- 1Guangzhou Jinan Biomedicine Research and Development Center, Institute of Biomedicine, College of Life Science and Technology, Jinan University, Guangzhou, China
- 2Key Laboratory of Virology of Guangzhou, Jinan University, Guangzhou, China
- 3Key Laboratory of Bioengineering Medicine of Guangdong Province, Jinan University, Guangzhou, China
- 4College of Pharmacy, Jinan University, Guangzhou, China
- 5Integrated Chinese and Western Medicine Postdoctoral Research Station, Jinan University, Guangzhou, China
Although the efficacy of herbal medicines (HMs) and traditional Chinese medicines (TCMs) in human diseases has long been recognized, their development has been hindered in part by a lack of a comprehensive understanding of their mechanisms of action. Indeed, most of the compounds extracted from HMs can be metabolized into specific molecules by host microbiota and affect pharmacokinetics and toxicity. Moreover, HMs modulate the constitution of host intestinal microbiota to maintain a healthy gut ecology. Dietary interventions also show great efficacy in treating some refractory diseases, and the commensal microbiota potentially has significant implications for the high inter-individual differences observed in such responses. Herein, we mainly discuss the contribution of the intestinal microbiota to high inter-individual differences in response to HMs and TCMs, and especially the already known metabolites of the HMs produced by the intestinal microbiota. The contribution of commensal microbiota to the inter-individual differences in response to dietary therapy is also briefly discussed. This review highlights the significance of intestinal microbiota-associated metabolites to the efficiency of HMs and dietary interventions. Our review may help further identify the mechanisms leading to the inter-individual differences in the effectiveness of HM and dietary intervention from the perspective of their interactions with the intestinal microbiota.
Background
The function of herbal medicines (HMs) and traditional Chinese medicines (TCMs) in the remedial and prophylactic management of human diseases has been recognized for a long time (Qiu, 2007; Fan et al., 2014; Wang et al., 2017; Xu et al., 2017; Nie et al., 2018; Wu and Tan, 2019), while the mechanisms of action of HMs remain largely unknown. Traditional studies focused on identifying the specific bioactive compounds in HMs, and such strategies have been successful in developing certain compounds isolated from HMs into novel drugs (Xu et al., 2017; Feng et al., 2019). However, most components extracted from HMs exhibit poor bioactivity and bioavailability (Xu et al., 2017; Feng et al., 2019). Indeed, the pharmacological activity of HMs largely depends on intestinal microbiota-dependent biotransformation (Xu et al., 2016; Aguilar-Toalá et al., 2018). Compared to the primary drugs, metabolites produced by the intestinal microbiota often exhibit greater pharmacological activity and are more easily absorbed (Inao et al., 2004; Hussain et al., 2016). Moreover, several components of HMs can serve as nutrition for the growth of specific microbiota and hence modulate the constitution of host intestinal microbiota (Xu et al., 2017; Feng et al., 2019). Therefore, the contribution of host intestinal microbiota-mediated biotransformation to the efficacy of HMs cannot be underestimated.
Indeed, the importance of the intestinal microbiota to human health and pathophysiology is indisputable. The beneficial effects of the intestinal microbiota are primarily contributed by the intrinsic constituents of the intestinal microbiota and the microbiota-associated metabolites, especially the subsets generated from beneficial bacteria (Rooks and Garrett, 2016; Bhat and Kapila, 2017; Hasegawa et al., 2017; Postler and Ghosh, 2017; Aguilar-Toalá et al., 2018; Cani, 2019; Silverman, 2019). The composition of the intestinal microbiota, and more specifically the metabolites generated through their biotransformation, has been shown to be closely associated with the large inter-individual differences observed in responses to drugs and dietary interventions (Coryell et al., 2018; Gong et al., 2018; Gopalakrishnan et al., 2018; Nie et al., 2018; Olson et al., 2018; Rothhammer et al., 2018; Routy et al., 2018; Maini Rekdal et al., 2019; Zimmermann et al., 2019a). Of note, in vivo drug activity, including pharmacokinetics and toxicity, is closely associated with the gut microbiota (Coryell et al., 2018; Gong et al., 2018; Gopalakrishnan et al., 2018; Nie et al., 2018; Olson et al., 2018; Routy et al., 2018; Maini Rekdal et al., 2019; Zimmermann et al., 2019a). Accumulating evidence reveals that intestinal microbiota are crucial contributors to the high inter-individual differences in dietary intervention efficacy in treating some refractory diseases (Flint et al., 2014; Thorburn et al., 2014; Buffington et al., 2016; Rioscovián et al., 2016; Hasegawa et al., 2017; Nie et al., 2018; Requena et al., 2018), such as the anti-seizure effect of the ketogenic diet (KD) (Olson et al., 2018). However, the interaction between HMs or diet therapy and the host intestinal microbiota remains largely unknown.
Owing to a range of factors, including host-intrinsic, host-extrinsic, and environmental factors, the taxonomic composition of the intestinal microbiota varies greatly among individuals (Tsb et al., 2018). It is critical to obtain a clear understanding of the links between HMs or dietary interventions and their metabolites from commensal microbiota. Herein, we mainly discuss the metabolites produced from TCMs and HMs by the intestinal microbiota (Figure 1). The contribution of commensal microbiota to the high inter-individual differences in dietary intervention efficacy is also briefly discussed. Our review further suggests that the effect of microbiota should be considered while developing new dietary guidelines or drugs for clinical application.
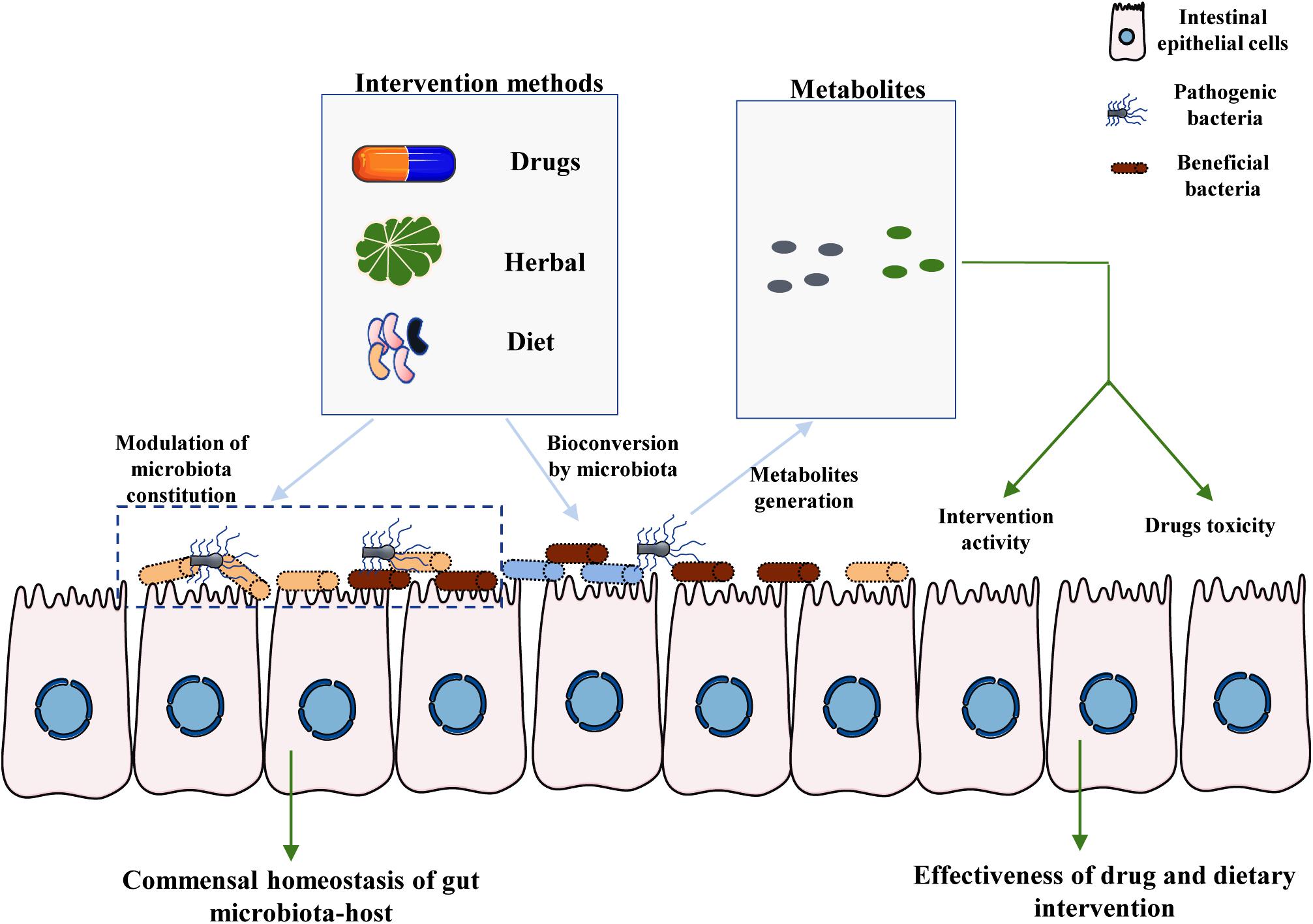
Figure 1. Crucial roles of intestinal microbiota-associated metabolites in the effectiveness of drug and dietary interventions. There are two main pathways by which the commensal microbiota affects the toxicity and efficacy of drug and dietary intervention. First, the specific components of HMs and diet provide nutrition to specific bacteria, including both beneficial bacteria and pathogenic bacteria, thereby modulating the homeostasis of the interaction between gut microbiota and the host. Moreover, particular components of the host diet and medicines can be metabolized by commensal microbiota to generate specific metabolites. The final metabolites may affect the toxicity and efficiency of drugs and dietary interventions, partly mediating the large inter-individual differences observed among hosts.
Intestinal Microbiota-Associated Metabolites of the Compounds Isolated From HMs
Herbal medicines have significantly contributed to human health through disease prophylaxis and therapy (Xu et al., 2017; Feng et al., 2019). The term HM covers raw and processed plants such as the roots, leaves, flowers, berries, and/or seeds from one or more plants (Feng et al., 2019). Materials derived from animals, fungi, and minerals are also regarded as HMs in some traditions (Xu et al., 2017; Feng et al., 2019). Although most of the supposed pharmacological effects of HMs were determined by preclinical researches or even empirical study alone, multiple traditional medicine systems, such as TCMs, Ayurveda, and Islamic medicine, are dominated by HMs (Xu et al., 2017). However, the mechanisms of action of most HMs and the reasons for the different responses of different individuals remain unclear (Xu et al., 2016, 2017; Singh et al., 2017; Nie et al., 2018; Maini Rekdal et al., 2019). Of note, most of the chemicals derived from HMs exhibit poor bioactivity and bioavailability (Xu et al., 2017). However, intestinal microbes are involved in the metabolism of drugs (Maini Rekdal et al., 2019; Zimmermann et al., 2019a, b), especially the compounds extracted from HMs (Nie et al., 2018; Tong et al., 2018). Such biotransformation may contribute to explaining the great inter-individual differences in response to HMs because the constitution of gut microbiota varies among individuals (Xu et al., 2016; Tsb et al., 2018; Maini Rekdal et al., 2019). In this section, we mainly attempt to gain a more comprehensive and detailed understanding of the interactions between HMs and the intestinal microbiota. The role of microbiota in the in vivo activity and toxicity of chemical drugs is also discussed.
The compounds extracted from HMs that can be metabolized by the intestinal microbiota are mainly classified into subsets based on their chemical skeletons and include flavonoids, glycosides, terpenoids, anthraquinones, alkaloids, and organic acids (Table 1). Of these compounds, flavonoids are the most thoroughly studied, and most are degraded into flavone glycosides by the microbiota once the flavonoid enters the large intestine (Table 1). However, the final metabolites vary according to the specific medication and particular gut bacterial composition (Table 1). Bifidobacteria may be the group of microorganisms that can metabolize the widest range of compounds, including soy isoflavones, puerarin, ginsenoside, and sennoside (Table 1). Moreover, several specific bacteria can metabolize different compounds into the same metabolites. For instance, Bifidobacterium can metabolize both soy isoflavones and puerarin into daidzein (Table 1). Of note, the polyphenolics of berries and pomegranate fruit, a compound in unconventional HMs, can be metabolized by Bifidobacterium pseudocatenulatum INIA p815 into urolithin A, which has multiple activities, including combating inflammation, oxidation, and aging, and enhancing gut barrier function (Singh et al., 2019). Collectively, the metabolism of HMs may not be highly dependent on a specific bacterium. However, the specific role of intestinal microbiota in the metabolism of HMs needs to be confirmed in clinical studies in the future, as the existing studies regarding their relationship refer only to preclinical studies.
In addition to the HMs, the gut microbiota is also closely associated with the in vivo activity of chemical drugs. Given that prior influential studies have revealed the gut microbes involved in drug metabolism and their potential genes (Zimmermann et al., 2019a, b), we briefly discuss the role of microbiota-mediated biotransformation in drug activity and toxicity through introducing several representative drugs (Table 2). For instance, gut microbes have been suggested to be crucial factors in the conversion of L-dopa to dopamine (Maini Rekdal et al., 2019). The bioconversion of L-dopa to dopamine depends on a pyridoxal phosphate-dependent tyrosine decarboxylase from Enterococcus faecalis followed by transformation of dopamine to m-tyramine by a molybdenum-dependent dehydroxylase from Eggerthella lenta (Maini Rekdal et al., 2019). In addition, the gut microbiota is responsible for varying responses to simvastatin treatment, resulting in vast differences in the hypolipidemic effect of simvastatin among patients (Krauss et al., 2013; He et al., 2017). Furthermore, although PD-1 inhibitors exhibit potent activity against cancer by blocking a “checkpoint” molecule on T cells, only 25% of patients respond well to PD-1 blockers. The gut microbiota is a crucial factor in determining the response of an individual to various treatments (Gopalakrishnan et al., 2018; Routy et al., 2018). Gut microbes are also a crucial factor affecting the in vivo drug toxicity. For example, diurnal variation in acute liver injury caused by acetaminophen is partly mediated by 1-phenyl-1,2-propanedione, a metabolite of acetaminophen generated by gut microbiota (Gong et al., 2018). Interestingly, acetaminophen hepatotoxicity can be reduced through postbiotic-induced autophagy by Lactobacillus fermentum (Dinic et al., 2017), which demonstrates that different bacteria play distinct roles in the toxicity of the same drug. These findings suggest that an understanding of the interaction between intestinal microbiota and drug metabolism is critical for developing new drugs that are efficacious, which is significant for the frequent emergence of drug-resistance.
Gut Microbes: Crucial Factors for the Function of TCM
It has long been known that TCM is effective for treating many human diseases, including influenza virus infection, cancer, diabetes, and cardiovascular diseases (Qiu, 2007; Fan et al., 2014; Wang et al., 2017; Xu et al., 2017; Nie et al., 2018; Wu and Tan, 2019). The fundamental functions and applications of TCM depend on the compatible application of herbal formulas (FuFang in Chinese) based on ancient empirical philosophies such as Yin-Yang (Dong et al., 2018). However, the mechanisms of action of TCM remain largely unclear or unknown. Recent insights into TCM have focused on its interactions with the gut microbiota (Xu et al., 2017; Feng et al., 2019; Wu and Tan, 2019). Firstly, the carbohydrates in HMs cannot be digested by the human body, while the human gut microbiome encodes thousands of carbohydrate-active enzymes to digest herbal carbohydrates (Xu et al., 2017; Lu et al., 2019). Secondly, the non-carbohydrate bioactive compounds in TCM, particularly triterpene glycosides, flavonoids, isoflavones, iridoid glycosides, alkaloids, and tannins, have poor lipophilicity, high hydrogen-bonding capacity, and high molecular flexibility, which limit the bioavailability of TCM (Xu et al., 2017). However, these non-carbohydrate compounds can be metabolized into several metabolites by the gut microbiota, increasing the efficiency of intestinal absorption and thereby improving their bioavailability (Xu et al., 2017). Moreover, most TCM formulas can reshape the structure of commensal flora, such as by increasing the level of beneficial bacteria and reducing the abundance of harmful bacteria (Table 3). Of note, the enrichment of beneficial gut microbes and the reduction of harmful gut microbes is not merely a result of disease symptom improvement, because the recovery of the balance of the gut microbiota usually occurs before an improvement in the disease symptoms (Xu et al., 2015). Collectively, the efficacy of TCMs may be the comprehensive outcome of both reshaping the microbiota structure and the complex interaction between intestinal microbiota and multiple chemical substances in TCMs.
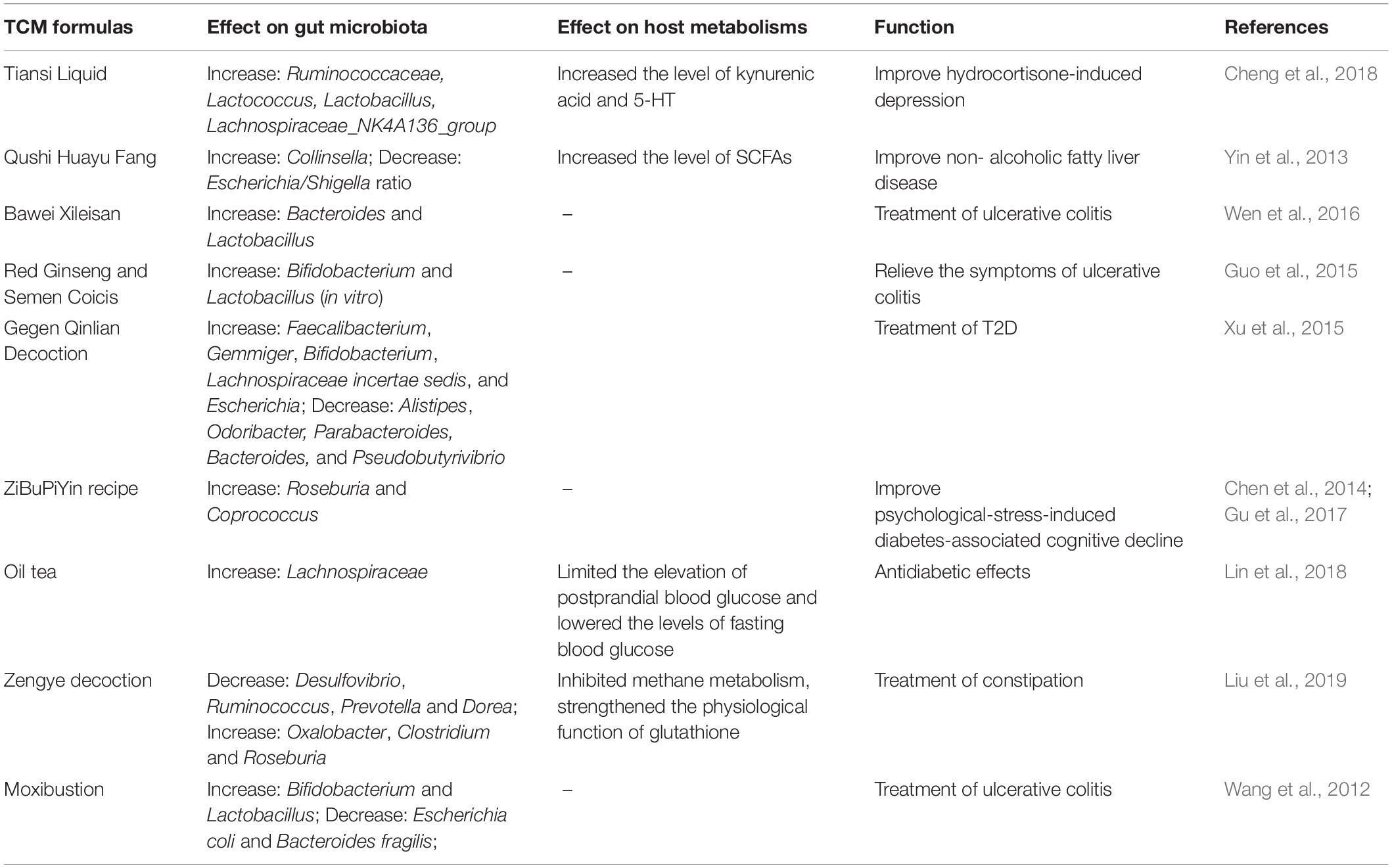
Table 3. Effect of Traditional Chinese medicines (TCM) formulas on the constitution of commensal microbiota and host metabolisms in indicated diseases.
The most typical example of this is the excellent efficacy of TCMs in the management of type 2 diabetes (T2D) (Xu et al., 2015; Nie et al., 2018; Tong et al., 2018; Cheng F. R. et al., 2019; Cheng J. et al., 2019; Han et al., 2019; Li et al., 2019; Lu et al., 2019; Shi et al., 2019; Wu et al., 2019; Yuan et al., 2019). The major component of HMs, such as the polysaccharides extracted from Hirsutella sinensis, provides nutrition to specific bacteria, thereby modulating the constitution of the intestinal microbiota to improve T2D (Xu et al., 2015, 2017; Nie et al., 2018; Tong et al., 2018; Wu et al., 2019; Table 3). Of note, a multicenter, randomized, open-label clinical trial revealed that metformin and the Chinese herbal formula AMC (including Rhizoma Anemarrhenae, Momordica charantia, Coptis chinensis, Salvia miltiorrhiza, red yeast rice, Aloe vera, Schisandra chinensis, and dried ginger) may ameliorate T2D with hyperlipidemia by enriching beneficial bacteria, including Blautia and Faecalibacterium spp. (Tong et al., 2018). In addition, treatment of Gegen Qinlian Decoction (GQD), another TCM formula, can enrich the gut in beneficial bacteria such as Faecalibacterium spp., which is associated with the anti-diabetic effect of GQD (Xu et al., 2015; Table 3). Indeed, under fermentation by the intestinal microbiota, HMs can be metabolized into various chemical substances with wide-ranging activities that improve host health (Yang et al., 2012; Nie et al., 2018; Wu et al., 2019) and jointly enhance the gut barrier, control insulin resistance, and reduce inflammation in the host (Nie et al., 2018). Furthermore, HMs regulate many complex chemical interactions in the gut, thereby maintaining a healthy gut ecology (Nie et al., 2018), which is important in recovery from gut dysbiosis. However, whether these altered microbiotas metabolized specific components in TCMs into functional molecules remains uncertain. Metabolomics analysis is an ideal method for determining the altered microbiota-associated metabolites of TCMs.
Effect of Intestinal Microbiota-Associated Metabolites on the Efficiency of Dietary Therapy
Dietary interventions have become an effective method for treating some refractory diseases, with the effects being associated with the commensal microbiota of the host Richards J. L. et al., 2016; Wu et al., 2016). The KD has long been known to exhibit high efficacy against refractory seizure, despite the response rate being low among tested patients (Kwan and Brodie, 2000; Olson et al., 2018). A recent influential study revealed that the gut microbiota was responsible for the high inter-individual differences observed in the anti-seizure effect of the KD (Olson et al., 2018). Ketogenic diet-associated Akkermansia and Parabacteroides confer seizure protection to mice fed a controlled diet by reducing the level of gamma-glutamyl amino acids and increasing the GABA and glutamate content in the brain (Olson et al., 2018). In addition, a Mediterranean diet, which is based on the high consumption of cereals, fruit, vegetables, and legumes, has been associated with the prevention of cardiovascular diseases and asthma (Castro-Rodriguez et al., 2008; Estruch et al., 2013; Blanco Mejía et al., 2019). The Mediterranean diet increases the abundance of Lactobacillus in the mammary gland microbiota and subsequently elevates the levels of bile acid and bacterial-modified metabolites in breast cyst fluid (Shively et al., 2018). However, the beneficial effects of the Mediterranean diet on human health also depend, in part, on non-bacterial metabolites, especially ω-3 fatty acids, which exert larger anti-inflammatory effects (Thorburn et al., 2014). Further, given that the Mediterranean diet is rich in fiber, SCFAs may mediate the beneficial effect of this diet, since the administration of SCFAs is associated with significant improvements in cardiovascular diseases (Richards L. B. et al., 2016); this requires further research. Of note, in the gastrointestinal tract of human patients with type II diabetes, the administration of Bifidobacterium increases the abundance of Akkermansia muciniphila, with both microbes being able to generate SCFAs, thereby improving insulin resistance and limiting inflammation and consequently improving the symptoms of obesity (Cani, 2019). Furthermore, arsenic poisoning arising from the ingestion of contaminated food and drinking water is a challenging disease to treat (Coryell et al., 2018). A promising finding is that gut microbes, especially Faecalibacterium, provide full protection against acute arsenic toxicity in a mouse model (Coryell et al., 2018).
However, some of the observed dietary effects have not yet been associated with specific intestinal microbes or with specific metabolites. For instance, a maternal high-fat diet negatively impacts the social behavior of offspring, resulting in a deficiency in synaptic plasticity in the ventral tegmental area and in oxytocin production, but the administration of Lactobacillus reuteri restores oxytocin levels, synaptic plasticity, and healthy social behaviors in mice (Buffington et al., 2016). It has also been recognized that a Malawian diet may induce kwashiorkor, an enigmatic form of severe acute malnutrition. In a study involving 317 Malawian twin pairs, researchers found that an altered gut microbiota constitution in response to the Malawian diet significantly contributed to the development of kwashiorkor, although the mechanism involved remains unknown (Smith et al., 2013). Notably, oligosaccharides were less abundant in the milk from mothers of severely stunted infants, and the administration of sialylated milk oligosaccharides reversed infant undernutrition in a microbiota-dependent manner (Smith et al., 2013). Such results were also confirmed in piglets that received the same diet as the human infants (Charbonneau et al., 2016), suggesting that microbiota associated-metabolites of oligosaccharides may be a crucial factor in such processes. In young children, a negative association between dietary fiber and plasma insulin levels has been observed only in those whose gut microbiota showed a high abundance of Bacteroides and Prevotella and not in those whose gut microbiota exhibited a higher proportion of Bifidobacterium (Zhong et al., 2019). This suggests a potential function for Bacteroides and Prevotella in elevating insulin levels. Indeed, convincing epidemiological studies have indicated that specific dietary components may be crucial for the pathogenesis of some diseases such as asthma and allergies (Eder et al., 2006; Graham, 2006). For example, a carnitine-rich diet induces the symptoms of atherosclerosis in a gut microbiota-dependent manner in humans and mice (Koeth et al., 2013). Specifically, the gut microbiota in humans and mice mediates the metabolism of dietary choline and phosphatidylcholine to produce trimethylamine, which is further transformed into trimethylamine-N-oxide by hepatic flavin monooxygenases, thereby promoting the development of atherosclerosis. However, the specific microbial taxa contributing to this process require further investigation.
Conclusion and Future Perspective
The beneficial effect of HMs and dietary therapy in several refractory diseases is generally appreciated, but the underlying mechanisms involved remain obscure. However, their interaction with the host microbiota seems to be a critical factor in such processes. Indeed, a growing number of studies indicate that the commensal microbiota plays a crucial role in maintaining host health and that the constitution of the intestinal microbiota exhibits large inter-individual differences. Moreover, most components in HMs and dietary interventions can modulate the constitution of the microbiota, which may disrupt or maintain homeostasis in the host. Collectively, it is not surprising that the gut microbiota, and especially microbiota-associated metabolites, may be a crucial mediator linking HMs or dietary therapy and the physiological status of the host. Therefore, it is important to consider the effects of biotransformation by commensal microbiota when designing herbal formula dietary therapy to achieve optimal success in treating diseases, particularly in the case of precision medicine. It is also essential to determine the optimal timing of administrating HMs and specific diets, in particular given that the composition of the gut microbiota exhibits diurnal variation. Indeed, microbiota-associated metabolites have several attractive properties, including known chemical structures and long shelf lives (Aguilar-Toalá et al., 2018). In particular, these metabolites are able to mimic the health effects mediated by probiotics while avoiding the administration of live bacteria, which can produce harmful reactions such as the local inflammatory response induced by the administration of Salmonella (Tsilingiri et al., 2012). However, the importance of postbiotics does not diminish the beneficial effect of probiotics when there is stable colonization of the gut, because live bacteria undoubtedly provide more metabolites than can be provided using postbiotics. The future of next-generation probiotics lies not only in supplementation using beneficial bacteria strains but also in providing and maintaining the ecological context necessary to sustain them. The direct administration of these probiotic-associated metabolites should provide a great advantage over traditional probiotics for several types of patients, including those harboring intestinal pathogens. Furthermore, since metabolites from the intestinal microbiota can also partially mediate the toxicity of some medicines in vivo, it will also be valuable to further examine these associations in order to assist in developing novel approaches to reducing the toxicity of HMs and TCMs.
Author Contributions
YlW contributed to the conception, design, collection and assembly of references, discussion, interpretation, and writing of the manuscript. SQ contributed to the collection and assembly of references, interpretation of the article, and writing of the manuscript. JJ, LH, and FL contributed to the collection and assembly of references. FJ, ZR, and YfW contributed to the conception, design, interpretation of the article, and the final article approval.
Funding
This work was supported by grants from the Key Laboratory of Virology of Guangzhou, China (201705030003), the National Natural Science Foundation of China (Nos. 81573471 and 81872908), Key Projects of Biological Industry Science and Technology of Guangzhou China (Grant No. 201504291048224), Guangzhou Industry, University and Research Collaborative Innovation Major Project (No. 201704030087), and the Public Service Platform of the South China Sea for R&D Marine Biomedicine Resources, Marine Biomedical Research Institute, Guangdong Medical University, Zhanjiang, China.
Conflict of Interest
The authors declare that the research was conducted in the absence of any commercial or financial relationships that could be construed as a potential conflict of interest.
References
Abdel-Hafez, A. A., Meselhy, M. R., Nakamura, N., Hattori, M., El-Gendy, M. A., Mahfouz, N. M., et al. (2010). New paeonilactone-A adducts formed by anaerobic incubation of paeoniflorin with Lactobacillus brevis in the presence of arylthiols. Chem. Inf. 32, 918–920. doi: 10.1248/cpb.49.918
Aguilar-Toalá, J. E., García-Varela, R., García, H. S., Mata-Haro, V., González-Córdova, A. F., Vallejo-Cordoba, B., et al. (2018). Postbiotics: an evolving term within the functional foods field. Trends Food Sci. Technol. 75, 105–114. doi: 10.1016/j.tifs.2018.03.009
Akao, T. (2000). Differences in the metabolism of glycyrrhizin, glycyrrhetic acid and glycyrrhetic acid monoglucuronide by human intestinal flora. Biol. Pharm. Bull. 23, 1418–1423. doi: 10.1248/bpb.23.1418
Akao, T., Che, Q. M., Kobashi, K., Hattori, M., and Namba, T. (1996). A purgative action of barbaloin is induced by Eubacterium sp. strain BAR, a human intestinal anaerobe, capable of transforming barbaloin to aloe-emodin anthrone. Biol. Pharm. Bull. 19, 136–138. doi: 10.1248/bpb.19.136
Alok, A., Singh, I. D., Singh, S., Kishore, M., Jha, P. C., and Iqubal, M. A. (2017). Probiotics: a new Era of biotherapy. Adv. Biomed. Res. 6:31. doi: 10.4103/2277-9175.192625
Bae, E. A., Han, M. J., Kim, E. J., and Kim, D. H. (2004). Transformation of ginseng saponins to ginsenoside rh2 by acids and human intestinal bacteria and biological activities of their transformants. Arch. Pharm. Res. 27, 61–67. doi: 10.1007/bf02980048
Baltina, L. A. (2003). Chemical modification of glycyrrhizic acid as a route to new bioactive compounds for medicine. Curr. Med. Chem. 10, 155–171. doi: 10.2174/0929867033368538
Bhat, M. I., and Kapila, R. (2017). Dietary metabolites derived from gut microbiota: critical modulators of epigenetic changes in mammals. Nutr. Rev. 75, 374–389. doi: 10.1093/nutrit/nux001
Bisanz, J. E., Spanogiannopoulos, P., Pieper, L. M., Bustion, A. E., and Turnbaugh, P. J. (2018). How to determine the role of the microbiome in drug disposition. Drug Metab. Dispos. 46, 1588–1595. doi: 10.1124/dmd.118.083402
Blanco Mejía, S., Viguiliouk, E., Khan, T., Kendall, C. W. C., Kahleova, H., Raheliæ, D., et al. (2019). Mediterranean diet, cardiovascular disease and mortality in diabetes: a systematic review and meta-analysis of prospective cohort studies and randomized clinical trials AU - becerra-tomás, nerea. Crit. Rev. Food Sci. Nutr. 24, 1–21. doi: 10.1080/10408398.2019.1565281
Borcsa, B., Widowitz, U., Csupor, D., Forgo, P., Bauer, R., and Hohmann, J. (2011). Semisynthesis and pharmacological investigation of lipo-alkaloids prepared from aconitine. Fitoterapia 82, 365–368. doi: 10.1016/j.fitote.2010.11.001
Braune, A., and Blaut, M. (2011). Deglycosylation of puerarin and other aromatic C-glucosides by a newly isolated human intestinal bacterium. Environ. Microbiol. 13, 482–494. doi: 10.1111/j.1462-2920.2010.02352.x
Buffington, S. A., Di Prisco, G. V., Auchtung, T. A., Ajami, N. J., Petrosino, J. F., and Costa-Mattioli, M. (2016). Microbial reconstitution reverses maternal diet-induced social and synaptic deficits in offspring. Cell 165, 1762–1775. doi: 10.1016/j.cell.2016.06.001
Cai, S., Yang, H., Wen, B., Zhu, K., Zheng, X., Huang, J., et al. (2018). Inhibition by microbial metabolites of Chinese dark tea of age-related neurodegenerative disorders in senescence-accelerated mouse prone 8 (SAMP8) mice. Food Funct. 9, 5455–5462. doi: 10.1039/c8fo01512k
Cani, P. D. (2019). Microbiota and metabolites in metabolic diseases. Nat. Rev. Endocrinol. 15, 69–70. doi: 10.1038/s41574-018-0143-9
Castro-Rodriguez, J. A., Luis, G. M., Rojas, J. D., Alfonseda Jose, V. M., and Manuel, S. S. (2008). Mediterranean diet as a protective factor for wheezing in preschool children. J. Pediatr. 152, 823.e–828.e. doi: 10.1016/j.jpeds.2008.01.003
Charbonneau, M. R., O’Donnell, D., Blanton, L. V., Totten, S. M., Davis, J. C., Barratt, M. J., et al. (2016). Sialylated milk oligosaccharides promote microbiota-dependent growth in models of infant undernutrition. Cell 164, 859–871. doi: 10.1016/j.cell.2016.01.024
Chen, J., Liang, L., Zhan, L., Zhou, Y., Zheng, L., Sun, X., et al. (2014). ZiBuPiYin recipe protects db/db mice from diabetes-associated cognitive decline through improving multiple pathological changes. PLoS One 9:e91680. doi: 10.1371/journal.pone.0091680
Cheng, D., Chang, H., Ma, S., Guo, J., She, G., Zhang, F., et al. (2018). Tiansi liquid modulates gut microbiota composition and tryptophan(-)kynurenine metabolism in rats with hydrocortisone-induced depression. Molecules 23:E2832. doi: 10.3390/molecules23112832
Cheng, F. R., Cui, H. X., Fang, J. L., Yuan, K., and Guo, Y. (2019). Ameliorative effect and mechanism of the purified anthraquinone-glycoside preparation from Rheum Palmatum L. on type 2 diabetes mellitus. Molecules 24:1454. doi: 10.3390/molecules24081454
Cheng, J., Zheng, J., Liu, Y. P., and Hao, P. P. (2019). Efficacy of traditional Chinese medication tangminling pill in Chinese patients with type 2 diabetes. Biosci. Rep. 39:BSR20181729. doi: 10.1042/BSR20181729
Coryell, M., McAlpine, M., Pinkham, N. V., McDermott, T. R., and Walk, S. T. (2018). The gut microbiome is required for full protection against acute arsenic toxicity in mouse models. Nat. Commun. 9:5424. doi: 10.1038/s41467-018-07803-9
Couteau, D., Mccartney, A. L., Gibson, G. R., Williamson, G., and Faulds, C. B. (2010). Isolation and characterization of human colonic bacteria able to hydrolyse chlorogenic acid. J. Appl. Microbiol. 90, 873–881. doi: 10.1046/j.1365-2672.2001.01316.x
Ding, H., Zhang, Y., Xu, C., Hou, D., Li, J., Zhang, Y., et al. (2014). Norathyriol reverses obesity- and high-fat-diet-induced insulin resistance in mice through inhibition of PTP1B. Diabetologia 57, 2145–2154. doi: 10.1007/s00125-014-3315-8
Dinic, M., Lukic, J., Djokic, J., Milenkovic, M., Strahinic, I., Golic, N., et al. (2017). Lactobacillus fermentum postbiotic-induced autophagy as potential approach for treatment of acetaminophen hepatotoxicity. Front. Microbiol. 8:594. doi: 10.3389/fmicb.2017.00594
Dong, J., Lu, L., Le, J., Yan, C., Zhang, H., and Li, L. (2018). Philosophical thinking of Chinese traditional medicine. Tradit. Med. Mod. Med. 01, 1–10. doi: 10.1142/s2575900018100018
Eder, W., Ege, M. J., and von Mutius, E. (2006). The asthma epidemic. N. Engl. J. Med. 355, 2226–2235.
Elghali, S., Mustafa, S., Amid, M., Abd Manap, M. Y., Ismail, A., and Abas, F. (2012). Bioconversion of daidzein to equol by Bifidobacterium breve 15700 and Bifidobacterium longum BB536. J. Funct. Foods 4, 736–745. doi: 10.1016/j.foodchem.2018.11.107
Estruch, R., Ros, E., and Martínez-González, M. (2013). Primary prevention of cardiovascular disease with a mediterranean diet. N. Engl. J. Med. 369, 1279–1290.
Eunah, B., Han, M. K., Sunyoung, P., and Donghyun, K. (2002). Metabolism of 20(S)- and 20(R)-ginsenoside Rg3 by human intestinal bacteria and its relation to in vitro biological activities. Biol. Pharm. Bull 25, 58–63. doi: 10.1248/bpb.25.58
Fan, T.-P., Briggs, J., Liu, L., Lu, A., van der Greef, J., Xu, A., et al. (2014). Integrating traditional medicine into modern health care. Science 346:1569.
Feng, W., Ao, H., Peng, C., and Yan, D. (2019). Gut microbiota, a new frontier to understand traditional Chinese medicines. Pharmacol. Res. 142, 176–191. doi: 10.1016/j.phrs.2019.02.024
Flint, H. J., Duncan, S. H., Scott, K. P., and Louis, P. (2014). Links between diet, gut microbiota composition and gut metabolism. Proc. Nutr. Soc. 74, 13–22. doi: 10.1017/s0029665114001463
Fuller, A. T. (1937). IS p-aminobenzenesulphonamide the active agent in prontosil therapy ? Lancet 229, 194–198. doi: 10.1016/s0140-6736(00)97447-6
Gong, S., Lan, T., Zeng, L., Luo, H., Yang, X., Li, N., et al. (2018). Gut microbiota mediates diurnal variation of acetaminophen induced acute liver injury in mice. J. Hepatol. 69, 51–59. doi: 10.1016/j.jhep.2018.02.024
Gonthier, M. P., Verny, M. A., Besson, C., Rémésy, C., and Scalbert, A. (2003). Chlorogenic acid bioavailability largely depends on its metabolism by the gut microflora in rats. J. Nutr. 133, 1853–1859. doi: 10.1093/jn/133.6.1853
Gopalakrishnan, V., Spencer, C. N., Nezi, L., Reuben, A., Andrews, M. C., Karpinets, T. V., et al. (2018). Gut microbiome modulates response to anti–PD-1 immunotherapy in melanoma patients. Science 359, 97–103.
Gotteland, M., Brunser, O., and Cruchet, S. (2006). Systematic review: are probiotics useful in controlling gastric colonization by Helicobacter pylori? Aliment. Pharmacol. Ther. 23, 1077–1086. doi: 10.1111/j.1365-2036.2006.02868.x
Graham, D. (2006). The increase in the prevalence of asthma and allergy: food for thought. Nat. Rev. Immunol. 6, 869–874. doi: 10.1038/nri1958
Gu, C., Zhou, W., Wang, W., Xiang, H., Xu, H., Liang, L., et al. (2017). ZiBuPiYin recipe improves cognitive decline by regulating gut microbiota in Zucker diabetic fatty rats. Oncotarget 8, 27693–27703. doi: 10.18632/oncotarget.14611
Guo, M. Z., Ding, S., Zhao, C. H., Gu, X. X., He, X. Y., Huang, K. L., et al. (2015). Red Ginseng and Semen Coicis can improve the structure of gut microbiota and relieve the symptoms of ulcerative colitis. J. Ethnopharmacol. 162, 7–13. doi: 10.1016/j.jep.2014.12.029
Guthrie, L., Gupta, S., Daily, J., and Kelly, L. (2017). Human microbiome signatures of differential colorectal cancer drug metabolism. NPJ Biofilms Microbiomes 3:27. doi: 10.1038/s41522-017-0034-1
Han, L. H., Li, T. G., Du, M., Chang, R., Zhan, B. Y., and Mao, X. Y. (2019). Beneficial Effects of potentilla discolor bunge water extract on inflammatory cytokines release and gut microbiota in high-fat diet and streptozotocin-induced type 2 diabetic mice. Nutrients 11:E670. doi: 10.3390/nu11030670
Hasegawa, S., Jao, T. M., and Inagi, R. (2017). Dietary Metabolites and Chronic Kidney Disease. Nutrients 9:E358.
He, X., Zheng, N., He, J., Liu, C., Feng, J., Jia, W., et al. (2017). Gut microbiota modulation attenuated the hypolipidemic effect of simvastatin in high-fat/cholesterol-diet fed mice. J. Proteome Res. 16, 1900–1910. doi: 10.1021/acs.jproteome.6b00984
Hsiu, S. L., Lin, Y. T., Wen, K. C., Hou, Y. C., and Chao, P. D. (2003). A deglucosylated metabolite of paeoniflorin of the root of Paeonia lactiflora and its pharmacokinetics in rats. Planta Med. 69, 1113–1118. doi: 10.1055/s-2003-45192
Hussain, A., Bose, S., Wang, J. H., Yadav, M. K., Mahajan, G. B., and Kim, H. (2016). Fermentation, a feasible strategy for enhancing bioactivity of herbal medicines. Food Res. Int. 81, 1–16. doi: 10.1016/j.foodres.2015.12.026
Inao, M., Mochida, S., Matsui, A., Eguchi, Y., Yulutuz, Y., Wang, Y., et al. (2004). Japanese herbal medicine Inchin-ko-to as a therapeutic drug for liver fibrosis. J. Hepatol. 41, 584–591. doi: 10.1016/j.jhep.2004.06.033
Jin, M. J., Kim, I. S., Kim, D. H., and Yoo, H. H. (2014). Effects of intestinal microbiota on the bioavailability of geniposide in rats. J. Agric. Food Chem. 62, 9632–9636. doi: 10.1021/jf502557f
Jung, I. H., Lee, J. H., Hyun, Y. J., and Kim, D. H. (2012). Metabolism of ginsenoside Rb1 by human intestinal microflora and cloning of its metabolizing β-D-glucosidase from Bifidobacterium longum H-1. Biol. Pharm. Bull. 35, 573–581. doi: 10.1248/bpb.35.573
Kang, M. J., Khanal, T., Kim, H. G., Lee, D. H., Yeo, H. K., Lee, Y. S., et al. (2012). Role of metabolism by human intestinal microflora in geniposide-induced toxicity in HepG2 cells. Arch. Pharm. Res. 35, 733–738. doi: 10.1007/s12272-012-0418-y
Khanal, T., Kim, H. G., Choi, J. H., Do, M. T., Min, J. K., Mi, J. K., et al. (2012). Biotransformation of geniposide by human intestinal microflora on cytotoxicity against HepG2 cells. Toxicol. Lett. 209, 246–254. doi: 10.1016/j.toxlet.2011.12.017
Koeth, R. A., Zeneng, W., Levison, B. S., Buffa, J. A., Elin, O., Sheehy, B. T., et al. (2013). Intestinal microbiota metabolism of L-carnitine, a nutrient in red meat, promotes atherosclerosis. Nat. Med. 19, 576–585. doi: 10.1038/nm.3145
Krauss, R. M., Zhu, H., and Kaddurah-Daouk, R. (2013). Pharmacometabolomics of statin response. Clin. Pharmacol. Ther. 94, 562–565. doi: 10.1038/clpt.2013.164
Kwan, P., and Brodie, M. J. (2000). Early identification of refractory epilepsy. N. Engl. J. Med. 342, 314–319. doi: 10.1056/nejm200002033420503
Lee, N. K., Choi, S. H., Park, S. H., Park, E. K., and Kim, D. H. (2004). Antiallergic activity of hesperidin is activated by intestinal microflora. Pharmacology 71, 174–180. doi: 10.1159/000078083
Li, C., Gan, H., Tan, X. L., Hu, Z. X., Deng, B., Sullivan, M. A., et al. (2019). Effects of active ingredients from traditional Chinese medicines on glycogen molecular structure in diabetic mice. Eur. Polym. J. 112, 67–72. doi: 10.1016/j.eurpolymj.2018.12.039
Li, J., Malakhova, M., Mottamal, M., Reddy, K., Kurinov, I., Carper, A., et al. (2012). Norathyriol suppresses skin cancers induced by solar ultraviolet radiation by targeting ERK kinases. Cancer Res. 72, 260–270. doi: 10.1158/0008-5472.CAN-11-2596
Li, L., Luo, G. A., Liang, Q. L., Hu, P., and Wang, Y. M. (2010). Rapid qualitative and quantitative analyses of Asian ginseng in adulterated American ginseng preparations by UPLC/Q-TOF-MS. J. Pharm. Biomed. Anal. 52, 66–72. doi: 10.1016/j.jpba.2009.12.017
Lin, R., He, X., Chen, H., He, Q., Yao, Z., Li, Y., et al. (2018). Oil tea improves glucose and lipid levels and alters gut microbiota in type 2 diabetic mice. Nutr. Res. 57, 67–77. doi: 10.1016/j.nutres.2018.05.004
Liu, D., Lin, L., Lin, Y., Zhong, Y., Zhang, S., Liu, W., et al. (2019). Zengye decoction induces alterations to metabolically active gut microbiota in aged constipated rats. Biomed. Pharmacother. 109, 1361–1371. doi: 10.1016/j.biopha.2018.11.013
Lu, Y. M., Xie, J. J., Peng, C. G., Wang, B. H., Wang, K. C., and Li, L. J. (2019). Enhancing clinical efficacy through the gut microbiota: a new field of traditional Chinese medicine. Engineering 5, 40–49. doi: 10.1016/j.eng.2018.11.013
Ludwig, I. A., Maria, P. D. P., Concepción, C., and Alan, C. (2013). Catabolism of coffee chlorogenic acids by human colonic microbiota. Biofactors 39, 623–632. doi: 10.1002/biof.1124
Maini Rekdal, V., Bess, E. N., Bisanz, J. E., Turnbaugh, P. J., and Balskus, E. P. (2019). Discovery and inhibition of an interspecies gut bacterial pathway for Levodopa metabolism. Science 364:eaau6323. doi: 10.1126/science.aau6323
Matsumoto, M., Ishige, A., Yazawa, Y., Kondo, M., Muramatsu, K., and Watanabe, K. (2012). Promotion of intestinal peristalsis by Bifidobacterium spp. Capable of hydrolysing sennosides in mice. PLoS One 7:e31700. doi: 10.1371/journal.pone.0031700
Merenstein, D., Murphy, M., Fokar, A., Hernandez, R. K., Park, H., Nsouli, H., et al. (2010). Use of a fermented dairy probiotic drink containing Lactobacillus casei (DN-114 001) to decrease the rate of illness in kids: the DRINK study A patient-oriented, double-blind, cluster-randomized, placebo-controlled, clinical trial. Eur. J. Clin. Nutr. 64, 669–677. doi: 10.1038/ejcn.2010.65
Myung-Ah, J., Se-Eun, J., Hong, S. W., Joo, H. M., and Dong-Hyun, K. (2012). The role of intestinal microflora in anti-inflammatory effect of baicalin in mice. Biomol. Ther. 20, 36–42. doi: 10.4062/biomolther.2012.20.1.036
Nie, Q., Chen, H., Hu, J., Fan, S., and Nie, S. (2018). Dietary compounds and traditional Chinese medicine ameliorate type 2 diabetes by modulating gut microbiota. Crit. Rev. Food Sci. Nutr. 59, 848–863. doi: 10.1080/10408398.2018.1536646
Odani, T., Tanizawa, H., and Takino, Y. (1983). Studies on the absorption, distribution, excretion and metabolism of ginseng saponins. II. The absorption, distribution and excretion of ginsenoside Rg1 in the rat. Chem. Pharm. Bull. 31, 292–298. doi: 10.1248/cpb.31.292
Olson, C. A., Vuong, H. E., Yano, J. M., Liang, Q. Y., Nusbaum, D. J., and Hsiao, E. Y. (2018). The gut microbiota mediates the anti-seizure effects of the ketogenic diet. Cell 173, 1728.e13–1741.e13. doi: 10.1016/j.cell.2018.04.027
Ozdal, T., Sela, D. A., Xiao, J. B., Boyacioglu, D., Chen, F., and Capanoglu, E. (2016). The reciprocal interactions between polyphenols and gut microbiota and effects on bioaccessibility. Nutrients 8:78. doi: 10.3390/nu8020078
Postler, T. S., and Ghosh, S. (2017). Understanding the holobiont: how microbial metabolites affect human health and shape the immune system. Cell Metab. 26, 110–130. doi: 10.1016/j.cmet.2017.05.008
Qiu, J. (2007). ‘Back to the future’ for Chinese herbal medicines. Nat. Rev. Drug Discov. 6, 506–507. doi: 10.1038/nrd2350
Requena, T., Martinez-Cuesta, M. C., and Pelaez, C. (2018). Diet and microbiota linked in health and disease. Food Funct. 9, 688–704. doi: 10.1039/c7fo01820g
Richards, J. L., Yu, A. Y., Mcleod, K. H., Mackay, C. R., and Mariño, E. (2016). Dietary metabolites and the gut microbiota: an alternative approach to control inflammatory and autoimmune diseases. Clin. Transl. Immunol. 5:e82. doi: 10.1038/cti.2016.29
Richards, L. B., Li, M., Esch, B. C. A. M. V., Garssen, J., and Folkerts, G. (2016). The effects of short-chain fatty acids on the cardiovascular system. Pharmanutrition 4, 68–111. doi: 10.1016/j.phanu.2016.02.001
Rio, D. D., Stalmach, A., Calani, L., and Crozier, A. (2010). Bioavailability of coffee chlorogenic acids and green tea flavan-3-ols. Nutrients 2, 820–833. doi: 10.3390/nu2080820
Rioscovián, D., Ruasmadiedo, P., Margolles, A., Gueimonde, M., Reyesgavilán, C. G. D. L., and Salazar, N. (2016). Intestinal short chain fatty acids and their link with diet and human health. Front. Microbiol. 7:185. doi: 10.3389/fmicb.2016.00185
Rooks, M. G., and Garrett, W. S. (2016). Gut microbiota, metabolites and host immunity. Nat. Rev. Immunol. 16, 341–352. doi: 10.1038/nri.2016.42
Rothhammer, V., Borucki, D. M., Tjon, E. C., Takenaka, M. C., Chao, C.-C., Ardura-Fabregat, A., et al. (2018). Microglial control of astrocytes in response to microbial metabolites. Nature 557, 724–728. doi: 10.1038/s41586-018-0119-x
Routy, B., Le Chatelier, E., Derosa, L., Duong, C. P. M., Alou, M. T., Daillère, R., et al. (2018). Gut microbiome influences efficacy of PD-1–based immunotherapy against epithelial tumors. Science 359, 91–97.
Sanugul, K., Akao, T., Li, Y., Kakiuchi, N., Nakamura, N., and Hattori, M. (2005). Isolation of a human intestinal bacterium that transforms mangiferin to norathyriol and inducibility of the enzyme that cleaves a C-glucosyl bond. Biol. Pharm. Bull. 28, 1672–1678. doi: 10.1248/bpb.28.1672
Schoefer, L., Mohan, R., Schwiertz, A., Braune, A., and Blaut, M. (2003). Anaerobic degradation of flavonoids by clostridium orbiscindens. Appl. Environ. Microbiol. 69, 5849–5854. doi: 10.1128/aem.69.10.5849-5854.2003
Serra, A., Macia, A., Romero, M. P., Reguant, J., Ortega, N., and Motilva, M. J. (2012). Metabolic pathways of the colonic metabolism of flavonoids (flavonols, flavones and flavanones) and phenolic acids. Food Chem. 130, 383–393. doi: 10.1080/03602532.2018.1485691
Shi, R. F., Wang, Y. P., An, X. F., Ma, J. H., Wu, T. Z., Yu, X. J., et al. (2019). Efficacy of Co-administration of liuwei dihuang pills and ginkgo biloba tablets on albuminuria in type 2 diabetes: a 24-month, multicenter, double-blind, placebo-controlled, randomized clinical trial. Front. Endocrinol. 10:100. doi: 10.3389/fendo.2019.00100
Shively, C. A., Register, T. C., Appt, S. E., Clarkson, T. B., Uberseder, B., Clear, K. Y. J., et al. (2018). Consumption of Mediterranean versus western diet leads to distinct mammary gland microbiome populations. Cell Rep. 25:E43. doi: 10.1016/j.celrep.2018.08.078
Silverman, G. J. (2019). The microbiome in SLE pathogenesis. Nat. Rev. Rheumatol. 15, 72–74. doi: 10.1038/s41584-018-0152-z
Singh, D., Lee, S., and Lee, C. H. (2017). Metabolomics for empirical delineation of the traditional Korean fermented foods and beverages. Trends n Food Sci. Technol. 61, 103–115. doi: 10.1016/j.tifs.2017.01.001
Singh, R., Chandrashekharappa, S., Bodduluri, S. R., Baby, B. V., Hegde, B., Kotla, N. G., et al. (2019). Enhancement of the gut barrier integrity by a microbial metabolite through the Nrf2 pathway. Nat. Commun. 10:89. doi: 10.1038/s41467-018-07859-7
Smith, M. I., Tanya, Y., Manary, M. J., Indi, T., Rajhab, M., Jiye, C., et al. (2013). Gut microbiomes of Malawian twin pairs discordant for kwashiorkor. Science 339, 548–554. doi: 10.1126/science.1229000
Spanogiannopoulos, P., Bess, E. N., Carmody, R. N., and Turnbaugh, P. J. (2016). The microbial pharmacists within us: a metagenomic view of xenobiotic metabolism. Nat. Rev. Microbiol. 14, 273–287. doi: 10.1038/nrmicro.2016.17
Steed, A. L., Christophi, G. P., Kaiko, G. E., Sun, L., Goodwin, V. M., Jain, U., et al. (2017). The microbial metabolite desaminotyrosine protects from influenza through type I interferon. Science 357, 498–502. doi: 10.1126/science.aam5336
Takayama, K., Morita, T., and Tabuch, N. (2014). The effect of anthraquinones in daiokanzoto on increasing the synthesis of sennoside A-metabolic enzyme derived from bifidobacteria. J. Tradit. Med. 30, 215–220.
Thorburn, A. N., Macia, L., and Mackay, C. R. (2014). Diet, metabolites, and “western-lifestyle” inflammatory diseases. Immunity 40, 833–842. doi: 10.1016/j.immuni.2014.05.014
Tomas-Barberan, F., García-Villalba, R., Quartieri, A., Raimondi, S., Amaretti, A., Leonardi, A., et al. (2014). In vitro transformation of chlorogenic acid by human gut microbiota. Mol. Nutr. Food Res. 58, 1122–1131. doi: 10.1002/mnfr.201300441
Tong, X., Xu, J., Lian, F., Yu, X., Zhao, Y., Xu, L., et al. (2018). Structural alteration of gut microbiota during the amelioration of human type 2 diabetes with hyperlipidemia by metformin and a traditional chinese herbal formula: a multicenter, randomized, open label clinical trial. MBio 9:e2392-17. doi: 10.1128/mBio.02392-17
Trinh, H. T., Joh, E. H., Kwak, H. Y., Baek, N. I., and Kim, D. H. (2010). Anti-pruritic effect of baicalin and its metabolites, baicalein and oroxylin A, in mice Anti-tumorPharmacology. Acta Pharmacol. Sin. 31, 718–724. doi: 10.1038/aps.2010.42
Tsb, S., Raes, J., and Bork, P. (2018). The human gut microbiome: from association to modulation. Cell 172:1198. doi: 10.1016/j.cell.2018.02.044
Tsilingiri, K., Barbosa, T., Penna, G., Caprioli, F., Sonzogni, A., Viale, G., et al. (2012). Probiotic and postbiotic activity in health and disease: comparison on a novel polarised ex-vivo organ culture model. Gut Microbes 61, 1007–1015. doi: 10.1136/gutjnl-2011-300971
Vissiennon, C., Nieber, K., Kelber, O., and Butterweck, V. (2012). Route of administration determines the anxiolytic activity of the flavonols kaempferol, quercetin and myricetin–are they prodrugs? J. Nutr. Biochem. 23, 733–740. doi: 10.1016/j.jnutbio.2011.03.017
Wallace, B. D., Wang, H., Lane, K. T., Scott, J. E., Orans, J., Koo, J. S., et al. (2010). Alleviating cancer drug toxicity by inhibiting a bacterial enzyme. Science 330, 831–835. doi: 10.1126/science.1191175
Wang, X., Zhang, A., Sun, H., Yan, G., Wang, P., and Han, Y. (2017). Traditional chinese medicine: current state, challenges, and applications. Serum Pharmacochem. Tradit. Chin. Med. 1–6. doi: 10.1016/B978-0-12-811147-5.00001-7
Wang, X. M., Lu, Y., Wu, L. Y., Yu, S. G., Zhao, B. X., Hu, H. Y., et al. (2012). Moxibustion inhibits interleukin-12 and tumor necrosis factor alpha and modulates intestinal flora in rat with ulcerative colitis. World J. Gastroenterol. 18, 6819–6828. doi: 10.3748/wjg.v18.i46.6819
Wen, J., Teng, B., Yang, P., Chen, X., Li, C., Jing, Y., et al. (2016). The potential mechanism of Bawei Xileisan in the treatment of dextran sulfate sodium-induced ulcerative colitis in mice. J. Ethnopharmacol. 188, 31–38. doi: 10.1016/j.jep.2016.04.054
Wozniak, M. A., Itzhaki, R. F., Shipley, S. J., and Dobson, C. B. (2007). Herpes simplex virus infection causes cellular β-amyloid accumulation and secretase upregulation. Neurosci. Lett. 429, 95–100. doi: 10.1016/j.neulet.2007.09.077
Wu, G., Zhang, C., Wang, J., Zhang, F., Wang, R., Shen, J., et al. (2016). Diminution of the gut resistome after a gut microbiota-targeted dietary intervention in obese children. Sci. Rep. 6:24030. doi: 10.1038/srep24030
Wu, T. R., Lin, C. S., Chang, C. J., Lin, T. L., Martel, J., Ko, Y. F., et al. (2019). Gut commensal Parabacteroides goldsteinii plays a predominant role in the anti-obesity effects of polysaccharides isolated from Hirsutella sinensis. Gut 68, 248–262. doi: 10.1136/gutjnl-2017-315458
Wu, X. M., and Tan, R. X. (2019). Interaction between gut microbiota and ethnomedicine constituents. Nat. Prod. Rep. 36, 788–809. doi: 10.1039/C8NP00041G
Xiaojiao, Z., Aihua, Z., Guoxiang, X., Yi, C., Linjing, Z., Houkai, L., et al. (2013). Melamine-induced renal toxicity is mediated by the gut microbiota. Sci. Transl. Med. 5:172ra122. doi: 10.1126/scitranslmed.3005114
Xin, Y., Pi, Z. F., Song, F. R., Liu, Z. Q., and Liu, S. Y. (2012). Study on the metabolic characteristics of aconite alkaloids in the extract of radix aconiti under intestinal bacteria of rat by uplc/msn technique. Chin. J. Chem. 30, 656–664. doi: 10.1002/cjoc.201100228
Xu, J., Chen, H. B., and Li, S. L. (2017). Understanding the molecular mechanisms of the interplay between herbal medicines and gut microbiota. Med. Res. Rev. 37, 1140–1185. doi: 10.1002/med.21431
Xu, J., Lian, F., Zhao, L., Zhao, Y., Chen, X., Zhang, X., et al. (2015). Structural modulation of gut microbiota during alleviation of type 2 diabetes with a Chinese herbal formula. ISME J. 9, 552–562. doi: 10.1038/ismej.2014.177
Xu, S., Dong, L., Shi, Y., Chen, L., Yuan, P., Wang, S., et al. (2016). The Novel Landscape of Long Non-coding RNAs in Response to Human Foamy Virus Infection Characterized by RNA-seq. Aids Res. Hum. Retroviruses 33, 452–464. doi: 10.1089/AID.2016.0156
Yang, C. Y., Tsai, S. Y., Chao, P. D. L., Yen, H. F., Chien, T. M., and Hsiu, S. L. (2002). Determination of hesperetin and its conjugate metabolites in serum and urine. J. Food Drug Anal. 10, 143–148.
Yang, H. L., Chen, S. C., Senthil Kumar, K. J., Yu, K. N., Lee Chao, P. D., Tsai, S. Y., et al. (2012). Antioxidant and anti-inflammatory potential of hesperetin metabolites obtained from hesperetin-administered rat serum: an ex vivo approach. J Agric. Food Chem. 60, 522. doi: 10.1021/jf2040675
Yin, X., Peng, J., Zhao, L., Yu, Y., Zhang, X., Liu, P., et al. (2013). Structural changes of gut microbiota in a rat non-alcoholic fatty liver disease model treated with a Chinese herbal formula. Syst. Appl. Microbiol. 36, 188–196. doi: 10.1016/j.syapm.2012.12.009
Yip, L. Y., Aw, C. C., Lee, S. H., Hong, Y. S., Ku, H. C., Xu, W. H., et al. (2018). The liver-gut microbiota axis modulates hepatotoxicity of tacrine in the rat. Hepatology 67, 282–295. doi: 10.1002/hep.29327
Yuan, L., Tang, P., Li, H. J., Hu, N., Zhong, X. Y., Lin, M., et al. (2019). Serum from Jiao-Tai-Wan treated rats increases glucose consumption by 3T3-L1 adipocytes through AMPK pathway signaling. Biosci. Rep. 39:BSR20181286. doi: 10.1042/BSR20181286
Zhong, H., Penders, J., Shi, Z., Ren, H., Cai, K., Fang, C., et al. (2019). Impact of early events and lifestyle on the gut microbiota and metabolic phenotypes in young school-age children. Microbiome 7:2. doi: 10.1186/s40168-018-0608-z
Zimmermann, M., Zimmermann-Kogadeeva, M., Wegmann, R., and Goodman, A. L. (2019a). Mapping human microbiome drug metabolism by gut bacteria and their genes. Nature 570, 462–467. doi: 10.1038/s41586-019-1291-3
Keywords: drug interventions, herbal medicines, traditional Chinese medicines, inter-individual differences, gut microbiota, metabolites
Citation: Wang Y, Qin S, Jia J, Huang L, Li F, Jin F, Ren Z and Wang Y (2019) Intestinal Microbiota-Associated Metabolites: Crucial Factors in the Effectiveness of Herbal Medicines and Diet Therapies. Front. Physiol. 10:1343. doi: 10.3389/fphys.2019.01343
Received: 12 February 2019; Accepted: 09 October 2019;
Published: 29 October 2019.
Edited by:
Liwei Xie, Guangdong Academy of Sciences, ChinaReviewed by:
Matthias J. Bahr, Sana Kliniken Lübeck, GermanySimona Bertoni, University of Parma, Italy
Copyright © 2019 Wang, Qin, Jia, Huang, Li, Jin, Ren and Wang. This is an open-access article distributed under the terms of the Creative Commons Attribution License (CC BY). The use, distribution or reproduction in other forums is permitted, provided the original author(s) and the copyright owner(s) are credited and that the original publication in this journal is cited, in accordance with accepted academic practice. No use, distribution or reproduction is permitted which does not comply with these terms.
*Correspondence: Fujun Jin, NDY0Njg5MzQxQHFxLmNvbQ==; Zhe Ren, cnpsNjIwMDBAcXEuY29t; Yifei Wang, dHdhbmcteWZAMTYzLmNvbQ==
†These authors have contributed equally to this work