- 1College of Life and Environmental Sciences, Hangzhou Normal University, Hangzhou, China
- 2Institute of Plant and Environment Protection, Beijing Academy of Agriculture and Forestry Sciences, Beijing, China
Harmonia axyridis is an important predatory insect and widely used in biological control of agricultural and forestry pests. Trehalose is directly involved in the energy storage of the H. axyridis and in the oxidative function of various physiological activities thereby providing an energy source for its growth and development. The aim of this study was to explore the potential function of membrane-bound-like trehalase (TRE2-like) and membrane-bound trehalase (TRE2) genes in H. axyridis by RNAi. In addition, the activity of soluble and membrane-bound trehalase and the expression of genes related to trehalose and glycogen metabolism were determined in the larvae injected with dsTRE2-like or dsTRE2. The results showed that wing abnormality and mortality appeared in adults, as well as the activity of soluble trehalase and glycogen contents increased when interfering with TRE2-like gene. However, the activity of membrane-bound trehalase, trehalose and glucose contents in the larvae decreased. The expression of glycogen synthase (GS) and glycogen phosphorylase (GP) genes were decreased after RNAi in the ecdysis stage. The expression of chitin synthase gene A (CHSA), chitin synthase gene B (CHSB), and trehalose-6-phosphate synthase genes (TPS) were decreased significantly after RNAi, especially in the ecdysis stage. These results indicated that RNA interference is capable of knocking down gene expression of TRE2-like and TRE2, thereby disrupting trehalose metabolism which affects the chitin synthesis pathway in turn and also leads to developmental defects, such as wing deformities. This study could provide some theoretical guidance for the function of TRE2 gene in other insects.
Introduction
Harmonia axyridis (Pallas) (Coleoptera: Coccinellidae) is an important natural enemy of aphids and other pests (Nakayama et al., 2010; Wu et al., 2016; Adachi-Hagimori et al., 2019). The typical breeding strategy of H. axyridis involves multiple mating and thus increasing its population (Awad et al., 2017; Du et al., 2019). When faced with various natural threats, H. axyridis adapts with different strategies, such as gathering in winter to protect against cold (Durieux et al., 2015) and releasing harmful exudates to expel natural enemies (Schmidtberg et al., 2019). As an immediate source of energy, trehalose plays a vital role in the energy storage of the H. axyridis, in the oxidative function of various physiological activities, and in the growth and development (Ge et al., 2011; Xie et al., 2013; Kojić et al., 2018; Pathak et al., 2018; Zhao et al., 2018). Trehalose is called the “blood sugar of insects” (Yu et al., 2008; Wu et al., 2019), and it is a non-reducing disaccharide composed of glucose units linked by two glycosides and is found in bacteria, yeasts, fungi, plants, insects, and other invertebrates (Elbein et al., 2003; Frison et al., 2007; Tang et al., 2008). Trehalose is widely distributed in different tissues and expressed in various development stages of insects, and mainly synthesized in the fat body of insects and released into the hemolymph rapidly (Thompson, 2003).
The decomposition of trehalose is catalyzed by a specific α-glucosidase-trehalase (TRE) (Tang et al., 2018). Trehalase hydrolyzes the α-1, 1-glycosidic linkage of trehalose to release two glucose molecules (Shukla et al., 2015; Łopieńska-Biernat et al., 2019). Trehalase was discovered in Aspergillus niger in 1893 and was classified into two types, namely, soluble trehalase (TRE1) and membrane-bound trehalase (TRE2) (Forcella et al., 2010; Ma et al., 2015). It has been identified in several insect species, such as Apis mellifera (Lee et al., 2007), Aphis glycines (Bansal et al., 2013), Spodoptera exigua (Tang et al., 2008; Zou et al., 2013), Tribolium castaneum (Parkinson et al., 2003; Tang et al., 2016), Nilaparvata lugens (Zhao et al., 2016), and Chironomus ramosus (Shukla et al., 2018). The first identified trehalase in insects was soluble trehalase cloned in Tenebrio molitor (Takiguchi et al., 1992). In addition, TRE2 was first discovered in silkworm in 2005 (Mitsumasu et al., 2005). Studies have shown that trehalase regulates insect homeostasis and development, and plays an important role in insect ecdysis (Chen et al., 2010; Tan et al., 2014).
TRE2 is an exogenous transmembrane enzyme involved in the hydrolysis of exogenous trehalose to provide energy (de Almeida et al., 2009). Some TRE2 proteins are present in an inactive state, but when activated they can destroy membrane integrity (Wegener et al., 2003). These are mainly present in the mitochondria as well as in the brain, stratum, cuticle, and midgut, with active sites outside the cell (Tang et al., 2008). In insects, TRE2 is involved in several physiological processes such as flight, reproduction, development, and digestion (Wegener et al., 2010). TRE2 gene has been cloned in a variety of insects, mainly in the fat body, midgut, and Malpighian tube. Gene expression patterns have shown differences among insect species suggesting a potential functional diversification of the trehalase enzymes during their evolution (Nardelli et al., 2019). There might be only two kinds of trehalases in insects, however, a membrane-bound-like trehalase (TRE2-like) that is a class of membrane-free proteins with high homology to membrane-bound proteins has been reported in Locusta migratoria manilensis (Liu et al., 2016). TRE2-like is likely to be an intermediate type of soluble trehalase, which is similar to the TRE2, with dominant and negative forms reported by Wegener et al. (2003), but TRE2-like functional role is still unclear. Trehalose is catabolized by trehalase, and it regulates energy metabolism and glucose production, which is a concern to be addressed in insect physiology (Zhang et al., 2019b). Studies on the function of TRE1 gene homologs are common, but studies on the specific functions of homologous genes of TRE2 are rare. Moreover, the action mechanisms of TRE2-like and TRE2 genes in insects remain unclear and little is known about their structure, tissue distribution, and expression patterns. Therefore, it is necessary to further understand and explore their functions.
As TRE genes are known to be vital to insect growth and development (Zhang et al., 2019a), the present study investigated the functions of TRE2-like and TRE2 genes in H. axyridis using RNAi via the injection of double-stranded RNA (dsRNA) corresponding to conserved TRE2-like and TRE2 genes structure. In this study, we aimed to synthesize dsRNA fragments from the conserved domains of TRE2-like and TRE2 genes from the TRE sequence of H. axyridis, analyzed the expression and function of TRE2-like, and TRE2 in the third and fourth instar larvae of H. axyridis. The results will elucidate the trend in gene expression, explain the metabolic mechanism, and provide a theoretical basis for exploring the function of TRE2-like and TRE2 genes.
Materials and Methods
Insects
Harmonia axyridis individuals were raised in the Key Laboratory of Animal Adaptation and Evolution of Hangzhou Normal University. They were maintained in an artificial climate chamber at 25 ± 1°C, 70 ± 5% relative humidity, and 14:10 h photoperiod. H. axyridis individuals were fed Aphis medicaginis (Homoptera, Aphididae) at a fixed time every day. The third and fourth instar larvae of H. axyridis were collected for microinjection; they were analyzed 48 h after injection and during ecdysis. About one hundred individuals were injected per treatment. Then, 3 to 5 individuals were selected from each injection group for Total RNA isolating which were repeated three times.
Total RNA Isolation, dsRNA Synthesis, and Treatments
TRIzol (Invitrogen, Carlsbad, CA, United States) was used to extract total RNA from H. axyridis. The total RNA integrity was determined by 1% agarose gel electrophoresis. The concentration and purity of the total RNA were determined using the NanoDrop 2000 spectrophotometer (Thermo Fisher Scientific, Waltham, MA, United States). The cDNA template was synthesized according to the instruction manual of the PrimeScript® RT Reagent Kit (NARISHIGE, JAPAN) and gDNA Eraser Reverse Transcription Kit; the reverse transcription product was stored at −20°C. According to the TRE2-like (KX349224.1, 2,133 bp, Supplementary Image S1) and TRE2 (KX349225.1, 2,374 bp, Supplementary Image S2) genes coding regions of H. axyridis, specific primers with the T7 promoter sequence in the 5′ end were designed. Based on the cDNA of H. axyridis (Table 1), fragments of the TRE2-like and TRE2 genes were amplified by real-time polymerase chain reaction (RT-PCR). The PCR conditions were as follows: 40 cycles at 95°C for 30 s, 58°C for 30 s, and 72°C for 45 s, and finally extended at 72°C for 10 min. The purified TRE amplicon synthesized in vitro was used to synthesize dsRNA using the T7 RiboMAX Express RNAi System (Promega Corporation, Madison, WI, United States) (Zhao et al., 2016). The samples were DNase and RNase free. The integrity of the dsRNA was detected by 1.5% agarose gel electrophoresis, and the concentration was determined using a micro spectrophotometer (Narishige, Japan). Moreover, the dsRNA of high quality was stored at −20°C. dsTRE2-like, dsTRE2, a mixture of dsTRE2-like and dsTRE2, and the control dsGFP were injected into the abdomen of the third and fourth instar of H. axyridis using the IM-31 microinjector (Narishige, Japan), and the injection amount of dsRNA was approximately 300 ng per individual. The larvae were sampled at 48 h after injection or during ecdysis.
Detection of the Relative Expression Level of Key Genes Involved in Carbohydrate Metabolism by qRT-PCR
The total RNA from treated H. axyridis larvae was extracted as a template, and specific primers (Table 2) were used for quantitative RT-qPCR. The relative mRNA expression level of TRE2-like or TRE2 was evaluated by qRT-PCR using the SYBR Green master mix (SYBR Green Premix Ex Taq, Takara, Japan) with the Bio-Rad CFX96TM Real-Time PCR Detection System (Bio-Rad Laboratories Inc.). Three biological replicates and three technical replicates were set for each treatment group. The total reaction mixture of volume 20 μL comprised of 10 μL of SYBR Green, 1 μL each of forward and reverse primers (10 μmol/L), 2 μL of cDNA template, and 6 μL of ddH2O. The PCR amplification conditions were as follows: pre-denaturation at 95°C for 30 s, denaturation at 95°C for 5 s, and extension at 60°C for 20 s, 40 cycles. The dissolution curve was drawn after each reaction to ensure no non-specific amplification.
Determination of Key Enzyme Activity and Carbohydrate Content During Ecdysis After RNAi in H. axyridis
The activities of soluble trehalase, membrane-bound trehalase and the content of sugars were analyzed in H. axyridis larvae sampled at 48 h or during ecdysis after injection. Three individuals were selected from each injection group for this part of the experiment. The TRE activity assay was performed according to a previously published method with some modifications (Tatun et al., 2008). Briefly, insect samples were ground with phosphate buffered saline (PBS), sonicated, and centrifuged at 4°C for 20 min at 10 000 g. Subsequently, 350 μL of the supernatant was collected and ultracentrifuged at 20, 800 g for 60 min at 4°C. The remaining supernatant was used to determine the protein, trehalose, and glycogen contents. The supernatant obtained from ultracentrifugation was used to determine the TRE1 activity. The pellet was resuspended in PBS (pH 7.0) and used to evaluate the TRE2 activity.
The contents of protein and glucose in the supernatant and pellet were determined. Briefly, 75 μL of 40 mM trehalose (Sigma-Aldrich, Saint Louis, MO, United States) and 165 μL of PBS (pH 7.0) were added to the supernatant (60 μL) to determine the TRE activity. The mixture was incubated at 37°C for 60 min and centrifuged at 12,000 g for 10 min at 4°C. The supernatant (50 μL) was used to measure the TRE activity using the glucose (Go) assay kit (Sigma-Aldrich). The protein content was determined using the BCA Protein Assay Kit (Beyotime, China).
The trehalose content was measured using the anthrone method following a previously published method (Leyva et al., 2008). Briefly, 30 μL of 1% sulfuric acid was added to 30 μL of the sample and incubated at 90°C for 10 min and placed in an ice bath for 3 min. After adding 30 μL of 30% KOH, the sample was incubated again at 90°C in a water bath for 10 min and in an ice bath for 3 min. Then, 600 μL of developer (0.02 g fluorenone + 100 mL of 80% H2SO4) was added, and the sample was placed in a water bath at 90°C for 10 min and cooled in an ice bath. The absorbance of the sample was measured at 630 nm using a microplate reader.
For glycogen content determination, 160 μL of supernatant obtained after centrifugation at 1 000 g was added to 600 μL of anthrone sulfate reagent, and the mixture was incubated at 90°C for 10 min, and then cooled in an ice bath. The absorbance of the sample was measured at 625 nm using a microplate reader. A glucose assay kit (SIGMA) was used to measure the glucose content. Briefly, 150 μL of supernatant obtained after centrifugation at 20, 800 g and the suspension of precipitation obtained after centrifugation at 20, 800 g were added to an Eppendorf tube, and then 300 μL of glucose analysis reagent was added to the sample, which is the same as the glucose standard sample. After incubation in water bath at 37°C for 30 min, 300 μL of 2 N H2SO4 was added to stop the reaction, and the absorbance of the samples was measured at 540 nm using a microplate reader.
Statistical Analyses
In the early stage, we tested the specificity and validity of the primers used in this experiment. The results showed that the dissolution curves were all single peaks, and the peak position was the set annealing temperature, indicating that the specificity of the amplification products was good and the experimental results were effective. In addition, GFP was used as a control for each plate in this experiment, and three biological replicates and three technical replicates were set for each treatment group. The qRT-PCR data were processed using the 2–ΔΔCT method (Livak and Schmittgen, 2001). The mRNA expression level in the dsGFP-injected groups was designated as controls. Statistical analysis was performed with STATISTICA 8.0 and Sigma Plot 10.0, all of the data obtained in this study are presented as means ± standard errors (SEs) of were analyzed using one-way analysis of variance (ANOVA) and Tukey’s test. A P-value of 0.05 or 0.01 was considered significant or highly significant, respectively. An asterisk indicates a statistically significant difference in mRNA levels between the dsGFP injection group and each of the dsTRE injection groups measured at the same time (P < 0.05, T-test), and a double asterisk indicates a highly significant difference (P < 0.01,T-test).
Results
Analyses of Phenotypes, Aberrations and Mortality Rates After TRE2-Like and TRE2 Gene RNAi
With the successful silencing of the TRE2-like, TRE2, and TRE2s genes, the adult H. axyridis exhibited wing abnormality (Figure 1A). About 14.82% of dsTRE2-like, 19.94% of dsTRE2 and 23.88% of dsTRE2s wing deformities were found in the adult H. axyridis due to RNAi knockdown (Figure 1B). After TRE2-like, TRE2, and TRE2s were silenced by RNAi, the mortality rate of axyridis larvae was 17.14, 10.62, and 12.95%, respectively (Figure 1C). The aberration and mortality rates of H. axyridis injected with control dsRNA against GFP were only 0 and 1.78%, respectively (Figures 1B,C). Furthermore, the pupa weights with injection of dsTRE2-like, dsTRE2, and dsTRE2s at the beginning of third instar were significantly decreased (P < 0.05) (Figure 1D). After the injection of dsTRE2-like and dsTRE2 to the fourth instar larvae, the pupal weights were decreased, but not significant. The weight loss of pupae after the injection of dsTRE2s was significant (P < 0.05) (Figure 1E).
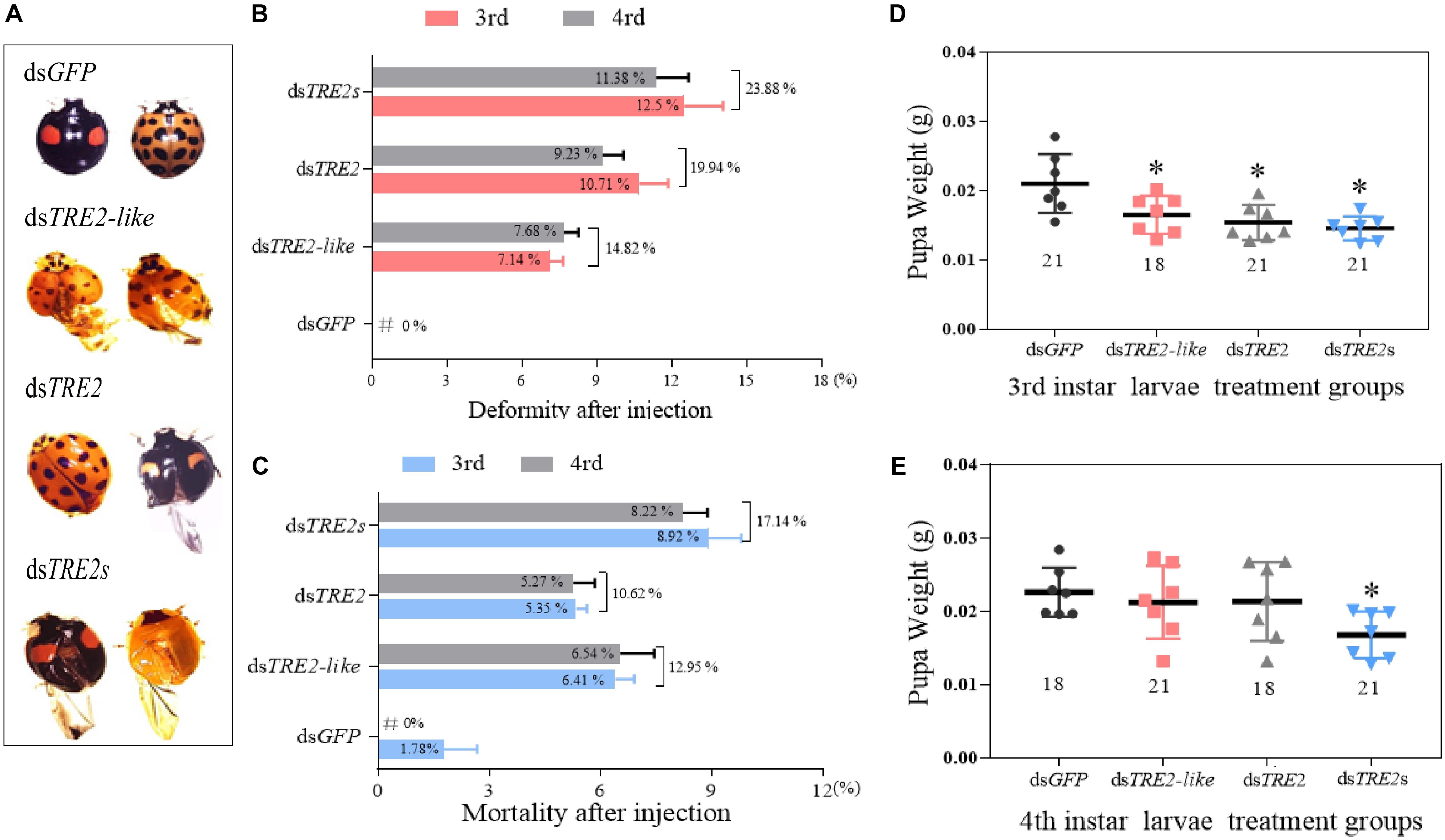
Figure 1. Changes in the wing phenotype, mortality, and body weight after RNAi. (A) Larval phenotypes after dsTRE2-like, dsTRE2, and dsTRE2s injection. They were taken under a Leica microscope (16X). (B) Phenotypic deformity rates after TRE2-like, dsTRE2, dsTRE2s, and dsGFP injection. (C) Mortality rates after TRE2-like, dsTRE2, dsTRE2s, and dsGFP injection. (D) The average pupal weight after the injection of dsTRE2-like, dsTRE2, and dsTRE2s. The third instar larvae were chosen as the targets for dsRNA injection. (E) The average pupal weight after the injection of dsTRE2-like, dsTRE2, and dsTRE2s. The third instar larvae were chosen as the targets for dsRNA injection. ∗P < 0.05 and ∗∗P < 0.01. Green fluorescence protein (GFP) was used as the control (Eighteen or twenty-one individuals were selected from each injection group for phenotypic observation and pupal weight measurement, which was repeated three times).
TRE2-Like and TRE2 mRNA Expression After RNAi
The mRNA levels of the relevant genes after RNAi was determined by qRT-PCR. All transcripts of the TRE2-like and TRE2 genes were significantly decreased (P < 0.01) compared with the dsGFP injected treatment after 48 h or ecdysis. The altered trend after the injection of dsTRE2s was similar to that of single gene silencing (Figures 2A,B).
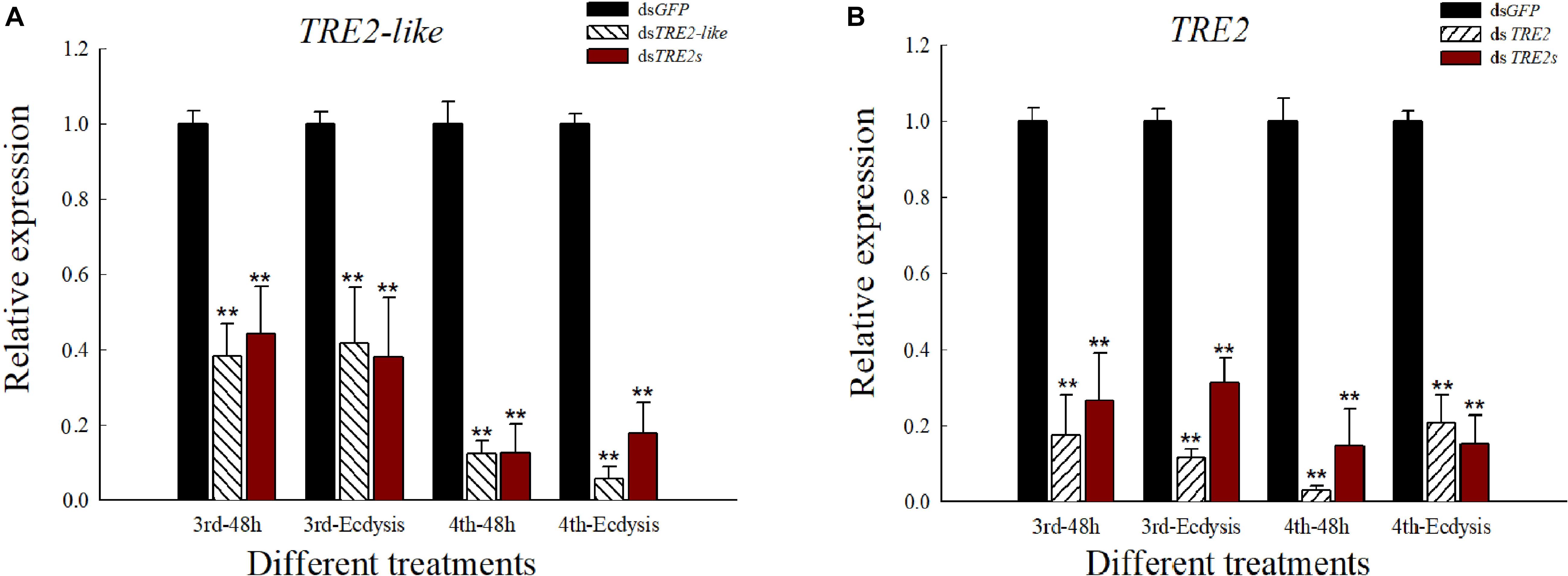
Figure 2. Expression level of TRE2-like and TRE2 in H. axyridis after RNAi. (A) The expression level of TRE2-like gene after the injection of dsTRE2-like and dsTRE2s. (B) The expression level of TRE2 after the injection of dsTRE2 and dsTRE2s. The third and fourth instar larvae were chosen as the targets for dsRNA injection, ∗P < 0.05 and ∗∗P < 0.01.
The Activity of Soluble Trehalase and Membrane-Bound Trehalase Changed After TRE2-Like and TRE2 Genes RNAi
Compared with the control group injected with dsGFP, the soluble trehalase activity mostly exhibited significant (P < 0.05) or highly significant (P < 0.01) increase after interference with the TRE2-like gene. At the same time, TRE2s RNAi also showed a very significant increase in the molting stage (P < 0.01). After interference with the TRE2 gene, there was a significant (P < 0.05) increase in the molting process of the third instar larvae. Others showed significant (P < 0.05) or highly significant (P < 0.01) reduction (Figure 3A). In the results of membrane-bound trehalase activity, we found that the activity at the molting stage was lower, and the dsTRE2-like developmental stage showed a decrease or a significant decrease. After 48 h of injection of dsTRE2 or dsTRE2s, the fourth instar larvae were significantly (P < 0.05) or highly significant (P < 0.01) reduced (Figure 3B).
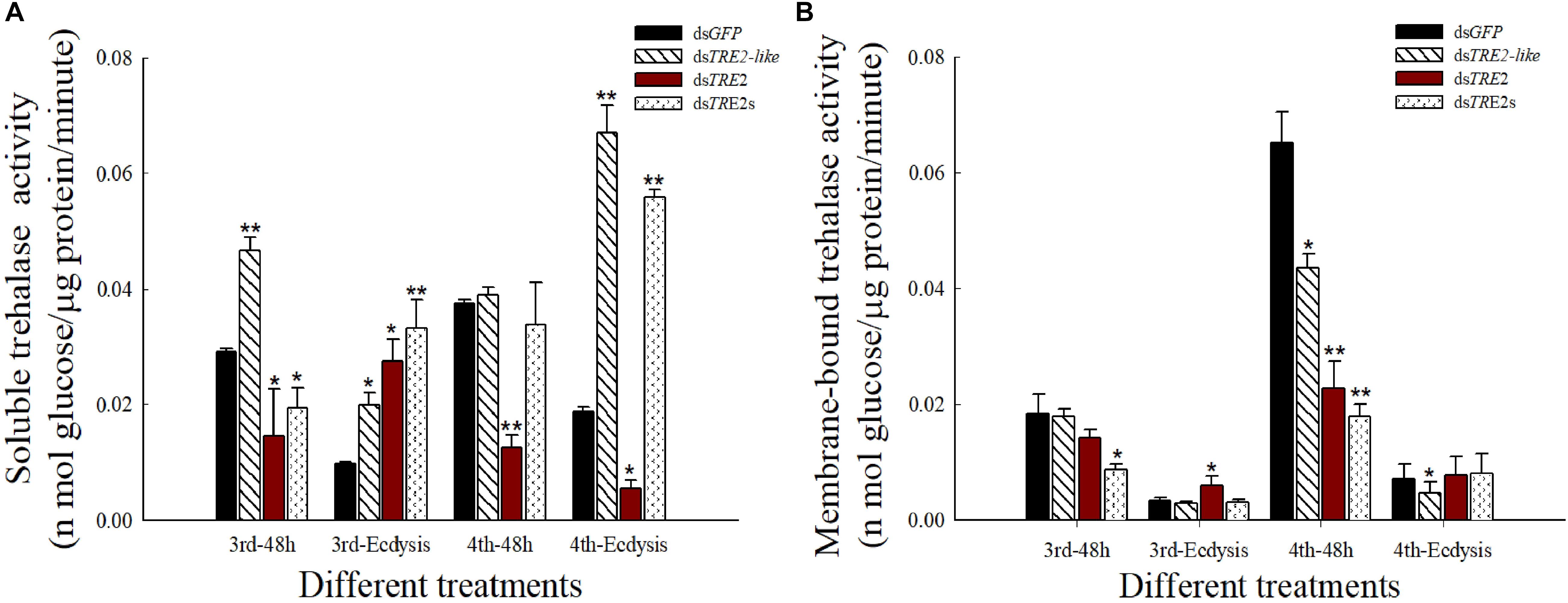
Figure 3. Effects of RNAi on the activity of soluble trehalase and membrane-bound trehalase in Harmonia axyridis. (A) The soluble trehalase activity in H. axyridis after the injection of dsTRE2-like, dsTRE2, dsTRE2s, and dsGFP. (B) The membrane-binding trehalase activity in H. axyridis after the injection of dsTRE2-like, dsTRE2, dsTRE2s, and dsGFP. The third and fourth instar larvae were chosen as the targets for dsRNA injection. ∗P < 0.05 and ∗∗P < 0.01.
Changes of Glycogen, Glucose, and Trehalose Content After RNAi
Compared with the control group injected with dsGFP, most of the glycogen content that interfered with TRE2-like showed a significant (P < 0.05) or highly significant (P < 0.01) increase (Figure 4A). After TRE2-like interference, most of the glucose content decreased or decreased significantly (P < 0.05). After interference with TRE2s, the third instar larvae and the fourth instar larvae also showed a significant decrease in interference for 48 h. After interference with TRE2, most of the glucose content increased significantly (P < 0.05) (Figure 4B). The interference of TRE2-like in the molting stage led to significant decrease in the trehalose content (P < 0.01). The trehalose content increased significantly (P < 0.01) during the 48th hour of TRE2 and the molting of the fourth instar larvae. The change in trehalose content after injection of dsTRE2s was consistent with the interference with the TRE2 gene, showing a significant increase (P < 0.05) (Figure 4C).
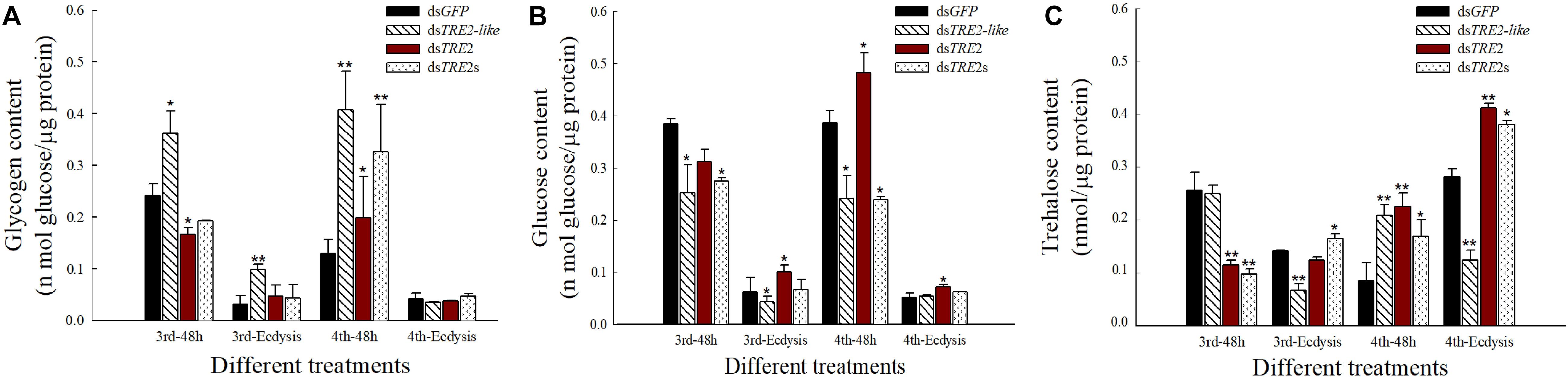
Figure 4. Content of glycogen, glucose, and trehalose in Harmonia axyridis after RNAi. (A) The glycogen content in H. axyridis after the injection of dsTRE2-like, dsTRE2, dsTRE2s, and dsGFP. (B) The glucose content in H. axyridis after the injection of dsTRE2-like, dsTRE2, dsTRE2s, and dsGFP. (C) The trehalose content in H. axyridis after the injection of dsTRE2-like, dsTRE2, dsTRE2s, and dsGFP. The third and fourth instar larvae were chosen as the targets for dsRNA injection. ∗P < 0.05 and ∗∗P < 0.01.
Effects of RNAi on the Expression of TPS, GS, and GP Genes
Compared with the control group injected with dsGFP, the expression of trehalose-6-phosphate synthase (TPS) gene in H. axyridis showed a decrease after the injection of dsTRE2-like, dsTRE2 or dsTRE2s. Particularly, the TPS gene expression in the third and fourth instar larvae showed a significant (P < 0.05) or highly significant (P < 0.01) decrease after RNAi (Figure 5A). With the injection of dsTRE2-like, dsTRE2 or dsTRE2s, the expression of glycogen synthase (GS) gene in H. axyridis was decreased. Furthermore, after interference in the ecdysis of fourth instar larvae, all the injection treatment groups showed a significant (P < 0.05) or highly significant (P < 0.01) decrease (Figure 5B). After the injection of dsTRE2-like, dsTRE2 or dsTRE2s, a significant (P < 0.05) or highly significant (P < 0.01) decrease of the expression of glycogen phosphorylase (GP) gene in all stages was recorded (Figure 5C).
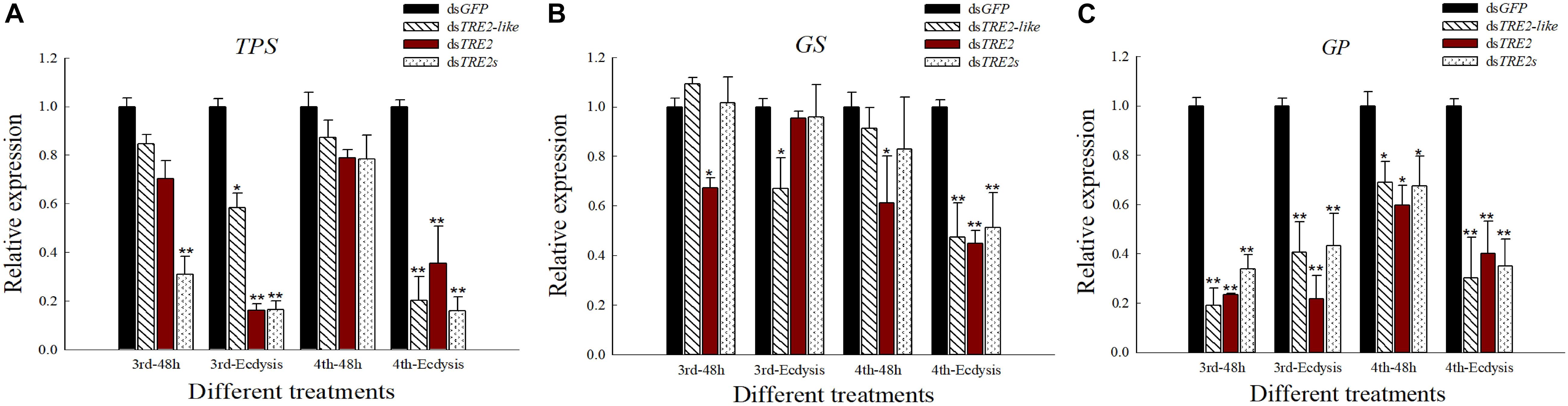
Figure 5. Effects of RNAi on the expression of TPS, GS, and GP genes in Harmonia axyridis. (A) The expression level of trehalose-6-phosphate synthase genes (TPS) gene in H. axyridis after RNAi. (B) The expression level of glycogen synthase (GS) gene in H. axyridis after RNAi. (C) The expression level of glycogen phosphorylase (GP) gene in H. axyridis after RNAi. The third and fourth instar larvae were chosen as the targets for dsRNA injection. ∗P < 0.05 and ∗∗P < 0.01.
The Expression of CHSA and CHSB Genes When TRE2-Like and TRE2 Silenced
Compared with the control group injected with dsGFP, the group injecting with dsTRE2-like, dsTRE2 or dsTRE2s showed a significant (P < 0.05) or highly significant (P < 0.01) decrease in the expression of chitin synthase gene A (CHSA) gene in H. axyridis (Figure 6A). Meanwhile, the trend of the expression of chitin synthase gene B (CHSB) gene was similar to the CHSA, except that there was no significant changes appeared in the fourth instar larvae with the injection of dsTRE2-like, dsTRE2 or dsTRE2s (Figure 6B).
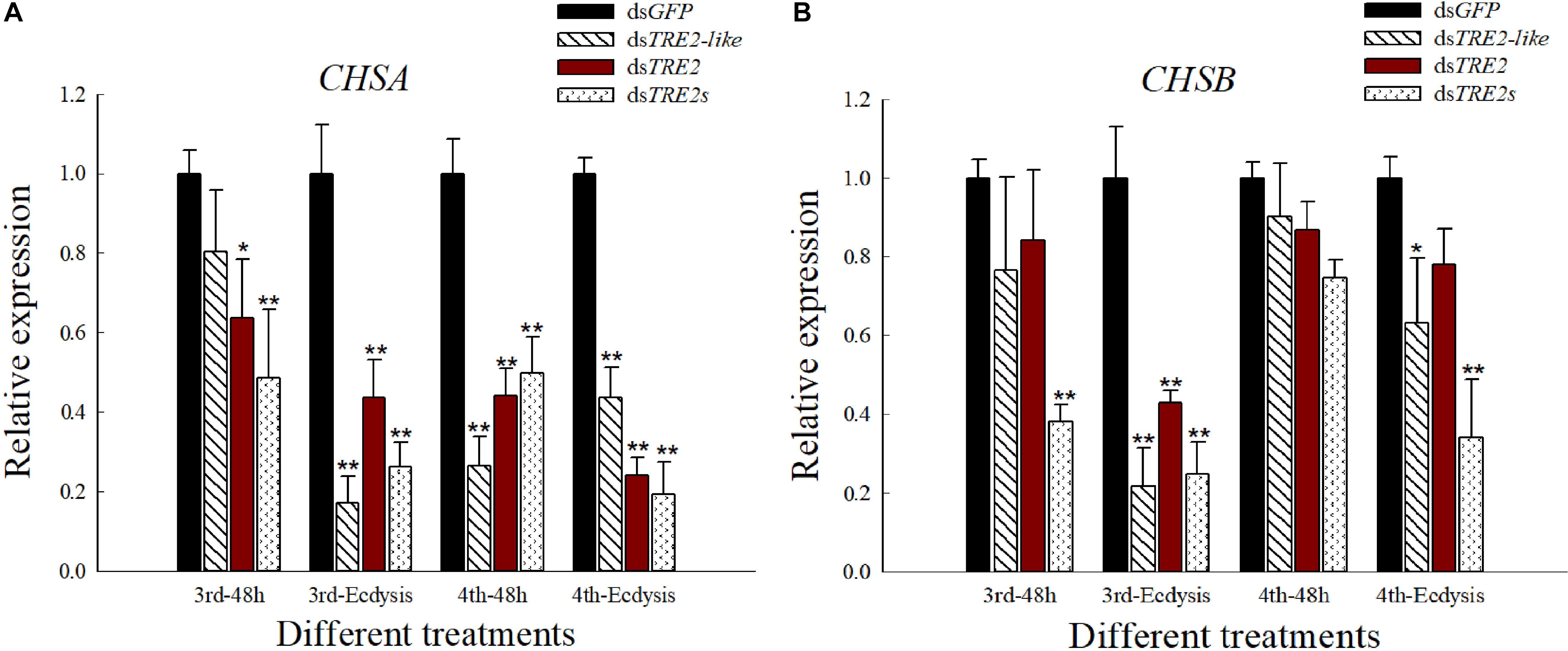
Figure 6. Effects of RNAi on the expression of CHSA and CHSB genes in Harmonia axyridis. (A) The expression level of chitin synthase gene A (CHSA) gene in H. axyridis after RNAi. (B) The expression level of chitin synthase gene B (CHSB) gene in H. axyridis after RNAi. The third and fourth instar larvae were chosen as the targets for dsRNA injection. ∗P < 0.05 and ∗∗P < 0.01.
The Effect of TRE2-Like and TRE2 RNAi on TRE1 Expressed Genes
Compared with the control group injected with dsGFP, the expression of TRE1-1 and TRE1-2 genes in both the dsTRE2-like, dsTRE2 and dsTRE2s injected groups showed significant (P < 0.05) or highly significant (P < 0.01) decrease (Figures 7A,B). After the injection of dsTRE2, the expression of TRE1-3 gene in H. axyridis was decreased or significantly (P < 0.05) decreased (Figure 7C). The expression of the TRE1-4 gene was significantly (P < 0.01) decreased at 48 h after the injection of dsTRE2 in the third instar larvae and during ecdysis in the fourth instar larvae (Figure 7D). As for the expression of the TRE5 gene, there was significant (P < 0.05) decrease with the injection of dsTRE2 (Figure 7E).
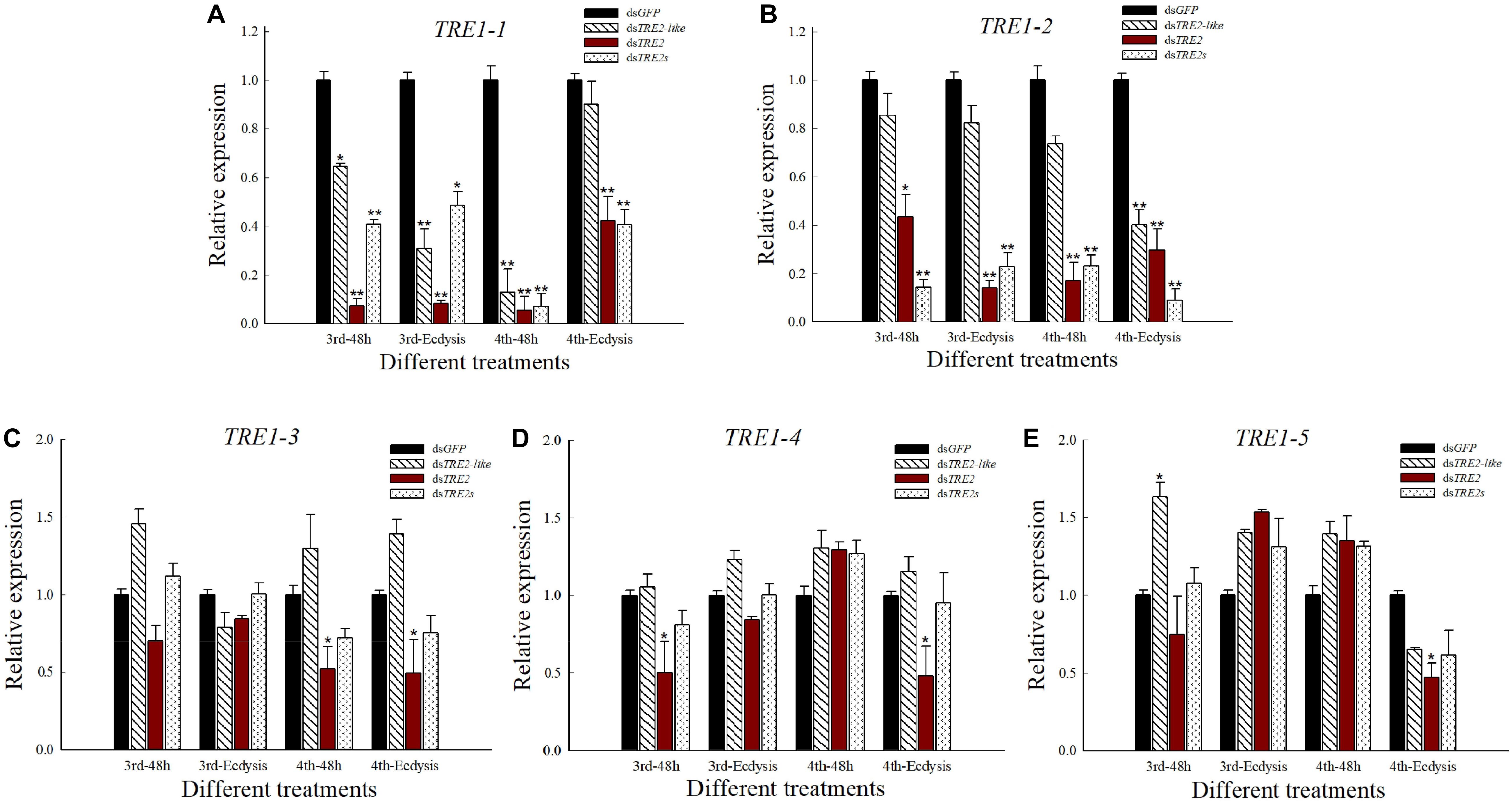
Figure 7. Expression level of TRE1 in Harmonia axyridis after RNAi. (A) The expression level of TRE1-1 gene in H. axyridis after RNAi. (B) The expression level of TRE1-2 in H. axyridis after RNAi. (C) The expression level of TRE1-3 in H. axyridis after RNAi. (D) The expression level of TRE1-4 in H. axyridis after RNAi. (E) The expression level of TRE1-5 in H. axyridis after RNAi. The third and fourth instar larvae were chosen as the targets for dsRNA injection. ∗P < 0.05 and ∗∗P < 0.01.
Discussion
At present, RNAi technology has been relatively mature used in a variety of insects, such as Bombyx mori (Ohnishi et al., 2006), and T. castaneum (Arakane et al., 2005). So, in this study, dsRNA of TRE2-like and TRE2 was artificially synthesized in vitro and introduced into the H. axyridis by microinjection, to study the expression and function of TRE2-like and TRE2 genes.
We have previously studied the TRE2-like and TRE2 gene silencing in the pupal stage of the H. axyridis and found that the effects of interference were significant, and there were wing deformities after the RNAi (Zhang et al., 2019a). Similar findings were observed in the present study, wherein we found that silencing TRE2-like and TRE2 genes seriously affected the whole nymph development process of H. axyridis leading to the inhibition of the growth of nymph or pupa, especially in the molting stage and further phenotypic abnormality and decreased survival rates (Figure 1). The results were similar to those obtained by inhibiting TRE gene expression in other insects, for example, phenotypic malformation occurs after interference with the TRE2 gene in S. exigua (Chen et al., 2010) or Brown planthopper (Zhao et al., 2016). It has been confirmed that the deformed phenotype was caused by a decrease in the transcription level of trehalase (Tang et al., 2016; Zhang et al., 2017b). Therefore, we tested the expression levels of TRE2-like and TRE2 genes after RNAi, the results show that the relative expression levels of TRE2-like and TRE2 genes were significantly decreased (Figure 2). The result is consistent with the above conclusion. Moreover, in the present study, we found that the pupal weight reduced by RNAi and found that silencing of TRE2-like or TRE2 genes have different effects on insect viability. In fact, the abnormal wings have been found owing to the significant decrease in the expression levels of chitin synthase and wing developmental network genes when TRE gene or TRE enzyme activity have been inhibited (Tang et al., 2017; Zhang et al., 2017a).
Trehalase is able to decompose the important energy storage material and the stress metabolite- trehalose in insects. The changes in gene expression and enzyme activity affect the life processes of insect molting, metamorphosis and reproduction (Tang et al., 2012). Studies have reported that sustained expression of TRE2 gene and activity of TRE2 are necessary for the metabolism of hemolymph trehalose to meet the energy requirements of the midgut cells (Tatun et al., 2008). The results of this study showed that the interference of TRE2-like and TRE2 genes decreased the activity of TRE2 in H. axyridis. It is speculated that after inhibiting the expression of the TRE2-like gene, the activity of the trehalase activity will be reduced (Figure 3), which has already been confirmed in silkworm larvae (Mitsumasu et al., 2005). In addition, based on the results of this experiment, we speculate that TRE2 gene may mainly regulate soluble trehalase activity, while TRE2-like gene tends to affect membrane-bound trehalase activity. But this view needs further confirmation and validation through other experiments. Trehalose and trehalase activities play a key role in regulating multiple physiological processes of insects (Ge et al., 2011). The decrease in trehalase gene expression and enzyme activity affects the rate of trehalose synthesis, resulting in insufficient energy, leading to wing deformity and stunting. Previous studies have shown that membrane-bound trehalase with higher activity is mainly present in the muscles of insects, hydrolyzing trehalose in food and providing energy for the movement and development of insects (Becker et al., 1996).
Trehalose has been well demonstrated in insect physiology as an energy source for insects, maintaining the glucose level (Santos et al., 2012). Studies have shown that the TRE gene affects the content of three sugars by regulating gene expression and enzyme activity (Santos et al., 2012; Shi et al., 2016). In the present study, after the successful interference of TRE2-like gene the activity of membrane-bound trehalose and the trehalose content were reduced and the glucose levels also declined (Figures 4B,C). Related reports have shown that when trehalose hydrolysis into glucose is inhibited, it affects the energy level and blood glucose content in various cells of insects, and thus affecting other physiological pathways (Chen et al., 2010). Meanwhile, we found that after the interference of TRE2-like gene, the glycogen content was increased (Figure 4A), it has been indicated that trehalose and glycogen are important energy storage substances in insects as well as play an important role in energy metabolism of insects (Tan et al., 2014). The increase in the glycogen content indicates that trehalose or glucose is decomposed into glycogen to ensure normal physiological activities.
Glycogen metabolism requires two enzymes, named GS gene for the synthesis and GP gene for the decomposition, and both these enzymes are controlled by hormones and play a key role in glycogen metabolic balance (Łopieńska-Biernat et al., 2019). In this study, we investigated the glycogen metabolism, after the injection of dsTRE2-like, the expression of GP gene showed decrease at the mRNA level and the GS gene expression decreased in the ecdysis stage (Figures 5B,C). Glycogen is converted into trehalose to increase the blood sugar concentration of insects and adapt to the adverse environment. GP gene can decompose glycogen into glucose-1-phosphate, which can then interact with uridine diphosphate (UDPG) and be converted into trehalose (Tang et al., 2018). The null mutants of GS and GP genes displayed growth defects and larval lethality indicating that glycogen plays a crucial role in larval development (Yamada et al., 2019).
In insects, trehalose is first synthesized by TPS, which is degraded to glucose by trehalase when demanding for energy supply (Tang et al., 2018). In the present study, we found that silencing TRE2-like and TRE2 genes reduced the relative expression of TPS genes, and caused the phenomenon of wing deformity (Figure 5A). Studies have shown that silencing TPS inhibits the expression of chitin biosynthetic pathways and lipid catabolism-related genes, thereby affecting the development of insect (Chen et al., 2018). Previous studies have shown that increased expression of TPS gene can increase anti-stress ability and protect cell structure. In addition, when the expression of TPS gene was severely inhibited, abnormal phenotypes exhibited, especially molting and wing deformities, as well as the increased mortality (Tang et al., 2016).
In addition to providing energy for the growth and development of insect, as the first key enzyme in the chitin synthesis pathway, trehalase is also closely related to the formation of insect chitin (Chen et al., 2010). In insects, trehalose synthesized by TPS is the main substrate for chitin biosynthesis (Shukla et al., 2015), and TPS gene can effectively regulate the biosynthesis or degradation of chitin by TRE gene. This hypothesis has been confirmed by knocking down the TPS genes in N. lugens, in which some genes involved in the chitin biosynthetic pathway and almost all CHS genes have decreased expression (Yang et al., 2017). In this study, silencing TRE2-like and TRE2 not only reduced the expression level of the target gene, but also reduced the relative expression levels of TPS, CHSA, and CHSB genes (Figure 6). Studies have shown that the synthesis of new epidermal chitin in migratory locust requires a large amount of trehalase, and the silencing of the gene mainly affects the synthesis of chitin in insect epidermis (Liu et al., 2016). Furthermore, research shows that insects renew the stratum corneum of the exoskeleton by degrading the chitin and cuticle proteins and synthesizing a new cuticle during ecdysis, which is a necessary process for the normal growth and development of insects (Merzendorfer and Zimoch, 2003; Deng et al., 2016; Ye et al., 2019). The function of the trehalase-encoding gene in the synthesis of chitin in Brown planthopper using RNAi technology showed that after inhibiting the expression of the trehalase-encoding gene, chitin synthase-encoding gene and other genes were also down-regulated correspondingly. The inhibition of chitin deacetylase expression can affect the ecdysis of larval-to-pupa and pupa-to-adult in Leptinotarsa decemlineata (Wu et al., 2019). These results indicate that once the supply balance of trehalose metabolism in insects is broken, it will directly affect the chitin synthesis of insects.
It is well known that the H. axyridis has five soluble trehalases and two membrane-bound trehalase genes. So, to further understand the function of the membrane-bound gene, we examined the relative expression levels of the five TRE1 genes after RNAi TRE2-like or TRE2. From the results, the effect of interference TRE2-like or TRE2 on TRE1-1 gene expression level was significant, indicating that TRE2-like, TRE2, and TRE1-1 may have similar functions. In addition, the interference of TRE2 gene significantly affected the expression of TRE1-1 and TRE1-2 genes levels, but had no significant effect on TRE1-3, TRE1-4, and TRE1-5, indicating TRE2 and TRE1-1 and TRE1-2 has a higher functional similarity and a greater influence on each other (Figure 7). The results are consistent with the relative expression changes of three trehalase genes in brown planthopper (Zhao et al., 2016). How to play the biological control role of H. axyridis depends on how to produce and put into use on a large scale. However, there is still no way to coordinate the production input of the H. axyridis with the pest outbreak time. Therefore, through the study of TRE molecular mechanism of H. axyridis, this experiment laid a foundation for the production of natural predators of H. axyridis and realized its biological control effect better.
Conclusion
In conclusion, the results revealed that RNAi can effectively reduce the expression of the target genes. The injection of dsTRE2-like and dsTRE2 into H. axyridis can disrupt the metabolism of trehalose in the body and affect the supply of glycogen and glucose, causing difficulty in the synthesis of chitin, consequently leading to wing deformity. The results lay a foundation for exploring the potential functions and regulatory mechanisms of insect TRE2. Therefore, it is particularly important to further study the molecular mechanism of trehalose in other insects.
Data Availability Statement
Publicly available datasets were analyzed in this study. These datasets can be found here: TRE2-like: KX349224.1 and TRE2: KX349225.1.
Author Contributions
BT and SW conceived and designed the manuscript structure. YL, XC, S-SW, B-YP, and S-GW performed the current articles collection and trehalose metabolism genes’ analysis. YL and S-SW wrote the manuscript.
Funding
This work was supported by the National Key Research and Development Program of China (Grant No. 2017YFD0201000), the Beijing Key Laboratory of Environment Friendly Management on Fruit Diseases and Pests in North China (Grant No. BZ0432), the Beijing Institute of Technology Programs (Grant No. D171100001617003), Technical Innovation Program of Beijing Academy of Agriculture and Forestry Sciences (Grant No. 20170107), Youth Scientific Research Fund of Beijing Academy of Agriculture and Forestry Sciences (Grant No. QNJJ201725), and the Hangzhou Science and Technology Development Program of China (Grant No. 20190101A01).
Conflict of Interest
The authors declare that the research was conducted in the absence of any commercial or financial relationships that could be construed as a potential conflict of interest.
The reviewer SN declared a past collaboration with one of the authors BT.
Supplementary Material
The Supplementary Material for this article can be found online at: https://www.frontiersin.org/articles/10.3389/fphys.2019.01371/full#supplementary-material
IMAGE S1 | Nucleotide and deduced amino acid sequences of Harmonia axyridis membrane-bound-like trehalase (TRE2-like). Initiation and termination codons are indicated by bold typeface and italic. The conserved domains used for dsRNA synthesis of TRE2-like (751–1362 bp) is shaded in gray. qRT–PCR primers sequences used to detect TRE2-lik is indicated by bold typeface and red-colored shading.
IMAGE S2 | Nucleotide and deduced amino acid sequences of Harmonia axyridis membrane-bound trehalase (TRE2). Initiation and termination codons are indicated by bold typeface and italic. The conserved domains used for dsRNA synthesis of TRE2 (1897–2308 bp) is shaded in gray. qRT–PCR primers sequences used to detect TRE2-lik is indicated by bold typeface and red-colored shading.
References
Adachi-Hagimori, T., Shibao, M., Seko, T., and Miura, K. (2019). Release of the flightless strain of Harmonia axyridis (Coleoptera: Coccinellidae) against two aphid species on Komatsuna (Brassica rapa var. perviridis) in open fields. J. Econ. Entomol. 112, 115–118. doi: 10.1093/jee/toy350
Arakane, Y., Muthukrishnan, S., Kramer, K. J., Specht, C. A., Tomoyasu, Y., Lorenzen, M. D., et al. (2005). The Tribolium chitin synthase genes TcCHS1 and TcCHS2 are specialized for synthesis of epidermal cuticle and midgut peritrophic matrix. Insect Mol. Biol. 4, 453–463. doi: 10.1111/j.1365-2583.2005.00576.x
Awad, M., Piálek, L., Krejčí, A., Laugier, G., and Nedvìd, O. (2017). Paternity following multiple mating in ladybird Harmonia axyridis. BioControl 62, 297–307. doi: 10.1007/s10526-017-9806-z
Bansal, R., Mian, M. A., Mittapalli, O., and Michel, A. P. (2013). Molecular characterization and expression analysis of soluble trehalase gene in Aphis glycines, a migratory pest of soybean. Bull. Entomol. Res. 103, 286–295. doi: 10.1017/S0007485312000697
Becker, A., Schlöder, P., Steele, J. E., and Wegener, G. (1996). The regulation of trehalose metabolism in insects. Experientia 52, 433–439. doi: 10.1007/bf01919312
Chen, J., Tang, B., Chen, H. X., Yao, Q., and Huang, X. F. (2010). Different functions of the insect soluble and membrane-bound trehalase genes in chitin biosynthesis revealed by RNA interference. PLoS One 5:e10133. doi: 10.1371/journal.pone.0010133
Chen, Q. W., Jin, S., Zhang, L., Shen, Q. D., Wei, P., Wei, Z. M., et al. (2018). Regulatory functions of trehalose-6-phosphate synthase in the chitin biosynthesis pathway in Tribolium castaneum (Coleoptera: Tenebrionidae) revealed by RNA interference. Bull. Entomol. Res. 108, 388–399. doi: 10.1017/S000748531700089X
de Almeida, F. M., Bonini, B. M., Beton, D., Jorge, J. A., Terenzi, H. F., and da Silva, A. M. (2009). Heterologous expression in Escherichia coli of Neurospora crassa neutral trehalase as an active enzyme. Protein Expr. Purif. 65, 185–189. doi: 10.1016/j.pep
Deng, H. M., Li, Y., Zhang, J. L., Liu, L., and Feng, Q. L. (2016). Analysis of expression and chitin-binding activity of the wing disc cuticle protein BmWCP4 in the silkworm, Bombyx mori. Insect Sci. 23, 782–790. doi: 10.1111/1744-7917.12231
Du, W., Zhao, Z., and Zheng, W. (2019). The recognition of development-related genes in the testis and MAGs of time-series Harmonia axyridis adults using a time-series analysis by RNA-seq. Gene 693, 52–60. doi: 10.1016/j.gene
Durieux, D., Fassotte, B., Deneubourg, J. L., Brostaux, Y., Vandereycken, A., Joie, E., et al. (2015). Aggregation behavior of Harmonia axyridis under non-wintering conditions. Insect Sci. 22, 670–678. doi: 10.1111/1744-7917.12144
Elbein, A. D., Pan, Y. T., Pastuszak, I., and Carroll, D. (2003). New insights on trehalose: a multifunctional molecule. Glycobiology 13, 17–27. doi: 10.1093/glycob/cwg047
Forcella, M., Cardona, F., Goti, A., Parmeggiani, C., Cipolla, L., Gregori, M., et al. (2010). A membrane-bound trehalase from Chironumus riparius larvae: purification and sensitivity to inhibition. Gycobiology 20, 1186–1195. doi: 10.1093/glycob/cwq087
Frison, M., Parrou, J. L., Guillaumot, D., Masquelier, D., François, J., Chaumont, F., et al. (2007). The Arabidopsis thaliana trehalase is a plasma membrane-bound enzyme with extracellular activity. FEBS Lett. 581, 4010–4016. doi: 10.1016/j.febslet
Ge, L. Q., Zhao, K. F., Huang, L. J., and Wu, J. C. (2011). The effects of triazophos on the trehalose content, trehalase activity and their gene expression in the brown planthopper Nilaparvata lugens (Stål) (Hemiptera: Delphacidae). Pestic. Biochem. Physiol. 100, 172–181. doi: 10.1016/j.pestbp
Kojić, D., Popović, ŽD., Orčić, D., Purać, J., Orčić, S., Vukašinović, E. L., et al. (2018). The influence of low temperature and diapause phase on sugar and polyol content in the European corn borer Ostrinia nubilalis (Hbn.). J. Insect Physiol. 109, 107–113.
Lee, J. H., Mori, H., Nishimoto, M., Okuyama, M., Kim, D., Wongchawalit, J., et al. (2007). Molecular cloning of cDNA for trehalase from the European honeybee, Apis mellifera land its heterologous expression in Pichia pastoris. Biosci. Biotechnol. Biochem. 71, 2256–2265. doi: 10.1271/bbb.70239
Leyva, A., Quintana, A., Sánchez, M., Rodríguez, E. N., Cremata, J., and Sánchez, J. C. (2008). Rapid and sensitive anthrone-sulfuric acid assay in microplate format to quantify carbohydrate in biopharmaceutical products: method development and validation. Biologicals 36, 134–141. doi: 10.1016/j.biologicals
Liu, X. J., Sun, Y. W., Cui, M., Ma, E. B., and Zhang, J. Z. (2016). Molecular characteristics and functional analysis of trehalase genes in Locusta migratoria. Sci. Agric. Sin. 49, 4375–4386. doi: 10.3864/j.issn.0578-1752.2016.22.010
Livak, K. J., and Schmittgen, T. D. (2001). Analysis of relative gene expression data using real-time quantitative PCR and the 2-ΔΔCT method. Methods 25, 402–408. doi: 10.1006/meth
Łopieńska-Biernat, E., Paukszto, ł, Jastrzêbski, J. P., Makowczenko, K., and Stryiński, R. (2019). Genes expression and in silico studies of functions of trehalases, specific gene family. Int. J. Biol. Macromol. 129, 957–964.
Ma, L., Dai, W., Li, X., Zhang, Y., and Zhang, C. (2015). Molecular cloning and expression analysis of soluble and membrane-bound trehalase genes in the cotton bollworm. Helicoverpa armigera. J. Asia Pac. Entomol. 18, 187–195. doi: 10.1016/j.aspen
Merzendorfer, H., and Zimoch, L. (2003). Chitin metabolism in insects: structure, function and regulation of chitin synthases and chitinases. J. Exp. Biol. 206, 4393–4412. doi: 10.1242/jeb.00709
Mitsumasu, K., Azuma, M., Niimi, T., Yamashita, O., and Yaginuma, T. (2005). Membrane-penetrating trehalase from silkworm Bombyx mori. molecular cloning and localization in larval midgut. Insect Mol. Biol. 14, 501–508. doi: 10.1111/j.1365-2583.2005.00581.x
Nakayama, S., Seko, T., Takatsuki, J. I., Miura, K., and Miyatake, T. (2010). Walking activity of flightless Harmonia axyridis (Coleoptera: Coccinellidae) as a biological control agent. J. Econ. Entomol. 103, 1564–1568. doi: 10.1603/EC09420
Nardelli, A., Vecchi, M., Mandrioli, M., and Manicardi, G. C. (2019). The evolutionary history and functional divergence of trehalase (treh) genes in insects. Front. Physiol. 10:62. doi: 10.3389/fphys.2019.00062
Ohnishi, A., Hull, J. J., and Matsumoto, S. (2006). Targeted disruption of genes in the Bombyx mori sex pheromone biosynthetic pathway. Proc. Natl. Acad. Sci. U.S.A. 103, 4398–4403. doi: 10.1073/pnas.0511270103
Parkinson, N. M., Conyers, C. M., Keen, J. N., MacNicoll, A. D., Smith, I., and Weaver, R. J. (2003). cDNAs encoding large venom proteins from the parasitoid wasp Pimpla hypochondriaca identified by random sequence analysis. Comp. Biochem. Physiol. 134, 513–520. doi: 10.1016/s1532-0456(03)00041-3
Pathak, A., Munjal, A., and Parkash, R. (2018). Cold acclimation conditions constrain plastic responses for resistance to cold and starvation in Drosophila immigrans. Biol. Open 7:bio034447. doi: 10.1242/bio.034447
Santos, R., Alves-Bezerra, M., Rosas-Oliveira, R., Majerowicz, D., Meyer-Fernandes, J. R., and Gondim, K. C. (2012). Gene identification and enzymatic properties of a membrane-bound trehalase from the ovary of Rhodnius prolixus. Arch. Insect. Biochem. Physiol. 81, 199–213. doi: 10.1002/arch.21043
Schmidtberg, H., Shukla, S. P., Halitschke, R., Vogel, H., and Vilcinskas, A. (2019). Symbiont-mediated chemical defense in the invasive ladybird Harmonia axyridis. Ecol. Evol. 9, 1715–1729. doi: 10.1002/ece3.4840
Shi, Z. K., Liu, X. J., Xu, Q. Y., Qin, Z., Wang, S., Zhang, F., et al. (2016). Two novel soluble trehalase genes cloned from Harmonia axyridis and regulation of the enzyme in a rapid changing temperature. Comp. Biochem. Physiol. B 198, 10–18. doi: 10.1016/j.cbpb
Shukla, E., Thorat, L., Bendre, A. D., Jadhav, S., Pal, J. K., Nath, B. B., et al. (2018). Cloning and characterization of trehalase: a conserved glycosidase from oriental midge, Chironomus ramosus. 3 Biotech 8:352. doi: 10.1007/s13205-018-1376-y
Shukla, E., Thorat, L. J., Nath, B. B., and Gaikwad, S. M. (2015). Insect trehalase: physiological significance and potential applications. Glycobiology 25, 357–367. doi: 10.1093/glycob/cwu125
Takiguchi, M., Niimi, T., Su, Z. H., and Yaginuma, T. (1992). Trehalase from male accessory-gland of an in-sect, Tenebrio molitor. cDNA sequencing and devel-opmental profile of the gene expression. Biochem. J. 288, 19–22. doi: 10.1016/0003-9861(92)90262-U
Tan, Y. A., Xiao, L. B., Sun, Y., Zhao, J., Bai, L. X., and Xiao, Y. F. (2014). Molecular characterization of soluble and membrane-bound trehalases in the cotton mirid bug, Apolygus lucorum. Arch. Insect Biochem. Physiol. 86, 107–121. doi: 10.1002/arch.21166
Tang, B., Chen, X. F., Liu, Y., Tian, H. G., Liu, J., Hu, J., et al. (2008). Characterization and expression patterns of a membrane-bound trehalase from Spodoptera exigua. BMC Mol. Biol. 9:51. doi: 10.1186/1471-2199-9-51
Tang, B., Wei, P., Zhao, L. N., Shi, Z. K., Shen, Q. D., Yang, M. M., et al. (2016). Knockdown of five trehalase genes using RNA interference regulates the gene expression of the chitin biosynthesis pathways in Tribolium castaneum. BMC Biotechnol. 16:67. doi: 10.1186/s12896-016-0297-2
Tang, B., Xu, Q., Zou, Q., Fang, Q., Wang, S. G., and Ye, G. Y. (2012). Sequencing and characterization of glycogen synthase and glycogen phosphorylase genes from Spodoptera exigua and analysis of their function in starvation and excessive sugar intake. Arch. Insect. Biochem. Physiol. 80, 42–62. doi: 10.1002/arch.21027
Tang, B., Yang, M. M., Shen, Q. D., Xu, Y. X., Wang, H. J., and Wang, S. G. (2017). Suppressing the activity of trehalase with validamycin disrupts the trehalose and chitin biosynthesis pathways in rice brown planthopper, Nilaparvata lugens. Pestic. Biochem. Physiol. 137, 81–90. doi: 10.1016/j.pestbp.2016.10.003
Tang, B., Zhang, L., Xiong, X. P., Wang, H. J., and Wang, S. G. (2018). Advances in trehalose metabolism and its regulation of insect chitin synthesis. Sci. Agric. Sin. 51, 697–707. doi: 10.3864/j.issn.0578-1752.2018.04.009
Tatun, N., Singtripop, T., and Sakurai, S. (2008). Dual control of midgut trehalase activity by 20-hydroxyecdysone and an inhibitory factor in the bamboo borer Omhisa fuscidentalis Hampson. J. Insect Physiol. 54, 351–357. doi: 10.1016/j.jinsphys
Thompson, S. N. (2003). Trehalose - the insect ‘blood’ sugar. Adv. Insect Physiol. 31, 203–285. doi: 10.1016/S0065-2806(03)31004-5
Wegener, G., Macho, C., Schlöder, P., Kamp, G., and Ando, O. (2010). Long-term effects of the trehalase inhibitor Trehazolin on trehalase activity in locust flight muscle. J. Exp. Biol. 213, 3852–3857. doi: 10.1242/jeb.042028
Wegener, G., Tschiedel, V., Schloder, P., and Ando, O. (2003). The toxic and lethal effects of the trehalase inhibitor Trehazolin in locusts are caused by hypoglycaemia. J. Exp. Biol. 206, 1233–1240. doi: 10.1242/jeb.00217
Wu, J. J., Chen, Z. C., Wang, Y. W., Fu, K. Y., Guo, W. C., and Li, G. Q. (2019). Silencing chitin deacetylase 2 impairs larval-pupal and pupal-adult molts in Leptinotarsa decemlineata. Insect Mol. Biol. 28, 52–64. doi: 10.1111/imb.12524
Wu, M. J., Xu, Q. Y., Liu, Y., Shi, X. R., Shen, Q. D., Yang, M. M., et al. (2016). The super cooling point change of Harmonia axyridis under low temperature stress and its cold-resistance genes’ expression analysis. Sci. Agric. Sin. 49, 677–685. doi: 10.3864/j.issn.0578-1752.2016.04.007
Xie, Y. F., Yang, W. J., Dou, W., and Wang, J. J. (2013). Characterization of the cDNA encoding membrane-bound trehalase, its expression and enzyme activity in Bactrocera dorsalis (Diptera:Tephritidae). Fla. Entomol. 96, 1233–1242. doi: 10.1653/024.096.0401
Yamada, T., Habara, O., Yoshii, Y., Matsushita, R., Kubo, H., Nojima, Y., et al. (2019). Role of glycogen in development and adult fitness in Drosophila. Development 146:dev176149. doi: 10.1242/dev.176149
Yang, M. M., Zhao, L. N., Shen, Q. D., Xie, G. Q., Wang, S. G., and Tang, B. (2017). Knockdown of two trehalose-6-phosphate synthases severely affects chitin metabolism gene expression in the brown planthopper Nilaparvata lugens. Pest Manag. Sci. 73, 206–216. doi: 10.1002/ps.4287
Ye, C., Jiang, Y. D., An, X., Yang, L., Shang, F., Niu, J., et al. (2019). Effects of RNAi-based silencing of chitin synthase gene on moulting and fecundity in pea aphids (Acyrthosiphon pisum). Sci. Rep. 9:3694. doi: 10.1038/s41598-019-39837-4
Yu, C. H., Lu, D., Lin, R. H., Wang, X. J., Jiang, H., and Zhao, F. (2008). Trealose—the blood sugar in insects. Chin. Bull. Entomol. 45, 832–837.
Zhang, D. W., Xiao, Z. J., Zeng, B. P., Li, K., and Tang, Y. L. (2019b). Insect behavior and physiological adaptation mechanisms under starvation stress. Front. Physiol. 10:163. doi: 10.3389/fphys.2019.00163
Zhang, D. W., Li, Y., Zang, M., Wang, S. S., Xiao, Z. J., Zeng, B. P., et al. (2019a). Expression and function of trehalase genes TRE2-like and TRE2 during adult eclosion in Harmonia axyridis (Coleoptera: Coccinellidae). Acta Entomol. Sin. 62, 663–671.
Zhang, L., Zhu, S. C., Zheng, H., Shen, Q. D., Wang, S. G., and Tang, B. (2017b). Regulatory function of trehalase genes on chitin metabolism in the cuticle of Nilaparvata lugens. Sci. Agric. Sin. 50, 1047–1056. doi: 10.3864/j.issn.0578-1752.2017.06.006
Zhang, L., Qiu, L. Y., Yang, H. L., Wang, H. J., Zhou, M., Wang, S. G., et al. (2017a). Study on the effect of wing bud chitin metabolism and its developmental network genes in the brown planthopper, Nilaparvata lugens by knockdown TRE gene. Front. Physiol. 8:750. doi: 10.3389/fphys.2017.00750
Zhao, L. N., Yang, M. M., Shen, Q. D., Liu, X., Shi, Z. K., Wang, S. G., et al. (2016). Functional characterization of three trehalase genes regulating the chitin metabolism pathway in rice brown planthopper using RNA interference. Sci. Rep. 6:27841. doi: 10.1038/srep27841
Zhao, X., Song, X. X., Li, Y. P., Yu, C. X., Zhao, Y., Gong, M., et al. (2018). Gene expression related to trehalose metabolism and its effect on Volvariella volvacea under low temperatures stress. Sci. Rep. 8:11011. doi: 10.1038/s41598-018-29116-z
Keywords: Harmonia axyridis, physiological activities, RNA interference, TRE2-like, TRE2
Citation: Li Y, Chen X, Wang S-S, Pan B-Y, Wang S-G, Wang S and Tang B (2019) Evaluation of the Expression and Function of the TRE2-like and TRE2 Genes in Ecdysis of Harmonia axyridis. Front. Physiol. 10:1371. doi: 10.3389/fphys.2019.01371
Received: 06 September 2019; Accepted: 15 October 2019;
Published: 01 November 2019.
Edited by:
Peng He, Guizhou University, ChinaReviewed by:
Kai Lu, Fujian Agriculture and Forestry University, ChinaShoaib Freed, Bahauddin Zakariya University, Pakistan
Senthil Kumar Nachimuthu, Mizoram University, India
Copyright © 2019 Li, Chen, Wang, Pan, Wang, Wang and Tang. This is an open-access article distributed under the terms of the Creative Commons Attribution License (CC BY). The use, distribution or reproduction in other forums is permitted, provided the original author(s) and the copyright owner(s) are credited and that the original publication in this journal is cited, in accordance with accepted academic practice. No use, distribution or reproduction is permitted which does not comply with these terms.
*Correspondence: Bin Tang, dGJ6bTYxMUB5YWhvby5jb20=
†These authors have contributed equally to this work