- Laboratory of Fish and Shellfish Nutrition, School of Marine Sciences, Ningbo University, Ningbo, China
An 8-week feeding trial was conducted to evaluate the effects of dietary carbohydrate to lipid (CHO:L) ratios on growth performance, muscle fatty acid composition, and intermediary metabolism in juvenile black seabream (Acanthopagrus schlegelii). Five isonitrogenous and isoenergetic diets (48.0% crude protein and 18.0 MJ kg–1 gross energy) were formulated to contain different CHO:L ratios ranging from 0.33 to 3.75. Triplicate groups of 20 fish averaging 0.51 ± 0.01 g were fed with experimental diets twice daily to apparent satiation. The results indicated that final body weight (FBW), percentage weight gain (PWG), specific growth rate (SGR), and protein efficiency ratio (PER) were significantly influenced by the dietary CHO:L ratios (p < 0.05). The highest FBW, PWG, and SGR were observed in fish fed the diet with a CHO:L ratio of 1.36 (p < 0.05). A two-slope broken-line regression analysis based on PWG indicated that the optimal dietary CHO:L is 1.08. Lipid content in the whole body decreased, and glycogen concentration in the liver increased with the increase of dietary CHO:L ratios from 0.33 to 3.75 (p < 0.05). Moreover, there was a positive correlation between muscle fatty acid composition and dietary fatty acid composition. The relative expression levels of genes involved in glucose metabolism, such as gk, pepck, and glut2 were upregulated by increasing the dietary CHO:L ratio. Also, the mRNA expression level of genes related to lipid synthesis, such as fas and accα were significantly upregulated with dietary CHO:L ratios increasing from 0.33 to 3.75. The highest expression of genes involved in fatty acid β-oxidation, such as cpt1 and acox1, were observed in fish fed the 1.36 CHO:L ratio diet. The gene expression of Δ6 fatty acyl desaturase (fads2) in the liver significantly increased with increase of dietary CHO:L ratios from 0.33 to 3.75. Fish fed the diet with CHO:L ratios of 2.26 and 3.75 had lower expression levels of elovl5 than those fed the other diets. These results demonstrate that dietary optimal CHO:L ratios could improve PWG and SGR but also influence expression of genes involved in glucose and lipid metabolism. Based on the overall results, the optimal dietary CHO:L ratio is 1.08 for black seabream.
Introduction
Development of the cost-effective and nutritionally adequate formulated diet is fundamental to the future feasibility for marine fish culture. The rapid growth of aquaculture output depends on the production of aquatic feed, whereas the supply of fish meal and fish oil, which are the most important ingredients in aqua-feeds, has remained comparatively static and gradually decreased over the last decade (Oliva-Teles et al., 2015; Matulić et al., 2020; Wang et al., 2020). In aquaculture, aqua-feed cost are very high, accounting for more than 60% of the total production cost. The fish meal is known as the best protein for aqua-feeds (Han et al., 2016; Hua et al., 2019). Fish meal resources are limited, and fish meal prices have recently increased as populations of wild fisheries have declined due to overfishing (Hutchins et al., 1998; Hardy, 2010; Raggi et al., 2019; Ma et al., 2020). To minimize dietary protein levels, much attention was given to analyzing the viability of non-protein energy substitutes and proved that providing sufficient energy with dietary lipids and carbohydrates can reduce the use of costly protein (Kim and Lee, 2005; Li et al., 2012a, b; Darias et al., 2015). Therefore, dietary carbohydrates and lipids are of increasing importance to aquaculture as these two main nutrients are used as non-protein energy sources in fish feeds and reduce protein requirements (Lin and Shiau, 2003; Li et al., 2012a, b, 2019).
Carbohydrates and lipids are cheaper sources of energy compared to proteins, and they can spare dietary protein for growth rather than being used as an energy source, which is also coupled with increased ammonia excretion into the water. Fish growth performance and metabolic efficiency vary depending on the dietary lipid and carbohydrate levels (Gümüş and Ikiz, 2009; Li et al., 2014; Xing et al., 2016). Any imbalances in the supply of lipids and carbohydrates negatively affect growth performance, nutrient utilization, and even the health status of fish (Erfanullah and Jafri, 1998; Li et al., 2012b). Recently, an increasing number of studies have investigated the interactions between lipid and carbohydrate levels in fish species, such as red drum (Sciaenops ocellatus; Ellis and Reigh, 1991), rainbow trout (Oncorhynchus mykiss; Gümüş and Ikiz, 2009), blunt snout bream (Megalobrama amblycephala; Li et al., 2014), and large yellow croaker (Larmichthys crocea; Zhou et al., 2016). However, most studies mainly focused on determining the most favorable carbohydrate-to-lipid ratio for growth performance (Gao et al., 2010; Miao et al., 2016). The effects of different dietary CHO:L ratios on the molecular glucose and lipid metabolism in fish have rarely been investigated.
There is a strong interaction between glucose and fatty acids in fish, and this affects glucose and lipid utilization (Menoyo et al., 2006; Song et al., 2018). Dietary lipids can be converted to glucose through gluconeogenesis, and alternatively, glucose can be deposited as lipids in fish tissue (Honorato et al., 2010). The dietary lipid and carbohydrate levels regulate relative expression of genes involved in glucose and lipid metabolism. The dietary carbohydrate or glucose administration was noted to enhance lipogenesis (Kamalam et al., 2012). On the contrary, the β-oxidation data are conflicting as either stimulation (Kamalam et al., 2013) or inhibition (Jin et al., 2014) effects have been reported. Regarding long-chain polyunsaturated fatty acid (LC-PUFA) biosynthesis, the expression of induction of desaturase and elongase were also reported in freshwater fish and salmonids (Seiliez et al., 2001). But in marine fish, such induction of genes was not clear (González-Rovira et al., 2009; Vagner and Santigosa, 2011). In salmonids, desaturases and elongases were upregulated by dietary carbohydrates (Seiliez et al., 2001; Kamalam et al., 2013), but in marine fish species, such an effect has never been confirmed (Castro et al., 2015). These studies suggest that the growth performance and intermediary metabolism of fish might be affected by the interaction between dietary CHO:L ratios, which were seldom evaluated in marine fish; thus, special attention is required in carbohydrate or lipid studies.
Black seabream (Acanthopagrus schlegelii) is a popular and commercially important marine carnivorous fish species cultured in China, Japan, Korea, and some countries of Southeast Asia due to its high economic value (Nip et al., 2003; Jin et al., 2017). This species is a good candidate for intensive culture because it has many desirable characteristics, such as resistance to disease, rapid growth rate, good meat quality, and ability to tolerate environmental changes (Hong and Zhang, 2003; Shao et al., 2008; Kalhoro et al., 2018). However, the feed used for black seabream farming are trash fish traditionally, and they do not meet the nutrient requirements to sustain optimum growth and cause water pollution (Ma et al., 2008; Jin et al., 2017). The nutritional studies demonstrate that black seabream need lipid and protein in diets, approximately 14 and 40%, respectively (Shao et al., 2008; Peng et al., 2009; Zhang et al., 2010). Currently, there is no report available regarding the optimal dietary carbohydrate-to-lipid ratio requirement and glucose utilization in black seabream. The objective of the present study was to evaluate the effects of dietary CHO:L ratio on growth performance, muscle fatty acid composition, and intermediary metabolism in juvenile black seabream. Furthermore, the results obtained from this study might present some new insight into the non-protein energy utilization by fish and might facilitate the advancement of the low-protein and high-energy feed for black seabream.
Materials and Methods
Diets Preparation
All feed ingredients were purchased from Ningbo Tech-Bank Corp., Ningbo, China. The formulation and proximate composition of the experimental diet are presented in Table 1. Five isonitrogenous and isoenergetic diets (48.0% crude protein and 18.0 MJ kg–1 gross energy) were formulated to contain various CHO:L ratios ranging from 0.33 to 3.75. Fish meal and soybean meal were used as protein sources. The soybean oil and fish oil in equal amounts were used as the lipid sources, and dextrin was used as the carbohydrate source. Cellulose was used to equilibrate the carbohydrate levels required. All dry ingredients were ground into fine powder with a particle size less than 177 microns and micro-components, such as minerals and vitamin premix, were added, followed by an appropriate quantity of oil and water (35% w/w). The ground ingredients were mixed in a Hobart-type mixer until homogenous, and cold-extruded pellets were produced using a twin screws extruder (F-26, machine factory of South China University of Technology, Guangzhou, China). The pellet strands were cut off into two uniform sizes of 2 and 4 mm in diameter using a granulating machine (G-250, Machine factory of South China University of Technology, Guangzhou, China). Pellets were steamed for 30 min at 90°C. Then pellets were air-dried to approximately 10% moisture. All diets were stored at −20°C in plastic-lined bags until use in the feeding trial. The fatty acid profiles of the experimental diets were determined with few modifications (Zuo et al., 2013; Jin et al., 2017). Fatty acid methyl esters were separated and measured by GC-MS (Agilent technologies 7890B-5977A). Results are presented as a percentage of total fatty acids in Table 2.
Feeding Trial
The juvenile black seabream were purchased from a local commercial hatchery at Xiangshan Bay, Ningbo, China. All fish were acclimated for 2 weeks prior to experimentation and were fed a commercial diet (Ningbo Tech-Bank Corp, Zhejiang, China; 45% crude protein and 12% lipid) as described previously (Jin et al., 2017). At the beginning of the experiment, fish were fasted for 24 h. Then, a total of 300 juvenile black seabream of almost similar size (initial weight 0.51 ± 0.01 g) were randomly distributed into 15 300-L cylindrical fiberglass tanks filled with 250 L of water at the stocking rate of 20 fish per tank. Each experimental diet was randomly assigned to three replicates. During the feeding trial, fish were fed with experimental diets twice a day (08:00 and 17:00) to apparent satiation. All tanks were provided with a continuous flow of water (0.5 L min–1) and water was continuously aerated with air stones to maintain the dissolved oxygen level near saturation. During the experimental period, water temperature was 27–33°C, pH was 6.7–7.7, salinity was 22–26 mg L–1, ammonia nitrogen was lower than 0.05 mg L–1, and dissolved oxygen content was 6.5–7.6 mg L–1, and all were measured daily with a YSI Pro plus instrument (YSI, Yellow Springs, OH, United States). The experimental units were under a natural light and dark cycle.
Sample Collection
In the present study, all procedures complied with Chinese law pertaining to experimental animals. The protocol was approved by the Ethic-Scientific Committee for Experiments on Animals of Ningbo University. At the end of the feeding trial, fish in each tank were sampled 24 h after the last feeding. The fish in each tank were anesthetized with MS-222 (Shanghai Reagent Corp., Shanghai, China) and then individually weighed, counted, and sampled to determine survival, percentage weight gain (PWG), specific growth rate (SGR), feed conversion ratio (FCR), and protein efficiency ratio (PER). Five fish from each tank were randomly sampled and frozen at −20°C to analyze whole-body proximate composition. Hepatosomatic index (HSI), viscerosomatic index (VSI), and condition factor (CF) were determined from three individual fish per tank by obtaining tissues (livers and viscera) and expressing ratios as a percentage of body weight. Muscle samples were also collected from three fish per tank to analyze the fatty acid composition. Blood was sampled from the caudal vasculature of five fish per tank using 1 ml heparinized syringes and stored at 4°C. Then the blood samples were centrifuged at 956 × g for 10 min at 4°C to separate the serum for biochemical indices analysis. The liver from five fish after taking a blood sample in each tank were pooled into 1.5 ml eppendorf tubes and immediately frozen in liquid nitrogen and then stored at −80°C for gene expression analysis.
Proximate Composition Analysis
Proximate composition of whole body and diets were analyzed following the standard procedures of the Association of Official Analytical Chemists (AOAC, 2006). Moisture content was determined by drying the samples to a constant weight at 105°C. Crude protein (N × 6.25) was determined via the Dumas combustion method with a protein analyzer (Leco FP528, St. Joseph, MI, United States). The crude lipid was determined by the ether extraction method using the Soxhlet Method (Soxtec System HT6, Tecator, Sweden), and ash content was determined by using a muffle furnace at 550°C for 8 h. The crude fiber was analyzed by the fritted glass crucible method using an automatic analyzer (ANKOM A2000i, Macedon, New York, NY, United States).
Serum Biochemical Analysis
The serum biochemical parameters, including glucose (GLU), triglyceride (TG), cholesterol (CHOL), and total protein (TP), were measured by an automatic biochemical blood analyzer (Selectra Pro-M 13-7476). The glycogen contents in liver were determined by the assay kit (No. A043; Jian Cheng Bioengineering Institute, Nanjing, China) as previously described (Hassid and Abraham, 1957).
Fatty Acid Analysis
The fatty acid profiles of experimental diets and fish muscle tissue were determined with a few modifications (Zuo et al., 2013). The freeze-dried samples were added to 12-ml volumetric glass screw cap tubes (Teflon gasket), 3 ml potassium hydroxide in methanol (1 N) was added and heated at 72°C in a water bath for 20 min. After cooling, 3 ml of 2 N HCl in methanol was added and the mixture heated at 72°C in a water bath for 20 min. Finally, 1 ml hexane was added to the mixture, shaken vigorously for 1 min, and then permitted to separate into two layers. Fatty acid methyl esters were separated and identified by GC-MS (Agilent technologies 7890B -5977A) as previously detailed (Jin et al., 2017). Results are presented as a percentage of total fatty acids.
Total RNA Extraction, Reverse Transcription and Real-Time PCR
Total RNA was extracted from the liver tissues using TRIzol reagent (Takara, Japan) according to the manufacturer’s instructions. Quantity and quality of isolated RNA were determined spectrophotometrically (Nanodrop 2000, Thermo Fisher Scientific) and on a 1.2% denaturing agarose gel, respectively. The cDNA was generated from 1,000 ng of DNase-treated RNA and synthesized by a Prime ScriptTM RT Reagent Kit with gDNA Eraser (perfect Realtime; Takara, Japan). The housekeeping gene β-actin was used as reference gene after confirming its stability across the experimental treatment. Specific primers for the candidate genes glucokinase (gk), pyruvate kinase (pk), phosphoenolpyruvate (pepck), glucose-6-phosphatase (g6pc), glucose transporter 2 (glut2), acetyl-coA carboxylase alpha (accα), fatty acid synthase (fas), carnitine palmitoyl transferase 1 (cpt1), acyl-COA oxidase (acox1), fatty acyl desaturase (fads2), and elongase 5 (elovl5) used for qPCR were designed using Premier 3.0 software (Table 3). The primer specificities of the candidate genes were checked as previously detailed (Bustin et al., 2010) by systematically running melting curve assays after the qPCR program and DNA sequencing technology (BGI, China). Amplification was performed using a quantitative thermal cycler (Roche, Light cycler 96, Switzerland). PCR measurements were performed in a total volume of 20 μL, containing 1.0 μL of each primer, 10 μL of 2 × conc. SYBR Green I Master (Roche, Switzerland), 2 μL of cDNA, and 6 μL DEPC-water. The procedure of quantitative PCR was employed: 95°C for 2 min, followed by 45 cycles of 95°C for 10 s, 58°C for 10 s, and 72°C for 20 s. Standard curves were generated using six different dilutions (in triplicate) and the amplification efficiency was analyzed as follows: E = 10(–1/Slope) − 1 (Jothikumar et al., 2006). In this study, the gene expression was presented as relative gene expression, which we used as the relative quantification method to analyze data from RT-qPCR experiment. Expression levels of target genes were calculated using the 2–ΔΔCt method (Livak and Schmittgen, 2001).
Calculations and Statistical Analysis
The parameters were calculated as follows:
Percent weight gain (PWG, %) = 100 × (Wt−Wi) / Wi
Survival (%) = 100×(final amount of fish) / (initial amount of fish)
Specific growth rate (SGR, % day−1) = 100 × (Ln Wt−Ln Wi)/ t
Protein efficiency ratio (PER) = weight gain (g) / protein intake (g)
Condition factor (CF, g cm−3) = 100 × (body weight, g) / (body length, cm) 3
Hepatosomatic index (HSI, %) = 100 × (liver weight / whole body weight)
Viscerosomatic index (VSI, %) = 100 × (viscera weight, g) / (body weight g)
Feed conversion ratio (FCR) = feed intake (g, dry weight) / weight gain (g, wet weight)
Here, Wt is the final body weight (g), Wi is the initial body weight (g), t is the experimental duration in days. The results are presented as the means ± SE (n = 3). Prior to statistical analysis, normality and homogeneity of variance were checked, and percentage data were subjected to arcsine transformation. When the ANOVA identified significant differences between dietary treatments (p < 0.05), multiple comparisons were then made with Tukey’s test. A two-slope, broken-line regression analysis was conducted based on PWG to determine the optimum CHO:L ratio (Figure 1). All statistical analyses were performed using SPSS 23.0 (SPSS, IBM, United States).
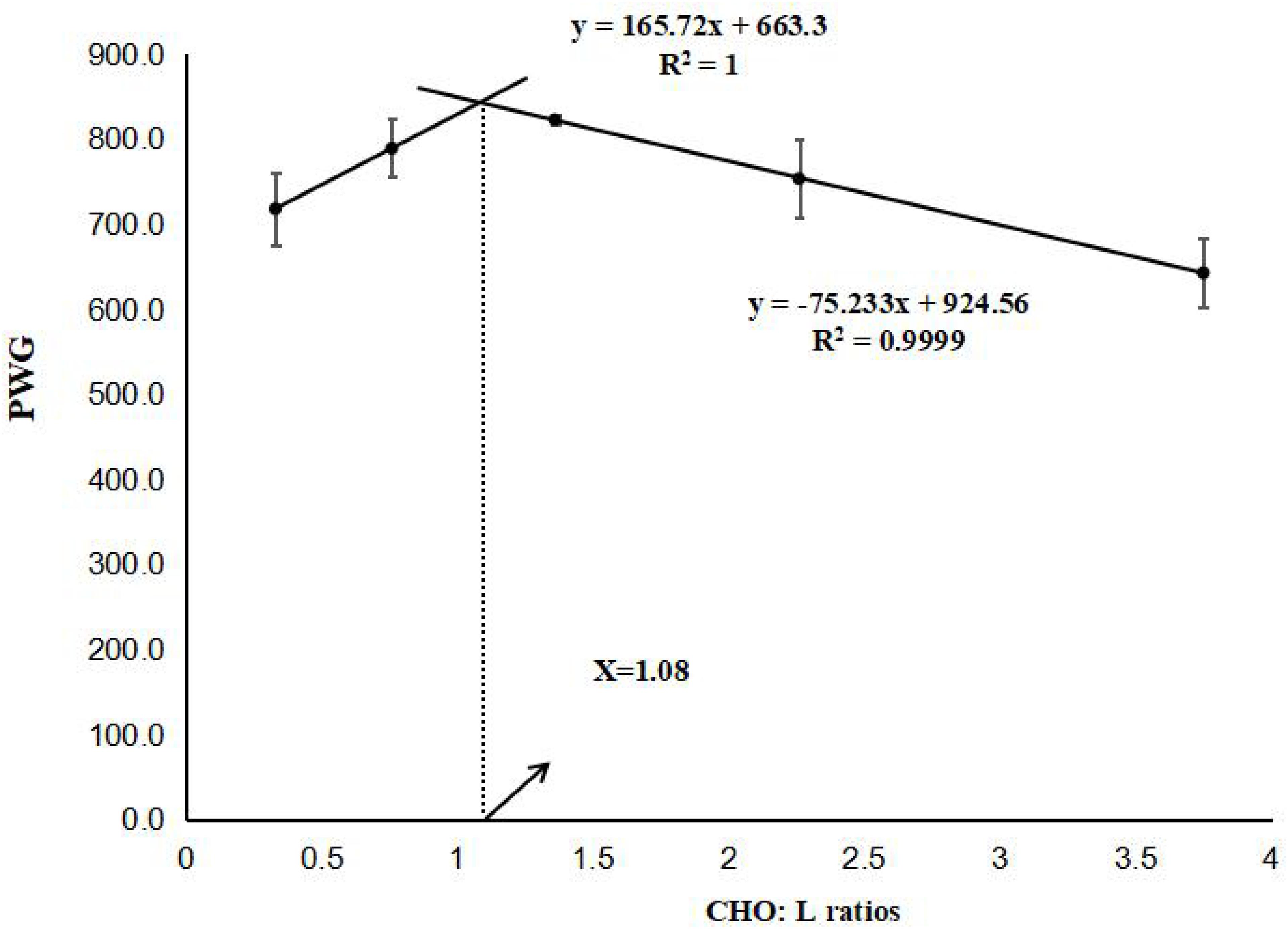
Figure 1. Relationship between percentage weight gain (PWG) and dietary (CHO:L) ratios based on two-slope, broken-line regression analysis, where Xopt represents the optimal dietary (CHO:L) ratio for the maximum PWG of black seabream.
Results
Growth Performance, Feed Utilization and Organosomatic Indices
The effects of different dietary CHO:L ratios on growth performance, feed utilization, and organosomatic indices are shown in Table 4. PWG, SGR, and PER were significantly influenced by dietary CHO:L ratio (p < 0.05). Fish fed the 1.36 CHO:L ratio diet had higher PWG, SGR, and PER than those fed the other diets. Two-slope, broken-line regression analysis of PWG against dietary CHO:L ratio indicated that the optimal dietary CHO:L ratio for juvenile black seabream is 1.08 (Figure 1). However, lower FCR was recorded in fish fed with 0.76, 1.36, and 2.26 CHO:L ratio diets than those fed the other diets. Survival ranged from 96 to 100%, and there was no significant difference among all dietary treatments (p > 0.05). HSI and VSI were significantly affected by the dietary CHO:L ratios (p < 0.05), but there were no significant differences observed in condition factor among all treatments (p > 0.05).
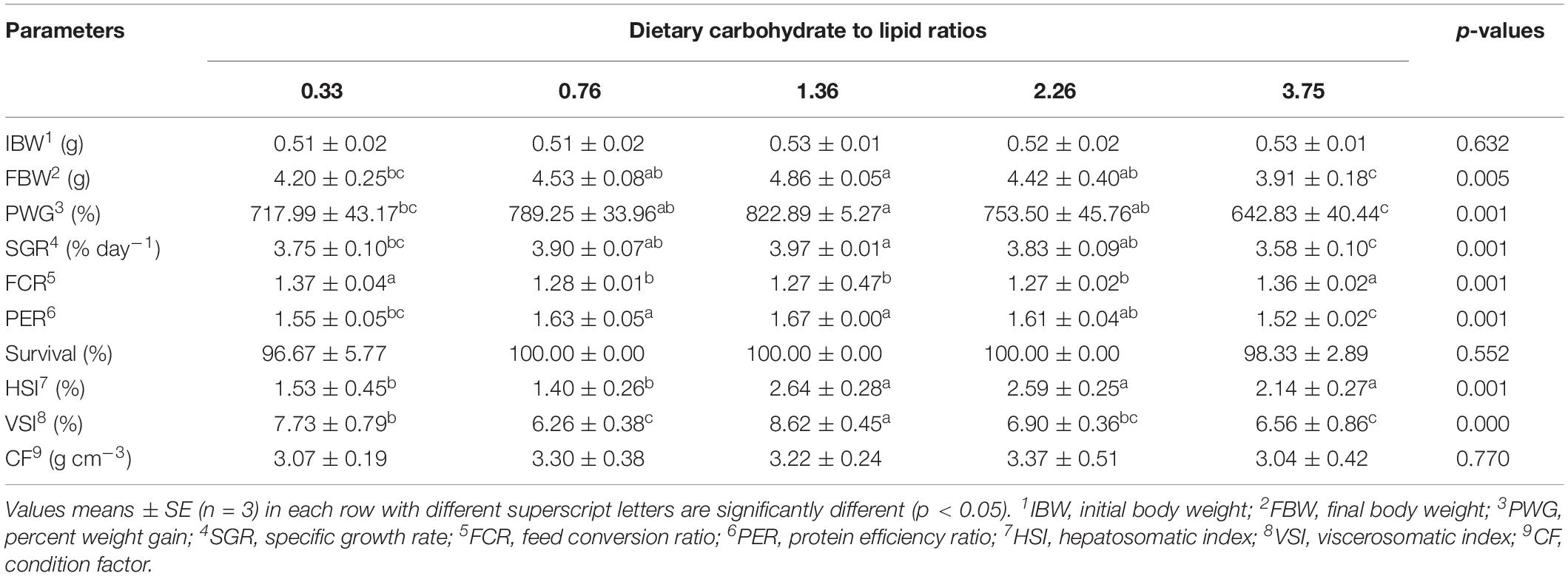
Table 4. Growth performance, feed utilization, and morphological indices of black seabream fed with experimental diets for 8 weeks.
Proximate Composition in the Whole Body
There were no significant differences in moisture, crude protein, and ash contents of the whole body among all treatments (p > 0.05). However, fish fed the 2.26 and 3.75 CHO:L diets had lower crude lipid content in the whole body than those fed the other diets (p < 0.05) (Table 5).
Serum Biochemical Parameters
The effects of dietary CHO:L ratios on contents of serum glucose (GLU), cholesterol (CHOL), triglycerides (TG), and total protein (TP) are presented in Table 6. The TG and CHOL concentrations in serum were significantly affected by dietary CHO:L ratios (p < 0.05). CHOL in serum significantly decreased with the dietary CHO:L ratios increasing from 0.33 to 3.75. However, GLU and TP concentrations in serum had no statistical differences among all dietary treatments (p > 0.05). Hepatic glycogen concentration significantly increased with the dietary CHO:L ratios increasing from 0.33 to 3.75 (p < 0.05; Table 6).
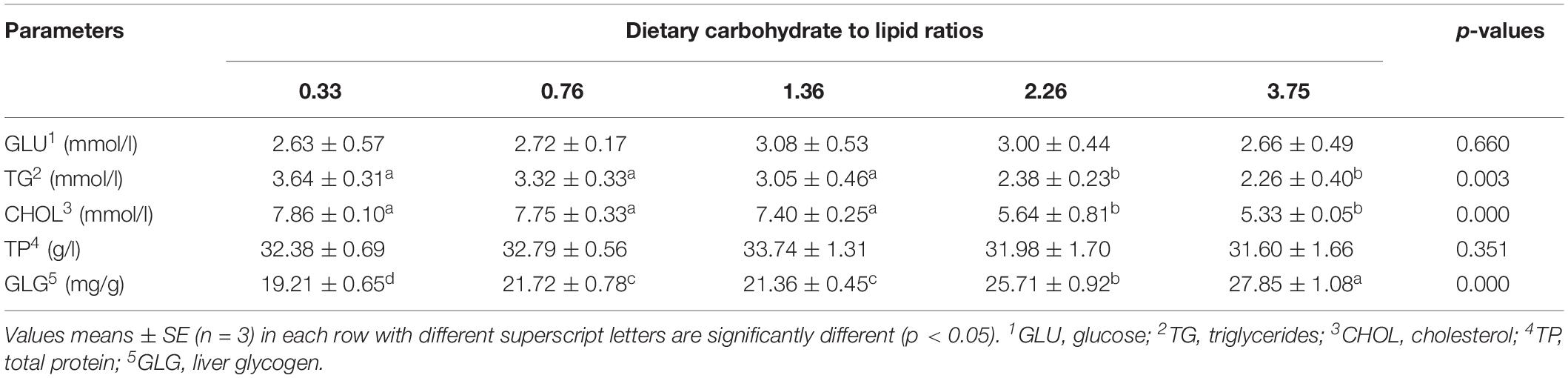
Table 6. Hematological indices and liver glycogen content of black seabream fed with experimental diets for 8 weeks.
Muscle Fatty Acid Composition
The fatty acid profiles (percentage of total fatty acids) of the muscle of black seabream fed different dietary CHO:L ratios are shown in Table 7. Overall, 19 fatty acids were observed and identified with the key fatty acids being palmitic acid (PA, C16:0), stearic acid (SA, C18:0), oleic acid (OA, C18:1n-9), linoleic acid (LA, C18:2n-6), eicosapentaenoic acid (EPA), and docosahexaenoic acid (DHA). Muscle fatty acid composition clearly reflected the dietary fatty acid composition. Significant differences were observed for most fatty acids in the muscle of black seabream fed the different dietary CHO:L ratios diets (p < 0.05). Fish fed the 1.36 CHO:L ratio diet showed significantly higher percentages of EPA, DHA, and n-3 PUFA in muscle than those fed the other diets (p < 0.05).
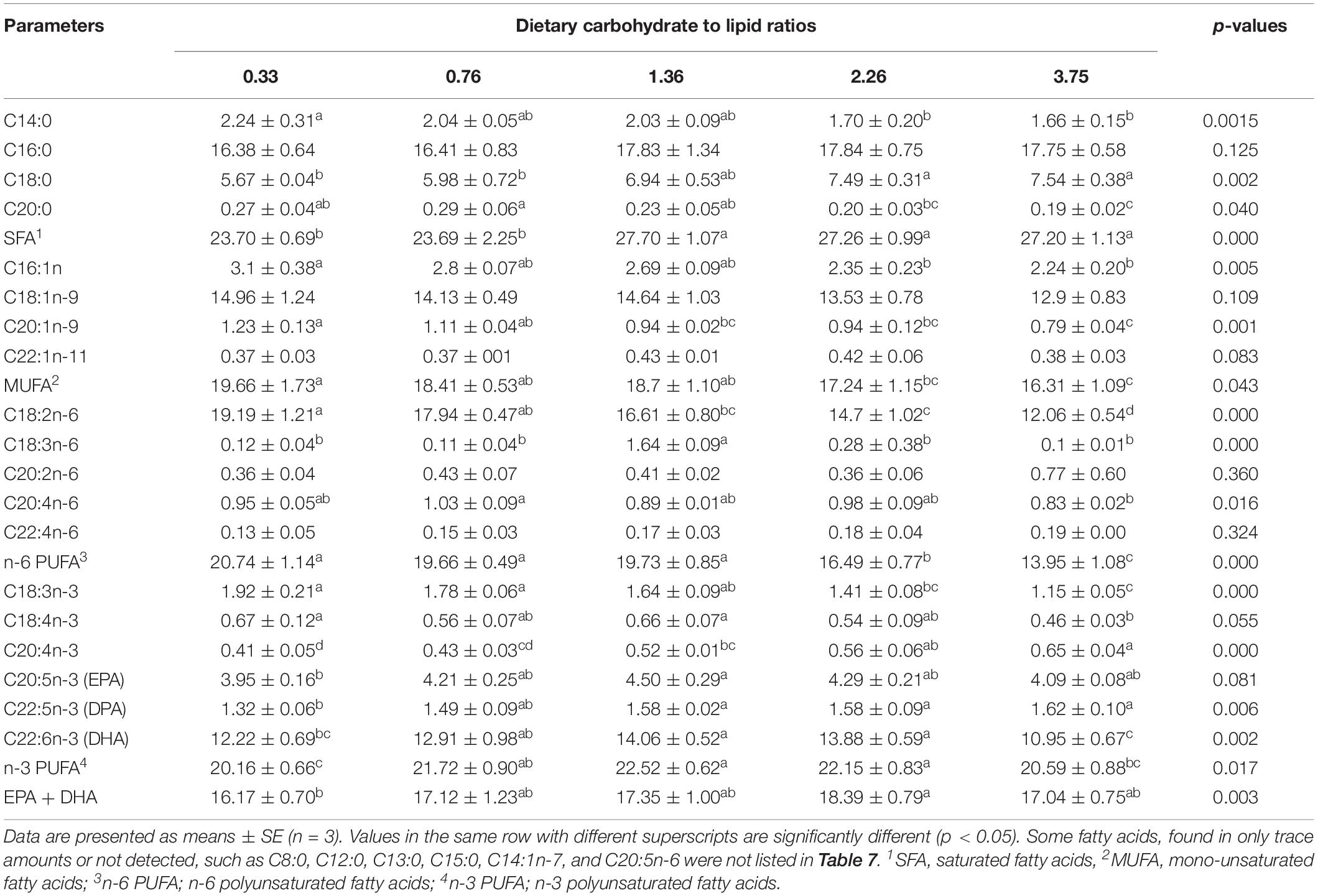
Table 7. Fatty acid composition (% of total fatty acid) of muscle of black seabream fed with experimental diets for 8 weeks.
Relative Expression of Lipid and Glucose Metabolism-Related Genes in Liver
The relative gene expression of glucose metabolism pathways in the liver of black seabream, including glycolysis (A), gluconeogenesis (B), and glucose transport (C), are shown in Figure 2. The expression level of glucokinase (gk), the key glycolytic enzyme, and of phosphoenolpyruvate carboxykinase (pepck), the key gluconeogenic enzyme, were significantly affected by dietary CHO:L ratios (p < 0.05). However, pyruvate kinase (pk) and glucose 6-phosphatase (g6pc), enzymes involved in the final step of glycolysis and gluconeogenesis, were not significantly influenced by dietary CHO:L ratios. The mRNA expression level of pepck was higher in fish fed with the carbohydrate-rich diet (p < 0.05). Moreover, the relative expression of gk significantly increased with increase of dietary CHO:L ratios. However, fish fed the 0.33 CHO:L diet had lower relative expression of glut2, which is involved in glucose transport, than those fed the 2.26 and 3.75 CHO:L ratio diets (p < 0.05).
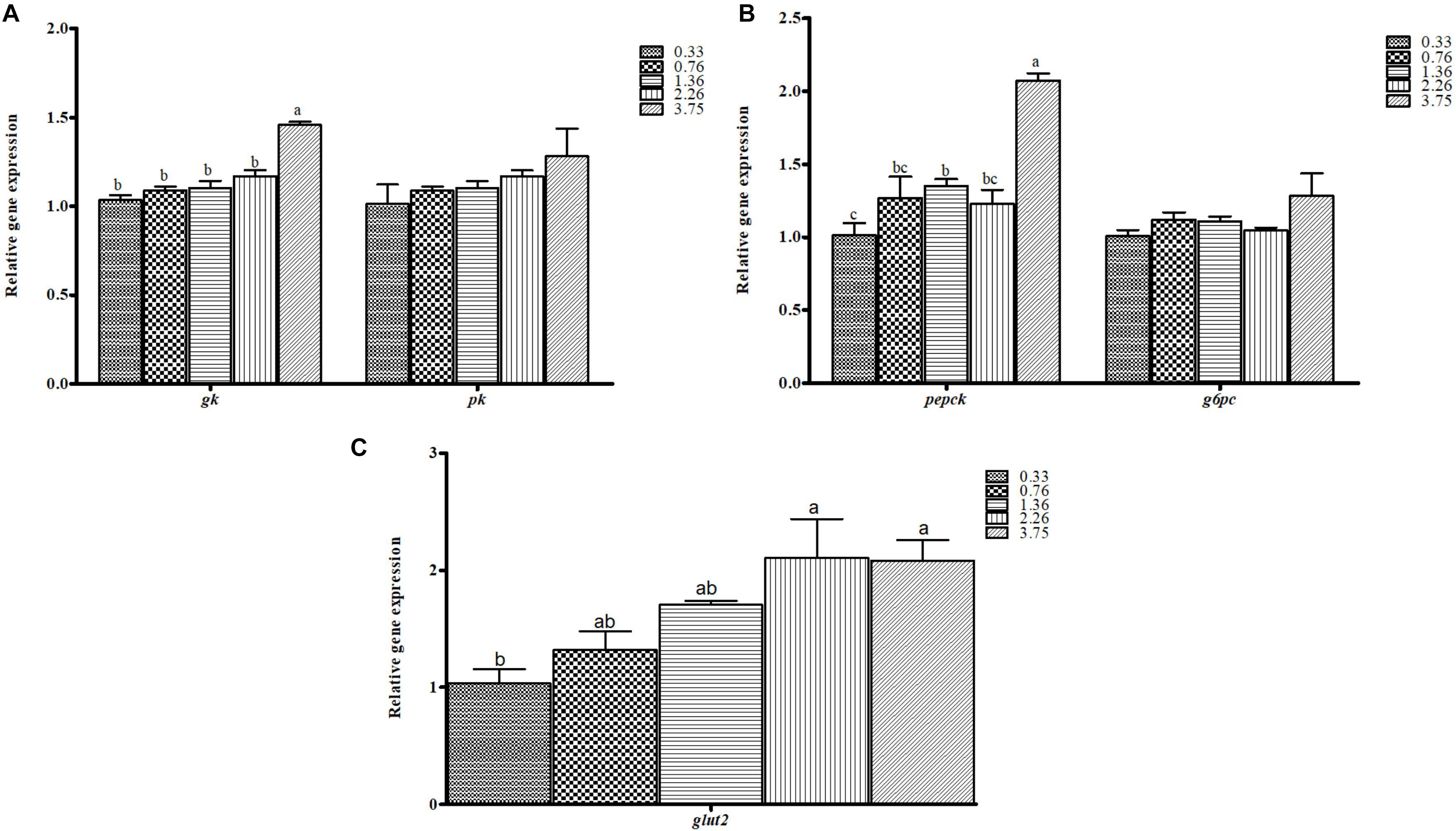
Figure 2. The mRNA expression levels of genes involved in (A) glycolysis (gk, glucokinase; pk, pyruvate kinase), (B) gluconeogenesis (pepck, phosphoenolpyruvate; g6pc, glucose-6-phosphatase), and (C) glucose transport (glut2, glucose transporter 2) in the liver of black seabream fed the different experimental diets. Expression values are normalized by β-actin. Data are expressed as means ± SE (n = 3). Values with different superscripts are significantly different (p < 0.05; Tukey’s range test).
The relative gene expressions involved in lipid biosynthesis in the liver of juvenile black seabream are shown in Figure 3. The higher mRNA expression level of lipogenic genes (fas and accα) and lower mRNA expression of fatty acid β-oxidation genes (cpt1 and acox1) were observed in fish fed with CHO:L of 1.36, 2.26, and 3.75 diets (p < 0.05). Fish fed the 1.36 CHO:L ratio had the highest expression of cpt1 and acox1 among all treatments. The mRNA expression levels of genes encoding key proteins involved in the LC-PUFA biosynthesis pathway (elovl5 and fads2) were upregulated in the liver of black seabream (p < 0.05). However, the relative expression of fads2 was significantly upregulated in fish fed the 3.75 CHO:L ratio diet.
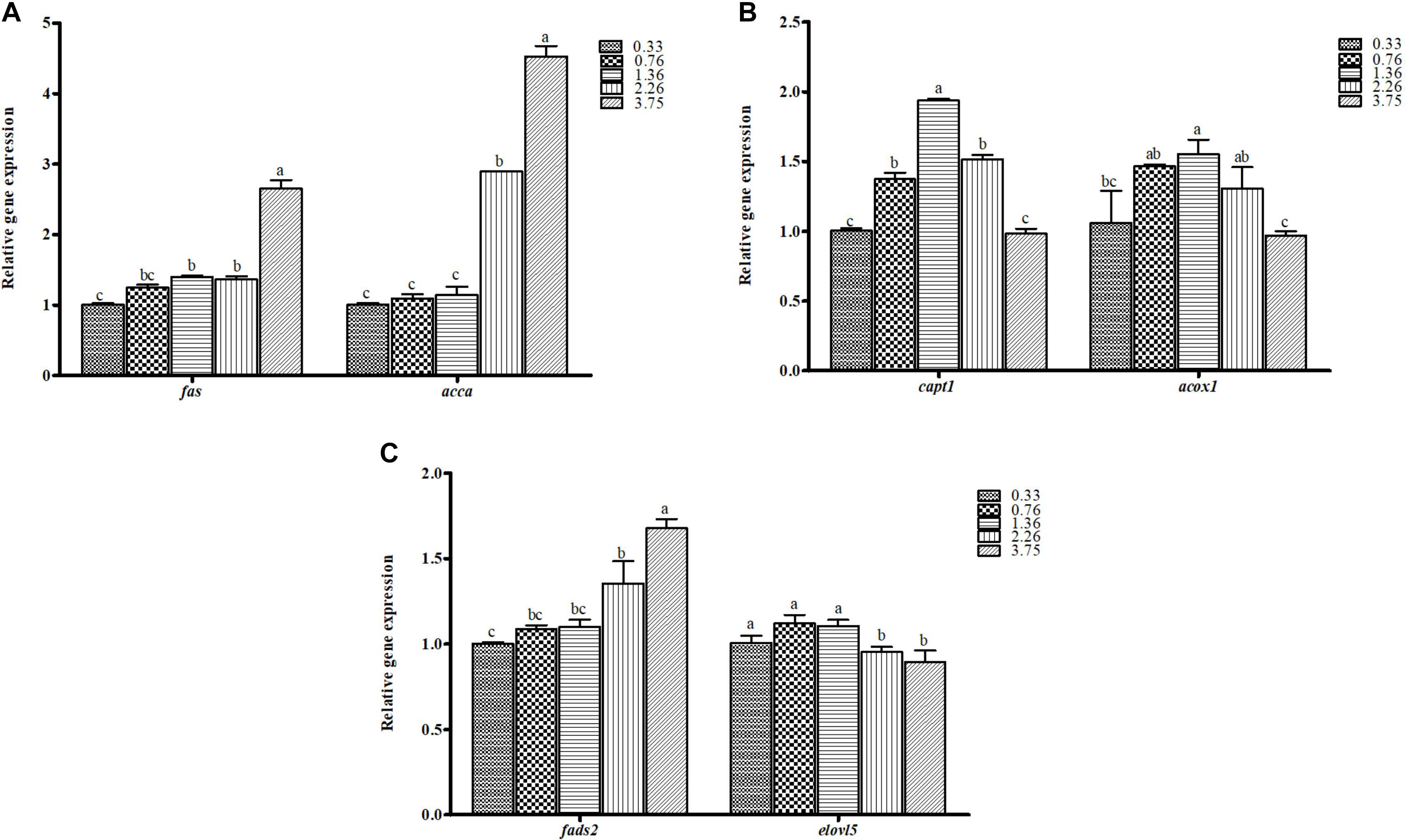
Figure 3. The mRNA expression levels of genes involved in (A) lipid synthesis (fas, fatty acid synthase; accα, acetyl-coA carboxylase alpha), (B) fatty acid β-oxidation (cpt1, carnitine palmitoyl transferase 1; acox1, acyl-CoA oxidase), and (C) long-chain PUFA biosynthesis (fads2, fatty acyl desaturase; elovl5, elongase 5) in the liver of black seabream fed the different experimental diets. Expression values are normalized by β-actin. Data are expressed as means ± SE (n = 3). Values with different superscripts are significantly different (p < 0.05; Tukey’s range test).
Discussion
The efficiency of protein can be enhanced through the utilization of carbohydrates and lipids in the diet for the cultured fish species (NRC, 2011). Various experimental studies have revealed that fish can efficiently utilize both carbohydrates and lipids to achieve better growth (Kim and Lee, 2005; Li et al., 2012a, b; Darias et al., 2015). In this study, the results demonstrated that PWG and SGR were significantly improved with dietary CHO:L ratios increasing up to 1.36, after which a decreasing trend was observed. These findings are consistent with previous studies indicating that appropriate dietary CHO:L ratios considerably improved growth performance in African catfish (Clarias gariepinus; Ali and Jauncey, 2004), dorado (Salminus brasiliensis; Moro et al., 2015), large yellow croaker (Zhou et al., 2016), and Nile tilapia (Oreochromis niloticus; Xie et al., 2017). These results also indicated that excessive dietary CHO:L ratios directly caused the growth depression in black seabream similar to other fish species (Ali and Al-Asgah, 2001; Moro et al., 2015; Xie et al., 2017). Hence, excessive increase in the input of non-protein energy sources always has a negative impact on growth performance, which appears to be closely associated with poor feed consumption by fish. Although an appropriate ratio of dietary carbohydrate and lipid is required, dietary carbohydrate is used to improve the palatability of the diet and maximize growth. However, lipids are used to fulfill the requirements for essential fatty acid in fish (Ng and Romano, 2013). In the present study, the survival was higher than 96% without any significant difference among all the treatments, suggesting that black seabream can thrive on a vast range of CHO:L ratios to attain better growth results.
Consistently, the feed ingredients not only affect the growth parameters of fish but also the physiological conditions, such as tissue physiology and plasma biochemical factors (Tian et al., 2012). In this study, the plasma triglyceride and cholesterol levels significantly decreased with increase of dietary CHO:L ratios, which was consistent with findings of other fish species (Hu et al., 2007; Zhou et al., 2016). The most favorable reason can be ascribed to active endogenous lipid transport in fish (Wang et al., 2014). However, the effects of CHO:L ratios on blood indexes, especially glucose, are still unclear, and further study is required.
In addition, a significant decrease in the whole-body lipid content with increase of dietary CHO:L ratios was observed in this study. It can be inferred that the whole-body lipid content in general accord with a previous study reported by Hu et al. (2007) that stated comparable results in yellowfin seabream (Sparus latus). However, conflicting conclusions observed in cobia (Rachycentron canadum) and European sea bass (Dicentrarchus labrax) were defined (Moreira et al., 2008; Ren et al., 2011). The present results also provided the credible fact that high carbohydrate intake resulted in a significantly high amount of HSI in fish (Brauge et al., 1994; Zuo et al., 2013). The high content of HSI with increasing levels of dietary carbohydrate was in agreement with the higher hepatic glycogen content, and these results were in accordance with the previous results on juvenile cobia (Ren et al., 2011) and rainbow trout (Oncorhynchus mykiss; Kamalam et al., 2012). The results of the present study indicate that the higher dietary carbohydrate level can promote glycogenesis and lipogenesis in fish (Moreira et al., 2008).
In the liver, the excess amount of dietary glucose is transformed into glycogen or lipids or used for energy. The production of pyruvate by glycolysis is either oxidized for energy or directed into pathways for lipogenesis (Uyeda and Repa, 2006). The glucose transportation rate and glycolysis potential can be increased by higher absorptions of carbohydrates, such as the upregulation of the hepatic genes glut2 and gk in black seabream. This is in agreement with results in gilthead sea bream (Sparus aurata) and rainbow trout (Panserat et al., 2000), where the activity of gk was intensely upregulated by a rich carbohydrate diet. Although the lack of transcriptional regulation of pk by dietary carbohydrates may probably be associated to a post-transcriptional mechanism (Enes et al., 2006), there was no transcriptional regulation of g6pc, and key gluconeogenic enzyme pepck was downregulated by dietary carbohydrates. Taking into account these outcomes, we suggest that carbohydrate catabolism is regulated at a nutritional level in this species. These analytical interpretations are in agreement with the results from European sea bass and gilthead sea bream (Enes et al., 2011), where the activity of pepck recommend that gluconeogenesis is partly regulated by dietary carbohydrates at the transcriptional level.
The lipid metabolism in the liver is a very complex process; hepatocytes not only import and export lipids via lipoprotein, but they also oxidize lipids through fatty acid oxidation or synthesize new lipid by de novo lipogenesis (Akie and Cooper, 2015). It is generally reported that fas is the significant lipogenic enzyme for the anabolic alteration of dietary carbohydrates to fatty acids (Chen et al., 2015), and accα is reflected as an important enzyme in the synthesis of long-chain poly-unsaturated fatty acids (Qian et al., 2015; Castro et al., 2016). In the present study, both fas and accα played a vital role in fatty acid biosynthesis. The expressions of fas and accα genes were all upregulated in the fish fed with a higher CHO:L ratio diet, and the results are parallel with previous work in other fish species (Xiong et al., 2014; He et al., 2015). The significant difference of these outcomes may possibly be due to the elevation of de novo lipogenesis in response to the elevated level of carbohydrates in the low-lipid diet (NRC, 2011). The gene expression of cpt1, a marker of mitochondrial FA β-oxidation was downregulated in the liver of fish fed with higher dietary carbohydrate and lower lipid diets. However, in a number of studies, the expression of the cpt1 gene was not nutritionally regulated (Kennedy et al., 2006; Morais et al., 2011; Kamalam et al., 2012). Furthermore, lipogenesis and FA β-oxidation are two different pathways generally regulated in opposite directions (Zheng et al., 2014; Bonacic et al., 2016). The downregulated expression of acox1 and cpt1 were potentially associated with increasing dietary CHO:L ratios from 2.26 to 3.75; similar results were reported for Ctenopharyngodon idellus (Li et al., 2016). The possible reason can be endorsed to the provision of digestible carbohydrates in diets that could spare the use of lipids as source of energy (Garcia-Meilan et al., 2014).
On the other hand, the high amount of ARA, EPA, and DHA in fish muscle can also affect the gene expression of cpt1 and acox1 because lipid accumulation mostly takes place when excess lipids that were consumed by fish could not be oxidized (Lu et al., 2014). Contrary to humans and other mammals, dietary excessive LC-PUFA in fish oil supplementation decreased lipogenesis and triglyceridaemia (Ikeda et al., 1998; Davidson, 2006; Harris et al., 2008), and such effects are not clear in fish. Many studies in fish showed that fish oil either depressed (Jordal et al., 2007), had no particular effects (Regost et al., 2003; Richard et al., 2006), or had contrary effects (Menoyo et al., 2004) on lipogenesis. In this study, upregulation of fads2 and elovl5 were noticed. The improved transcript levels of fads2 and elovl5 were also examined in rainbow trout (Seiliez et al., 2001; Kamalam et al., 2013) and European sea bass (Geay et al., 2010). The maximum fads2 efficiency is considered to be regulated by the levels of substrate and product availability (Vagner and Santigosa, 2011). In addition, this regulation may explain the capacity of conversion of C18 PUFA into LC-PUFA at an appreciable rate in fish species. Furthermore, the amount of n-3 LC-PUFA, principally EPA and DHA, decreased in the muscle of fish fed a carbohydrate-rich diet. The reduced n-3 LC-PUFA content in fish fed a diet with higher CHO:L ratios could be interconnected to an increase in SFA derived by lipogenesis from carbohydrates as in earlier findings in other species, such as rainbow trout and European sea bass (Alvarez et al., 1998; Castro et al., 2015). This suggested that the CHO:L ratios might affect the tissue FA composition and also FA biosynthesis of fish differently according to fish species, feeding habits, dietary carbohydrate level, and lipid sources (Castro et al., 2016). A future study concerning the dietary CHO:L ratios effect on the FA biosynthesis of black seabream is needed to elucidate. However, the levels of n-3 LC-PUFA (EPA and DHA) in the muscle of black seabream fed a dietarily optimal CHO:L ratio contributed possible health benefits to fish consumers.
Conclusion
In conclusion, based on two-slope, broken-line regression analysis, the optimal dietary CHO:L ratio is recommended to be 1.08 for juvenile black seabream. Dietary CHO:L ratio could influence the tissue fatty acid profile and the accumulation of glycogen in tissues. Moreover, dietary CHO:L ratios upregulated or downregulated relative expression levels of genes involved in glucose and lipid metabolism. The results of the present study could provide important insight for molecular studies on fish nutrition and sustainable aquaculture development of black seabream.
Data Availability Statement
The raw data supporting the conclusions of this article will be made available by the authors, without undue reservation, to any qualified researcher.
Ethics Statement
In the present study, all procedures complied with Chinese law pertaining to experimental animals. The protocol was approved by the Ethic-Scientific Committee for Experiments on Animals of Ningbo University.
Author Contributions
ST formulated the research question, designed the study, carried out the study, analyzed the data and wrote the manuscript. MJ designed the study, assisted in the correction, and developed the questions. MI designed the study, assisted in the correction, and revised the manuscript. YY assisted in the correction. HA was involved in data analysis. QZ formulated the research question, designed the study, and revised the manuscript. All the authors approved the final version of the manuscript.
Funding
This study was supported by the National Key R&D Program of China (2018YFD0900400), National Natural Science Foundation of China (31802303), Nature Science Foundation of Zhejiang Province (LY17C190002), Key Research Program of Zhejiang Province of China (2018C02037), Zhejiang Aquaculture Nutrition & Feed Technology Service Team (ZJANFTST2017-2), and Natural Science Foundation of Ningbo (2018A610343). This research was also sponsored by the K. C. Wong Magna Fund in Ningbo University.
Conflict of Interest
The authors declare that the research was conducted in the absence of any commercial or financial relationships that could be construed as a potential conflict of interest.
Acknowledgments
We expressed our thanks to the technical staff of FSN Laboratory involved in this experiment. We would also like to thank T. T. Zhu, T. T. Pan, P. Sun, X. X. Wang for their valuable assistance during the feeding trial, sampling, and chemical analysis.
Abbreviations
acc α, acetyl-CoA carboxylase alpha; acox1, acyl-CoA oxidase 1; cpt1, carnitine palmitoyl transferase 1; elovl5, elongase 5; g6pc, glucose-6-phosphatase; glut2, glucose transporter 2; gk, glucokinase; fads2, fatty acyl desaturase 2; fas, fatty acid synthase; pepck, phosphoenolpyruvate; pk, pyruvate kinase.
References
Akie, T. E., and Cooper, M. P. (2015). Determination of fatty acid oxidation and lipogenesis in mouse primary hepatocytes. J. Vis. Exp. 102:e52982. doi: 10.3791/52982
Ali, A., and Al-Asgah, N. (2001). Effect of feeding different carbohydrate to lipid ratios on the growth performance and body composition of Nile Tilapia (Oreochromis niloticus) fingerlings. Anim. Res. 50, 91–100. doi: 10.1051/animres:2001119
Ali, M., and Jauncey, K. (2004). Optimal dietary carbohydrate to lipid ratio in African catfish Clarias gariepinus. Aquac. Int. 12, 169–180. doi: 10.1023/B:AQUI.0000032065.28059.5b
Alvarez, M. J., Lopez Bote, C. J., Diez, A., Corraze, G., Arzel, J., Dias, J., et al. (1998). Dietary fish oil and digestible protein modify susceptibility to lipid peroxidation in the muscle of rainbow trout (Oncorhynchus mykiss) and sea bass (Dicentrarchus labrax). Br. J. Nutr. 80, 281–289. doi: 10.1017/S0007114598001330
AOAC (2006). Official Methods of Analysis of the Association of Official Analytical Chemists, 18th Edn. Arlington, VA: AOAC.
Bonacic, K., Estevez, A., Bellot, O., Conde-Sieira, M., Gisbert, E., and Morais, S. (2016). Dietary fatty acid metabolism is affected more by lipid level than source in Senegalese sole juveniles: interactions for optimal dietary formulation. Lipids 51, 105–122. doi: 10.1007/s11745-015-4089-6
Brauge, C., Medale, F., and Corraze, G. (1994). Effect of dietary carbohydrate levels on growth, body composition and glycaemia in rainbow trout, Oncorhynchus mykiss, reared in seawater. Aquaculture 123, 109–120. doi: 10.1016/0044-8486(94)90123-6
Bustin, S. A., Beaulieu, J. F., Huggett, J., Jaggi, R., Kibenge, F. S. B., Olsvik, P. A., et al. (2010). MIQE précis: practical implementation of minimum standard guidelines for fluorescence based quantitative real-time PCR experiments. BMC Mol. Biol. 11:74. doi: 10.1186/1471-2199-11-74
Castro, C., Corraze, G., Basto, A., Larroquet, L., Panserat, S., and Oliva-Teles, A. (2016). Dietary lipid and carbohydrate interactions: implications on lipid and glucose absorption, transport in gilthead seabream (Sparus aurata) juveniles. Lipids 51, 743–755. doi: 10.1007/s11745-016-4140-2
Castro, C., Corraze, G., Pérez-Jiménez, A., Larroquet, L., Cluzeaud, M., Panserat, S., et al. (2015). Dietary carbohydrate and lipid source affect cholesterol metabolism of European seabass (Dicentrarchus labrax) juveniles. Br. J. Nutr. 114, 1143–1156. doi: 10.1017/S0007114515002731
Chen, Q. L., Luo, Z., Huang, C., Zheng, J. L., Pan, Y. X., Song, Y. F., et al. (2015). Molecular cloning and tissue mRNA levels of 15 genes involved in lipid metabolism in Synechogobius hasta. Eur. J. Lipid Sci. Technol. 117, 471–482. doi: 10.1002/ejlt.201400164
Darias, M. J., Castro-Ruiz, D., Estivals, G., Quazuguel, P., Fernández-Mendez, C., Nunez-Rodriguez, J., et al. (2015). Influence of dietary protein and lipid levels on growth performance and the incidence of cannibalism in Pseudoplatystoma punctifer (Castelnau, 1855) larvae and early juveniles. J. Appl. Icthyol. 31, 74–82. doi: 10.1111/jai.12978
Davidson, M. H. (2006). Mechanisms for the hypotriglyceridemic effect of marine omega-3 fatty acids. Am. J. Cardiol. 98, 27–33. doi: 10.1016/j.amjcard.2005.12.024
Ellis, S. C., and Reigh, R. C. (1991). Effects of dietary lipid and carbohydrate levels on growth and body composition of juvenile red drum, Sciaenops ocellatus. Aquaculture 97, 383–394. doi: 10.1016/0044-8486(91)90330-A
Enes, P., Panserat, S., Kaushik, S., and Oliva-Teles, A. (2006). Effect of normal and waxy maize starch on growth, food utilization and hepatic glucose metabolism in European sea bass (Dicentrarchus labrax) juveniles. Comp. Biochem. Phys. A. 143, 89–96. doi: 10.1016/j.cbpa.2005.10.027
Enes, P., Panserat, S., Kaushik, S., and Oliva-Teles, A. (2011). Dietary carbohydrate utilization by European seabass (Dicentrarchus labrax L.) and gilthead seabream (Sparus aurata L.) Juveniles. Rev. Fish Sci. 19, 201–215. doi: 10.1080/10641262.2011.579363
Erfanullah, and Jafri, A. K. (1998). Effect of dietary carbohydrate to lipid ratio on growth and body composition of walking catfish (Clarias batrachus). Aquaculture 161, 159–168. doi: 10.1016/S0044-8486(97)00267-6
Gao, W., Liu, Y. J., Tian, L. X., Mai, K. S., Liang, G. Y., Yang, H. J., et al. (2010). Effect of dietary carbohydrate to lipid ratios on growth performance, body composition, nutrient utilization and hepatic enzymes activities of herbivorous grass carp (Ctenopharyngodon idella). Aquac. Nutr. 16, 327–333. doi: 10.1111/j.1365-2095.2009.00668.x
Garcia-Meilan, I., Ordóñez-Grande, B., and Gallardo, M. A. (2014). Meal timing affects protein-sparing effect by carbohydrates in sea bream: effects on digestive and absorptive processes. Aquaculture 434, 121–128. doi: 10.1016/j.aquaculture.2014.08.005
Geay, F., Santigosa, I., Culi, E., Corporeau, C., Boudry, P., Dreano, Y., et al. (2010). Regulation of FADS2 expression and activity in European sea bass (Dicentrarchus labrax. L.) fed a vegetable diet. Comp. Biochem. Phys. B 156, 237–243. doi: 10.1016/j.cbpb.2010.03.008
González-Rovira, A., Mourente, G., Zheng, X., Tocher, D. R., and Pendón, C. (2009). Molecular and functional characterization and expression analysis of a Δ6 fatty acyl desaturase cDNA of European seabass (Dicentrarchus labrax L.). Aquaculture 298, 90–100. doi: 10.1016/j.aquaculture.2009.10.012
Gümüş, E., and Ikiz, R. (2009). Effect of dietary levels of lipid and carbohydrate on growth performance, chemical contents and digestibility in rainbow trout, Oncorhynchus mykiss Walbaum, 1792. Pak. Vet. J. 29, 59–63.
Han, D., Shan, X., Zhang, W., Chen, Y., Wang, Q., Li, Z., et al. (2016). A revisit to fishmeal usage and associated consequences in Chinese aquaculture. Rev. Aquac. 10, 493–507. doi: 10.1111/raq.12183
Hardy, R. W. (2010). Utilization of plant proteins in fish diets: effects of global demand and supplies of fishmeal. Aquac. Res. 41, 770–776. doi: 10.1111/j.1365-2109.2009.02349.x
Harris, W. S., Miller, M., Tighe, A. P., Davidson, M. H., and Schaefer, E. J. (2008). Omega-3 fatty acids and coronary heart disease risk: clinical and mechanistic perspectives. Atherosclerosis 197, 12–24. doi: 10.1016/j.atherosclerosis.2007.11.008
Hassid, W. Z., and Abraham, S. (1957). Chemical procedures for analysis of polysaccharides. Method Enzymol. 3, 34–50. doi: 10.1016/S0076-6879(57)03345-5
He, A. Y., Ning, L. J., Chen, L. Q., Chen, Y. L., Xing, Q., Li, J. M., et al. (2015). Systemic adaptation of lipid metabolism in response to low and high fat diet in Nile tilapia (Oreochromis niloticus). Physiol. Rep. 3:e12485. doi: 10.14814/phy2.12485
Hong, W., and Zhang, Q. (2003). Review of captive bred species and fry production of marine fish in China. Aquaculture 227, 305–318. doi: 10.1016/S0044-8486(03)00511-8
Honorato, C. A., Almeida, L. C., Da Silva Nunes, C., Carneiro, D. J., and Moraes, G. (2010). Effects of processing on physical characteristics of diets with distinct levels of carbohydrates and lipids: the outcomes on the growth of pacu (Piaractus mesopotamicus). Aquac. Nutr. 16, 91–99. doi: 10.1111/j.1365-2095.2008.00644.x
Hu, Y. H., Liu, Y. J., Tian, L. X., Yang, H. J., Liang, G. Y., and Gao, W. (2007). Optimal dietary carbohydrate to lipid ratio for juvenile yellowfin seabream (Sparus latus). Aquac. Nutr. 13, 291–297. doi: 10.1111/j.1365-2095.2007.00476.x
Hua, K., Cobcroft, J. M., Cole, A., Condon, K., Jerry, D. R., Mangott, A., et al. (2019). The future of aquatic protein: implications for protein sources in aquaculture diets. One Earth 1, 316–329. doi: 10.1016/j.oneear.2019.10.018
Hutchins, C. G., Rawles, S. D., and Gatlin, D. M. III (1998). Effects of dietary carbohydrate kind and level on growth, body composition and glycemic response of juvenile sunshine bass (Morone chrysops ♀ × M. saxatilis.). Aquaculture 161, 187–199.
Ikeda, I., Cha, J. Y., Yanagita, T., Nakatani, N., Oogami, K., Imaizumi, K., et al. (1998). Effects of dietary α-Linolenic, eicosapentaenoic and docosahexaenoic acids on hepatic lipogenesis and β-oxidation in rats. Biosci. Biotech. Biochem. 62, 675–680. doi: 10.1271/bbb.62.675
Jin, J., Medale, F., Kamalam, B. S., Aguirre, P., Veron, V., and Panserat, S. (2014). Comparison of glucose and lipid metabolic gene expressions between fat and lean lines of rainbow trout after a glucose load. PLoS One 9:e105548. doi: 10.1371/journal.pone.0105548
Jin, M., Lu, Y., Yuan, Y., Li, Y., Qiu, H., Sun, P., et al. (2017). Regulation of growth, antioxidant capacity, fatty acid profiles, hematological characteristics and expression of lipid related genes by different dietary n-3 highly unsaturated fatty acids in juvenile black seabream (Acanthopagrus schlegelii). Aquaculture 471, 55–65. doi: 10.1016/j.aquaculture.2017.01.004
Jordal, A. E. O., Lie, O., and Torstensen, B. E. (2007). Complete replacement of dietary fish oil with a vegetable oil blend affect liver lipid and plasma lipoprotein levels in Atlantic salmon (Salmo salar L.). Aquac. Nutr. 13, 114–130. doi: 10.1111/j.1365-2095.2007.00455.x
Jothikumar, N., Robertson, B., Meng, X. J., and Hill, V. (2006). A broadly reactive one step real-time RT-PCR assay for rapid and sensitive detection of Hepatitis E virus. J. Virol. Methods 131, 65–71. doi: 10.1016/j.jviromet.2005.07.004
Kalhoro, H., Zhou, J., Hua, Y., Ng, W. K., Ye, L., Zhang, J., et al. (2018). Soy protein concentrate as a substitute for fishmeal in diets for juvenile Acanthopagrus schlegelii: effects on growth, phosphorus discharge and digestive enzyme activity. Aquac. Res. 49, 1896–1906. doi: 10.1111/are.13645
Kamalam, B. S., Medale, F., Kaushik, S., Polakof, S., Skiba-Cassy, S., and Panserat, S. (2012). Regulation of metabolism by dietary carbohydrates in two lines of rainbow trout divergently selected for muscle fat content. J. Exp. Biol. 215, 2567–2578. doi: 10.1242/jeb.070581
Kamalam, B. S., Médale, F., Larroquet, L., Corraze, G., and Panserat, S. (2013). Metabolism and fatty acid profile in fat and lean rainbow trout lines fed with vegetable oil: effect of carbohydrates. PLoS One 8:e76570. doi: 10.1371/journal.pone.0076570
Kennedy, S. R., Leaver, M. J., Campbell, P. J., Zheng, X., Dick, J. R., and Tocher, D. R. (2006). Influence of dietary oil content and conjugated linoleic acid (CLA) on lipid metabolism enzyme activities and gene expression in tissues of Atlantic salmon (Salmo salar L.). Lipids 41, 423–436. doi: 10.1007/s11745-006-5116-4
Kim, L. O., and Lee, S. M. (2005). Effects of the dietary protein and lipid levels on growth and body composition of bagrid catfish, Pseudobagrus fulvidraco. Aquaculture 243, 323–329. doi: 10.1016/j.aquaculture.2004.11.003
Li, H., Xu, W., Jin, J., Zhu, X., Yang, Y., Han, D., et al. (2019). Effects of dietary carbohydrate and lipid concentrations on growth performance, feed utilization, glucose, and lipid metabolism in two strains of gibel carp. Front. Vet. Sci. 6:165. doi: 10.3389/fvets.2019.00165
Li, X., Zhu, X., Han, D., Yang, Y., Jin, J., and Xie, S. (2016). Carbohydrate utilization by herbivorous and omnivorous freshwater fish species: a comparative study on gibel carp (Carassius auratus gibelio) and grass carp (Ctenopharyngodon idellus). Aquac. Res. 47, 128–139. doi: 10.1111/are.12476
Li, X. F., Jiang, Y., Liu, W. B., and Ge, X. P. (2012a). Protein-sparing effect of dietary lipid in practical diets for blunt snout bream (Megalobrama amblycephala) fingerlings: effects on digestive and metabolic responses. Fish. Physiol. Biochem. 38, 529–541. doi: 10.1007/s10695-011-9533-9
Li, X. F., Liu, W. B., Lu, K. L., Xu, W. N., and Wang, Y. (2012b). Dietary carbohydrate/lipid ratios affect stress, oxidative status and non specific immune responses of fingerling blunt snout bream, Megalobrama amblycephala. Fish Shellfish Immun. 33, 316–323. doi: 10.1016/j.fsi.2012.05.007
Li, X. F., Lu, K. L., Liu, W. B., Jiang, G. Z., and Xu, W. N. (2014). Effects of dietary lipid and carbohydrate and their interaction on growth performance and body composition of juvenile blunt snout bream, Megalobrama amblycephala. Isr. J. Aquac. 66:931.
Lin, Y. H., and Shiau, S. Y. (2003). Dietary lipid requirement of grouper, Epinephelus malabaricus, and effects on immune responses. Aquaculture 225, 243–250. doi: 10.1016/S0044-8486(03)00293-X
Livak, K. J., and Schmittgen, T. D. (2001). Analysis of relative gene expression data using real-time quantitative PCR and the 2-ΔΔCt method. Methods 25, 402–408. doi: 10.1006/meth.2001.1262
Lu, K. L., Xu, W. N., Wang, L. N., Zhang, D. D., Zhang, C. N., and Liu, W. B. (2014). Hepatic β-oxidation and regulation of carnitine palmitoyltransferase (CPT) I in blunt snout bream Megalobrama amblycephala fed a high fat diet. PLoS One 9:e93135. doi: 10.1371/journal.pone.0093135
Ma, J. J., Xu, Z. R., Shao, Q. J., Xu, J. Z., Hung, S. S. O., Hu, W. L., et al. (2008). Effect of dietary supplemental l-carnitine on growth performance, body composition and antioxidant status in juvenile black sea bream, Sparus macrocephalus. Aquac. Nutr. 14, 464–471. doi: 10.1111/j.1365-2095.2007.00551.x
Ma, Y., Li, M., Xie, D., Chen, S., Dong, Y., Wang, M., et al. (2020). Fishmeal can be replaced with a high proportion of terrestrial protein in the diet of the carnivorous marine teleost (Trachinotus ovatus). Aquaculture 519:734910. doi: 10.1016/j.aquaculture.2019.734910
Matulić, D., Barisić, J., Anicić, I., Tomljanovic, T., Safner, R., Treer, T., et al. (2020). Growth, health aspects and histopathology of brown bullhead (Ameiurus nebulosus L.): replacing fishmeal with soybean meal and brewer’s yeast. Sci. Rep. 10:1104. doi: 10.1038/s41598-020-57722-3
Menoyo, D., Diez, A., Lopez-Bote, C. J., Casado, S., Obach, A., and Bautista, J. M. (2006). Dietary fat type affects lipid metabolism in Atlantic salmon (Salmo salar L.) and differentially regulates glucose transporter GLUT4 expression in muscle. Aquaculture 261, 294–304. doi: 10.1016/j.aquaculture.2006.07.018
Menoyo, D., Izquierdo, M., Robaina, L., Ginés, R., Lopez, C., and Bautista, J. (2004). Adaptation of lipid metabolism, tissue composition and flesh quality in gilthead sea bream (Sparus aurata) to the replacement of dietary fish oil by linseed and soyabean oils. Br. J. Nutr. 92, 41–52. doi: 10.1079/BJN20041165
Miao, S., Nie, Q., Miao, H., Zhang, W., and Mai, K. (2016). Effects of dietary carbohydrate-to-lipid ratio on the growth performance and feed utilization of juvenile turbot (Scophthalmus maximus). J. Ocean Univ. China 15, 660–666. doi: 10.1007/s11802-016-2934-8
Morais, S., Pratoomyot, J., Torstensen, B. E., Taggart, J. B., Guy, D. R., Gordon, B. J., et al. (2011). Diet genotype interactions in hepatic cholesterol and lipoprotein metabolism in Atlantic salmon (Salmo salar) in response to replacement of dietary fish oil with vegetable oil. Br. J. Nutr. 106, 1457–1469. doi: 10.1017/S0007114511001954
Moreira, I. S., Peres, H., Couto, A., Enes, P., and Oliva-Teles, A. (2008). Temperature and dietary carbohydrate level effects on performance and metabolic utilisation of diets in European sea bass (Dicentrarchus labrax) juveniles. Aquaculture 274, 153–160. doi: 10.1016/j.aquaculture.2007.11.016
Moro, G., Silva, T., Zanon, R., and Cyrino, J. E. (2015). Starch and lipid in diets for dourado Salminus brasiliensis (Cuvier 1816): growth, nutrient utilization and digestive enzymes. Aquac. Nutr. 22, 890–898. doi: 10.1111/anu.12305
Ng, W. K., and Romano, N. (2013). A review of the nutrition and feeding management of farmed tilapia throughout the culture cycle. Rev. Aquac. 5, 220–254. doi: 10.1111/raq.12014
Nip, T., Ho, W. Y., and Wong, C. (2003). Feeding ecology of larval and juvenile black seabream (Acanthopagrus schlegeli) and Japanese seaperch (Lateolabrax japonicus) in Tolo Harbour, Hong Kong. Environ. Biol. Fish 66, 197–209. doi: 10.1023/A:1023611207492
NRC (2011). “Carbohydrates and Fibre” in Nutrient Requirements of Fish and Shrimp. Washington, DC: The National Academies Press, 135–162. doi: 10.1007/s10499-011-9480-6
Oliva-Teles, A., Enes, P., and Peres, H. (2015). “Replacing fishmeal and fish oil in industrial aquafeeds for carnivorous fish,” in Feed and Feeding Practices in Aquaculture, ed. D. A. Davis (Amsterdam: Elsevier), 203–233. doi: 10.1016/B978-0-08-100506-4.00008-8
Panserat, S., Médale, F., Blin-Wakkach, C., Brčque, J., Vachot, C., Elisabeth, P. J., et al. (2000). Hepatic glucokinase is induced by dietary carbohydrates in rainbow trout, gilthead seabream, and common carp. Am. J. Physiol. Regul. Integr. Comp. Physiol. 278, R1164–R1170. doi: 10.1152/ajpregu.2000.278.5.R1164
Peng, S., Chen, L., Qin, J. G., Hou, J., Yu, N., Long, Z., et al. (2009). Effects of dietary vitamin E supplementation on growth performance, lipid peroxidation and tissue fatty acid composition of black sea bream (Acanthopagrus schlegeli) fed oxidized fish oil. Aquac. Nutr. 15, 329–337. doi: 10.1111/j.1365-2095.2009.00657.x
Qian, Y., Li, X. F., Zhang, D. D., Cai, D. S., Tian, H. Y., and Liu, W. B. (2015). Effects of dietary pantothenic acid on growth, intestinal function, anti-oxidative status and fatty acids synthesis of juvenile blunt snout bream Megalobrama amblycephala. PLoS One 10:e0119518–e0119518. doi: 10.1371/journal.pone.0119518
Raggi, T., Tacon, A. G. J., and Lemos, D. (2019). Feeding of juvenile cobia, Rachycentron canadum: evaluation of practical feeds, comparison of commercial fishmeal replacers, and estimation of essential amino acid requirements. World Aquacult. Soc. 50, 317–335. doi: 10.1111/jwas.12587
Regost, C., Arzel, J., Robin, J., Rosenlund, G., and Kaushik, S. J. (2003). Total replacement of fish oil by soybean or linseed oil with a return to fish oil in turbot (Psetta maxima): 1. Growth performance, flesh fatty acid profile, and lipid metabolism. Aquaculture 217, 465–482. doi: 10.1016/S0044-8486(02)00259-4
Ren, M., Ai, Q., Mai, K., Ma, H., and Wang, X. (2011). Effect of dietary carbohydrate level on growth performance, body composition, apparent digestibility coefficient and digestive enzyme activities of juvenile cobia, Rachycentron canadum L. Aquac. Res. 42, 1467–1475. doi: 10.1111/j.1365-2109.2010.02739.x
Richard, N., Mourente, G., Kaushik, S., and Corraze, G. (2006). Replacement of a large portion of fish oil by vegetable oils does not affect lipogenesis, lipid transport and tissue lipid uptake in European seabass (Dicentrarchus labrax L.). Aquaculture 261, 1077–1087. doi: 10.1016/j.aquaculture.2006.07.021
Seiliez, I., Panserat, S., Kaushik, S., and Bergot, P. (2001). Cloning, tissue distribution and nutritional regulation of a Δ6-desaturase-like enzyme in rainbow trout. Comp. Biochem. Phys. B. 130, 83–93. doi: 10.1016/S1096-4959(01)00410-9
Shao, Q. J., Ma, J., Xu, Z., Hu, W., Xu, J., and Xie, S. (2008). Dietary phosphorus requirement of juvenile black seabream, Sparus macrocephalus. Aquaculture 277, 92–100. doi: 10.1016/j.aquaculture.2008.01.029
Song, X., Marandel, L., Skiba-Cassy, S., Corraze, G., Dupont-Nivet, M., Quillet, E., et al. (2018). Regulation by dietary carbohydrates of intermediary metabolism in liver and muscle of two isogenic lines of rainbow trout. Front. Physiol. 9:1579. doi: 10.3389/fphys.2018.01579
Tian, L. X., Liu, Y. J., Yang, H. J., Liang, G. Y., and Niu, J. (2012). Effects of different dietary wheat starch levels on growth, feed efficiency and digestibility in grass carp (Ctenopharyngodon idella). Aquac. Int. 20, 283–293. doi: 10.1007/s10499-011-9456-6
Uyeda, K., and Repa, J. J. (2006). Carbohydrate response element binding protein, ChREBP, a transcription factor coupling hepatic glucose utilization and lipid synthesis. Cell Metab. 4, 107–110. doi: 10.1016/j.cmet.2006.06.008
Vagner, M., and Santigosa, E. (2011). Characterization and modulation of gene expression and enzymatic activity of delta-6 desaturase in teleosts: a review. Aquaculture 315, 131–143. doi: 10.1016/j.aquaculture.2010.11.031
Wang, J., Clark, G., Ju, M., Castillo, S., and Gatlin, D. M. (2020). Effects of replacing menhaden fishmeal with cottonseed flour on growth performance, feed utilization and body composition of juvenile red drum Sciaenops ocellatus. Aquaculture 523:735217. doi: 10.1016/j.aquaculture.2020.735217
Wang, L. N., Liu, W. B., Lu, K. L., Xu, W. N., Cai, D. S., Zhang, C. N., et al. (2014). Effects of dietary carbohydrate/lipid ratios on non specific immune responses, oxidative status and liver histology of juvenile yellow catfish Pelteobagrus fulvidraco. Aquaculture 426, 41–48. doi: 10.1016/j.aquaculture.2014.01.022
Xie, D., Yang, L., Yu, R., Chen, F., Lu, R., Qin, C., et al. (2017). Effects of dietary carbohydrate and lipid levels on growth and hepatic lipid deposition of juvenile tilapia, Oreochromis niloticus. Aquaculture 479, 696–703. doi: 10.1016/j.aquaculture.2017.07.013
Xing, S., Sun, R., Pan, X., Ma, J., Zhang, W., and Mai, K. (2016). Effects of dietary carbohydrate-to-lipid ratio on growth performance, body composition, digestive enzyme activities, and hepatic enzyme activities in juvenile large yellow croaker, Larimichthys crocea. World Aquac. Soc. 47, 297–307. doi: 10.1111/jwas.12259
Xiong, Y., Huang, J., Li, X., Zhou, L., Dong, F., Ye, H., et al. (2014). Deep sequencing of the tilapia (Oreochromis niloticus) liver transcriptome response to dietary protein to starch ratio. Aquaculture 433, 299–306. doi: 10.1016/j.aquaculture.2014.06.009
Zhang, J., Zhou, F., Wang, L. L., Shao, Q. J., Xu, Z., and Xu, J. (2010). Dietary protein requirement of juvenile black seabream, Sparus macrocephalus. World Aquac. Soc. 41, 151–164. doi: 10.1111/j.1749-7345.2010.00356.x
Zheng, J. L., Luo, Z., Zhuo, M. Q., Pan, Y. X., Song, Y. F., Hu, W., et al. (2014). Dietary l-carnitine supplementation increases lipid deposition in the liver and muscle of yellow catfish (Pelteobagrus fulvidraco) through changes in lipid metabolism. Br. J. Nutr. 112, 698–708. doi: 10.1017/S0007114514001378
Zhou, P., Wang, M., Xie, F., Deng, D. F., and Zhou, Q. (2016). Effects of dietary carbohydrate to lipid ratios on growth performance, digestive enzyme and hepatic carbohydrate metabolic enzyme activities of large yellow croaker (Larmichthys crocea). Aquaculture 452, 45–51. doi: 10.1016/j.aquaculture.2015.10.010
Zuo, R., Ai, Q., and Xu, W. (2013). Effects of conjugated linoleic acid on growth, non-specific immunity, antioxidant capacity, lipid deposition and related gene expression in juvenile large yellow croaker (Larmichthys crocea) fed soybean oil-based diets. Br. J. Nutr. 110, 1–13. doi: 10.1017/S0007114513000378
Keywords: black seabream, CHO:L ratios, glycolysis, gluconeogenesis, lipogenesis, long-chain PUFA
Citation: Taj S, Irm M, Jin M, Yuan Y, Andriamialinirina HJT and Zhou Q (2020) Effects of Dietary Carbohydrate to Lipid Ratios on Growth Performance, Muscle Fatty Acid Composition, and Intermediary Metabolism in Juvenile Black Seabream (Acanthopagrus schlegelii). Front. Physiol. 11:507. doi: 10.3389/fphys.2020.00507
Received: 20 January 2020; Accepted: 24 April 2020;
Published: 03 June 2020.
Edited by:
Samad Rahimnejad, University of South Bohemia in České Budějovice, CzechiaReviewed by:
Omid Safari, Ferdowsi University of Mashhad, IranBahram Falahatkar, University of Guilan, Iran
Ebrahim Sotoudeh, Persian Gulf University, Iran
Copyright © 2020 Taj, Irm, Jin, Yuan, Andriamialinirina and Zhou. This is an open-access article distributed under the terms of the Creative Commons Attribution License (CC BY). The use, distribution or reproduction in other forums is permitted, provided the original author(s) and the copyright owner(s) are credited and that the original publication in this journal is cited, in accordance with accepted academic practice. No use, distribution or reproduction is permitted which does not comply with these terms.
*Correspondence: Min Jin, jinmin@nbu.edu.cn; Qicun Zhou, zhouqicun@nbu.edu.cn