboxymethyllysine, Soluble receptor for advanced glycation end products (sRAGE), and inflammation markers in women during normal pregnancy. J. Matern. Fetal Neonatal. Med. 25, 1–6. doi: 10.1080/14767058.2018.1481948
Ramasamy, R., Yan, S. F., Herold, K., Clynes, R., and Schmidt, A. M. (2008). Receptor for advanced glycation end products: fundamental roles in the inflammatory response: winding the way to the pathogenesis of endothelial dysfunction and atherosclerosis. Ann. N. Y. Acad. Sci. 1126, 7–13. doi: 10.1196/annals.1433.056
Romero, R., Miranda, J., Chaemsaithong, P., Chaiworapongsa, T., Kusanovic, J. P., Dong, Z., et al. (2015). Sterile and microbial-associated intra-amniotic inflammation in preterm prelabor rupture of membranes. J. Matern. Fetal Neonatal. Med. 28, 1394–1409. doi: 10.3109/14767058.2014.958463
Rzepka, R., Dolegowska, B., Rajewska, A., Kwiatkowski, S., Salata, D., Budkowska, M., et al. (2015). Soluble and endogenous secretory receptors for advanced glycation end products in threatened preterm labor and preterm premature rupture of fetal membranes. BioMed Res. Int. 2015:568042. doi: 10.1155/2015/568042
Rzepka, R., Dolegowska, B., Rajewska, A., Salata, D., Budkowska, M., Kwiatkowski, S., et al. (2016). Diagnostic potential of evaluation of SDF-1alpha and SRAGE levels in threatened premature labor. Biomed. Res. Int. 2016:2719460. doi: 10.1155/2016/2719460
Sheller-Miller, S., Urrabaz-Garza, R., Saade, G., and Menon, R. (2017). Damage-associated molecular pattern markers HMGB1 and cell-free fetal telomere fragments in oxidative-stressed amnion epithelial cell-derived exosomes. J. Reprod. Immunol. 123, 3–11. doi: 10.1016/j.jri.2017.08.003
van Niel, G., D’Angelo, G., and Raposo, G. (2018). Shedding light on the cell biology of extracellular vesicles. Nat. Rev. Mol. Cell Biol. 19, 213–228. doi: 10.1038/nrm.2017.125
Keywords: preterm premature rupture of membranes, extracellular vesicles, soluble receptor for advanced glycation end products, advanced glycation end products, high-mobility group box 1, S100A8/A9
Citation: Bouvier D, Giguère Y, Blanchon L, Bujold E, Pereira B, Bernard N, Gallot D, Sapin V and Forest J-C (2020) Study of sRAGE, HMGB1, AGE, and S100A8/A9 Concentrations in Plasma and in Serum-Extracted Extracellular Vesicles of Pregnant Women With Preterm Premature Rupture of Membranes. Front. Physiol. 11:609. doi: 10.3389/fphys.2020.00609
Received: 20 February 2020; Accepted: 15 May 2020;
Published: 23 June 2020.
Edited by:
Ramkumar Menon, The University of Texas Medical Branch at Galveston, United StatesReviewed by:
Nardhy Gomez-Lopez, Wayne State University School of Medicine, United StatesCarlos Salomon, The University of Queensland, Australia
Copyright © 2020 Bouvier, Giguère, Blanchon, Bujold, Pereira, Bernard, Gallot, Sapin and Forest. This is an open-access article distributed under the terms of the Creative Commons Attribution License (CC BY). The use, distribution or reproduction in other forums is permitted, provided the original author(s) and the copyright owner(s) are credited and that the original publication in this journal is cited, in accordance with accepted academic practice. No use, distribution or reproduction is permitted which does not comply with these terms.
*Correspondence: Damien Bouvier, dbouvier@chu-clermontferrand.fr