- 1Key Laboratory of Marine Biotechnology of Fujian Province, Institute of Oceanology, Fujian Agriculture and Forestry University, Fuzhou, China
- 2Laboratory for Marine Biology and Biotechnology, Qingdao National Laboratory for Marine Science and Technology, Qingdao, China
- 3CAS Key Laboratory of Experimental Marine Biology, Institute of Oceanology, Chinese Academy of Sciences, Qingdao, China
- 4Shandong Cigna Detection Technology Co., Ltd., Qingdao, China
Fish silage (FS) has been confirmed as a high-quality feed ingredient because of its balanced nutrition, low cost, and environmental friendliness. In the present study, we evaluated the performance of replacing fishmeal by FS in the diet of white shrimp, Litopenaeus vannamei. Five isonitrogenous (410 g kg–1) and isolipidic (75 g kg–1) diets were formulated with replacement of fishmeal by 0% (FM), 25% (FS25%), 50% (FS50%), 75% (FS75%), and 100% (FS100%) FS. After an 8-week trial, shrimps fed low FS diets (FM and FS25%) had significantly higher final weight (FW), weight gain (WG), and specific growth ratio (SGR) (P < 0.05). No significant differences were found in body composition and most antioxidant enzyme activities of all groups (P > 0.05). Compared to high FS groups (FS75% and FS100%), low FS replacement levels (0 and 25%) had enhanced trypsin activity. And trypsin transcriptional level presented a similar trend with trypsin activity. In terms of intestinal histopathology, no obvious histological damage was observed in the intestine of all groups. tor and s6k of low replacement level groups (FM and FS25%) were significantly upregulated (P < 0.05), which indicated activation of mammalian target of rapamycin (mTOR) signaling pathway in low FS groups at transcriptional level. The enhanced performances of growth and mTOR signaling pathway in low FS groups (FM and FS25%) provided us some insights into the regulation mechanism of nutrient signal on growth. Based on the above, dietary FS could influence the growth of shrimp by regulating mTOR at the transcriptional level, and FS is a potential substitute of fishmeal in shrimp feed.
Introduction
With the limitation of capture fishery production, aquaculture has been growing dramatically all over the world. Especially in 2014, aquaculture sector’s contribution to the food supply for humans overtook that of capture fishery for the first time (FAO, 2017). As the scale of aquaculture continues to expand, the demand for aquafeed has also increased. Fishmeal is recognized as the most important protein source in aquafeed industry because of its nutritional quality and palatability (Abasubong et al., 2018). However, due to climate change and overfishing, fishmeal shortages and its price surge. In addition, the excessive use of fishmeal may cause a series of environmental problems because of water and gas pollution during fishmeal production process (Sales, 2009). Therefore, finding alternative sources of fishmeal has become a hot spot for aquatic animal nutrition and feed research.
Farming of white shrimp, Litopenaeus vannamei is a fast-growing aquacultural activity in the world (Pauly and Froese, 2012). In 2016, the production of white shrimp around the world reached 4.16 million tons (FAO, 2018). Thus, the use of fishmeal in white shrimp feed increased enormously in the past, and finding alternative protein sources to replace fishmeal in shrimp feed is very essential. Recently, more and more potential protein sources, such as microalgae meal (Basri et al., 2014), fermented soybean meal (Shao et al., 2019), soy protein concentrate (Xie et al., 2016), protein hydrolysates (Shao et al., 2018), yeast extract (Zhao L. et al., 2017), and biofloc meal (Shao et al., 2017) were used to replace fishmeal in shrimp feed. Although some plant protein sources have achieved good results in fishmeal replacement research, the existence of anti-nutritional factors still constrained their application in actual production (Dossou et al., 2018). Hence, we pay much more attention to replacing fishmeal by animal protein sources.
Fish silage (FS) is produced from low-value fish or fish processing waste with liquification by adding a mixture of acids and enzymes (Haider et al., 2015). Due to its balanced nutrition, low cost, and environmental friendliness, FS is considered as a high-quality feed ingredient (Olsen and Toppe, 2017). And FS has been successfully used as a substitute of fishmeal in several fish species (Liang et al., 2006; Goosen et al., 2016). Recently, more and more studies were conducted to evaluate the effect of dietary FS in shrimp at different nutrient combination conditions (Gallardo et al., 2012; Rodríguez-González et al., 2018) and biofloc system (Gonçalves et al., 2019; Lobato et al., 2019). Here, we examined the performances of replacement of fishmeal in L. vannamei diet with FS. Additionally, many experiments have been carried out to evaluate the effect of fishmeal replacement. However, the underlying mechanism that limits fishmeal replacement remains largely unknown. Mammalian target of rapamycin (mTOR) signaling pathway is a regulator of cell growth, which could be modulated by stress, amino acids, and energy (Jewell et al., 2013). It regulates protein synthesis through ribosomal protein S6 kinase (s6k) and the eukaryotic translation initiation factor 4e-binding protein (4e-bp) (Wullschleger et al., 2006). This pathway has been described as an integration point which is closely related to nutrient sensing, metabolism, and growth in multiple species (Wullschleger et al., 2006; Loewith and Hall, 2011; Weisman, 2016). More and more evidence suggested the close relationship between mTOR and growth in aquatic animals (Tang et al., 2013; Tu et al., 2015; Liang et al., 2016). The study of mTOR signaling pathway in the present research provided us new insights into the nutrient sensing and growth in shrimp.
Materials and Methods
Feed Preparation
Fish silage powder was bought from Qingdao Blue Earthworm Corporation, and its composition was shown in Table 1. Five isonitrogenous (410 g kg–1) and isolipidic (75 g kg–1) diets were formulated with replacement of fishmeal by 0% (FM), 25% (FS25%), 50% (FS50%), 75% (FS75%), and 100% (FS100%) FS, respectively (Table 2). The essential amino acid profiles of diets were listed in Table 3, and the composition reached the requirements of shrimp. Feed production process was carried out as the previous description of our laboratory (Shao et al., 2018). Finished dry diets were stored at −20°C until use.
Feeding Trial
Pathogen-free shrimps used in this study were obtained from a commercial shrimp farm (Rizhao, Shandong, China). And the experiment was conducted in a test site of Institute of Oceanology in Qingdao City, China. Prior to the feeding trial, all shrimps were acclimated for 1 week in concrete ponds feeding with commercial feed (Dale Feed Corporation, Yantai, China). Then, 750 similar size shrimps (0.26 ± 0.03 g) were randomly distributed into 15 cylindrical tanks (500 L volume, containing 400 L water and 50 shrimps, 125 shrimps/m3) with water exchange and uninterrupted oxygenation system. The five experimental diets (FM, FS25%, FS50%, FS75%, and FS100%) were randomly assigned to 15 tanks with three replicates.
A total of 8-week feeding trial was performed. Feed was offered four times a day at 5:00, 11:00, 17:00, and 23:00. Daily ration was 3–5% of total body weight per tank. Residue was collected, and water exchange was conducted twice daily. During the experiment period, water quality was kept as follows: water temperature (28–31°C), pH (7.6–8.1), salinity (29–31 ppt), dissolved oxygen (5.6–6.2 mg/L), ammonia–nitrogen (0.12–0.20 mg/L), and nitrite–nitrogen (0.02–0.08 mg/L).
Sample Collection
At the end of the feeding trial, all shrimps per tank were counted and weighed to calculate growth performance. Ten shrimps per tank were randomly collected and aseptically sacrificed. Hepatopancreas and muscle samples were rapidly frozen in liquid nitrogen and stored at −80°C for enzyme activity assays and real-time quantitative PCR analysis, and intestine was obtained for histopathology. Another 10 shrimps per tank were collected and stored at −20°C for analysis of body composition. All the experiments were conducted in accordance with the recommendations in the Guide for the Care and Use of Laboratory Animals of the National Institutes of Health (NIH). The study protocol and all experimental designs were conducted with approval from the Experimental Animal Ethics Committee of the Institute of Oceanology, Chinese Academy of Sciences.
Chemical Analysis
Chemical analysis of experimental feed and shrimp body composition was performed according to standard methods (AOAC, 2012). Moisture was calculated following drying the samples at 105°C, and ash was determined by combustion in a muffle furnace at 550°C for 24 h. Crude protein was determined by nitrogen (N × 6.25) using the Kjeldahl method (Kjeltec TM8400, FOSS, Sweden). Crude lipid was measured by Soxhlet method (Buchi 36680, Switzerland).
Enzyme Activity Assays
Hepatopancreas samples were homogenized and preprocessed as the previous study conducted by our laboratory (Shao et al., 2018). Digestive enzyme and antioxidant enzyme activities were analyzed with enzymatic kits according to the manufacturer’s protocol (Jiancheng, Nanjing, China). The source and information of each kit used in this study were as follows: lipase (Cat. No. A054-1-1), α-amylase (Cat. No. C016-1-1), trypsin (Cat. No. A080-1-1), superoxide dismutase (SOD; Cat. No. A001-3-2), glutathione peroxidase (GPX; Cat. No. A005-1-2), glutathione S-transferase (GST; Cat. No. A004-1-1), and catalase (CAT; Cat. No. A007-1-1).
Histopathology
Intestine tissues of shrimp from FM, FS25%, FS50%, FS75%, and FS100% groups were fixed in 10% formalin for 24 h, dehydrated in an ascending alcohol series (50–95%). Dehydrated tissues were embedded in paraffin and sectioned into 4-μm thick with a microtome. The 4-μm-thick tissue sections were stained with hematoxylin and eosin (H&E), then examined using an ECHO microscope (California, CA, United States). To estimate histological damage degree, a scoring system was conducted as previous studies (Labrie et al., 2003; Abad-Rosales et al., 2010). Briefly, severe, moderate, mild, and none = 100%, <75%, <25%, and 0% of the fields with histological damage, compared to the control group (FM group).
Gene Expression Analysis
Total RNA was extracted from hepatopancreas and muscle using RNA extraction kit according to the manufacturer’s instructions (Takara, Japan). The quality and yield of extracted total RNA were assessed by a 1.0% denaturing agarose gel and NanoDrop spectrophotometer (ND-2000, Thermo Fisher Scientific, United States). cDNA synthesis was performed using a One-Step gDNA Removal and cDNA Synthesis Kit according to the manufacturer’s recommendations (TransGen Biotech Co., Ltd., China). mTOR signaling pathway genes (tor and s6k) and trypsin were investigated in muscle and hepatopancreas, respectively. Specific primers were designed based on partial cDNA sequences in L. vannamei transcriptome analysis (Zhao W. et al., 2017), and β-actin was selected as a reference gene (Table 4). Real-time PCR was carried out in a sequence detection system (ABI7500, Thermo Fisher Scientific, United States) with three replicates of each sample as follows: 94°C for 30 s, then 40 temperature cycles of 94°C for 5 s and 60°C for 30 s. Gene expression level was analyzed using the 2–ΔΔCt method (Livak and Schmittgen, 2001).
Calculations and Statistical Analysis
The parameters were calculated as follows:
WT and W0 are final and initial weights (g), and T is the feeding period (56 days).
Statistical analysis was conducted using SPSS 19.0 (SPSS, Chicago, IL, United States). One-way ANOVA was used to test the main effect of dietary different feed on shrimps. Data throughout the text were presented as the means ± SD. Differences were compared using Duncan’s multiple range test after homogeneity of variance was checked. When P-value < 0.05, differences were considered statistically significant.
Results
Growth Performance
During the experimental period, shrimps fed with diets FM and FS25% performed significantly better in final weight (FW), WG, and SGR (P < 0.05), whereas diet FS100% significantly reduced growth performance of shrimps compared to the other dietary treatments (P < 0.05; Table 5). FE and PER decreased with increasing FS intake, which may be due to the poor nutritional properties of FS compared to fishmeal.

Table 5. Growth performance of shrimps fed with different experimental diets for eight weeks (means of triplicate ± SD).
Body Composition
Results of body composition were presented in Table 6. There were no significant differences in moisture, crude protein, crude lipid, and ash contents among five treatment groups (P > 0.05).

Table 6. Proximate moisture, protein, lipid, and ash (% of wet weight) composition of shrimps fed with different experimental diets for eight weeks (means of triplicate ± SD).
Enzyme Activity
No significant differences were observed in lipase and α-amylase activities among all dietary treatments (P > 0.05), whereas trypsin activity of FM and FS25% was significantly higher than that of FS75% and FS100% (P < 0.05; Table 7), which indicated that excessive FS intake negatively affected digestive enzyme activities. The mRNA transcript of trypsin in FM was significantly upregulated than that of diets with FS (P < 0.05; Figure 1). In terms of antioxidant enzymes, SOD, GST, and CAT enzyme activities showed no significant differences in all groups (P > 0.05), while GPX enzyme activity of FS100% was significantly lower than that of FM and FS25% (P < 0.05; Figure 2).

Table 7. Digestive enzyme activities in hepatopancreas of shrimps fed with different experimental diets for eight weeks (means of triplicate ± SD).
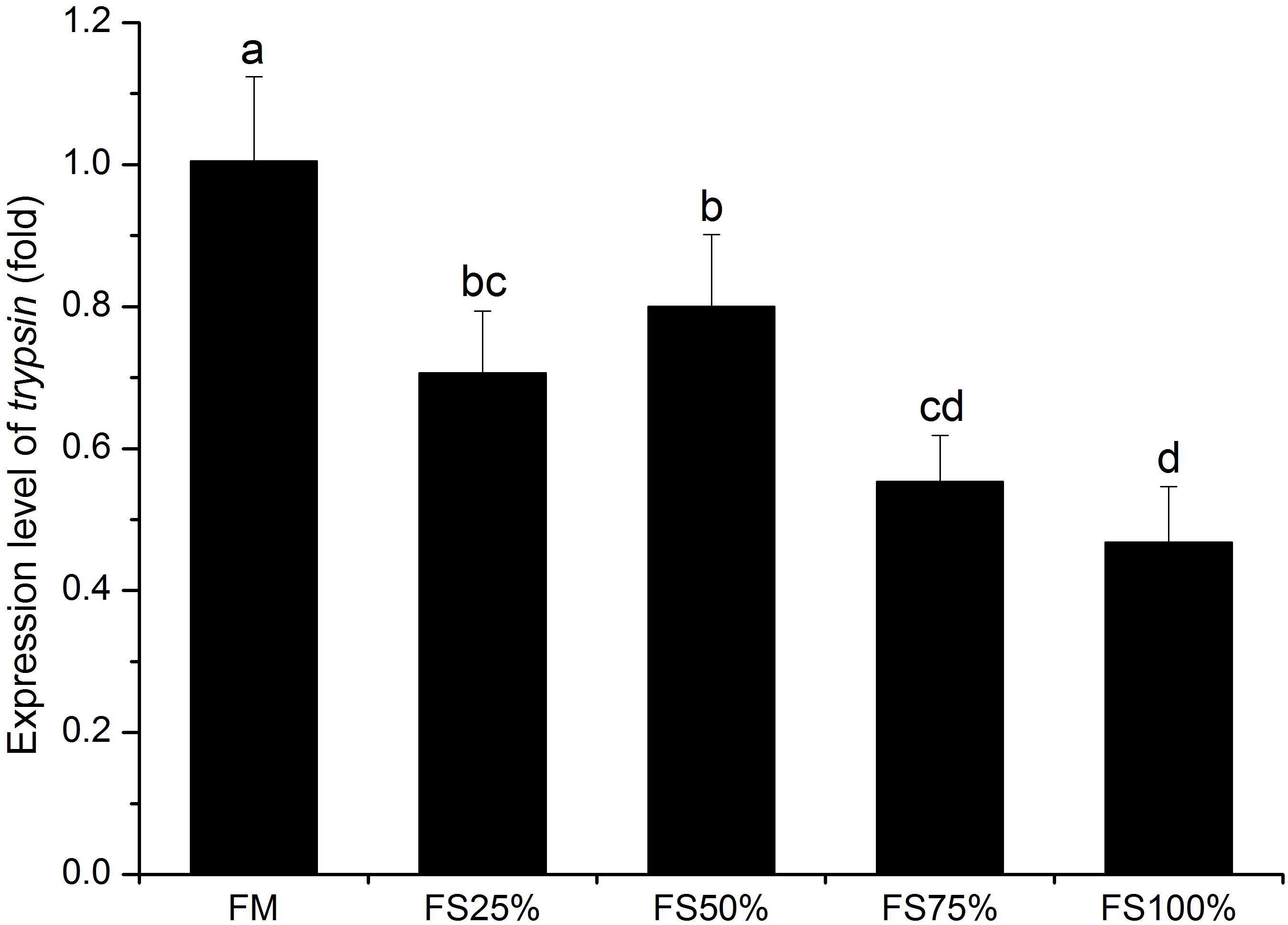
Figure 1. Relative expression level of trypsin in hepatopancreas of white shrimp (Litopenaeus vannamei) fed with five different diets. Results are shown as the mean ± SD, and different letters above a bar represent a significant difference (P < 0.05).
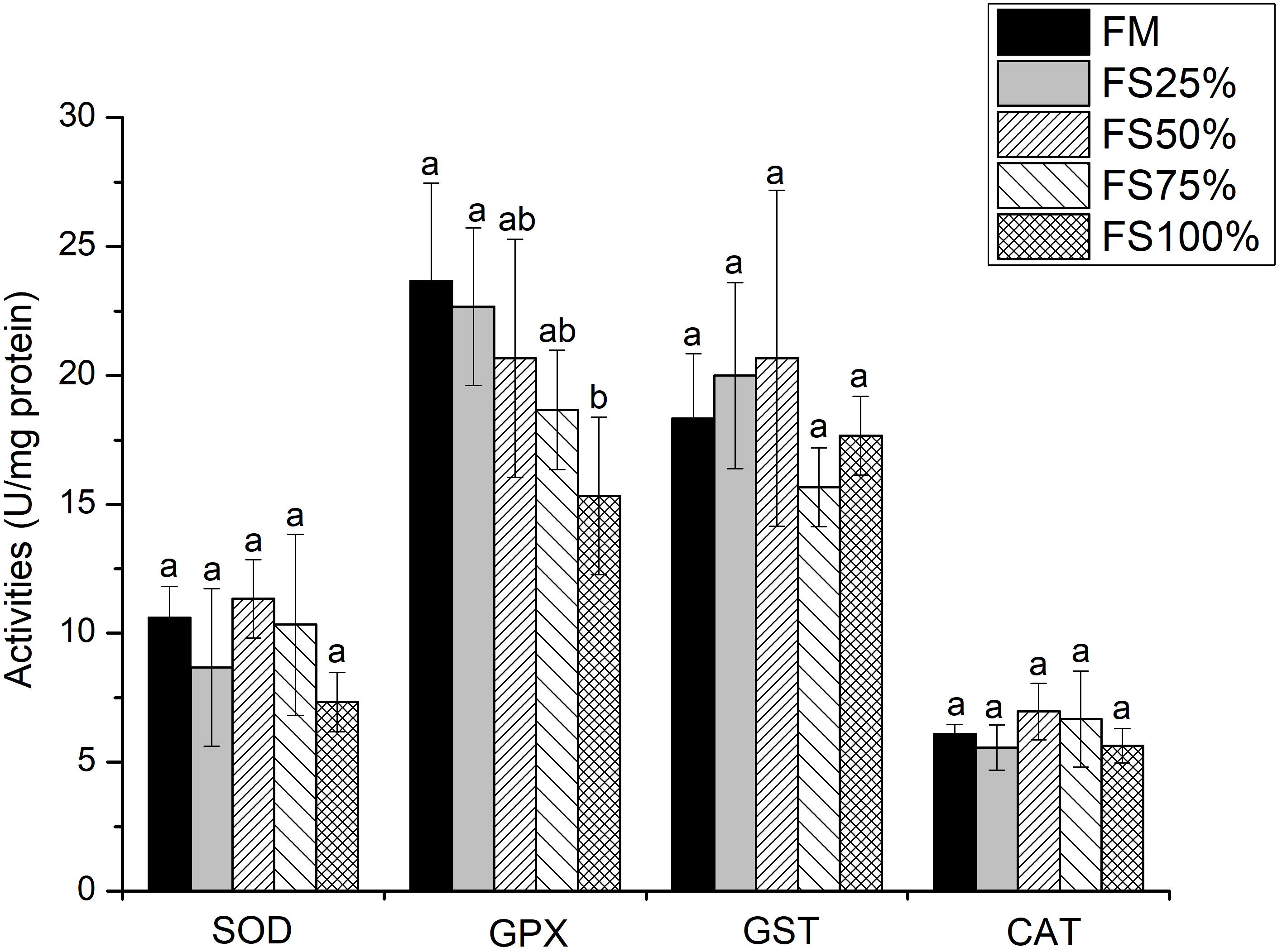
Figure 2. Specific activities of hepatopancreas antioxidant enzymes of shrimp fed with five different diets. Results are shown as the mean ± SD, and different letters above a bar represent a significant difference (P < 0.05). SOD, superoxide dismutase; GST, glutathione S-transferase; GPX, glutathione peroxidase; CAT, catalase.
Intestinal Histopathology
Histological damage scores were presented in Supplementary Table S1. No obvious histological damage was observed in terms of basement membrane (BM) thickening, increased leukocyte infiltration, epithelial necrosis, and blood capillary hyperemia in the intestine of all groups (Figure 3). But compared to high replacement level groups (FS75% and FS100%), the BM and circular muscle layers (CMLs) of low replacement level groups (FM and FS25%) were more closely combined (Figure 3 and Supplementary Table S1).

Figure 3. Histological analysis of intestine from of shrimp fed diets with 0 (a), 25% (b), 50% (c), 75% (d), and 100% (e) replacement of fishmeal by fish silage (FS). BM, basement membrane; CMLs, circular muscle layers; IEL, intestinal epithelial layer. Stained with hematoxylin and eosin (H&E), 200×.
Mammalian Target of Rapamycin Signaling Pathway
Relative expression levels of mTOR signaling pathway genes in muscle were shown in Figure 4. tor expression level showed no significant difference between FM and FS25%. Both tor and s6k expression levels of FM and FS25% were significantly upregulated than those of FS50%, FS75%, and FS100% (P < 0.05).
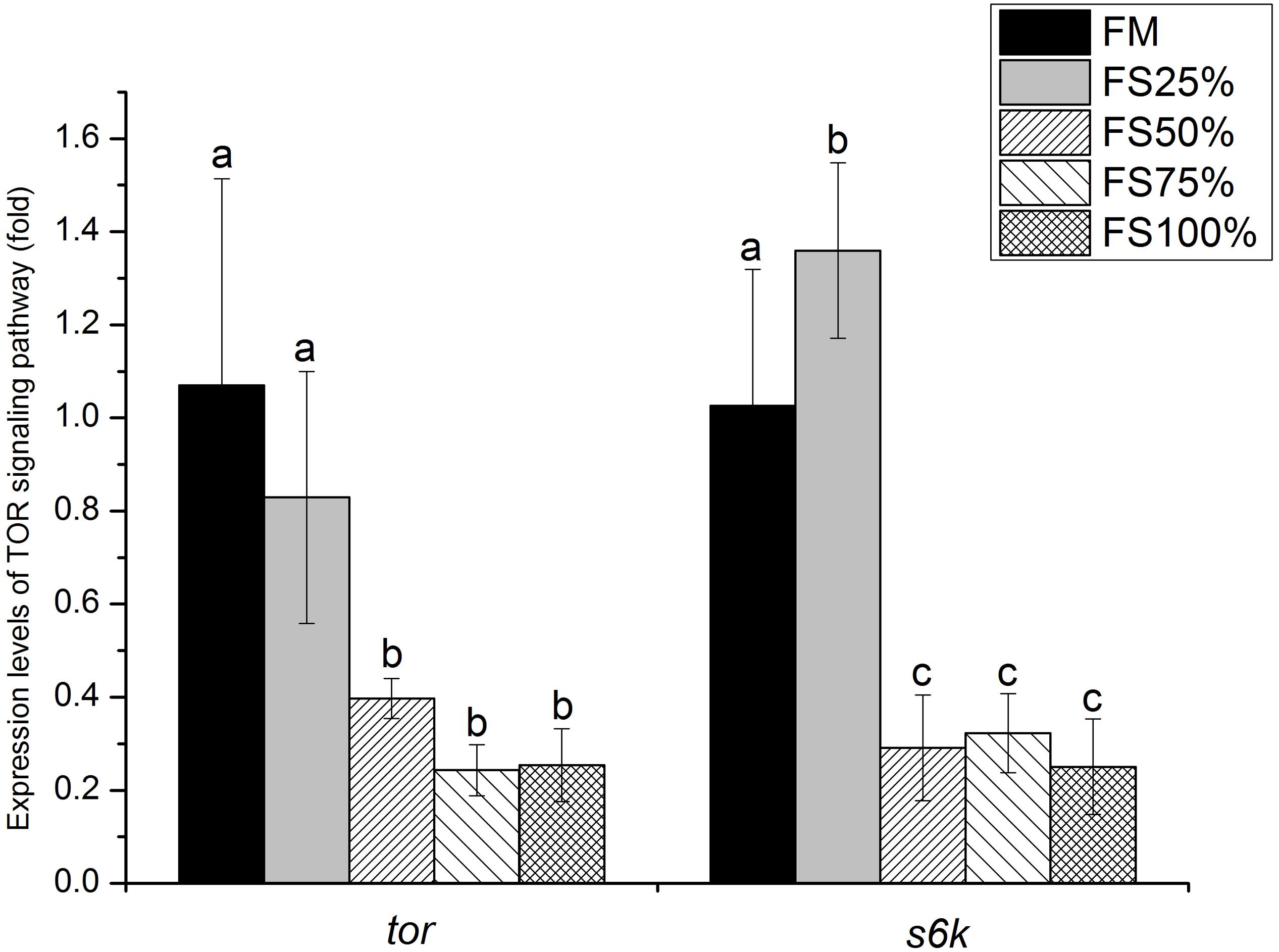
Figure 4. Relative expression levels of tor and s6k in muscle of white shrimp (Litopenaeus vannamei) fed with five different diets. Results are shown as the mean ± SD, and different letters above a bar represent a significant difference (P < 0.05).
Discussion
Fish silage is a high-quality ingredient of feed, which has been successfully used in livestock and poultry, including quails (Panda et al., 2017), lambs (Tejeda-Arroyo et al., 2015), and pigs (Nørgaard et al., 2015). In the field of aquafeed, the use of FS on replacement of fishmeal also made significant progress. Based on the study of Liang et al. (2006), enhanced growth performance was obtained in Japanese sea bass (Lateolabrax japonicus) when 15% of fishmeal in a fishmeal-based diet was replaced by FS. And recently, the study on Mozambique tilapia (Oreochromis mossambicus) also documented that low replacement level of fishmeal with FS did not cause a negative effect on growth performance (Goosen et al., 2016). Many researchers also focused on the effects of dietary FS in shrimp. Rodríguez-González et al. (2018) found that a combination of 18.75% FS/39.75% Jatropha curcas kernel meals in shrimp diets made better growth performance. And in a biofloc system, up to 6% of tilapia silage inclusion did not compromise shrimp meat quality (Gonçalves et al., 2019). We evaluated growth performance and growth-regulating gene expression levels of replacement of fishmeal in L. vannamei diet with FS in the present study.
In this research, 25% replacement of fishmeal with FS had no effects on the growth performance of shrimps compared to FM group, while high replacement levels (75 and 100%) obviously had depressed performance in FW, WG, and SGR. Although FS is a high-quality protein source, its nutritional properties still have some deficiencies compared to fishmeal, such as crude protein and essential amino acid composition (Table 1). Excessive intake of FS in the diet can cause aquaculture animals to lose growth efficiency. Therefore, based on the result of growth performance, high replacement levels of fishmeal by FS is not a suitable option. Different from previous studies (Liang et al., 2006; Goosen et al., 2016), we designed a wide range of substitution (from 25 to 100%) of fishmeal with FS in our study. Previous studies conducted in fish did not use FS to replace more than 25% fishmeal. The effect of high replacement levels of fishmeal by FS on aquatic animals remains unknown. Therefore, we try to replace fishmeal with much more FS in shrimp feed. Although high replacement levels of fishmeal by FS did not achieve satisfactory results on growth performance, it is still a significative try to alleviate fishmeal shortage.
Overproduction of reactive oxygen species (ROS) and residual ROS cause serious damage to cells and tissues. To protect themselves against damage by ROS, cells have developed a set of antioxidant defense systems, involving many antioxidant enzymes, such as SOD, GST, GPX, and CAT (Duan et al., 2015). Therefore, antioxidative capacity is an important index to reflect the healthy status of crustaceans. In the present study, SOD, GST, and CAT enzyme activities showed no significant differences in all groups, and only GPX enzyme activity of FS100% decreased significantly. While many previous studies showed that dietary high levels of plant protein sources decreased more antioxidant enzyme activities in shrimp (Xie et al., 2016; Wan et al., 2018). FS is a better protein source without anti-nutritional factors than plant protein sources. The excellent nutritional properties of FS reduce its negative effect on the antioxidant enzyme activities of shrimp.
Intestinal histopathology was conducted to evaluate the intestinal health of shrimp fed with different levels of FS. No obvious histological damage was observed in the intestine of all groups, which indicated the excellent nutrition characteristics of FS for shrimp. It is also found that the BM and CMLs of low replacement level group (FM and FS25%) were more closely combined. The loose connection between BM and CMLs in high replacement level groups (FS75% and FS100%) may lead to barriers to nutrient absorption and transportation, which is related to the worse growth performance of high replacement level groups.
Trypsin plays a vital role in the assimilation of nutrition in hepatopancreas of shrimps (Muhliaalmazan, 2003). In our present study, dietary low FS replacement levels (0 and 25%) made positive effects on both trypsin activity and trypsin gene expression, which may contribute to growth of shrimp in low FS groups. Many previous studies also have found a close relationship between digestive enzyme activity and growth in shrimp. Based on the study of Pakravan et al. (2017), dietary 50% replacement level of fishmeal by microalgae stimulated trypsin activity and further enhanced growth of shrimp. More recently, Huang et al. (2019) found that dietary T-2 toxin decreased both digestive enzyme activity and growth performance. These evidences demonstrated the important effect of trypsin on grow performance in shrimp.
The vital role of trypsin in growth of shrimp has been verified by our present research and some previous studies (Sha et al., 2016; Pakravan et al., 2017), while the downstream regulation pathway of trypsin remains unknown. Amino acid concentration could be sensed to modulate mTOR signaling pathway activity and further regulate cell growth (Jewell et al., 2013). Trypsin activity directly affects the efficiency of protein digestion. In the present study, key genes (tor and s6k) of mTOR signaling pathway in low FS groups (FM and FS25%) were significantly upregulated at the transcriptional level. Interestingly, low FS groups also had higher trypsin activity and better growth performance. We speculated that trypsin activity influenced amino acid concentration in shrimp tissue and further provided mTOR signaling pathway with a signal to regulate cell growth. In addition, mTOR signaling pathway has been confirmed as an effector of cell growth and proliferation via the regulation of protein synthesis in model species and mammals (Miron et al., 2003; Fingar and Blenis, 2004; Loewith and Hall, 2011; Kakanj et al., 2016). In aquatic animals, several studies showed that dietary essential amino acid supplementation can activate TOR signaling pathway and further influenced the growth of fish (Tang et al., 2013; Ren et al., 2015; Wu et al., 2017). And Shao et al. (2018) found that dietary biofloc meal could make a difference on the key genes of mTOR signaling pathway and growth performance of shrimp. Therefore, we believe that different dietary replacement levels of fishmeal by FS could influence the growth of white shrimp by regulating the mTOR signaling pathway.
Conclusion
Fish silage is a kind of high-quality feed ingredient because of its balanced nutrition, low cost, and environmental friendliness. In the present study, we evaluated the potential of replacing fishmeal by FS in feed of white shrimp. The results indicated that replacement of fishmeal by FS at 25% performed better than high replacement levels on growth performance. And we further found that dietary FS could regulate growth of shrimp by mTOR signaling pathway. In brief, FS is a potential substitute of fishmeal in shrimp feed to reduce the pressure of demand for fishmeal.
Data Availability Statement
The datasets generated for this study are available on request to the corresponding author.
Ethics Statement
The animal study was reviewed and approved by the Experimental Animal Ethics Committee of Institute of Oceanology, Chinese Academy of Sciences.
Author Contributions
JS and ML made most contributions to this research, such as experiment design, sample collection, analysis of data, and drafting the manuscript, etc. LW made contribution to the design of this study and drafting the manuscript. XS participated in sample collection and analysis.
Funding
This work was supported by China Agriculture Research System (CARS-47), the National Natural Science Foundation of China (41406151) and the Marine S&T Fund of Shandong Province for Pilot National Laboratory for Marine Science and Technology (Qingdao) (No. 2018SDKJ0502-2).
Conflict of Interest
XS was employed by the company Shandong Cigna Detection Technology Co., Ltd.
The remaining authors declare that the research was conducted in the absence of any commercial or financial relationships that could be construed as a potential conflict of interest.
Supplementary Material
The Supplementary Material for this article can be found online at: https://www.frontiersin.org/articles/10.3389/fphys.2020.00359/full#supplementary-material
References
Abad-Rosales, S. M., Frías-Espericueta, M. G., Inzunza-Rojas, A., Osuna-López, I., Páez-Osuna, F., Lozano-Olvera, R., et al. (2010). Histological effects of Cu2+ to white shrimp Litopenaeus vannamei (Crustacea: Decapoda) juveniles at low salinities. Revist. Biol. Mar. Oceanogr. 45, 99–105.
Abasubong, K. P., Liu, W. B., Zhang, D. D., Yuan, X. Y., Xia, S. L., Xu, C., et al. (2018). Fishmeal replacement by rice protein concentrate with xylooligosaccharides supplement benefits the growth performance, antioxidant capability and immune responses against Aeromonas hydrophila in blunt snout bream (Megalobrama amblycephala). Fish Shellfish Immunol. 78, 177–186. doi: 10.1016/j.fsi.2018.04.044
AOAC, (2012). Official Methods for Analysis, 19th Edn. Washington, DC: Association of Official Analytical Chemists.
Basri, N. A., Shaleh, S. R. M., Matanjun, P., Noor, N. M., and Shapawi, R. (2014). The potential of microalgae meal as an ingredient in the diets of early juvenile Pacific white shrimp, Litopenaeus vannamei. J. Appl. Phycol. 27, 857–863. doi: 10.1007/s10811-014-0383-6
Dossou, S., Koshio, S., Ishikawa, M., Yokoyama, S., Dawood, M. A. O., El Basuini, M. F., et al. (2018). Effect of partial replacement of fish meal by fermented rapeseed meal on growth, immune response and oxidative condition of red sea bream juvenile, Pagrus major. Aquaculture 490, 228–235. doi: 10.1016/j.aquaculture.2018.02.010
Duan, Y., Zhang, J., Dong, H., Wang, Y., Liu, Q., and Li, H. (2015). Oxidative stress response of the black tiger shrimp Penaeus monodon to Vibrio parahaemolyticus challenge. Fish Shellfish Immunol. 46, 354–365. doi: 10.1016/j.fsi.2015.06.032
FAO, (2017). Fishery and Aquaculture Statistics 2015. Rome: Food and Agriculture Organization of the United Nations.
FAO, (2018). Fishery and Aquaculture Statistics 2016. Rome: Food and Agriculture Organization of the United Nations.
Fingar, D. C., and Blenis, J. (2004). Target of rapamycin (TOR): an integrator of nutrient and growth factor signals and coordinator of cell growth and cell cycle progression. Oncogene 23, 3151–3171. doi: 10.1038/sj.onc.1207542
Gallardo, P., Gaxiola, G., Soberano, S., Taboada, J. G., Perez, M., Rosas, C., et al. (2012). Nutritive value of diets containing fish silage for juvenile Litopenaeus vannamei (Bonne, 1931). J. Sci. Food. Agric. 92, 2320–2325. doi: 10.1002/jsfa.5632
Gonçalves, A. A., Emerenciano, M. G. C., Ribeiro, F. D. A. S., and Soares Neto, J. D. R. (2019). The inclusion of fish silage in Litopenaeus vannamei diets and rearing systems (biofloc and clear-water) could affect the shrimp quality during subsequent storage on ice? Aquaculture 507, 493–499. doi: 10.1016/j.aquaculture.2019.04.032
Goosen, N. J., de Wet, L. F., and Görgens, J. F. (2016). Rainbow trout silage as immune stimulant and feed ingredient in diets for Mozambique tilapia (Oreochromis mossambicus). Aquac. Res. 47, 329–340. doi: 10.1111/are.12497
Haider, M. S., Ashraf, M., Azmat, H., Khalique, A., Javid, A., Atique, U., et al. (2015). Nutritive evaluation of fish acid silage in Labeo rohita fingerlings feed. J. Appl. Anim. Res. 44, 158–164. doi: 10.1080/09712119.2015.1021811
Huang, Z., Wang, Y., Qiu, M., Sun, L., Deng, Y., Wang, X., et al. (2019). Effects of T-2 toxin on digestive enzyme activity, intestinal histopathology and growth in shrimp Litopenaeus vannamei. Sci. Rep. 9:13175. doi: 10.1038/s41598-019-49004-4
Jewell, J. L., Russell, R. C., and Guan, K. L. (2013). Amino acid signalling upstream of mTOR. Nat. Rev. Mol. Cell Biol. 14, 133–139. doi: 10.1038/nrm3522
Kakanj, P., Moussian, B., Gronke, S., Bustos, V., Eming, S. A., Partridge, L., et al. (2016). Insulin and TOR signal in parallel through FOXO and S6K to promote epithelial wound healing. Nat. Commun. 7:12972. doi: 10.1038/ncomms12972
Labrie, L., Roque, A., Gomez-Gil, B., and Turnbull, J. F. (2003). Effect of methyl parathion on the susceptibility of shrimp Litopenaeus vannamei to experimental vibriosis. Dis. Aquat. Organ. 57, 265–270. doi: 10.3354/dao057265
Liang, H., Ren, M., Habte-Tsion, H. M., Ge, X., Xie, J., Mi, H., et al. (2016). Dietary arginine affects growth performance, plasma amino acid contents and gene expressions of the TOR signaling pathway in juvenile blunt snout bream, Megalobrama amblycephala. Aquaculture 461, 1–8. doi: 10.1016/j.aquaculture.2016.04.009
Liang, M., Wang, J., Chang, Q., and Mai, K. (2006). Effects of different levels of fish protein hydrolysate in the diet on the nonspecific immunity of Japanese sea bass, Lateolabrax japonicus (Cuvieret Valenciennes, 1828). Aquac. Res. 37, 102–106. doi: 10.1111/j.1365-2109.2005.01392.x
Livak, K. J., and Schmittgen, T. D. (2001). Analysis of relative gene expression data using real-time quantitative PCR and the 2−ΔΔCT method. Methods 25, 402–408. doi: 10.1006/meth.2001.1262
Lobato, O. S. C., de Azevedo Silva Ribeiro, F., Miranda-Baeza, A., and Emerenciano, M. G. C. (2019). Production performance of Litopenaeus vannamei (Boone, 1931) fed with different dietary levels of tilapia processing waste silage reared in biofloc system using two carbon sources. Aquaculture 501, 515–518. doi: 10.1016/j.aquaculture.2018.12.006
Loewith, R., and Hall, M. N. (2011). Target of rapamycin (TOR) in nutrient signaling and growth control. Genetics 189, 1177–1201. doi: 10.1534/genetics.111.133363
Millamena, O. M., Bautista-Tereul, M. N., Reyes, O. S., and Kanazawa, A. (1997). Threonine requirement of juvenile marine shrimp Penaeus monodon. Aquaculture 151, 9–14.
Millamena, O. M., Bautista-Tereul, M. N., Reyes, O. S., and Kanazawa, A. (1998). Requirements of juvenile marine shrimp, Penaeus monodon for lysine and arginine. Aquaculture 164, 95–104. doi: 10.1016/s0044-8486(98)00179-3
Millamena, O. M., Bautista-Tereul, M. N., Reyes, O. S., and Kanazawa, A. (1999). Quantitative dietary requirement of postlarval tiger shrimp, Penaeus monodon, for histidine, isoleucine, leucine, phenylalanine and tryptophan. Aquaculture 179, 169–179. doi: 10.1016/s0044-8486(99)00160-x
Miron, M., Lasko, P., and Sonenberg, N. (2003). Signaling from Akt to FRAP/TOR targets both 4E-BP and S6K in Drosophila melanogaster. Mol. Cell. Biol. 23, 9117–9126. doi: 10.1128/mcb.23.24.9117-9126.2003
Molina-Poveda, C., Lucas, M., and Jover, M. (2013). Evaluation of the potential of Andean lupin meal (Lupinus mutabilis Sweet) as an alternative to fish meal in juvenile Litopenaeus vannamei diets. Aquaculture 410, 148–156. doi: 10.1016/j.aquaculture.2013.06.007
Muhliaalmazan, A. (2003). Effects of dietary protein on the activity and mRNA level of trypsin in the midgut gland of the white shrimp Penaeus vannamei. Comp. Biochem. Physiol. B Biochem. Mol. Biol. 135, 373–383. doi: 10.1016/s1096-4959(03)00091-5
Nørgaard, J. V., Petersen, J. K., Tørring, D. B., Jørgensen, H., and Lærke, H. N. (2015). Chemical composition and standardized ileal digestibility of protein and amino acids from blue mussel, starfish, and fish silage in pigs. Anim. Feed Sci. Technol. 205, 90–97. doi: 10.1016/j.anifeedsci.2015.04.005
Olsen, R. L., and Toppe, J. (2017). Fish silage hydrolysates: not only a feed nutrient, but also a useful feed additive. Trends Food Sci. Tech. 66, 93–97. doi: 10.1016/j.tifs.2017.06.003
Pakravan, S., Akbarzadeh, A., Sajjadi, M. M., Hajimoradloo, A., and Noori, F. (2017). Partial and total replacement of fish meal by marine microalga Spirulina platensis in the diet of Pacific white shrimp Litopenaeus vannamei: growth, digestive enzyme activities, fatty acid composition and responses to ammonia and hypoxia stress. Aquac. Res. 48, 5576–5586. doi: 10.1111/are.13379
Panda, S., Babu, L. K., Panda, A. K. S. T., Mohanty, A., Panigrahy, K. K., and Samal, P. (2017). Effect of dietary supplementation of fermented fish silage on serum biochemical parameters of broiler Japanese quails (Coturnix coturnix japonica). Vet. World 10, 380–385. doi: 10.14202/vetworld.2017.380-385
Pauly, D., and Froese, R. (2012). Comments on FAO’s state of fisheries and aquaculture, or ‘SOFIA 2010’. Mar. Policy 36, 746–752. doi: 10.1016/j.marpol.2011.10.021
Ren, M., Habte-Tsion, H. M., Liu, B., Miao, L., Ge, X., Xie, J., et al. (2015). Dietary leucine level affects growth performance, whole body composition, plasma parameters and relative expression of TOR and TNF-α in juvenile blunt snout bream, Megalobrama amblycephala. Aquaculture 448, 162–168. doi: 10.1016/j.aquaculture.2015.06.008
Richard, L., Blanc, P. P., Rigolet, V., Kaushik, S. J., and Geurden, I. (2010). Maintenance and growth requirements for nitrogen, lysine and methionine and their utilisation efficiencies in juvenile black tiger shrimp, Penaeus monodon, using a factorial approach. Br. J. Nutr. 103, 984–995. doi: 10.1017/S0007114509992844
Rodríguez-González, H., López-Aguilar, M. R., Fonseca-Madrigal, J., Martínez-Palacios, C. A., and García-Ulloa, M. (2018). Use of a mixture of vegetal (Jatropha curcas) and animal (fish silage) byproducts as protein source in shrimp (Litopenaeus vannamei) diets. Revist. Bras. Zootec. 47:e20170165. doi: 10.1590/rbz4720170165
Sales, J. (2009). The effect of fish meal replacement by soyabean products on fish growth: a meta-analysis. Br. J. Nutr. 102:1709. doi: 10.1017/s0007114509991279
Sha, Y., Wang, L., Liu, M., Jiang, K., Xin, F., and Wang, B. (2016). Effects of lactic acid bacteria and the corresponding supernatant on the survival, growth performance, immune response and disease resistance of Litopenaeus vannamei. Aquaculture 452, 28–36. doi: 10.1016/j.aquaculture.2015.10.014
Shao, J., Zhao, W., Liu, X., and Wang, L. (2018). Growth performance, digestive enzymes, and TOR signaling pathway of Litopenaeus vannamei are not significantly affected by dietary protein hydrolysates in practical conditions. Front. Physiol. 9:998. doi: 10.3389/fphys.2018.00998
Shao, J., Liu, M., Wang, B., Jiang, K., Wang, M., and Wang, L. (2017). Evaluation of biofloc meal as an ingredient in diets for white shrimp Litopenaeus vannamei under practical conditions: effect on growth performance, digestive enzymes and TOR signaling pathway. Aquaculture 479, 516–521. doi: 10.1016/j.aquaculture.2017.06.034
Shao, J., Zhao, W., Han, S., Chen, Y., Wang, B., and Wang, L. (2019). Partial replacement of fishmeal by fermented soybean meal in diets for juvenile white shrimp (Litopenaeus vannamei). Aquac. Nutr. 25, 145–153. doi: 10.1111/anu.12838
Tang, L., Feng, L., Sun, C. Y., Chen, G. F., Jiang, W. D., Hu, K., et al. (2013). Effect of tryptophan on growth, intestinal enzyme activities and TOR gene expression in juvenile Jian carp (Cyprinus carpio var. Jian): studies in vivo and in vitro. Aquaculture 412, 23–33. doi: 10.1016/j.aquaculture.2013.07.002
Tejeda-Arroyo, E., Cipriano-Salazar, M., Camacho-Diaz, L. M., Salem, A. Z., Kholif, A. E., Elghandour, M. M., et al. (2015). Diet inclusion of devil fish (Plecostomus spp.) silage and its impacts on ruminal fermentation and growth performance of growing lambs in hot regions of Mexico. Trop. Anim. Health Prod. 47, 861–866. doi: 10.1007/s11250-015-0800-0
Teshima, S., Alam, M. S., Koshio, S., Ishikawa, M., and Kanazawa, A. (2002). Assessment of requirement values for essential amino acids in the prawn, Marsupenaeus japonicus (Bate). Aquac. Res. 33, 395–402. doi: 10.1046/j.1365-2109.2002.00684.x
Tu, Y., Xie, S., Han, D., Yang, Y., Jin, J., and Zhu, X. (2015). Dietary arginine requirement for gibel carp (Carassis auratus gibelio var. CAS III) reduces with fish size from 50g to 150g associated with modulation of genes involved in TOR signaling pathway. Aquaculture 449, 37–47. doi: 10.1016/j.aquaculture.2015.02.031
Wan, M., Yin, P., Fang, W., Xie, S., Chen, S. J., Tian, L. X., et al. (2018). The effect of replacement of fishmeal by concentrated dephenolization cottonseed protein on the growth, body composition, haemolymph indexes and haematological enzyme activities of the Pacific white shrimp (Litopenaeus vannamei). Aquac. Nutr. 24, 1845–1854. doi: 10.1111/anu.12823
Weisman, R. (2016). Target of rapamycin (TOR) regulates growth in response to nutritional signals. Microbiol. Spectr. 4, 1–13. doi: 10.1128/microbiolspec.FUNK-0006-2016
Wullschleger, S., Loewith, R., and Hall, M. N. (2006). TOR signaling in growth and metabolism. Cell 124, 471–484. doi: 10.1016/j.cell.2006.01.016
Wu, M., Lu, S., Wu, X., Jiang, S., Luo, Y., Yao, W., et al. (2017). Effects of dietary amino acid patterns on growth, feed utilization and hepatic IGF-I, TOR gene expression levels of hybrid grouper (Epinephelus fuscoguttatus ♀× Epinephelus lanceolatus ♂) juveniles. Aquaculture 468, 508–514. doi: 10.1016/j.aquaculture.2016.11.019
Xie, S. W., Liu, Y. J., Zeng, S., Niu, J., and Tian, L. X. (2016). Partial replacement of fish-meal by soy protein concentrate and soybean meal based protein blend for juvenile Pacific white shrimp, Litopenaeus vannamei. Aquaculture 464, 296–302. doi: 10.1016/j.aquaculture.2016.07.002
Zhao, L., Wang, W., Huang, X., Guo, T., Wen, W., Feng, L., et al. (2017). The effect of replacement of fish meal by yeast extract on the digestibility, growth and muscle composition of the shrimp Litopenaeus vannamei. Aquac. Res. 48, 311–320. doi: 10.1111/are.12883
Keywords: Litopenaeus vannamei, fish silage, growth performance, intestinal histopathology, mammalian target of rapamycin signaling pathway
Citation: Shao J, Wang L, Shao X and Liu M (2020) Dietary Different Replacement Levels of Fishmeal by Fish Silage Could Influence Growth of Litopenaeus vannamei by Regulating mTOR at Transcriptional Level. Front. Physiol. 11:359. doi: 10.3389/fphys.2020.00359
Received: 29 October 2019; Accepted: 27 March 2020;
Published: 06 May 2020.
Edited by:
Francesca Carella, University of Naples Federico II, ItalyReviewed by:
Wei Zhao, Jinan University, ChinaJin Niu, Sun Yat-sen University, China
Martha Guadalupe Nieto López, Autonomous University of Nuevo León, Mexico
Copyright © 2020 Shao, Wang, Shao and Liu. This is an open-access article distributed under the terms of the Creative Commons Attribution License (CC BY). The use, distribution or reproduction in other forums is permitted, provided the original author(s) and the copyright owner(s) are credited and that the original publication in this journal is cited, in accordance with accepted academic practice. No use, distribution or reproduction is permitted which does not comply with these terms.
*Correspondence: Jianchun Shao, shaojianchun16@mails.ucas.ac.cn; Mei Liu, liumei@qdio.ac.cn