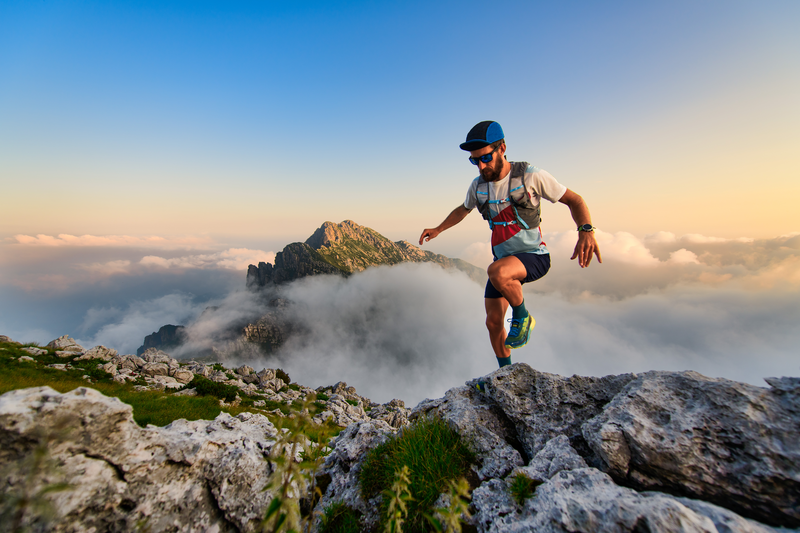
95% of researchers rate our articles as excellent or good
Learn more about the work of our research integrity team to safeguard the quality of each article we publish.
Find out more
MINI REVIEW article
Front. Physiol. , 10 July 2019
Sec. Integrative Physiology
Volume 10 - 2019 | https://doi.org/10.3389/fphys.2019.00859
Smoking and physical inactivity are important preventable causes of disability and early death worldwide. Reduced exercise tolerance has been described in smokers, even in those who do not fulfill the extant physiological criteria for chronic obstructive pulmonary disease (COPD) and are not particularly sedentary. In this context, it is widely accepted that exercise capacity depends on complex cardio-pulmonary interactions which support oxygen (O2) delivery to muscle mitochondria. Although peripheral muscular factors, O2 transport disturbances (including the effects of increased carboxyhemoglobin) and autonomic nervous system unbalance have been emphasized, other derangements have been more recently described, including early microscopic emphysema, pulmonary microvascular disease, ventilatory and gas exchange inefficiency, and left ventricular diastolic dysfunction. Using an integrative physiological approach, the present review summarizes the recent advances in knowledge on the effects of smoking on the lung-heart-muscle axis under the stress of exercise. Special attention is given to the mechanisms connecting physiological abnormalities such as early cardio-pulmonary derangements, inadequate oxygen delivery and utilization, and generalized bioenergetic disturbances at the muscular level with the negative sensations (sense of heightened muscle effort and breathlessness) that may decrease the tolerance of smokers to physical exercise. A deeper understanding of the systemic effects of smoking in subjects who did not (yet) show evidences of COPD and ischemic heart disease – two devastating smoking related diseases – might prove instrumental to fight their ever-growing burden.
“So marked is the effect of tobacco in relaxing the whole of the muscular system, that before the days of chloroform it was employed in surgical operation, in which it was necessary that the muscles should be perfectly cleaned.”
Sir Morell Mackenzie, in: The Tobacco Habit. Its History and Pathology. Herbert H. Tidswell, London, J&H Churchil, 1912.
Cigarette smoking, the most important preventable cause of death worldwide, is strongly associated with the poor quality of life and health-care resources utilization. (World Health Organization, 2011) Physical inactivity, a common finding in smokers, has also been mechanistically linked to a plethora of nontransmissible diseases (World Health Organization, 2010; Lee et al., 2012). There is, therefore, increasing awareness of the link between exercise intolerance and smoking (Clini et al., 2016); moreover, the last decades witnessed a growing debate on the consequences of preclinical chronic obstructive pulmonary disease (COPD) – the prototype of a smoking-related disease – on clinical outcomes, including exercise intolerance (Caram et al., 2016; Chen et al., 2016; Rhee et al., 2017; Soriano et al., 2018).
In this context, reduced maximal and submaximal exercise tolerance and breathlessness on daily life (modified Medical Research Council dyspnea score, ≥2) have been described in a subgroup of smokers, even when they do not fulfill the extant physiological criteria for COPD (Klein et al., 1992; Misigoj-Durakovic et al., 2012; Liu et al., 2015; Regan et al., 2015; Elbehairy et al., 2016, 2017a; Woodruff et al., 2016; Di Marco et al., 2017; Martinez et al., 2017; Walter Barbosa et al., 2017; Fuertes et al., 2018) and they are not particularly sedentary (Misigoj-Durakovic et al., 2012; Fuertes et al., 2018). It is widely accepted that exercise intolerance is the final result of abnormalities in the complex interaction between large systems (mainly pulmonary and cardiocirculatory) which support O2 delivery to muscle mitochondria (Burtscher, 2013; Gabriel and Zierath, 2017). In smokers, these abnormalities have been mainly ascribed to peripheral muscular factors (Wüst et al., 2008a,c; Degens et al., 2015; Al-Bashaireh et al., 2018; Nogueira et al., 2018). Oxygen (O2) transport disturbances [including high blood carboxy-hemoglobin levels (HbCO); Huie, 1996; Maehara et al., 1997; Bye et al., 2008; Barn et al., 2018] and, potentially, cardiocirculatory abnormalities (Gidding et al., 1995; Lauer et al., 1997; Bernaards et al., 2003; Kobayashi et al., 2004; Tello et al., 2005; Papathanasiou et al., 2007), and autonomic nervous system unbalance (Kotamäki, 1995; Mendonca et al., 2011; Ide and Tabira, 2013). Of note, influential reviews on the topic (Huie, 1996; Heishman et al., 2010) did not consider the modulatory effects of abnormalities that only recently have gained more attention, including early emphysema (Sashidhar et al., 2002; Fain et al., 2006; Grydeland et al., 2009; Harris et al., 2012; Mohamed Hoesein et al., 2014; Regan et al., 2015; Alcaide et al., 2017; Crossley et al., 2018), pulmonary microvascular disease (Nana-Sinkam et al., 2007; Schweitzer et al., 2011; Harris et al., 2012; Estépar et al., 2013; Schmekel et al., 2013; Iyer et al., 2016; Rizzi et al., 2016; Aaron et al., 2017, 2018; Saruya et al., 2017), ventilatory inefficiency, (Gläser et al., 2011; Elbehairy et al., 2016; Walter Barbosa et al., 2017), gas exchange abnormalities (Gläser et al., 2010, 2011, 2013; Elbehairy et al., 2015), and left ventricular diastolic dysfunction (Tello et al., 2005; Payne et al., 2006; Gulel et al., 2007; Yilmaz et al., 2007; Bennet et al., 2010; Talukder et al., 2011; Leary, 2016; Nadruz et al., 2016).
As smoking duration is the main trigger for symptoms and risk for COPD, (Liu et al., 2015; Bhatt et al., 2018), it is conceivable that there is a continuum from reduced maximal exercise capacity at a young age toward exercise intolerance in middle-age and older smokers. In fact, some smokers do experience a larger-than-expected (by aging) decrease in maximal O2 consumption (V′O2max) (0.2–0.5 mL × min−1 × kg−1/year) (Burtscher, 2013; Roman et al., 2016; Rodrigues et al., 2018), an effect that might be, at least in part, genetically determined (Ross et al., 2018). Thus, there is growing evidence in favor of derangements in the muscle-lung-heart axis (Bye et al., 2008; Grydeland et al., 2009; Gläser et al., 2013; Degens et al., 2015; Regan et al., 2015; Leary, 2016; Alcaide et al., 2017; Saruya et al., 2017; Walter Barbosa et al., 2017; Barn et al., 2018) that may have sensory consequences and contribute to poor exercise tolerance in ever-smokers or former smokers without COPD (Figure 1, above). Overall, these derangements are followed by clinical traits increasingly described in the medical literature (Figure 1, below).
Figure 1. Potential mechanisms underlying exercise (in)tolerance in symptomatic smokers and comparative main clinical abnormalities. Above, key respiratory, cardiocirculatory, and peripheral muscular (including mithocondrial) effects of chronic cigarette smoking are highlighted. Ventilation (V)/perfusion (Q) mismatch, in particular, may increase the ventilatory demand to exercise (left graph, reprinted with permission of the American Thoracic Society. Annals ATS. Vol. 14, No. Supplement_1, Jul 01, 2017) which, in association, with other source of ventilatory stimuli (and potential psychological/behavioral traits) increases exertional dyspnea at a given work rate (right graph, reproduced with permission of the ©ERS (2019): Elbehairy et al., 2016). Below, main characteristic clinical abnormalities of symptomatic (ex)smokers compared with asymptomatic smokers, healthy nonsmokers, COPD GOLD 1 and COPD GOLD 2. See text for further elaboration. PFT, pulmonary function tests; AO, airways obstruction; GOLD, global initiative for obstructive lung disease; COPD, chronic obstructive pulmonary disease; CO, carbon monoxide; DLco, lung diffusion capacity for CO; ATP, adenosine triphosphate; LVH, left ventricular hypertrophy; LVDD, left ventricular diastolic dysfunction; N/A, not applicable; mMRC, modified Medical Research Council; CAT, COPD Assessment Test; NE, not evaluated; CHF, chronic heart failure; SGRQ, St George’s Respiratory Questionnaire; V′O2peak, peak oxygen consumption; *p < 0.05 at similar work-rate.
The present review aims to succinctly summarize the recent advances in our knowledge on the effects of smoking on the muscle-lung-heart axis under the stress of exercise. Despite the fact that there is an acute-on-chronic effect of current smoking on these interactions, we will refrain from discussing the large body of clinical and experimental evidence showing the deleterious effects of acute tobacco smoking in nicotine-naive subjects (Johnston et al., 2018). Thus, we will focus on the chronic consequences of smoking on exercise intolerance from an integrative physiological perspective, giving special attention to the ancillary effects of aging, and physical inactivity.
Despite its relevance to exercise intolerance in subjects with COPD (Aliverti and Macklem, 2008; Debigaré and Maltais, 2008), there is a lack of in-depth discussion about the effects of smoking on the “muscle-mitochondria compartment” as a potential limiting factor in non-COPD smokers. In any case, reduced muscle strength and/or mass have been described in smokers (Montes de Oca et al., 2008; Wüst et al., 2008b, 2009; Kok et al., 2012; Rom et al., 2012; Degens et al., 2015); of note, two meta-analyses suggested an independent effect (from physical inactivity) of cigarette smoking on reducing muscle mass (Rom et al., 2012; Steffl et al., 2015). Conversely, chronic sympathetic nerve over-excitation induced by nicotine may counterbalance the potential deleterious effects of smoking on muscle mass (Mündel and Jones, 2006). Muscle wasting after chronic tobacco exposure in some smokers might be related to increased ubiquitin-mediated proteolysis (Petersen et al., 2007; Liu et al., 2011; Rom et al., 2012). In addition, smoking may inhibit anabolic pathways and protein synthesis in the quadriceps (Degens et al., 2015; Madani et al., 2018) (as extensively reviewed in Degens et al., 2015). Current and former experimental data support changes in muscle fiber endotype toward a less oxidative profile (Orlander et al., 1979; Montes de Oca et al., 2008; Krüger et al., 2015, 2018). However, reduced muscle capillarization remain questionable (Montes de Oca et al., 2008; Wüst et al., 2008b; Nogueira et al., 2018). At the subcellular level, mitochondrial DNA might be damaged in smokers without COPD (Fetterman et al., 2017).
These structural changes might negatively impact on muscle bioenergetics and metabolism. Spillover of inflammatory mediators produced by lung epithelial cells in response to smoking may reach the striated muscles with negative bioenergetic consequences (Fetterman et al., 2017; Madani et al., 2018). The mitochondrion, as important source of biochemical and thermal energy, is a key target for smoking toxicity, leading to reduced respiration, decreased ATP content, and increased production of free radicals in a dose- and time-dependent manner (Neves et al., 2016; Fetterman et al., 2017; Madani et al., 2018). As a consequence, smokers may present with impaired oxidative phosphorylation (as extensively reviewed by Fetterman et al., 2017). Other metabolic derangements with a potential to impact on physical performance include: An appreciable (10%) increase in energy expenditure at rest compared to nonsmoking subjects (Hofstetter et al., 1986), impaired sarcoplasmic reticulum Ca++ uptake in myofibres (Nogueira et al., 2018), and impaired insulin-dependent glycogen recovery from exercise (Jensen et al., 1995). An early lactate threshold might be the final consequence of these bioenergetic derangements in association with chronically low levels of muscle activation, i.e., sedentarism (Miyatake et al., 2011; Lauria et al., 2017).
However, only a few studies showed reduced peripheral muscle strength (Kok et al., 2012) or reduced fatigue resistance to electrical stimulation under controlled conditions in smokers compared with nonsmokers with similar physical (in)activity scores (Wüst et al., 2008b,c). In fact, this is a major confounder as several studies failed to show lower scores of peripheral muscle fatigue (Orlander et al., 1979; Larsson and Orlander, 1984) or force generation (Wüst et al., 2008b,c) in smokers when compared to controls paired by self-reported physical activity. Diminished resistance to fatigue in smokers was demonstrated using effort-independent techniques, such as electrically evoked muscle contractions (Wüst et al., 2008c) and CO inhalation (Morse et al., 2008). Interestingly, however, nicotine may also have ergogenic effects through augmented release of adrenaline and enhanced performance of fast-twitch muscle fibers (Johnston et al., 2018). Thus, any potential increase in muscle fatigability in smokers might be compensated by the central excitatory actions of nicotine leading to preserved time to task failure compared to equally sedentary controls (Orlander et al., 1979; Larsson and Orlander, 1984).
Large population-based studies found subtle cardiac structure and function abnormalities, which could be mechanistically related to smoking (Gidding et al., 1995; Lauer et al., 1997; Bernaards et al., 2003; Payne et al., 2006; Nadruz et al., 2016); of note, some of these studies suggested increased left ventricular mass and chronotropic incompetence during exercise (Gidding et al., 1995; Lauer et al., 1997; Payne et al., 2006). Key abnormalities found in chronic smokers include increased autonomic activity (Kotamäki, 1995; Lauer et al., 1997; Mendonca et al., 2011; Ide and Tabira, 2013), elevated catecholamine levels (Laustiola et al., 1988; Kotamäki, 1995; Chelland Campbell et al., 2008), acute and chronic rest systemic arterial hypertension, largely related with arterial stiffness and secondary to tobacco induced endothelial dysfunction (Scallan et al., 2010), altered exercise heart rate-systolic blood pressure product (Papathanasiou et al., 2007), left ventricular diastolic dysfunction (Tello et al., 2005; Payne et al., 2006; Gulel et al., 2007; Yilmaz et al., 2007; Bennet et al., 2010; Leary, 2016; Nadruz et al., 2016), and direct myocardial depression due to CO in heavy smokers (Bye et al., 2008). Of note, despite the fact that exposure to tobacco smoke is a strong risk factor for pulmonary hypertension and chronic thromboembolic disease, (Schiess et al., 2010; Weissmann et al., 2012; Keusch et al., 2014), there is a lack of studies addressing potential abnormalities in pulmonary vascular conductance during exercise in symptomatic non-COPD smokers. Isolated left ventricular diastolic dysfunction was previously associated with reduced exercise capacity in some populations (Genovesi-Ebert et al., 1994; Barmeyer et al., 2009; Grewal et al., 2009). However, there was only a weak relationship between left ventricular dysfunction and tolerance to stress exercise testing in smokers without COPD (Grewal et al., 2009). Of note, we could not confirm these findings in patients with COPD (Muller et al., 2018). Downregulation of β-adrenoceptors (Laustiola et al., 1988) and blunted heart rate during exercise are described maladaptations to chronic smoking (Lauer et al., 1997). Conversely, resting heart rate is commonly increased, likely due to the combined effects of: the pharmacological action of nicotine (Turner and McNicol, 1993), increased circulating levels of catecholamine (Laustiola et al., 1988; Kotamäki, 1995; Chelland Campbell et al., 2008), modulatory effects on baroreflex function (Bernaards et al., 2003; Papathanasiou et al., 2007; Mendonca et al., 2011) and chronic reduction in the vagal drive (Mendonca et al., 2011). Thus, resting tachycardia, in association with myocardium stiffness (Gidding et al., 1995) and diastolic dysfunction, (Tello et al., 2005; Payne et al., 2006; Gulel et al., 2007; Yilmaz et al., 2007; Talukder et al., 2011) may critically interfere with the ideal diastolic time-pressure product necessary to optimize left ventricular filling (Fisher, 2014). In fact, O2-pulse – a surrogate for stroke volume under certain conditions – was found lower during submaximal exercise in smokers compared to nonsmoker controls (Kobayashi et al., 2004). In addition, Kimura et al. (2007) using near-infrared spectroscopy showed increased O2 extraction at the right vastus lateralis during incremental exercise testing in the majority of “healthy” smokers compared to nonsmokers. This is in line with potential decrements in muscle O2 delivery caused by central derangements.
Muscle hyperemia on exercise due to microcirculatory adaptations is highly dependent on shear stress to induce nitric oxide (NO) release, i.e., endothelium-dependent vascular relaxation (Green et al., 2017). Smoking-induced oxidative stress is a trigger for a generalized vascular inflammation (Golbidi et al., 2018; Madani et al., 2018), the latter being associated with: lower expression of endothelial NO synthetase, increased expression of TNF-α, IL-6, and IL-1β (Golbidi et al., 2018), downregulation of IL-10 (Allam et al., 2013), increased adhesion of inflammatory cells stimulated by ICAM-1 and IL-8 (Madani et al., 2018) and, ultimately, disruption of endothelial integrity as a protective barrier (Golbidi et al., 2018). These abnormalities may impair the endothelium-dependent hyperemic response to exercise (Barua et al., 2002) and increase arterial vascular resistance (Degens et al., 2015). It is noteworthy that impairment in endothelium-dependent hyperemia has been associated with lower exercise tolerance in smokers (Heffernan et al., 2010; Montero, 2015).
In addition to these cardiocirculatory abnormalities, muscle O2 delivery on exercise may be impaired due to the deleterious consequences of increased (HbCO) as CO has a ~ 250 higher affinity to Hb compared to O2 (Maehara et al., 1997; Kimura et al., 2007; Keramidas et al., 2012). Smokers may show up to 9% HbCO leading to decrements in O2 content similar to those found in hypoxemic patients (Degens et al., 2015). High levels (two to three times normal range) can persist up to 90 min after smoking (Jarvis et al., 1987). Accordingly, several animal- and human-based studies demonstrated the deleterious effects of HbCO on submaximal (Maehara et al., 1997; Keramidas et al., 2012) and maximal exercise capacity (Vogel and Gleser, 1972; Aronow and Cassidy, 1975; Aronow et al., 1977; Klausen et al., 1983). These findings should be tempered with others which failed to show alterations in endurance (Turner and McNicol, 1993; Ryan et al., 2016). These discrepancies might be linked to the large inter-study variability on CO exposure or individual differences in CO clearance (Zavorsky et al., 2012). Of note, exercise on room air accelerates CO elimination compared to resting and moderate exercise is as effective as breathing 100% O2 at rest on this regard (Zavorsky et al., 2012). The deleterious consequences of high (HbCO) might be particularly important in the presence of comorbidities: low-dose inhaled CO (Aronow, 1976) and nicotine patch in substitution to smoking (Mahmarian et al., 1997) have been implicated in lower exercise capacity seen in smokers with ischemic heart disease.
Spirometrically occult airways and lung parenchymal disease, pulmonary microvascular disease, gas exchange, and respiratory muscle abnormalities could potentially contribute to decrease exercise tolerance due to exertional dyspnea in symptomatic smokers (Hamari et al., 2010; Estépar et al., 2013; Rennard and Drummond, 2015; Elbehairy et al., 2016; Woodruff et al., 2016; Bodduluri et al., 2017; Martinez et al., 2017; Bostanci et al., 2019). Airway disease with chronic bronchitic symptoms is largely recognized in smokers without COPD (Rennard and Drummond, 2015; Woodruff et al., 2016; Martinez et al., 2017). It is conceivable that dysfunction in cystic fibrosis transmembrane conductance regulator (CFTR) might be mechanistically involved in the chronic bronchitis seen in some smokers without COPD, thereby leading to a clinical phenotype similar to mild cystic fibrosis (Raju et al., 2016). There is limited evidence that these symptoms might be related to reduced daily physical activity, independent of age and sex (Woodruff et al., 2016). In a large observational study (SPIROMICS) (Martinez et al., 2017), imaging evidence of initial airway disease, more frequent exacerbations, and poorer exercise tolerance were found in symptomatic current or former smokers with normal pulmonary function compared to nonsmokers and asymptomatic smokers with airflow limitation. Of note, about 50% of smokers without airway obstruction have symptoms such as dyspnea (Regan et al., 2015). Symptomatic smokers with dyspnea and preserved lung function may present with abnormally increased airway wall thickening on high-resolution computerized tomography scans (HRCT), suggesting early involvement of the small airways (Regan et al., 2015; Woodruff et al., 2016). Interestingly, although airway wall thickening decreases with higher age, smokers maintain higher airway wall thickening throughout aging (Telenga et al., 2017). In fact, increased closure volume of the small airways and high peripheral airway resistance by impulse oscillometry might be seen in smokers with dyspnea on exertion (Di Marco et al., 2017). Incipient/mild emphysema can also be found in heavy smokers with preserved spirometry (Regan et al., 2015), being occasionally associated with poor exercise tolerance and increased self-reported activity limitation on daily life (Regan et al., 2015; Alcaide et al., 2017). Kirby et al. (2013) and Pike et al. (2015) using advanced magnetic resonance imaging (MRI) identified substantial ventilation inhomogeneity in ex-smokers without airflow limitation; of note, this was spatially coincident with incipient/mild emphysema demonstrated on CT.
How those abnormalities could be mechanistically linked to activity-related dyspnea in smokers? Heightened awareness of increased efferent activity from bulbopontine and cortical motor centers to the inspiratory muscles are closely linked to exertional dyspnea (Ward et al., 2005). Elbehairy et al. (2016) found that higher fractional inspiratory neural drive to the diaphragm in smokers without COPD was secondary to compensatory increases in inspiratory diaphragm electromyographic activity to overcome increased airways resistance and lower maximal activation (Figure 2). Severe leg discomfort also contributed to exercise intolerance in this study: peripheral muscle weakness (Degens et al., 2015), greater motor command output (Ward et al., 2005; Elbehairy et al., 2016), and high perceived effort (relative to maximum) (Furlanetto et al., 2014) could be mechanistically involved in these findings (see also Peripheral Muscular Abnormalities section). Also, there are limited data pointing out for attenuated peripheral metaboreflex in non-COPD smokers (Drew et al., 2012). Of note, no study to date has specifically investigated whether symptomatic smokers without COPD may present with impaired respiratory muscle metaboreflex. If this is experimentally demonstrated, such results would provide the basis for additional studies exploring the hypothesis of blood flow redistribution from the locomotor muscles to the overburden respiratory muscles in these subjects (Oliveira et al., 2015).
Figure 2. Diaphragm electromyography (EMGdi, A and C), transdiaphragmatic pressure (Pdi, B,D and E), and oesophageal pressure (Poes, F) are shown during incremental cycle exercise in smokers without chronic obstructive pulmonary disease (COPD) and age-matched healthy controls. Dynamic maximal measurements during inspiratory capacity (IC) manoeuvres are also shown. (Reproduced with permission of the ©ERS 2019: Elbehairy et al., 2016). Data are presented as mean ± SEM. *p < 0.05 smokers without COPD versus healthy controls at rest, at standardized work rates or at peak exercise. Insp, inspiratory; Exp, expiratory.
It is also plausible that increased chemo-stimulation as a result of higher physiological dead space (increased V′E/V′CO2, ventilatory inefficiency) contributes to a higher inspiratory neural drive during tidal breathing in some smokers (Elbehairy et al., 2016; Weatherald et al., 2018). Increased V′E/V′CO2, likely reflecting high VD/VT, was found in smokers with only mild spirometric abnormalities (Elbehairy et al., 2017a,b) and smokers with low lung diffusion capacity for CO (Walter Barbosa et al., 2017). Importantly, a large populational study found that, after careful control for confounders, chronic cigarette smoking was associated with increased alveolar-arterial gradient and dead space on exercise (Gläser et al., 2010, 2013). Despite the absence of overt hypoxemia, there is evidence that smokers without COPD may present with large carotid bodies (Cramer et al., 2014; Tan et al., 2019), potentially increasing peripheral chemosensitivity and the inordinary ventilatory response to exercise found in some smokers. In any case, high dead space might reflect enlarged areas of increased ventilation/perfusion relationship independent of emphysema, i.e., early microvascular disease (Harris et al., 2012; Estépar et al., 2013; Saruya et al., 2017). Another piece of indirect evidence suggesting early pulmonary microvascular disease is the common finding of out-of-proportion decrease in DLco relative to macroscopic emphysema burden in symptomatic smokers (Kirby et al., 2013). It is also noteworthy that, at least in smoking rodent models, pulmonary vascular changes with neomuscularization of precapillary arteries may precede the development of emphysema (Ferrer et al., 2009, 2011) – as proposed by Liebow six decades ago (Liebow, 1959). Moreover, significant remodeling of the pulmonary arteries has been observed in heavy smokers (Santos et al., 2002). Smoking has been associated with endothelial (Schweitzer et al., 2011; Schmekel et al., 2013) and epithelial damage (Madani et al., 2018): cigarette smoke products may cause pulmonary vascular remodeling through either a direct effect on endothelial cells or an inflammatory mechanism (Barua et al., 2002; Allam et al., 2013; Madani et al., 2018). Indeed, elevated amounts of circulating endothelial microparticles were found in smokers (Badrnya et al., 2014; Liu et al., 2014; Mobarrez et al., 2014).
Clinically, there is growing evidence that a subset of non-COPD smokers present with imaging evidence of microvascular pruning or constriction (Iyer et al., 2016; Saruya et al., 2016) and functional abnormalities consistent with the areas of increased ventilation-perfusion relationship (Gläser et al., 2013; Rizzi et al., 2016; Bodduluri et al., 2017). Interestingly, ventilatory inefficiency has been associated with impaired flow-mediated dilation in smokers, supporting a generalized vasculopathy (Gläser et al., 2011). Impaired ability in recruiting pulmonary vessels during exercise has been demonstrated in light smokers (Rizzi et al., 2016) or second-hand smokers (Arjomandi et al., 2012). Moreover, a large population-based study showed the presence of pulmonary artery enlargement on HRCT in smokers without COPD (Lindenmaier et al., 2016). Overall, compensatory increases in minute ventilation are likely useful to maintain alveolar ventilation and arterial blood gas homeostasis in symptomatic smokers but this might hasten dynamic mechanical constraints thereby contributing to dyspnea and exercise intolerance. (Elbehairy et al., 2016; Di Marco et al., 2017) These physiological considerations should be tempered with the observation that smokers have two to four times more panic-depression and anxiety disturbances compared to controls (Zvolensky et al., 2004; Moylan et al., 2012; Fadda et al., 2013). These abnormalities are associated with a chaotic breathing pattern and hyperventilation sindromes (Bokov et al., 2016; Bansal et al., 2018), and accordingly, contemporary models of fatigue point out to complex interactions between physiological activity and psychological state (Gruet, 2018). Hence, such complex “coordinated deadaptation”(Burtscher, 2013) in symptomatic smokers might lead to perceived fatigability (sensations about fatigue) and performance fatigability (incapacity of the neuromuscular system to meet the requirements of a given task) (Enoka and Duchateau, 2016). Thus, objective and subjective mechanisms may dynamically interact and prompt early exercise cessation in susceptible smokers.
Finally, there is limited evidence that some smokers may present with reduced inspiratory muscle strength (Formiga et al., 2018; Bostanci et al., 2019) and endurance though this is not a universal finding (Elbehairy et al., 2016). Owing to exquisitive sensitivity of the diaphragm to hypoxia (Zhu et al., 2005; Lewis and O’Halloran, 2016), low-grade inflammation (Haegens et al., 2012), and oxidative stress (Lawler and Powers, 1998; Barreiro et al., 2006), it remains possible that it might suffer the consequences of chronic smoking. During exercise, O2 delivery to the respiratory muscles might be impaired in some smokers – similarly to what has been demonstrated in the peripheral muscles in non-COPD smokers – at very high levels of ventilation (Kimura et al., 2007). In the above-mentioned study by Elbehairy et al. (2016), the authors found that the rib cage and accessory muscles contributed to a greater extent to meet a heightened ventilatory response to exercise in symptomatic smokers. This might constitute a useful strategy to spare a mechanically stressed diaphragm. In view of the experimental data supporting diaphragm wasting secondary to tobacco exposure, (Carlos et al., 2014; Vieira Ramos et al., 2018) increased ventilatory demands during exercise might overload the diaphragm, thereby contributing to exertional dyspnea.
Multiple interrelated mechanisms may decrease the ability of smokers without COPD to face the challenges brought by physical exercise. In fact, the stress of exercise constitutes a physiologically elegant – and clinically relevant – model to expose the deleterious effects of oxidative stress, pro-inflammatory status, sustained high-circulating nicotine levels, low O2 content, and high carbon monoxide on human health well before they are apparent at rest. Although physical inactivity and, potentially, specific psychological traits are major confounders, there seems to exist a subset of smokers who are particularly intolerant to exertion. Complex and yet poorly understood interactions among cardiopulmonary and muscular abnormalities might underlie this specific phenotype of “symptomatic smokers on exertion.” A deeper understanding of the systemic effects of smoking in subjects who did not (yet) show evidences of devastating smoking related diseases, such as COPD and ischemic heart disease, might prove instrumental to fight their ever-growing burden.
All authors listed have made a substantial, direct and intellectual contribution to the work, and approved it for publication.
This study was supported by Graduate Program in Movement Sciences and Graduate Program on Health and Development in West Central Region at the Federal University of Mato Grosso do Sul (Brazil). This study was financed in part by the National Council for Scientific and Technological Development (CNPq) and Coordenação de Aperfeiçoamento de Pessoal de Nível Superior – Brazil (CAPES) – Finance Code 001.
The authors declare that the research was conducted in the absence of any commercial or financial relationships that could be construed as a potential conflict of interest.
Aaron, C. P., Hoffman, E. A., Lima, J. A. C., Kawut, S. M., Bertoni, A. G., Vogel-Claussen, J., et al. (2017). Pulmonary vascular volume, impaired left ventricular filling and dyspnea: the MESA Lung Study. PLoS One 12:e0176180. doi: 10.1371/journal.pone.0176180
Aaron, C. P., Schwartz, J. E., Hoffman, E. A., Angelini, E., Austin, J. H. M., Cushman, M., et al. (2018). A longitudinal cohort study of aspirin use and progression of emphysema-like lung characteristics on CT imaging: the MESA lung study. Chest 154, 41–50. doi: 10.1016/j.chest.2017.11.031
Al-Bashaireh, A. M., Haddad, L. G., Weaver, M., Kelly, D. L., Chengguo, X., and Yoon, S. (2018). The effect of tobacco smoking on musculoskeletal health: a systematic review. J. Environ. Public Health 2018:4184190. doi: 10.1155/2018/4184190
Alcaide, A. B., Sanchez-Salcedo, P., Bastarrika, G., Campo, A., Berto, J., Ocon, M. D., et al. (2017). Clinical features of smokers with radiological emphysema but without airway limitation. Chest 151, 358–365. doi: 10.1016/j.chest.2016.10.044
Aliverti, A., and Macklem, P. T. (2008). The major limitation to exercise performance in COPD is inadequate energy supply to the respiratory and locomotor muscles. J. Appl. Physiol. 105, 749–751. doi: 10.1152/japplphysiol.90336.2008
Allam, E., Delacruz, K., Ghoneima, A., Sun, J., and Windsor, L. J. (2013). Effects of tobacco on cytokine expression from human endothelial cells. Oral Dis. 19, 660–665. doi: 10.1111/odi.12050
Arjomandi, M., Haight, T., Sadeghi, N., Redberg, R., and Gold, W. M. (2012). Reduced exercise tolerance and pulmonary capillary recruitment with remote secondhand smoke exposure. PLoS One 7:e34393. doi: 10.1371/journal.pone.0034393
Aronow, W. S. (1976). Effect of cigarette smoking and of carbon monoxide on coronary heart disease. Chest 70, 514–518. doi: 10.1378/chest.70.4.514
Aronow, W. S., and Cassidy, J. (1975). Effect of carbon monoxide on maximal treadmill exercise. A study in normal persons. Ann. Intern. Med. 83, 496–499.
Aronow, W. S., Ferlinz, J., and Glauser, F. (1977). Effect of carbon monoxide on exercise performance in chronic obstructive pulmonary disease. Am. J. Med. 63, 904–908. doi: 10.1016/0002-9343(77)90544-7
Badrnya, S., Baumgartner, R., and Assinger, A. (2014). Smoking alters circulating plasma microvesicle pattern and microRNA signatures. Thromb. Haemost. 112, 128–136. doi: 10.1160/TH13-11-0977
Bansal, T., Haji, G. S., Rossiter, H. B., Polkey, M. I., and Hull, J. H. (2018). Exercise ventilatory irregularity can be quantified by approximate entropy to detect breathing pattern disorder. Respir. Physiol. Neurobiol. 255, 1–6. doi: 10.1016/j.resp.2018.05.002
Barmeyer, A., Müllerleile, K., Mortensen, K., and Meinertz, T. (2009). Diastolic dysfunction in exercise and its role for exercise capacity. Heart Fail. Rev. 14, 125–134. doi: 10.1007/s10741-008-9105-y
Barn, P., Giles, L., Héroux, M. E., and Kosatsky, T. (2018). A review of the experimental evidence on the toxicokinetics of carbon monoxide: the potential role of pathophysiology among susceptible groups. Environ. Health 17:13. doi: 10.1186/s12940-018-0357-2
Barreiro, E., Gáldiz, J. B., Mariñán, M., Alvarez, F. J., Hussain, S. N., and Gea, J. (2006). Respiratory loading intensity and diaphragm oxidative stress: N-acetyl-cysteine effects. J. Appl. Physiol. 100, 555–563. doi: 10.1152/japplphysiol.00780.2005
Barua, R. S., Ambrose, J. A., Eales-Reynolds, L. J., DeVoe, M. C., Zervas, J. G., and Saha, D. C. (2002). Heavy and light cigarette smokers have similar dysfunction of endothelial vasoregulatory activity: an in vivo and in vitro correlation. J. Am. Coll. Cardiol. 39, 1758–1763. doi: 10.1016/S0735-1097(02)01859-4
Bennet, L., Larsson, C., Söderström, M., Råstam, L., and Lindblad, U. (2010). Diastolic dysfunction is associated with sedentary leisure time physical activity and smoking in females only. Scand. J. Prim. Health Care 28, 172–178. doi: 10.3109/02813432.2010.506803
Bernaards, C. M., Twisk, J. W., Van Mechelen, W., Snel, J., and Kemper, H. C. (2003). A longitudinal study on smoking in relationship to fitness and heart rate response. Med. Sci. Sports Exerc. 35, 793–800. doi: 10.1249/01.MSS.0000064955.31005.E0
Bhatt, S. P., Kim, Y. I., Harrington, K. F., Hokanson, J. E., Lutz, S. M., Cho, M. H., et al. (2018). Smoking duration alone provides stronger risk estimates of chronic obstructive pulmonary disease than pack-years. Thorax 73, 414–421. doi: 10.1136/thoraxjnl-2017-210722
Bodduluri, S., Reinhardt, J. M., Hoffman, E. A., Newell, J. D., Nath, H., Dransfield, M. T., et al. (2017). Signs of gas trapping in normal lung density regions in smokers. Am. J. Respir. Crit. Care Med. 196, 1404–1410. doi: 10.1164/rccm.201705-0855OC
Bokov, P., Fiamma, M. N., Chevalier-Bidaud, B., Chenivesse, C., Straus, C., Similowski, T., et al. (2016). Increased ventilatory variability and complexity in patients with hyperventilation disorder. J. Appl. Physiol. 120, 1165–1172. doi: 10.1152/japplphysiol.00859.2015
Bostanci, Ö., Mayda, H., Yılmaz, C., Kabadayı, M., Yılmaz, A. K., and Özdal, M. (2019). Inspiratory muscle training improves pulmonary functions and respiratory muscle strength in healthy male smokers. Respir. Physiol. Neurobiol. 264, 28–32. doi: 10.1016/j.resp.2019.04.001
Burtscher, M. (2013). Exercise limitations by the oxygen delivery and utilization systems in aging and disease: coordinated adaptation and deadaptation of the lung-heart muscle axis - a mini-review. Gerontology 59, 289–296. doi: 10.1159/000343990
Bye, A., Sørhaug, S., Ceci, M., Høydal, M. A., Stølen, T., Heinrich, G., et al. (2008). Carbon monoxide levels experienced by heavy smokers impair aerobic capacity and cardiac contractility and induce pathological hypertrophy. Inhal. Toxicol. 20, 635–646. doi: 10.1080/08958370701883821
Caram, L. M., Ferrari, R., Bertani, A. L., Garcia, T., Mesquita, C. B., Knaut, C., et al. (2016). Smoking and early COPD as independent predictors of body composition, exercise capacity, and health status. PLoS One 11:e0164290. doi: 10.1371/journal.pone.0164290
Carlos, S. P., Dias, A. S., Forgiarini Júnior, L. A., Patricio, P. D., Graciano, T., Nesi, R. T., et al. (2014). Oxidative damage induced by cigarette smoke exposure in mice: impact on lung tissue and diaphragm muscle. J. Bras. Pneumol. 40, 411–420. doi: 10.1590/S1806-37132014000400009
Chelland Campbell, S., Moffatt, R. J., and Stamford, B. A. (2008). Smoking and smoking cessation -- the relationship between cardiovascular disease and lipoprotein metabolism: a review. Atherosclerosis 201, 225–235. doi: 10.1016/j.atherosclerosis.2008.04.046
Chen, C., Jian, W., Gao, Y., Xie, Y., Song, Y., and Zheng, J. (2016). Early COPD patients with lung hyperinflation associated with poorer lung function but better bronchodilator responsiveness. Int. J. Chron. Obstruct. Pulmon. Dis. 11, 2519–2526. doi: 10.2147/COPD.S110021
Clini, E. M., Beghé, B., and Fabbri, L. M. (2016). What is the origin of dyspnoea in smokers without airway disease? Eur. Respir. J. 48, 604–607. doi: 10.1183/13993003.01170-2016
Cramer, J. A., Wiggins, R. H., Fudim, M., Engelman, Z. J., Sobotka, P. A., and Shah, L. M. (2014). Carotid body size on CTA: correlation with comorbidities. Clin. Radiol. 69, e33–e36. doi: 10.1016/j.crad.2013.08.016
Crossley, D., Renton, M., Khan, M., Low, E. V., and Turner, A. M. (2018). CT densitometry in emphysema: a systematic review of its clinical utility. Int. J. Chron. Obstruct. Pulmon. Dis. 13, 547–563. doi: 10.2147/COPD.S143066
Debigaré, R., and Maltais, F. (2008). The major limitation to exercise performance in COPD is lower limb muscle dysfunction. J. Appl. Physiol. 105, 751–753. discussion 755-757. doi: 10.1152/japplphysiol.90336.2008a
Degens, H., Gayan-Ramirez, G., and van Hees, H. W. (2015). Smoking-induced skeletal muscle dysfunction: from evidence to mechanisms. Am. J. Respir. Crit. Care Med. 191, 620–625. doi: 10.1164/rccm.201410-1830PP
Di Marco, F., Terraneo, S., Job, S., Rinaldo, R. F., Sferrazza Papa, G. F., Roggi, M. A., et al. (2017). Cardiopulmonary exercise testing and second-line pulmonary function tests to detect obstructive pattern in symptomatic smokers with borderline spirometry. Respir. Med. 127, 7–13. doi: 10.1016/j.rmed.2017.04.006
Drew, R., Muller, M. D., Cui, J., Blaha, C., Mast, J., and Sinoway, L. I. (2012). FASEB J. 26, 1087.10–1087.10.
Elbehairy, A. F., Ciavaglia, C. E., Webb, K. A., Guenette, J. A., Jensen, D., Mourad, S. M., et al. (2015). Pulmonary gas exchange abnormalities in mild chronic obstructive pulmonary disease. Implications for dyspnea and exercise intolerance. Am. J. Respir. Crit. Care Med. 191, 1384–1394. doi: 10.1164/rccm.201501-0157OC
Elbehairy, A. F., Faisal, A., Guenette, J. A., Jensen, D., Webb, K. A., Ahmed, R., et al. (2017a). Resting physiological correlates of reduced exercise capacity in smokers with mild airway obstruction. COPD 14, 267–275. doi: 10.1080/15412555.2017.1281901
Elbehairy, A. F., Guenette, J. A., Faisal, A., Ciavaglia, C. E., Webb, K. A., Jensen, D., et al. (2016). Mechanisms of exertional dyspnoea in symptomatic smokers without COPD. Eur. Respir. J. 48, 694–705. doi: 10.1183/13993003.00077-2016
Elbehairy, A. F., Parraga, G., Webb, K. A., Neder, J. A., and O’Donnell, D. E., Canadian Respiratory Research Network (CRRN) (2017b). Mild chronic obstructive pulmonary disease: why spirometry is not sufficient! Expert Rev. Respir. Med. 11, 549–563. doi: 10.1080/17476348.2017.1334553
Enoka, R. M., and Duchateau, J. (2016). Translating Fatigue to Human Performance. Med. Sci. Sports Exerc. 48, 2228–2238. doi: 10.1249/MSS.0000000000000929
Estépar, R. S., Kinney, G. L., Black-Shinn, J. L., Bowler, R. P., Kindlmann, G. L., Ross, J. C., et al. (2013). Computed tomographic measures of pulmonary vascular morphology in smokers and their clinical implications. Am. J. Respir. Crit. Care Med. 188, 231–239. doi: 10.1164/rccm.201301-0162OC
Fadda, E., Galimberti, E., Cammino, S., and Bellodi, L. (2013). Smoking, physical activity and respiratory irregularities in patients with panic disorder. Riv. Psichiatr. 48, 293–300. doi: 10.1708/1319.14625
Fain, S. B., Panth, S. R., Evans, M. D., Wentland, A. L., Holmes, J. H., Korosec, F. R., et al. (2006). Early emphysematous changes in asymptomatic smokers: detection with 3He MR imaging. Radiology 239, 875–883. doi: 10.1148/radiol.2393050111
Ferrer, E., Peinado, V. I., Castañeda, J., Prieto-Lloret, J., Olea, E., González-Martín, M., et al. (2011). Effects of cigarette smoke and hypoxia on pulmonary circulation in the guinea pig. Eur. Respir. J. 38, 617–627. doi: 10.1183/09031936.00105110
Ferrer, E., Peinado, V. I., Díez, M., Carrasco, J. L., Musri, M. M., Martínez, A., et al. (2009). Effects of cigarette smoke on endothelial function of pulmonary arteries in the guinea pig. Respir. Res. 10:76. doi: 10.1186/1465-9921-10-76
Fetterman, J. L., Sammy, M. J., and Ballinger, S. W. (2017). Mitochondrial toxicity of tobacco smoke and air pollution. Toxicology 391, 18–33. doi: 10.1016/j.tox.2017.08.002
Fisher, J. P. (2014). Autonomic control of the heart during exercise in humans: role of skeletal muscle afferents. Exp. Physiol. 99, 300–305. doi: 10.1113/expphysiol.2013.074377
Formiga, M. F., Campos, M. A., and Cahalin, L. P. (2018). Inspiratory muscle performance of former smokers and nonsmokers using the test of incremental respiratory endurance. Respir. Care 63, 86–91. doi: 10.4187/respcare.05716
Fuertes, E., Carsin, A. E., Antó, J. M., Bono, R., Corsico, A. G., Demoly, P., et al. (2018). Leisure-time vigorous physical activity is associated with better lung function: the prospective ECRHS study. Thorax 73, 376–384. doi: 10.1136/thoraxjnl-2017-210947
Furlanetto, K. C., Mantoani, L. C., Bisca, G., Morita, A. A., Zabatiero, J., Proença, M., et al. (2014). Reduction of physical activity in daily life and its determinants in smokers without airflow obstruction. Respirology 19, 369–375. doi: 10.1111/resp.12236
Gabriel, B. M., and Zierath, J. R. (2017). The limits of exercise physiology: from performance to health. Cell Metab. 25, 1000–1011. doi: 10.1016/j.cmet.2017.04.018
Genovesi-Ebert, A., Marabotti, C., Palombo, C., Giaconi, S., Rossi, G., and Ghione, S. (1994). Echo Doppler diastolic function and exercise tolerance. Int. J. Cardiol. 43, 67–73. doi: 10.1016/0167-5273(94)90092-2
Gidding, S. S., Xie, X., Liu, K., Manolio, T., Flack, J. M., and Gardin, J. M. (1995). Cardiac function in smokers and nonsmokers: the CARDIA study. The coronary artery risk development in young adults study. J. Am. Coll. Cardiol. 26, 211–216.
Gläser, S., Ittermann, T., Koch, B., Schäper, C., Felix, S. B., Völzke, H., et al. (2013). Influence of smoking and obesity on alveolar-arterial gas pressure differences and dead space ventilation at rest and peak exercise in healthy men and women. Respir. Med. 107, 919–926. doi: 10.1016/j.rmed.2013.02.013
Gläser, S., Koch, B., Ittermann, T., Schäper, C., Dörr, M., Felix, S. B., et al. (2010). Influence of age, sex, body size, smoking, and beta blockade on key gas exchange exercise parameters in an adult population. Eur. J. Cardiovasc. Prev. Rehabil. 17, 469–476. doi: 10.1097/HJR.0b013e328336a124
Gläser, S., Obst, A., Opitz, C. F., Dörr, M., Felix, S. B., Empen, K., et al. (2011). Peripheral endothelial dysfunction is associated with gas exchange inefficiency in smokers. Respir. Res. 12:53. doi: 10.1186/1465-9921-12-53
Golbidi, S., Edvinsson, L., and Laher, I. (2018). Smoking and endothelial dysfunction. Curr. Vasc. Pharmacol. 16, 1–10. doi: 10.2174/1573403X14666180913120015
Green, D. J., Hopman, M. T., Padilla, J., Laughlin, M. H., and Thijssen, D. H. (2017). Vascular adaptation to exercise in humans: role of hemodynamic stimuli. Physiol. Rev. 97, 495–528. doi: 10.1152/physrev.00014.2016
Grewal, J., McCully, R. B., Kane, G. C., Lam, C., and Pellikka, P. A. (2009). Left ventricular function and exercise capacity. JAMA 301, 286–294. doi: 10.1001/jama.2008.1022
Gruet, M. (2018). Fatigue in chronic respiratory diseases: theoretical framework and implications for real-life performance and rehabilitation. Front. Physiol. 9:1285. doi: 10.3389/fphys.2018.01285
Grydeland, T. B., Dirksen, A., Coxson, H. O., Pillai, S. G., Sharma, S., Eide, G. E., et al. (2009). Quantitative computed tomography: emphysema and airway wall thickness by sex, age and smoking. Eur. Respir. J. 34, 858–865. doi: 10.1183/09031936.00167908
Gulel, O., Soylu, K., Yazici, M., Demircan, S., Durna, K., and Sahin, M. (2007). Longitudinal diastolic myocardial functions are affected by chronic smoking in young healthy people: a study of color tissue Doppler imaging. Echocardiography 24, 494–498. doi: 10.1111/j.1540-8175.2007.00421.x
Haegens, A., Schols, A. M., Gorissen, S. H., van Essen, A. L., Snepvangers, F., Gray, D. A., et al. (2012). NF-κB activation and polyubiquitin conjugation are required for pulmonary inflammation-induced diaphragm atrophy. Am. J. Physiol. Lung Cell. Mol. Physiol. 302, L103–L110. doi: 10.1152/ajplung.00084.2011
Hamari, A., Toljamo, T., Nieminen, P., and Kinnula, V. L. (2010). High frequency of chronic cough and sputum production with lowered exercise capacity in young smokers. Ann. Med. 42, 512–520. doi: 10.3109/07853890.2010.505933
Harris, B., Klein, R., Jerosch-Herold, M., Hoffman, E. A., Ahmed, F. S., Jacobs, D. R., et al. (2012). The association of systemic microvascular changes with lung function and lung density: a cross-sectional study. PLoS One 7:e50224. doi: 10.1371/journal.pone.0050224
Heffernan, K. S., Karas, R. H., Patvardhan, E. A., and Kuvin, J. T. (2010). Endothelium-dependent vasodilation is associated with exercise capacity in smokers and non-smokers. Vasc. Med. 15, 119–125. doi: 10.1177/1358863X09358750
Heishman, S. J., Kleykamp, B. A., and Singleton, E. G. (2010). Meta-analysis of the acute effects of nicotine and smoking on human performance. Psychopharmacology 210, 453–469. doi: 10.1007/s00213-010-1848-1
Hofstetter, A., Schutz, Y., Jéquier, E., and Wahren, J. (1986). Increased 24-hour energy expenditure in cigarette smokers. N. Engl. J. Med. 314, 79–82. doi: 10.1056/NEJM198601093140204
Huie, M. J. (1996). The effects of smoking on exercise performance. Sports Med. 22, 355–359. doi: 10.2165/00007256-199622060-00003
Ide, H., and Tabira, K. (2013). Changes in sympathetic nervous system activity in male smokers after moderate-intensity exercise. Respir. Care 58, 1892–1898. doi: 10.4187/respcare.02240
Iyer, K. S., Newell, J. D., Jin, D., Fuld, M. K., Saha, P. K., Hansdottir, S., et al. (2016). Quantitative dual-energy computed tomography supports a vascular etiology of smoking-induced inflammatory lung disease. Am. J. Respir. Crit. Care Med. 193, 652–661. doi: 10.1164/rccm.201506-1196OC
Jarvis, M. J., Tunstall-Pedoe, H., Feyerabend, C., Vesey, C., and Saloojee, Y. (1987). Comparison of tests used to distinguish smokers from nonsmokers. Am. J. Public Health 77, 1435–1438. doi: 10.2105/AJPH.77.11.1435
Jensen, E. X., Fusch, C., Jaeger, P., Peheim, E., and Horber, F. F. (1995). Impact of chronic cigarette smoking on body composition and fuel metabolism. J. Clin. Endocrinol. Metab. 80, 2181–2185. doi: 10.1210/jcem.80.7.7608276
Johnston, R., Doma, K., and Crowe, M. (2018). Nicotine effects on exercise performance and physiological responses in nicotine-naïve individuals: a systematic review. Clin. Physiol. Funct. Imaging 38, 527–538. doi: 10.1111/cpf.12443
Keramidas, M. E., Kounalakis, S. N., Eiken, O., and Mekjavic, I. B. (2012). Carbon monoxide exposure during exercise performance: muscle and cerebral oxygenation. Acta Physiol. 204, 544–554. doi: 10.1111/j.1748-1716.2011.02363.x
Keusch, S., Hildenbrand, F. F., Bollmann, T., Hallank, M., Held, M., Keiser, R., et al. (2014). Tobacco smoke exposure in pulmonary arterial and thromboembolic pulmonary hypertension. Respiration 88, 38–45. doi: 10.1159/000359972
Kimura, Y., Nakamoto, Y., Shitama, H., Ohmine, S., Ide, M., and Hachisuka, K. (2007). Influence of moderate smoking on physical fitness and local muscle oxygenation profile during incremental exercise. J. UOEH 29, 149–158. doi: 10.7888/juoeh.29.149
Kirby, M., Owrangi, A., Svenningsen, S., Wheatley, A., Coxson, H. O., Paterson, N. A., et al. (2013). On the role of abnormal DL(CO) in ex-smokers without airflow limitation: symptoms, exercise capacity and hyperpolarised helium-3 MRI. Thorax 68, 752–759. doi: 10.1136/thoraxjnl-2012-203108
Klausen, K., Andersen, C., and Nandrup, S. (1983). Acute effects of cigarette smoking and inhalation of carbon monoxide during maximal exercise. Eur. J. Appl. Physiol. Occup. Physiol. 51, 371–379. doi: 10.1007/BF00429074
Klein, J. S., Gamsu, G., Webb, W. R., Golden, J. A., and Müller, N. L. (1992). High-resolution CT diagnosis of emphysema in symptomatic patients with normal chest radiographs and isolated low diffusing capacity. Radiology 182, 817–821. doi: 10.1148/radiology.182.3.1535900
Kobayashi, Y., Takeuchi, T., Hosoi, T., and Loeppky, J. A. (2004). Effects of habitual smoking on cardiorespiratory responses to sub-maximal exercise. J. Physiol. Anthropol.Appl. Human Sci. 23, 163–169. doi: 10.2114/jpa.23.163
Kok, M. O., Hoekstra, T., and Twisk, J. W. (2012). The longitudinal relation between smoking and muscle strength in healthy adults. Eur. Addict. Res. 18, 70–75. doi: 10.1159/000333600
Kotamäki, M. (1995). Smoking induced differences in autonomic responses in military pilot candidates. Clin. Auton. Res. 5, 31–36. doi: 10.1007/BF01845496
Krüger, K., Dischereit, G., Seimetz, M., Wilhelm, J., Weissmann, N., and Mooren, F. C. (2015). Time course of cigarette smoke-induced changes of systemic inflammation and muscle structure. Am. J. Physiol. Lung Cell. Mol. Physiol. 309, L119–L128. doi: 10.1152/ajplung.00074.2015
Krüger, K., Seimetz, M., Ringseis, R., Wilhelm, J., Pichl, A., Couturier, A., et al. (2018). Exercise training reverses inflammation and muscle wasting after tobacco smoke exposure. Am. J. Phys. Regul. Integr. Comp. Phys. 314, R366–R376. doi: 10.1152/ajpregu.00316.2017
Larsson, L., and Orlander, J. (1984). Skeletal muscle morphology, metabolism and function in smokers and non-smokers. A study on smoking-discordant monozygous twins. Acta Physiol. Scand. 120, 343–352. doi: 10.1111/j.1748-1716.1984.tb07394.x
Lauer, M. S., Pashkow, F. J., Larson, M. G., and Levy, D. (1997). Association of cigarette smoking with chronotropic incompetence and prognosis in the Framingham Heart Study. Circulation 96, 897–903. doi: 10.1161/01.CIR.96.3.897
Lauria, V. T., Sperandio, E. F., de Sousa, T. L., de Oliveira Vieira, W., Romiti, M., de Toledo Gagliardi, A. R., et al. (2017). Evaluation of dose-response relationship between smoking load and cardiopulmonary fitness in adult smokers: a cross-sectional study. Rev. Port. Pneumol. 23, 79–84. doi: 10.1016/j.rppnen.2016.11.007
Laustiola, K. E., Lassila, R., and Nurmi, A. K. (1988). Enhanced activation of the renin-angiotensin-aldosterone system in chronic cigarette smokers: a study of monozygotic twin pairs discordant for smoking. Clin. Pharmacol. Ther. 44, 426–430. doi: 10.1038/clpt.1988.175
Lawler, J. M., and Powers, S. K. (1998). Oxidative stress, antioxidant status, and the contracting diaphragm. Can. J. Appl. Physiol. 23, 23–55. doi: 10.1139/h98-002
Leary, P. J. (2016). Causality, correlation, and cardiac disease: does smoking cause cardiac hypertrophy and diastolic dysfunction? Circ. Cardiovasc. Imaging 9:e005441. doi: 10.1161/CIRCIMAGING.116.005441
Lee, I. M., Shiroma, E. J., Lobelo, F., Puska, P., Blair, S. N., Katzmarzyk, P. T., et al. (2012). Effect of physical inactivity on major non-communicable diseases worldwide: an analysis of burden of disease and life expectancy. Lancet 380, 219–229. doi: 10.1016/S0140-6736(12)61031-9
Lewis, P., and O’Halloran, K. D. (2016). Diaphragm muscle adaptation to sustained hypoxia: lessons from animal models with relevance to high altitude and chronic respiratory diseases. Front. Physiol. 7:623. doi: 10.3389/fphys.2016.00623
Liebow, A. A. (1959). Pulmonary emphysema with special reference to vascular changes. Am. Rev. Respir. Dis. 80, 67–93. doi: 10.1164/arrd.1959.80.1P2.67
Lindenmaier, T. J., Kirby, M., Paulin, G., Mielniczuk, L., Cunningham, I. A., Mura, M., et al. (2016). Pulmonary artery abnormalities in ex-smokers with and without airflow obstruction. COPD 13, 224–234. doi: 10.3109/15412555.2015.1074666
Liu, H., Ding, L., Zhang, Y., and Ni, S. (2014). Circulating endothelial microparticles involved in lung function decline in a rat exposed in cigarette smoke maybe from apoptotic pulmonary capillary endothelial cells. J. Thorac. Dis. 6, 649–655. doi: 10.3978/j.issn.2072-1439.2014.06.26
Liu, Y., Pleasants, R. A., Croft, J. B., Wheaton, A. G., Heidari, K., Malarcher, A. M., et al. (2015). Smoking duration, respiratory symptoms, and COPD in adults aged ≥45 years with a smoking history. Int. J. Chron. Obstruct. Pulmon. Dis. 10, 1409–1416. doi: 10.2147/COPD.S82259
Liu, Q., Xu, W. G., Luo, Y., Han, F. F., Yao, X. H., Yang, T. Y., et al. (2011). Cigarette smoke-induced skeletal muscle atrophy is associated with up-regulation of USP-19 via p38 and ERK MAPKs. J. Cell. Biochem. 112, 2307–2316. doi: 10.1002/jcb.23151
Madani, A., Alack, K., Richter, M. J., and Krüger, K. (2018). Immune-regulating effects of exercise on cigarette smoke-induced inflammation. J. Inflamm. Res. 11, 155–167. doi: 10.2147/JIR.S141149
Maehara, K., Riley, M., Galassetti, P., Barstow, T. J., and Wasserman, K. (1997). Effect of hypoxia and carbon monoxide on muscle oxygenation during exercise. Am. J. Respir. Crit. Care Med. 155, 229–235. doi: 10.1164/ajrccm.155.1.9001317
Mahmarian, J. J., Moyé, L. A., Nasser, G. A., Nagueh, S. F., Bloom, M. F., Benowitz, N. L., et al. (1997). Nicotine patch therapy in smoking cessation reduces the extent of exercise-induced myocardial ischemia. J. Am. Coll. Cardiol. 30, 125–130.
Martinez, C. H., Murray, S., Barr, R. G., Bleecker, E., Bowler, R. P., Christenson, S. A., et al. (2017). Respiratory symptoms items from the COPD assessment test identify ever-smokers with preserved lung function at higher risk for poor respiratory outcomes. An analysis of the subpopulations and intermediate outcome measures in COPD study cohort. Ann. Am. Thorac. Soc. 14, 636–642. doi: 10.1513/AnnalsATS.201610-815OC
Mendonca, G. V., Pereira, F. D., and Fernhall, B. (2011). Effects of cigarette smoking on cardiac autonomic function during dynamic exercise. J. Sports Sci. 29, 879–886. doi: 10.1080/02640414.2011.572991
Misigoj-Durakovic, M., Bok, D., Soric, M., Dizdar, D., Durakovic, Z., and Jukic, I. (2012). The effect of cigarette smoking history on muscular and cardiorespiratory endurance. J. Addict. Dis. 31, 389–396. doi: 10.1080/10550887.2012.735567
Miyatake, N., Numata, T., Nishii, K., Sakano, N., Suzue, T., Hirao, T., et al. (2011). Relation between cigarette smoking and ventilatory threshold in the Japanese. Environ. Health Prev. Med. 16, 185–190. doi: 10.1007/s12199-010-0178-6
Mobarrez, F., Antoniewicz, L., Bosson, J. A., Kuhl, J., Pisetsky, D. S., and Lundbäck, M. (2014). The effects of smoking on levels of endothelial progenitor cells and microparticles in the blood of healthy volunteers. PLoS One 9:e90314. doi: 10.1371/journal.pone.0090314
Mohamed Hoesein, F. A., de Jong, P. A., Lammers, J. W., Mali, W. P., Mets, O. M., Schmidt, M., et al. (2014). Contribution of CT quantified emphysema, air trapping and airway wall thickness on pulmonary function in male smokers with and without COPD. COPD 11, 503–509. doi: 10.3109/15412555.2014.933952
Montero, D. (2015). The association of cardiorespiratory fitness with endothelial or smooth muscle vasodilator function. Eur. J. Prev. Cardiol. 22, 1200–1211. doi: 10.1177/2047487314553780
Montes de Oca, M., Loeb, E., Torres, S. H., De Sanctis, J., Hernández, N., and Tálamo, C. (2008). Peripheral muscle alterations in non-COPD smokers. Chest 133, 13–18. doi: 10.1378/chest.07-1592
Morse, C. I., Pritchard, L. J., Wüst, R. C., Jones, D. A., and Degens, H. (2008). Carbon monoxide inhalation reduces skeletal muscle fatigue resistance. Acta Physiol. 192, 397–401. doi: 10.1111/j.1748-1716.2007.01757.x
Moylan, S., Jacka, F. N., Pasco, J. A., and Berk, M. (2012). Cigarette smoking, nicotine dependence and anxiety disorders: a systematic review of population-based, epidemiological studies. BMC Med. 10:123. doi: 10.1186/1741-7015-10-123
Muller, P. T., Utida, K. A. M., Augusto, T. R. L., Spreafico, M. V. P., Mustafa, R. C., Xavier, A. W., et al. (2018). Left ventricular diastolic dysfunction and exertional ventilatory inefficiency in COPD. Respir. Med. 145, 101–109. doi: 10.1016/j.rmed.2018.10.014
Mündel, T., and Jones, D. A. (2006). Effect of transdermal nicotine administration on exercise endurance in men. Exp. Physiol. 91, 705–713. doi: 10.1113/expphysiol.2006.033373
Nadruz, W., Claggett, B., Gonçalves, A., Querejeta-Roca, G., Fernandes-Silva, M. M., Shah, A. M., et al. (2016). Smoking and cardiac structure and function in the elderly: the ARIC study (Atherosclerosis Risk in Communities). Circ. Cardiovasc. Imaging 9:e004950. doi: 10.1161/CIRCIMAGING.116.004950
Nana-Sinkam, S. P., Lee, J. D., Sotto-Santiago, S., Stearman, R. S., Keith, R. L., Choudhury, Q., et al. (2007). Prostacyclin prevents pulmonary endothelial cell apoptosis induced by cigarette smoke. Am. J. Respir. Crit. Care Med. 175, 676–685. doi: 10.1164/rccm.200605-724OC
Neves, C. D., Lacerda, A. C., Lage, V. K., Lima, L. P., Tossige-Gomes, R., Fonseca, S. F., et al. (2016). Oxidative stress and skeletal muscle dysfunction are present in healthy smokers. Braz. J. Med. Biol. Res. 49:e5512. doi: 10.1590/1414-431x20165512
Nogueira, L., Trisko, B. M., Lima-Rosa, F. L., Jackson, J., Lund-Palau, H., Yamaguchi, M., et al. (2018). Cigarette smoke directly impairs skeletal muscle function through capillary regression and altered myofibre calcium kinetics in mice. J. Physiol. 596, 2901–2916. doi: 10.1113/JP275888
Oliveira, M. F., Zelt, J. T., Jones, J. H., Hirai, D. M., O’Donnell, D. E., Verges, S., et al. (2015). Does impaired O2 delivery during exercise accentuate central and peripheral fatigue in patients with coexistent COPD-CHF? Front. Physiol. 5:514. doi: 10.3389/fphys.2014.00514
Orlander, J., Kiessling, K. H., and Larsson, L. (1979). Skeletal muscle metabolism, morphology and function in sedentary smokers and nonsmokers. Acta Physiol. Scand. 107, 39–46. doi: 10.1111/j.1748-1716.1979.tb06440.x
Papathanasiou, G., Georgakopoulos, D., Georgoudis, G., Spyropoulos, P., Perrea, D., and Evangelou, A. (2007). Effects of chronic smoking on exercise tolerance and on heart rate-systolic blood pressure product in young healthy adults. Eur. J. Cardiovasc. Prev. Rehabil. 14, 646–652. doi: 10.1097/HJR.0b013e3280ecfe2c
Payne, J. R., Eleftheriou, K. I., James, L. E., Hawe, E., Mann, J., Stronge, A., et al. (2006). Left ventricular growth response to exercise and cigarette smoking: data from LARGE Heart. Heart 92, 1784–1788. doi: 10.1136/hrt.2006.088294
Petersen, A. M., Magkos, F., Atherton, P., Selby, A., Smith, K., Rennie, M. J., et al. (2007). Smoking impairs muscle protein synthesis and increases the expression of myostatin and MAFbx in muscle. Am. J. Physiol. Endocrinol. Metab. 293, E843–E848. doi: 10.1152/ajpendo.00301.2007
Pike, D., Kirby, M., Guo, F., McCormack, D. G., and Parraga, G. (2015). Ventilation heterogeneity in ex-smokers without airflow limitation. Acad. Radiol. 22, 1068–1078. doi: 10.1016/j.acra.2015.04.006
Raju, S. V., Solomon, G. M., Dransfield, M. T., and Rowe, S. M. (2016). Acquired cystic fibrosis transmembrane conductance regulator dysfunction in chronic bronchitis and other diseases of mucus clearance. Clin. Chest Med. 37, 147–158. doi: 10.1016/j.ccm.2015.11.003
Regan, E. A., Lynch, D. A., Curran-Everett, D., Curtis, J. L., Austin, J. H., Grenier, P. A., et al. (2015). Clinical and radiologic disease in smokers with normal spirometry. JAMA Intern. Med. 175, 1539–1549. doi: 10.1001/jamainternmed.2015.2735
Rennard, S. I., and Drummond, M. B. (2015). Early chronic obstructive pulmonary disease: definition, assessment, and prevention. Lancet 385, 1778–1788. doi: 10.1016/S0140-6736(15)60647-X
Rhee, C. K., Kim, K., Yoon, H. K., Kim, J. A., Kim, S. H., Lee, S. H., et al. (2017). Natural course of early COPD. Int. J. Chron. Obstruct. Pulmon. Dis. 12, 663–668. doi: 10.2147/COPD.S122989
Rizzi, M., Tarsia, P., La Spina, T., Cristiano, A., Frassanito, F., Macaluso, C., et al. (2016). A new approach to detect early lung functional impairment in very light smokers. Respir. Physiol. Neurobiol. 231, 1–6. doi: 10.1016/j.resp.2016.05.010
Rodrigues, F. M., Loeckx, M., Hornikx, M., Van Remoortel, H., Louvaris, Z., Demeyer, H., et al. (2018). Six years progression of exercise capacity in subjects with mild to moderate airflow obstruction, smoking and never smoking controls. PLoS One 13:e0208841. doi: 10.1371/journal.pone.0208841
Rom, O., Kaisari, S., Aizenbud, D., and Reznick, A. Z. (2012). Sarcopenia and smoking: a possible cellular model of cigarette smoke effects on muscle protein breakdown. Ann. N. Y. Acad. Sci. 1259, 47–53. doi: 10.1111/j.1749-6632.2012.06532.x
Roman, M. A., Rossiter, H. B., and Casaburi, R. (2016). Exercise, ageing and the lung. Eur. Respir. J. 48, 1471–1486. doi: 10.1183/13993003.00347-2016
Ross, J. C., Castaldi, P. J., Cho, M. H., Hersh, C. P., Rahaghi, F. N., Sánchez-Ferrero, G. V., et al. (2018). Longitudinal modeling of lung function trajectories in smokers with and without chronic obstructive pulmonary disease. Am. J. Respir. Crit. Care Med. 198, 1033–1042. doi: 10.1164/rccm.201707-1405OC
Ryan, B. J., Goodrich, J. A., Schmidt, W., Kane, L. A., and Byrnes, W. C. (2016). Ten days of intermittent, low-dose carbon monoxide inhalation does not significantly alter hemoglobin mass, aerobic performance predictors, or peak-power exercise tolerance. Int. J. Sports Med. 37, 884–889. doi: 10.1055/s-0042-108197
Santos, S., Peinado, V. I., Ramírez, J., Melgosa, T., Roca, J., Rodriguez-Roisin, R., et al. (2002). Characterization of pulmonary vascular remodelling in smokers and patients with mild COPD. Eur. Respir. J. 19, 632–638. doi: 10.1183/09031936.02.00245902
Saruya, S., Matsuoka, S., Yamashiro, T., Matsushita, S., Fujikawa, A., Yagihashi, K., et al. (2016). Quantitative CT measurements of small pulmonary vessels in chronic obstructive pulmonary disease: do they change on follow-up scans? Clin. Physiol. Funct. Imaging 36, 211–217. doi: 10.1111/cpf.12215
Saruya, S., Yamashiro, T., Matsuoka, S., Matsushita, S., Yagihashi, K., and Nakajima, Y. (2017). Decrease in small pulmonary vessels on chest computed tomography in light smokers without COPD: an early change, but correlated with smoking index. Lung 195, 179–184. doi: 10.1007/s00408-017-9985-5
Sashidhar, K., Gulati, M., Gupta, D., Monga, S., and Suri, S. (2002). Emphysema in heavy smokers with normal chest radiography. Detection and quantification by HCRT. Acta Radiol. 43, 60–65. doi: 10.1080/028418502127347457
Scallan, C., Doonan, R. J., and Daskalopoulou, S. S. (2010). The combined effect of hypertension and smoking on arterial stiffness. Clin. Exp. Hypertens. 32, 319–328. doi: 10.3109/10641960903443558
Schiess, R., Senn, O., Fischler, M., Huber, L. C., Vatandaslar, S., Speich, R., et al. (2010). Tobacco smoke: a risk factor for pulmonary arterial hypertension? A case-control study. Chest 138, 1086–1092. doi: 10.1378/chest.09-2962
Schmekel, B., Blomstrand, P., and Venge, P. (2013). Serum lysozyme - a surrogate marker of pulmonary microvascular injury in smokers? Clin. Physiol. Funct. Imaging 33, 307–312. doi: 10.1111/cpf.12029
Schweitzer, K. S., Hatoum, H., Brown, M. B., Gupta, M., Justice, M. J., Beteck, B., et al. (2011). Mechanisms of lung endothelial barrier disruption induced by cigarette smoke: role of oxidative stress and ceramides. Am. J. Phys. Lung Cell. Mol. Phys. 301, L836–L846. doi: 10.1152/ajplung.00385.2010
Soriano, J. B., Polverino, F., and Cosio, B. G. (2018). What is early COPD and why is it important? Eur. Respir. J. 52:1801448. doi: 10.1183/13993003.01448-2018
Steffl, M., Bohannon, R. W., Petr, M., Kohlikova, E., and Holmerova, I. (2015). Relation between cigarette smoking and sarcopenia: meta-analysis. Physiol. Res. 64, 419–426.
Talukder, M. A., Johnson, W. M., Varadharaj, S., Lian, J., Kearns, P. N., El-Mahdy, M. A., et al. (2011). Chronic cigarette smoking causes hypertension, increased oxidative stress, impaired NO bioavailability, endothelial dysfunction, and cardiac remodeling in mice. Am. J. Physiol. Heart Circ. Physiol. 300, H388–H396. doi: 10.1152/ajpheart.00868.2010
Tan, J., Xiong, B., Zhu, Y., Yao, Y., Qian, J., Rong, S., et al. (2019). Carotid body enlargement in hypertension and other comorbidities evaluated by ultrasonography. J. Hypertens. 37, 1455–1462. doi: 10.1097/HJH.0000000000002068
Telenga, E. F., Oudkerk, M., Ooijen, P. M. A., Vliegenthart, R., Hacken, N. H. T., Postma, D. S., et al. (2017). Airways wall thickness on HRCT scans decreases with age and increases with smoking. BMC Pulm. Med. 17:27. doi: 10.1186/s12890-017-0363-0
Tello, A., Marín, F., Roldán, V., Lorenzo, S., Moltó, J. M., and Sogorb, F. (2005). Influence of smoking habit on cardiac functional capacity and diastolic function in healthy people. Int. J. Cardiol. 98, 517–518. doi: 10.1016/j.ijcard.2003.11.025
Turner, J. A., and McNicol, M. W. (1993). The effect of nicotine and carbon monoxide on exercise performance in normal subjects. Respir. Med. 87, 427–431. doi: 10.1016/0954-6111(93)90068-B
Vieira Ramos, G., Choqueta de Toledo-Arruda, A., Maria Pinheiro-Dardis, C., Liyoko Suehiro, C., Luiz de Russo, T., Vieira, R. P., et al. (2018). Exercise prevents diaphragm wasting induced by cigarette smoke through modulation of antioxidant genes and metalloproteinases. Biomed. Res. Int. 2018:5909053. doi: 10.1155/2018/5909053
Vogel, J. A., and Gleser, M. A. (1972). Effect of carbon monoxide on oxygen transport during exercise. J. Appl. Physiol. 32, 234–239. doi: 10.1152/jappl.1972.32.2.234
Walter Barbosa, G., Neder, J. A., Utida, K., O’Donnell, D. E., and de Tarso Müller, P. (2017). Impaired exercise ventilatory efficiency in smokers with low transfer factor but normal spirometry. Eur. Respir. J. 49:1602511. doi: 10.1183/13993003.02511-2016
Ward, D. S., Dahan, A., and Teppema, L. (2005). Pharmacology and pathophysiology of the control of breathing. Boca Raton: Taylor & Francis.
Weatherald, J., Sattler, C., Garcia, G., and Laveneziana, P. (2018). Ventilatory response to exercise in cardiopulmonary disease: the role of chemosensitivity and dead space. Eur. Respir. J. 51:1700860. doi: 10.1183/13993003.00860-2017
Weissmann, N., Grimminger, F., and Seeger, W. (2012). Smoking: Is it a risk factor for pulmonary vascular diseases? Pulm. Circ. 2, 395–396. doi: 10.4103/2045-8932.105027
Woodruff, P. G., Barr, R. G., Bleecker, E., Christenson, S. A., Couper, D., Curtis, J. L., et al. (2016). Clinical significance of symptoms in smokers with preserved pulmonary function. N. Engl. J. Med. 374, 1811–1821. doi: 10.1056/NEJMoa1505971
World Health Organization (2010). Global recommendations on physical activity for health [pp. 1 online resource (1 PDF file (58 pages)]. Available at: https://www.who.int/dietphysicalactivity/factsheet_recommendations/en/ (Accessed May 2019).
World Health Organization (2011). WHO report on the global tobacco epidemic. Available at: https://www.who.int/tobacco/global_report/2011/en/ (Accessed April 2019).
Wüst, R. C., Degens, H., and Jones, D. A. (2008a). Muscle function in smokers: clearing up the smoke. Chest 134, 219–220. author reply 220. doi: 10.1378/chest.08-0564
Wüst, R. C., Gibbings, S. L., and Degens, H. (2009). Fiber capillary supply related to fiber size and oxidative capacity in human and rat skeletal muscle. Adv. Exp. Med. Biol. 645, 75–80. doi: 10.1007/978-0-387-85998-9_12
Wüst, R. C., Jaspers, R. T., van der Laarse, W. J., and Degens, H. (2008b). Skeletal muscle capillarization and oxidative metabolism in healthy smokers. Appl. Physiol. Nutr. Metab. 33, 1240–1245. doi: 10.1139/H08-116
Wüst, R. C., Morse, C. I., de Haan, A., Rittweger, J., Jones, D. A., and Degens, H. (2008c). Skeletal muscle properties and fatigue resistance in relation to smoking history. Eur. J. Appl. Physiol. 104, 103–110. doi: 10.1007/s00421-008-0792-9
Yilmaz, A., Yalta, K., Turgut, O. O., Yilmaz, M. B., Erdem, A., Karadas, F., et al. (2007). The effect of smoking on cardiac diastolic parameters including Vp, a more reliable and newer parameter. Cardiol. J. 14, 281–286.
Zavorsky, G. S., Smoliga, J. M., Longo, L. D., Uhranowsky, K. A., Cadman, C. R., Duffin, J., et al. (2012). Increased carbon monoxide clearance during exercise in humans. Med. Sci. Sports Exerc. 44, 2118–2124. doi: 10.1249/MSS.0b013e3182602a00
Zhu, X., Heunks, L. M., Versteeg, E. M., van der Heijden, H. F., Ennen, L., van Kuppevelt, T. H., et al. (2005). Hypoxia-induced dysfunction of rat diaphragm: role of peroxynitrite. Am. J. Physiol. Lung Cell. Mol. Physiol. 288, L16–L26. doi: 10.1152/ajplung.00412.2003
Keywords: smoking, exercise intolerance, physical activity, dyspnea, Fatigue
Citation: Muller PT, Barbosa GW, O’Donnell DE and Neder JA (2019) Cardiopulmonary and Muscular Interactions: Potential Implications for Exercise (In)tolerance in Symptomatic Smokers Without Chronic Obstructive Pulmonary Disease. Front. Physiol. 10:859. doi: 10.3389/fphys.2019.00859
Received: 03 May 2019; Accepted: 20 June 2019;
Published: 10 July 2019.
Edited by:
Mathieu Gruet, Université de Toulon, FranceReviewed by:
Leonardo Alexandre Peyré-Tartaruga, Federal University of Rio Grande do Sul, BrazilCopyright © 2019 Muller, Barbosa, O’Donnell and Neder. This is an open-access article distributed under the terms of the Creative Commons Attribution License (CC BY). The use, distribution or reproduction in other forums is permitted, provided the original author(s) and the copyright owner(s) are credited and that the original publication in this journal is cited, in accordance with accepted academic practice. No use, distribution or reproduction is permitted which does not comply with these terms.
*Correspondence: Paulo de Tarso Muller, cGF1bG8ubXVsbGVyQHVmbXMuYnI=; cGF1bG91Zm1zbXVsbGVyQGdtYWlsLmNvbQ==
Disclaimer: All claims expressed in this article are solely those of the authors and do not necessarily represent those of their affiliated organizations, or those of the publisher, the editors and the reviewers. Any product that may be evaluated in this article or claim that may be made by its manufacturer is not guaranteed or endorsed by the publisher.
Research integrity at Frontiers
Learn more about the work of our research integrity team to safeguard the quality of each article we publish.