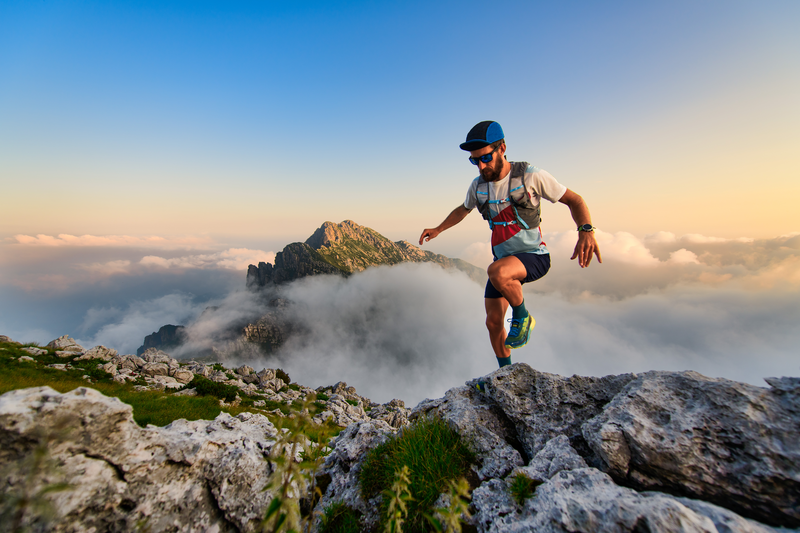
95% of researchers rate our articles as excellent or good
Learn more about the work of our research integrity team to safeguard the quality of each article we publish.
Find out more
ORIGINAL RESEARCH article
Front. Physiol. , 04 October 2019
Sec. Exercise Physiology
Volume 10 - 2019 | https://doi.org/10.3389/fphys.2019.01249
Background: Interleukin-6 (IL-6) is released from skeletal muscle during exercise and systemic IL-6 levels therefore increase acutely in response to a single bout of exercise. We recently showed that an acute increase in IL-6 delayed gastric emptying rate and improved postprandial glycemia. Here we investigate whether repeated increases in IL-6, induced by exercise training, influence gastric emptying rate and moreover if IL-6 is required for exercise-induced adaptations in glycemic control including secretion of glucagon and glucagon-like peptide-1 (GLP-1).
Methods: A total of 52 abdominally obese non-diabetic men and women were randomly assigned into four groups performing 12 weeks of endurance exercise or no exercise with or without IL-6 receptor blockade (tocilizumab). The primary endpoint was change in gastric emptying rate in response to the intervention and other endpoints included changes in glycemic control, glucagon, and GLP-1 secretion.
Results: There was no change in gastric emptying rate in any of the four groups following the intervention and comparing differences in change between groups also revealed no difference. Postprandial glucose remained unchanged in all groups but the exercise + tocilizumab group, which improved postprandial glucose in response to the intervention. The area under the curve for meal-stimulated glucagon, active and total GLP-1 increased in response to IL-6 receptor blockade, this effect was independent of exercise.
Conclusion: Exercise training and long-term IL-6 receptor blockade did not change gastric emptying rates in obese humans. IL-6 receptor blockade increased glucagon and GLP-1 secretion and implicate IL-6 in the regulation of the human alpha and L cells.
Gastric emptying is a critical regulator of postprandial glucose contributing to as much as 35% of peak glucose following a meal (Horowitz et al., 1993). Postprandial glucose levels are important for glycated hemoglobin (HbA1c) (Ceriello et al., 2004) emphasizing the relevance of in-depth understanding of the physiological regulators of gastric emptying.
Interleukin-6 (IL-6) is a cytokine influencing glucose homeostasis (Matthews et al., 2010). We recently showed that acutely increased IL-6, following an IL-6 infusion, delayed gastric emptying rate and improved postprandial glucose (Lehrskov et al., 2018). Consistent with these effects, IL-6 receptor blockade enhanced the rate of gastric emptying after an acute bout of exercise suggesting that IL-6 regulation of gastric emptying may be a physiological phenomenon (Lehrskov et al., 2018).
IL-6 is also a myokine released from skeletal muscle in response to exercise (Pedersen et al., 2001). During exercise, systemic IL-6 levels increase acutely and return to baseline within hours (Fischer, 2006). Exercise on a regular basis (training) is therefore associated with repeated increases in plasma IL-6. Whether exercise-induced IL-6 plays a role in the metabolic adaptations to exercise training is unclear. In some ways, IL-6 regulation of glucose metabolism bears resemblances to the effects of exercise on glucose metabolism. Infusion of IL-6 has shown beneficial effects of IL-6 on peripheral glucose uptake (Febbraio et al., 2004; Carey et al., 2006) and insulin stimulated glucose uptake (Carey et al., 2006). Whether repetitive increases in IL-6 induced by exercise training changes gastric emptying rate has not been investigated.
Most studies investigating exercise effects on gastric emptying have studied the acute effect of a single bout of exercise on gastric emptying during a post exercise meal (Horner et al., 2015b). A meta-analysis of these studies revealed a possible effect of exercise intensity on gastric emptying (Horner et al., 2015b). The mechanism behind this exercise intensity-dependent regulation of gastric emptying has not been identified.
The incretin hormone, glucagon-like peptide-1 (GLP-1) is secreted from the intestinal L cells in response to nutrients; however, GLP-1 has also been shown to increase during an acute exercise bout (Martins et al., 2007) and some studies have demonstrated an increased secretory capacity of the L cell following an exercise training intervention (Chanoine et al., 2008; Martins et al., 2010).
IL-6 stimulates GLP-1 secretion from L cells under certain circumstances in vitro (Ellingsgaard et al., 2011); and in rodents, exercise-induced GLP-1 was found to be dependent on IL-6 (Ellingsgaard et al., 2011). A link between training-induced adaptations of the L cell and IL-6 signaling has not been described in humans. The pancreatic alpha cell has been shown to be under the influence of IL-6, and in vitro and in vivo studies have shown that IL-6 stimulates glucagon secretion (Ellingsgaard et al., 2008; Tweedell et al., 2011; Fernández-Millán et al., 2013; Chow et al., 2014; Lehrskov et al., 2018). Whether long-term IL-6 receptor blockade influences glucagon secretion has not been demonstrated.
To investigate the role of IL-6 in training-induced adaptations of gastric emptying, glycemic control, and GLP-1 secretion, abdominally obese inactive individuals performed a 12-week exercise training intervention or no exercise training (control) in the presence and absence of the IL-6 receptor blockade (tocilizumab). We hypothesized that repetitive increases in IL-6 induced by exercise training would decelerate gastric emptying rate, and moreover that this effect would be abolished in the presence of IL-6 receptor blockade.
This study was a randomized exercise training intervention study with the overall primary endpoint to investigate the role of IL-6 in exercise training-induced regulation of visceral adipose tissue mass (Wedell-Neergaard et al., 2018). Data regarding visceral adipose tissue are published previously (Wedell-Neergaard et al., 2018). The study was performed between August 2016 and April 2018 at the Centre for Physical Activity Research (CFAS) in Copenhagen, Denmark. The study was approved by the ethical committee of the Capital Region of Denmark (H-16018062) and reported to the Danish Data Protection Agency (2012-58-0004). The study was registered at ClinicalTrials.gov NCT02901496 and was conducted in accordance with the guidelines for Good Clinical Practice and the Declaration of Helsinki. All participants provided written informed consent.
The full study protocol is published (Christensen et al., 2018). Briefly, participants were eligible if they were physically inactive (defined as less than 2.5 h of physical activity per week) (Tremblay et al., 2017) and abdominally obese (waist to height ratio ≥ 0.5 and/or waist circumference ≥ 88/102 cm for women/men, respectively) and age > 18 years old. Key exclusion criteria were diabetes (HbA1c ≥ 48 mmol/mol or fasting glucose ≥ 7.0 mmol/l), pregnancy or breastfeeding, ischemic heart disease, infectious or immunosuppressive disease, treatment with NSAID, treatment with biologic drugs for rheumatic diseases, systemic prednisolone, other immunotherapy or health conditions that prevented participation in the exercise intervention (e.g., severe obesity). Permitted medication should be taken at a stable dose for at least 4 weeks prior to randomization and preferably remain stable throughout the study period.
Data related to adverse events are published (Wedell-Neergaard et al., 2018).
Eligible participants were block-randomized (1:1:1:1:1) into five groups as described in the protocol paper (Christensen et al., 2018). The randomization sequence was generated by one researcher who did not participate in any clinical study procedures (KK) and concealed from all other researchers. The principal study investigators thus remained blinded to the training modality as well as the infusions (tocilizumab/saline). The five randomized groups were: no exercise + placebo, no exercise + tocilizumab, exercise + placebo, exercise + tocilizumab, or resistance training + placebo as described in the protocol paper (Christensen et al., 2018), all participants except those allocated to resistance training were included in the analyzes of this particular study (Figure 1).
The recombinant humanized monoclonal IL-6 receptor antibody tocilizumab (Roche, Basel, Switzerland) was reconstituted in saline and a total volume of 100 ml was infused monthly over 1 h at the maximal recommended dose of 8 mg/ml or a maximum of 800 mg (RoActemra | European Medicines Agency, n.d.). European Public Assessment Report for RoActemra. The concentration of tocilizumab following infusion of 8 mg/kg tocilizumab ranges from 10 to 183 μg/ml. Complete blockade is achieved at 4 μg/ml (Song and Yoshizaki, 2015). Given the half-life of tocilizumab of 13 days, it can be anticipated tocilizumab fully blocked IL-6 actions during the intervention period.
Before and after the intervention all participants went through several study visits including medical screening, blood sampling, mixed meal tolerance tests (MMTT), Dual X-ray Absorptiometry (DXA) scans, and maximal oxygen consumption rate (VO2 max) tests. Continuous glucose monitoring (CGM) and diet registration were performed over a 3-day period before and after the intervention. The intervention consisted of 12 weeks of supervised endurance exercise or no exercise combined with monthly infusion of tocilizumab. Saline was infused as placebo. Each exercise bout was 45 min high-intensity interval training and was performed on an ergometer bicycle. The intensity progressively increased over the 12-week training period. Safety and compliance were assessed every 4 weeks.
Dual X-ray Absorptiometry scans were used to assess changes in body composition (Lunar Prodigy Advance, GE Medical systems, Milwaukee, United States). VO2 max was determined during a bicycle ergometer test (Monark Ltd., Varberg, Sweden). After a 5 min warm-up at 70 w, the load was increased by 15 w every minute until exhaustion. Ventilation rate, oxygen uptake and expired carbon dioxide levels as well as heart rate were measured throughout the duration of the test (Quark b2, Cosmed, Rome, Italy). A test was valid if the respiratory exchange ratio > 1.1, a plateau in oxygen intake was reached or the heart rate was ±10 heartbeats from the estimated maximal heart rate (Edvardsen et al., 2014). The test result was used to determine the exercise workload for participants randomized to exercise and to assess changes in aerobic capacity in all four groups following the intervention.
To obtain insight into everyday glycemic control, a continuous glucose sensor (Enlite, Medtronic, Friedly, MN, United States) was inserted into the abdominal subcutaneous adipose tissue of all participants. The sensor was connected to a CGM monitor (iPro2, Medtronic) and calibrated four times daily with a glucometer (Contour, Bayer, Basel, Switzerland). The obtained data were analyzed for mean amplitude glycemic excursions (MAGE) a marker for glycemic variability (Service et al., 1970). Self-reported dietary intake was registered over 3 days before and after the intervention. Average daily calorie intake was calculated using madlog-vita.dk. Free-living physical activity was assessed using accelerometry (AX3, Newcastle, United Kingdom), sampling frequency 100 Hz, over 4 days before and after the intervention (Treuth et al., 2004).
The mixed meal was a 360 mL liquid meal containing 385 kcal. The energy distribution was 15% fat, 20% protein, and 65% carbohydrate. The meal contained 1.5 g paracetamol to allow assessment of gastric emptying rate (Heading et al., 1973). The meal was ingested over less than 2 min. To measure paracetamol concentrations and parameters of glycemic control blood samples were drawn regularly (−10, 0, 15, 30, 60, 90, 120, 150, and 180 min) relative to ingestion of the meal. To ensure that no acute effects of exercise impacted on the MMTT, exercise was not allowed 48 h prior to the test.
Baseline blood parameters, safety blood parameters, HbA1c, glucose, insulin, and paracetamol were measured at the Department of Clinical Biochemistry, Rigshospitalet, Copenhagen University Hospital. Plasma levels of total GLP-1 were determined using a radioimmunoassay developed in-house (codename 390) that is COOH-terminal and therefore measuring GLP-1 (7-36NH2 as well as the metabolite GLP-1 9-36NH2) as described previously (Orskov et al., 1994). Plasma levels of active GLP-1 was assessed with a commercially assay (Alpco, Salem, NH, United States). Plasma glucagon were measured using a C-terminal based radioimmunoassay (codename 4305) as described previously (Wewer Albrechtsen et al., 2014). Inter and intra assay variations for the GLP-1 and glucagon assays were less than 15 and 6%, respectively, and the lower limit of quantification was around 1 pmol/L with a precision of 1 pmol/L (Wewer Albrechtsen et al., 2015).
Whole body insulin sensitivity index was calculated by the Matsuda and DeFronzo method as follows (Matsuda and DeFronzo, 1999).
Whole body insulin sensitivity index
The insulinogenic index was calculated based on insulin and glucose levels after the meal load during the MMTT (Phillips et al., 1994; Wareham et al., 1995):
The oral disposition index was assessed during the first 30 min of the MMTT as Matsuda × the insulinogenic index (Solomon et al., 2014).
The main outcome was change in gastric emptying rate determined as changes in paracetamol kinetics following the intervention. Other outcomes included changes in postprandial plasma levels of glucose, insulin. Moreover, changes in postprandial plasma levels of glucagon, total and active GLP-1, as well as changes in whole body insulin sensitivity index, insulinogenic index, and the oral disposition index, were assessed as explorative outcomes. Also changes in HbA1c and glycemic variability were considered as explorative outcomes.
Results are expressed as mean ± SEM in figures and as mean ± SD in Table 1. Changes within groups and differences in change between groups are expressed as mean (95% CI).
The level of significance was set at p < 0.05. Statistical analyses were performed using GraphPad Prism version 7.02 (GraphPad Software, La Jolla, CA, United States). Within-group differences in plasma concentrations of paracetamol, glucose, insulin, glucagon, total GLP-1, and active GLP-1 were determined using the two-way repeated-measure analysis of variance (RM-ANOVA). Within-group differences in AUC were analyzed using Student’s paired t-test and differences in change between groups were assessed using the one-way ANOVA and Student’s unpaired t-test.
We enrolled 56 participants in the study. Due to protocol violations four participants were excluded from the data analysis. The remaining 52 participants were randomized to no exercise + placebo (n = 12), no exercise + tocilizumab (n = 13), exercise + placebo (n = 14), and exercise + tocilizumab (n = 13) and included in the per protocol analysis (Figure 1). In the CGM analysis another five participants were excluded due to incomplete availability of data. Data from the remaining 47 participants, distributed as follows: no exercise + placebo (n = 11), no exercise + tocilizumab (n = 12), exercise + placebo (n = 11), and exercise + tocilizumab (n = 13) were included in the CGM analysis. Participant characteristics and intervention-induced changes are shown in Table 1.
Data in Table 1 are published (Wedell-Neergaard et al., 2018) except for the changes in HbA1c, glycemic variability and parameters of postprandial glycemic control.
As anticipated, VO2 max increased in the exercise + placebo group and in the exercise + tocilizumab group. All participants in the two training groups complied with the minimum of 29 training sessions, and compliance was similar in the two groups. Body weight remained unchanged in all groups following the intervention (Table 1), and there were no differences in free-living physical activity and dietary intake between the groups. All data described in this section can be found in our previously published paper (Wedell-Neergaard et al., 2018).
As expected, baseline plasma levels of IL-6 increased in response to tocilizumab (Zhang et al., 2017). Exercise training for 12 weeks had no effect on baseline IL-6 levels. These data are previously reported (Wedell-Neergaard et al., 2018). IL-6 increased significantly in response to an acute exercise bout in the exercise + placebo group from 0.77 pg/ml (SD: 0.27) to 1.14 pg/ml (SD: 0.39) and from 10.23 pg/ml (SD: 5.25) to 11.33 pg/ml (SD: 5.18) in the exercise + tocilizumab group.
In contrast to our hypothesis there was no effect of exercise training, IL-6 receptor blockade, or the combination of the two, on the appearance of paracetamol into the circulation (Figure 2). Time to appearance, rate of appearance (Figure 2) and area under the curve (0–60 min) (Figure 2 and Table 2) remained unchanged in all four groups following the intervention and there was no difference in change between groups (Table 2). Hence, exercise training and long-term IL-6 receptor blockade did not affect gastric emptying in the present study.
Figure 2. Paracetamol and glucose. (A–D) Plasma paracetamol concentrations during a MMTT (left) and paracetamol AUC 0–60 min (right) before and after (A) no exercise + placebo, (B) no exercise + tocilizumab, (C) exercise + placebo, and (D) exercise + tocilizumab. (E–H) Plasma glucose concentrations during a MMTT (left) and blood glucose AUC before and after (E) no exercise + placebo, (F) no exercise + tocilizumab, (G) exercise + placebo, and (H) exercise + tocilizumab. Tocilizumab is an IL-6 receptor antibody. Data represent mean ± SEM. ∗p < 0.05 using Student’s paired t-test (right).
Postprandial glucose remained generally unaffected by training and IL-6 receptor blockade (Figure 2 and Table 2), however, in the exercise + tocilizumab group glucose AUC was significantly reduced following the intervention (Figure 2). Comparing differences in change in glucose AUC between groups showed no difference (Table 2).
The average glucose concentration, assessed by HbA1c, revealed only small and mostly non-significant changes following the intervention (Table 1). Consistent with the overall subtle changes in glycemic control, assessment of glycemic variability, determined as the MAGE, did not change in response to any of the interventions (Table 1). Fasting plasma glucose levels were within the normal range and less than 5.4 mM at baseline and remained unchanged in all groups following the intervention (Table 1).
To further elaborate on the adaptations related to glycemic control we assessed changes in plasma insulin, glucagon and GLP-1. Plasma levels of fasting and meal-stimulated insulin remained unchanged following the intervention (Tables 1, 2, and Figure 3). Modeling insulin sensitivity and insulin secretion using the Matsuda (Matsuda and DeFronzo, 1999) and insulinogenic index (Phillips et al., 1994; Wareham et al., 1995) also revealed no effect of the interventions (Table 1). In line with reduced postprandial glucose in the exercise + tocilizumab group, the oral disposition index increased in this group following the intervention (Table 1), however, comparing the changes in disposition index between groups showed no significant difference in change (Table 2).
Figure 3. Insulin and glucagon. (A–D) Plasma insulin concentrations during a mixed meal tolerance tests (MMTT) (left) and insulin AUC (right) before and after (A) no exercise + placebo, (B) no exercise + tocilizumab, (C) exercise + placebo, and (D) exercise + tocilizumab. (E–H) Plasma glucagon concentrations during a MMTT (left) and glucagon AUC (right) before and after (E) no exercise + placebo, (F) no exercise + tocilizumab, (G) exercise + placebo, and (H) exercise + tocilizumab. Tocilizumab is an IL-6 receptor antibody. Data represent mean ± SEM. ∗p < 0.05 using Student’s paired t-test (right).
Despite vague changes in glycemia and no changes in insulin secretion, a significant stimulatory effect of IL-6 receptor blockade on meal-induced glucagon secretion (AUC) was observed in both groups receiving tocilizumab (Figure 3). Comparing the changes in glucagon secretion between groups revealed no significant difference in change (Table 2).
The AUC for total and active GLP-1 increased in response to tocilizumab independently of exercise (Figure 4). Comparing changes in active and total GLP-1 between groups mostly revealed no significant difference in change (Table 2).
Figure 4. Total and active GLP-1. (A–D) Plasma concentrations of total GLP-1 during a MMTT (left) and total GLP-1 AUC (right) before and after (A) no exercise + placebo, (B) no exercise + tocilizumab, (C) exercise + placebo, and (D) exercise + tocilizumab. (E–H) Plasma concentrations of active GLP-1 during a MMTT (left) and active GLP-1 AUC (right) before and after (E) no exercise + placebo, (F) no exercise + tocilizumab, (G) exercise + placebo, and (H) exercise + tocilizumab. Tocilizumab is an IL-6 receptor antibody. Data represent mean ± SEM. ∗p < 0.05 using Student’s paired t-test (right).
Given that we previously observed a delay in gastric emptying following an acute increase in IL-6 (Lehrskov et al., 2018) we hypothesized that repetitive increases of IL-6, induced by exercise training, would also delay gastric emptying, but in contrast to our hypothesis exercise training had no effect on gastric emptying in this study. Our knowledge about the long-term effects of exercise training on gastric emptying is primarily based on cross-sectional data which suggests increased gastric emptying rate in trained individuals (Horner et al., 2011, 2015a). Studies investigating the effect of a single bout of exercise on gastric emptying during an immediate post exercise meal have demonstrated a role for exercise intensity, where increased exercise intensity is related to increased gastric emptying rate (Horner et al., 2015b). Nevertheless, our data showed no effect of a 12-week exercise-training period on gastric emptying in physically inactive and abdominally obese people. Whether the absence of an effect is related to exercise intensity or merely reflects that exercise training does not change gastric emptying remains unclear.
Gastric emptying also remained unchanged following long-term IL-6 receptor blockade. We have however, previously observed that the same dose of IL-6 receptor blockade increased gastric emptying during a post exercise meal (Lehrskov et al., 2018) suggesting that exercise-induced IL-6 delays gastric emptying. In the present study gastric emptying was assessed when IL-6 levels were at baseline and it is possible that tocilizumab regulates gastric emptying only when IL-6 levels are elevated, such as following an exercise bout. Given the pharmacokinetic profile of tocilizumab, we can anticipate that the IL-6 receptor blockade was complete during the post intervention assessments (Song and Yoshizaki, 2015). Increased plasma levels of IL-6 and reduced CRP levels support this. Training-induced adaptations of glycemic control were surprisingly mild, however, given that participants were normoglycemic at baseline prior to the training intervention the shortfall of improvements may be a reflection of a poor potential for change. One of the early adaptations to training is improved insulin sensitivity (Dela et al., 1992; Kirwan et al., 2009), yet modeling insulin sensitivity using the Matsuda index revealed no effect of training alone. Combining exercise training and IL-6 receptor blockade significantly reduced postprandial glucose excursions, which combined with no change in insulin resulted in a tendency toward improved Matsuda index and improved disposition index. Such an effect of long-term IL-6 blockade is in contrast to the insulin sensitizing effect observed in response to an infusion of IL-6 (Carey et al., 2006) and suggest that the improved insulin sensitivity may be secondary to IL-6 blockade.
That IL-6 receptor blockade increased glucagon secretion is quite contradictory to studies in rodent and human alpha cells (Ellingsgaard et al., 2008; Fernández-Millán et al., 2013) and to in vivo studies in rodents (Tweedell et al., 2011; Chow et al., 2014) and humans (Lehrskov et al., 2018) which all have demonstrated increased glucagon secretion in response to IL-6. However, while most of these studies evaluated the acute effect of increased IL-6, the present study investigated the consequence of long-term IL-6 receptor blockade. Thus, the stimulatory effect of tocilizumab on glucagon secretion may not reflect the inhibition of a direct effect of IL-6 on the alpha cell, but rather secondary adaptations of IL-6 receptor blockade. Participants receiving tocilizumab increased visceral adipose tissue mass (Wedell-Neergaard et al., 2018) and showed similar trends for whole body fat mass; therefore on a very speculative note, the augmented glucagon secretion could be secondary to steatosis (Suppli et al., 2016).
That both active and total GLP-1 levels were elevated in response to tocilizumab suggests an effect on the secretion of GLP-1 (Kuhre et al., 2015) rather than on a DPP-4 mediated modulation of GLP-1. Increased GLP-1 secretion in response to IL-6 receptor blockade may seem surprising given the stimulatory effect of IL-6 on GLP-1 secretion in GLUTag cells (Ellingsgaard et al., 2011). In humans, however, infusion of IL-6 had no effect on GLP-1 secretion, whereas infusion of IL-6 receptor blockade increased GLP-1 (Lehrskov et al., 2018). Thus, it seems that in humans both acute and long-term IL-6 receptor blockade increases meal-stimulated GLP-1 secretion. As for glucagon, it is unclear whether the effect of IL-6 receptor blockade on GLP-1 secretion is a consequence of inhibiting direct effects of IL-6 on L cells or a result of secondary adaptations to long term IL-6 blockade. Increased gastric emptying leads to increased GLP-1 secretion (Holst et al., 2016) which may explain the increased GLP-1 secretion following acute IL-6 receptor blockade (Lehrskov et al., 2018), long-term use of IL-6 receptor blockade did, however, not affect gastric emptying, suggesting that a different mechanism is involved in the regulation of GLP-1 secretion following long-term IL-6 receptor blockade.
Based on the available data there are no clear implications of the increased postprandial glucagon and GLP-1 secretion. Small numerical improvements in postprandial glucose were observed (significant in the exercise + tocilizumab group), but these were not associated with changes in insulin secretion or insulin sensitivity. Of note, both glucagon and GLP-1 levels remained unchanged in the fasting condition and only increased in response to the mixed meal, and whether these meal-associated changes have implications for long-term glycemic control remains unclear.
In conclusion, our study demonstrated that in obese and physically inactive people, gastric emptying rates are unaffected by exercise training and IL-6 receptor blockade. The study also showed that IL-6 receptor blockade increased glucagon and GLP-1 secretion and thus implicates IL-6 in the regulation of the human alpha and L cells.
Assessing gastric emptying using the paracetamol approach may be a limitation as it may not be a sensitive enough method to detect training-induced changes. Despite that participants were abdominally obese and physically inactive they were normoglycemic at baseline, this should be taken into account when evaluating the data on glycemic control. The power analysis of the study was based on changes in visceral adipose tissue mass (Christensen et al., 2018; Wedell-Neergaard et al., 2018) and not on changes in gastric emptying and glycemic control, it is therefore possibly that the secondary analyses were underpowered. Moreover, the sample size was relatively small and conclusions should be interpreted with caution.
The datasets generated for this study are available on request to the corresponding author.
The study was approved by the ethical committee of the Capital Region of Denmark (H-16018062) and reported to the Danish Data Protection Agency (2012-58-0004).
BP, HE, RK-M, KK, JR, MR-L, A-SW-N, RC, and LL conceived and designed the study. LL, RC, A-SW-N, GL, ED, ML, MH, NL, SF, SS, SN, MB, NV, and CD performed the experiments. LL, HE, NW, and JH analyzed and interpreted the data. BP primarily provided the resources. LL and HE wrote the original draft and visualized the data. All authors contributed to the writing, reviewing, and editing of the manuscript.
This study was funded by TrygFonden. The Centre for Physical Activity Research (CFAS) was supported by a grant from TrygFonden and the Centre of Inflammation and Metabolism (CIM) is a member of DD2 – the Danish Centre for Strategic Research in Type 2 Diabetes (the Danish Council for Strategic Research, Grant Nos. 09-067009 and 09-075724). Salary to RC was financed by a grant from the Danish Heart Foundation (16-R107-A6704-22970). Salary to LL was partly financed by a grant from Danish Diabetes Academy which was supported by the Novo Nordisk Foundation. Running costs were financially supported by Aase and Ejnar Danielsen Grant (10-001271). JH and NW were supported by a grant from the Novo Nordisk Foundation to the Center for Basic Metabolic Research. This study was also supported by the Novo Nordisk Foundation (NNF) Center for Basic Metabolic Research, University of Copenhagen, NNF Application Number: 13563, NNF Project support in Endocrinology and Metabolism – Nordic Region, NNF Application Number: 34250.
The authors declare that the research was conducted in the absence of any commercial or financial relationships that could be construed as a potential conflict of interest.
We acknowledge all the subjects for their participation. Moreover, we thank Lene Foged, Anne-Kirstine Lundby, Lene Albaek (University of Copenhagen, Denmark), Birgitte Egedie Sander Nielsen (Rigshospitalet, Denmark) for laboratory assistance.
Carey, A. L., Steinberg, G. R., Macaulay, S. L., Thomas, W. G., Holmes, A. G., Ramm, G., et al. (2006). Interleukin-6 increases insulin-stimulated glucose disposal in humans and glucose uptake and fatty acid oxidation in vitro via AMP-activated protein kinase. Diabetes Metab. Res. Rev. 55, 2688–2697.
Ceriello, A., Hanefeld, M., Leiter, L., Monnier, L., Moses, A., Owens, D., et al. (2004). Postprandial glucose regulation and diabetic complications. Arch. Intern. Med. 164, 2090–2095.
Chanoine, J.-P., Mackelvie, K. J., Barr, S. I., Wong, A. C., Meneilly, G. S., and Elahi, D. H. (2008). GLP-1 and appetite responses to a meal in lean and overweight adolescents following exercise. Obesity 16, 202–204. doi: 10.1038/oby.2007.39
Chow, S. Z., Speck, M., Yoganathan, P., Nackiewicz, D., Hansen, A. M., Ladefoged, M., et al. (2014). Glycoprotein 130 receptor signaling mediates alpha-cell dysfunction in a rodent model of type 2 diabetes. Diabetes Metab. Res. Rev. 63, 2984–2995. doi: 10.2337/db13-1121
Christensen, R. H., Wedell-Neergaard, A.-S., Lehrskov, L. L., Legård, G. E., Dorph, E. B., Nymand, S., et al. (2018). The role of exercise combined with tocilizumab in visceral and epicardial adipose tissue and gastric emptying rate in abdominally obese participants: protocol for a randomised controlled trial. Trials 19:266. doi: 10.1186/s13063-018-2637-0
Dela, F., Mikines, K. J., von Linstow, M., Secher, N. H., and Galbo, H. (1992). Effect of training on insulin-mediated glucose uptake in human muscle. Am. J. Physiol. 263, E1134–E1143. doi: 10.1152/ajpendo.2006.263.6.E1134
Edvardsen, E., Hem, E., and Anderssen, S. A. (2014). End criteria for reaching maximal oxygen uptake must be strict and adjusted to sex and age: a cross-sectional study. PLoS One 9:e85276. doi: 10.1371/journal.pone.0085276
Ellingsgaard, H., Ehses, J. A., Hammar, E. B., Van Lommel, L., Quintens, R., Martens, G., et al. (2008). Interleukin-6 regulates pancreatic alpha-cell mass expansion. Proc. Natl. Acad. Sci. U.S.A. 105, 13163–13168. doi: 10.1073/pnas.0801059105
Ellingsgaard, H., Hauselmann, I., Schuler, B., Habib, A. M., Baggio, L. L., Meier, D. T., et al. (2011). Interleukin-6 enhances insulin secretion by increasing glucagon-like peptide-1 secretion from L cells and alpha cells. Nat. Med. 17, 1481–1489. doi: 10.1038/nm.2513
Febbraio, M. A., Hiscock, N., Sacchetti, M., Fischer, C. P., and Pedersen, B. K. (2004). Interleukin-6 is a novel factor mediating glucose homeostasis during skeletal muscle contraction. Diabetes Metab. Res. Rev. 53, 1643–1648.
Fernández-Millán, E., de Toro-Martín, J., Lizárraga-Mollinedo, E., Escrivá, F., and Álvarez, C. (2013). Role of endogenous IL-6 in the neonatal expansion and functionality of Wistar rat pancreatic alpha cells. Diabetologia 56, 1098–1107. doi: 10.1007/s00125-013-2862-8
Fischer, C. P. (2006). Interleukin-6 in acute exercise and training: what is the biological relevance? Exerc. Immunol. Rev. 12, 6–33.
Heading, R. C., Nimmo, J., Prescott, L. F., and Tothill, P. (1973). The dependence of paracetamol absorption on the rate of gastric emptying. Br. J. Pharmacol. 47, 415–421.
Holst, J. J., Gribble, F., Horowitz, M., and Rayner, C. K. (2016). Roles of the gut in glucose homeostasis. Diabetes Care 39, 884–892. doi: 10.2337/dc16-0351
Horner, K. M., Byrne, N. M., Cleghorn, G. J., and King, N. A. (2015a). Influence of habitual physical activity on gastric emptying in healthy males and relationships with body composition and energy expenditure. Br. J. Nutr. 114, 489–496. doi: 10.1017/S0007114515002044
Horner, K. M., Schubert, M. M., Desbrow, B., Byrne, N. M., and King, N. A. (2015b). Acute exercise and gastric emptying: a meta-analysis and implications for appetite control. Sports Med. 45, 659–678. doi: 10.1007/s40279-014-0285-4
Horner, K. M., Byrne, N. M., Cleghorn, G. J., Näslund, E., and King, N. A. (2011). The effects of weight loss strategies on gastric emptying and appetite control. Obes. Rev. 12, 935–951. doi: 10.1111/j.1467-789X.2011.00901.x
Horowitz, M., Edelbroek, M. A., Wishart, J. M., and Straathof, J. W. (1993). Relationship between oral glucose tolerance and gastric emptying in normal healthy subjects. Diabetologia 36, 857–862.
Kirwan, J. P., Solomon, T. P. J., Wojta, D. M., Staten, M. A., Holloszy, J. O., Jp, K., et al. (2009). Effects of 7 days of exercise training on insulin sensitivity and responsiveness in type 2 diabetes mellitus. Am. J. Physiol. Endocrinol. Metab. 297, E151–E156. doi: 10.1152/ajpendo.00210.2009
Kuhre, R. E., Frost, C. R., Svendsen, B., and Holst, J. J. (2015). Molecular mechanisms of glucose-stimulated GLP-1 secretion from perfused rat small intestine. Diabetes Metab. Res. Rev. 64, 370–382.
Lehrskov, L. L., Lyngbaek, M. P., Soederlund, L., Legaard, G. E., Ehses, J. A., Heywood, S. E., et al. (2018). Interleukin-6 delays gastric emptying in humans with direct effects on glycemic control. Cell Metabol. 27, 1–11. doi: 10.1016/j.cmet.2018.04.008
Martins, C., Kulseng, B., King, N. A., Holst, J. J., and Blundell, J. E. (2010). The effects of exercise-induced weight loss on appetite-related peptides and motivation to eat. J. Clin. Endocrinol. Metab. 95, 1609–1616. doi: 10.1210/jc.2009-2082
Martins, C., Morgan, L. M., Bloom, S. R., and Robertson, M. D. (2007). Effects of exercise on gut peptides, energy intake and appetite. J. Endocrinol. 193, 251–258.
Matsuda, M., and DeFronzo, R. A. (1999). Insulin sensitivity indices obtained from oral glucose tolerance testing: comparison with the euglycemic insulin clamp. Diabetes Care 22, 1462–1470.
Matthews, V. B., Allen, T. L., Risis, S., Chan, M. H. S., Henstridge, D. C., Watson, N., et al. (2010). Interleukin-6-deficient mice develop hepatic inflammation and systemic insulin resistance. Diabetologia 53, 2431–2441. doi: 10.1007/s00125-010-1865-y
Orskov, C., Rabenhøj, L., Wettergren, A., Kofod, H., and Holst, J. J. (1994). Tissue and plasma concentrations of amidated and glycine-extended glucagon-like peptide I in humans. Diabetes Metab. Res. Rev. 43, 535–539.
Pedersen, B. K., Steensberg, A., and Schjerling, P. (2001). Exercise and interleukin-6. Curr. Opin. Hematol. 8, 137–141.
Phillips, D. I., Clark, P. M., Hales, C. N., and Osmond, C. (1994). Understanding oral glucose tolerance: comparison of glucose or insulin measurements during the oral glucose tolerance test with specific measurements of insulin resistance and insulin secretion. Diabet. Med. 11, 286–292.
RoActemra | European Medicines Agency, (n.d.) Available at: https://www.ema.europa.eu/en/medicines/human/EPAR/roactemra. (accessed July 19, 2019).
Service, F. J., Molnar, G. D., Rosevear, J. W., Ackerman, E., Gatewood, L. C., and Taylor, W. F. (1970). Mean amplitude of glycemic excursions, a measure of diabetic instability. Diabetes Metab. Res. Rev. 19, 644–655.
Solomon, T. P. J., Malin, S. K., Karstoft, K., Knudsen, S. H., Haus, J. M., Laye, M. J., et al. (2014). Determining pancreatic β-cell compensation for changing insulin sensitivity using an oral glucose tolerance test. Am. J. Physiol. Endocrinol. Metab. 307, E822–E829. doi: 10.1152/ajpendo.00269.2014
Song, S.-N. J., and Yoshizaki, K. (2015). Tocilizumab for treating rheumatoid arthritis: an evaluation of pharmacokinetics/pharmacodynamics and clinical efficacy. Expert Opin. Drug Metab. Toxicol. 11, 307–316. doi: 10.1517/17425255.2015.992779
Suppli, M. P., Lund, A., Bagger, J. I., Vilsbøll, T., and Knop, F. K. (2016). Involvement of steatosis-induced glucagon resistance in hyperglucagonaemia. Med. Hypotheses 86, 100–103. doi: 10.1016/j.mehy.2015.10.029
Tremblay, M. S., Aubert, S., Barnes, J. D., Saunders, T. J., Carson, V., Latimer-Cheung, A. E., et al. (2017). Sedentary behavior research network (SBRN) - terminology consensus project process and outcome. Int. J. Behav. Nutr. Phys. Act. 14:75. doi: 10.1186/s12966-017-0525-8
Treuth, M. S., Schmitz, K., Catellier, D. J., McMurray, R. G., Murray, D. M., Almeida, M. J., et al. (2004). Defining accelerometer thresholds for activity intensities in adolescent girls. Med. Sci. Sports Exerc. 36, 1259–1266.
Tweedell, A., Mulligan, K. X., Martel, J. E., Chueh, F.-Y., Santomango, T., and McGuinness, O. P. (2011). Metabolic response to endotoxin in vivo in the conscious mouse: role of interleukin-6. Metabolism 60, 92–98. doi: 10.1016/j.metabol.2009.12.022
Wareham, N. J., Phillips, D. I., Byrne, C. D., and Hales, C. N. (1995). The 30 minute insulin incremental response in an oral glucose tolerance test as a measure of insulin secretion. Diabet. Med. 12:931.
Wedell-Neergaard, A.-S., Lang Lehrskov, L., Christensen, R. H., Legaard, G. E., Dorph, E., Larsen, M. K., et al. (2018). Exercise-induced changes in visceral adipose tissue mass are regulated by IL-6 signaling: a randomized controlled trial. Cell Metabol. 29, 1–12. doi: 10.1016/j.cmet.2018.12.007
Wewer Albrechtsen, N. J., Bak, M. J., Hartmann, B., Christensen, L. W., Kuhre, R. E., Deacon, C. F., et al. (2015). Stability of glucagon-like peptide 1 and glucagon in human plasma. Endocr. Connect. 4, 50–57. doi: 10.1530/EC-14-0126
Wewer Albrechtsen, N. J., Hartmann, B., Veedfald, S., Windeløv, J. A., Plamboeck, A., Bojsen-Møller, K. N., et al. (2014). Hyperglucagonaemia analysed by glucagon sandwich ELISA: nonspecific interference or truly elevated levels? Diabetologia 57, 1919–1926. doi: 10.1007/s00125-014-3283-z
Keywords: interleukin-6, gastric emptying, exercise, tocilizumab, glucagon, GLP-1
Citation: Lang Lehrskov L, Christensen RH, Wedell-Neergaard A-S, Legaard GE, Dorph E, Larsen MK, Henneberg M, Launbo N, Fagerlind SR, Seide SK, Nymand S, Ball M, Vinum N, Dahl C, Wewer Albrechtsen NJ, Holst JJ, Ried-Larsen M, Rosenmeier JB, Krogh-Madsen R, Karstoft K, Pedersen BK and Ellingsgaard H (2019) Effects of Exercise Training and IL-6 Receptor Blockade on Gastric Emptying and GLP-1 Secretion in Obese Humans: Secondary Analyses From a Double Blind Randomized Clinical Trial. Front. Physiol. 10:1249. doi: 10.3389/fphys.2019.01249
Received: 19 March 2019; Accepted: 12 September 2019;
Published: 04 October 2019.
Edited by:
Brian Keith McFarlin, University of North Texas, United StatesReviewed by:
Audrey J. Stone, The University of Texas at Austin, United StatesCopyright © 2019 Lang Lehrskov, Christensen, Wedell-Neergaard, Legaard, Dorph, Larsen, Henneberg, Launbo, Fagerlind, Seide, Nymand, Ball, Vinum, Dahl, Wewer Albrechtsen, Holst, Ried-Larsen, Rosenmeier, Krogh-Madsen, Karstoft, Pedersen and Ellingsgaard. This is an open-access article distributed under the terms of the Creative Commons Attribution License (CC BY). The use, distribution or reproduction in other forums is permitted, provided the original author(s) and the copyright owner(s) are credited and that the original publication in this journal is cited, in accordance with accepted academic practice. No use, distribution or reproduction is permitted which does not comply with these terms.
*Correspondence: Louise Lang Lehrskov, bG91aXNlLmxhbmcubGVocnNrb3YuMDFAcmVnaW9uaC5kaw==
†These authors have contributed equally to this work
Disclaimer: All claims expressed in this article are solely those of the authors and do not necessarily represent those of their affiliated organizations, or those of the publisher, the editors and the reviewers. Any product that may be evaluated in this article or claim that may be made by its manufacturer is not guaranteed or endorsed by the publisher.
Research integrity at Frontiers
Learn more about the work of our research integrity team to safeguard the quality of each article we publish.