- 1The Belt and Road Joint Laboratory for Winter Sports, Department of Exercise Physiology, Beijing Sport University, Beijing, China
- 2Department of Physical Education, Shanxi Medical University, Taiyuan, China
- 3School of Physical Education, Yan’an University, Yan’an, China
- 4Turku PET Centre, Department of Clinical Physiology and Nuclear Medicine, University of Turku, Turku, Finland
- 5Rydberg Laboratory for Applied Sciences, Department of Environmental and Biosciences, Halmstad University, Halmstad, Sweden
- 6Department of Exercise Science, East Stroudsburg University of Pennsylvania, East Stroudsburg, PA, United States
Background: Altitude training stimulates erythropoietin hormone (EPO) release and increases blood hemoglobin (Hb) mass, which may result in improved oxygen (O2) transport capacity. It was hypothesized in the present study that periodic inhalation of carbon monoxide (CO) might elicit similar physiological adaptations compared to altitude training.
Methods: Twelve male college student athletes, who were well-trained soccer players, participated. They performed a 4-week treadmill-training program, five times a week. Participants were randomly assigned into an experimental group with inhaling CO (INCO) (1 mL/kg body weight for 2 min) in O2 (4 L) before all training sessions and a control group without inhaling CO (NOCO). CO and EPO concentrations in venous blood were first measured acutely at the 1st, 2nd, 4th, 6th, and 8th hour after INCO, and total hemoglobin mass (tHb), running economy and VO2max were measured before and after the 4 weeks training intervention.
Results: HbCO% increased from 0.7 to 4.4% (P < 0.05) after 1 h of CO inhalation and EPO increased from 1.9 to 2.7 mIU/mL after 4 h post CO inhalation (P < 0.05) acutely before the intervention. After the training, the tHb and VO2max in the INCO group increased significantly by 3.7 and 2.7%, respectively, while no significant differences were observed in the NOCO condition. O2 uptake at given submaximal speeds declined by approximately 4% in the INCO group.
Conclusion: Acutely, EPO increased sharply post CO inhalation, peaking at 4 h post inhalation. 4-weeks of training with CO inhalation before exercise sessions improved tHb and VO2max as well as running economy, suggesting that moderate CO inhalation could be a new method to improve the endurance performance in athletes.
Introduction
Altitude training has been widely applied in endurance sports for enhancing athletes’ maximal aerobic power (Levine and Stray-Gundersen, 1997). The main physiological mechanism of the maximal aerobic power improvement may result from altitude training stimulating the secretion of EPO from the kidneys, which increases the formation of red blood cells (RBC) from the bone marrow and raises circulating total hemoglobin (tHb) mass (Chapman et al., 1998). Consequently, altitude training increases the ability of an individual to transport O2 to the working muscles, which enhance exercise power. In addition, altitude training has been documented to improve performance through enhancing the ability of skeletal muscle to buffer lactate and improving running economy (Gore et al., 2001; Saunders et al., 2004).
Carbon monoxide (CO) is an important endogenous molecule that can also be inhaled (Prabhakar, 1998). It has long been established that CO has an Hb binding ability greater than that of O2. Therefore, when an individual inhales CO, this can be considered to induce mild hypoxia in vivo, as carboxy-hemoglobin (HbCO) is incapable of carrying enough O2 and there is reduced amount of O2 bound to Hb and delivery to active muscle. As persistent CO inhalation reduces Hb ability to carry O2, acute exposure to small doses of CO could, in theory, induce similar performance benefits to that of altitude training. Previous research showed that increased ventilation in mild exercise rapidly removes CO from the blood in humans (Zavorsky et al., 2012). The clearance half-time in males is slower in comparison to their female counterparts due to their larger tHb (Zavorsky et al., 2014). If CO can enhance sporting performance, a sample of well-trained male individuals, who can have up to a 37% higher tHb in comparison to untrained athletes, (Kjellberg et al., 1949) is a suitable subject population as CO can remain in the blood for prolonged periods of time. Hence, it is hypothesized that an appropriate dosage of inhaled CO might have sufficient time to provoke mild hypoxia before clearance, causing similar physiological adaptive responses to that of altitude training.
Since a periodic inhalation of small amounts of CO at sea level might have potential to simulate the hypoxic effects of altitude and increase tHb mass and enhance maximal aerobic power, it is worthwhile to investigate if CO inhalation could offer a more convenient and less expensive method of improving exercise performance. In a study, by Fröscher and Uhlmann (2016) 10 days of intermittent, low-dose CO inhalation at rest did not lead to improvements in Hb mass or aerobic peak power, but to the best of our knowledge combined inhalation of CO and exercise training intervention has not been performed. Consequently, the aim of this study was to examine the effect of inhaling a small amount of CO on EPO secretion and total hemoglobin mass (tHb), running economy and maximal aerobic power in a combination with treadmill training in well trained young adults.
Materials and Methods
Subjects
Twelve healthy young adult soccer players from Beijing Sport University were recruited in this study by network and lecture recruitment. All subjects were living at low altitude, were non-smokers and had never participated in altitude training or hypoxic research, while they had participated in sport training for more than 10 years prior to the experiments. Moreover, all subjects understood the protocol and risks of the experiment and provided written informed consent. The study was approved by the University’s Sports Science Experimental Ethics Committee. A description of the subjects is presented in Table 1.
Test Protocol
One-Time CO Inhalation Testing
The study was divided into two separate experimental sections (Figure 1). Firstly, an acute preliminary experiment, where INCO group’s subjects inhaled a mixture of CO in O2 (4 L) over a 2 min time period by mask (1 mL/kg of body weight). The individuals in the INCO group remained at rest after inhalation of CO. Exhaled air was analyzed in numerous time points including pre, immediately post, and at each 1, 2, 4, 6, and 8 h interval post CO inhalation for exhaled gas CO concentration. EPO concentration was measured in venous serum (15 min, 3000 rpm) and venous blood HbCO% was measured at the same time points by Radiometer ABL 725.
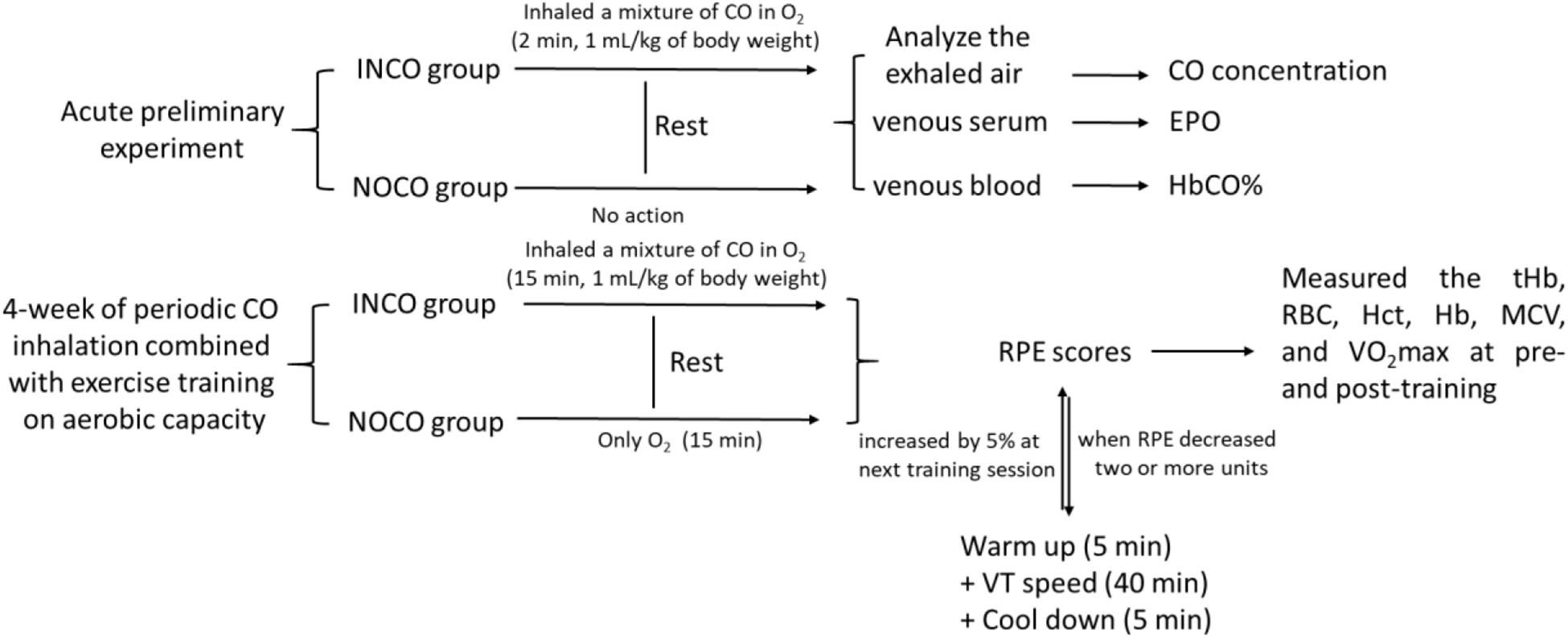
Figure 1. The test protocol of study. The INCO, NOCO, VT, tHb, RBC, Hct, Hb, MCV, and RPE are training group with CO inhalation, control group with no CO inhalation, ventilatory threshold, total hemoglobin, red blood cells, hematocrit, hemoglobin, mean corpuscular volume, and ratings of perceived exertion, respectively.
4-Week of Periodic CO Inhalation Testing
The second experimental section examined the effects of 4-week of periodic CO inhalation combined with exercise training on maximal aerobic power. Both pre- and post-training, the total hemoglobin (tHb), red blood cells (RBC), hematocrit (Hct), hemoglobin (Hb), mean corpuscular volume (MCV), running economy (RE), and VO2max were measured and compared between two groups, respectively. The exercise-training program consisted of treadmill running for 4-weeks, 5 times a week, and 50 min per session. Prior to each training session, all subjects inhaled gas for a period of 2 min continually experiment group (INCO): A mixture of CO (1 mL/kg of body weight) in O2 (4 L); Control group (NOCO): only O2 (4 L). CO inhalation occurred 15 min before commence of the training session. The treadmill speed commenced at 90% of ventilator threshold and was maintained for 40 min. 5 min prior to and post each run, subjects completed a warm up and cool down, resulting in a total running time of 50 min. At the end of each training session, the participants were requested to provide ratings of perceived exertion (RPE) scores. When the RPE decreased two or more units, the speed of the next training session was increased by 5%.
Determination of the RBC, Hct, Hb, and MCV
Blood red blood cells (RBC), hematocrit (Hct), hemoglobin (Hb), and mean corpuscular volume (MCV) tested from the venous blood samples by Bayer ADVIA 120, Germany.
Determination of Maximum Oxygen Uptake (VO2max)
VO2max was measured twice, once 24 h prior and again 24 h post 4 weeks of training, utilizing an incremental running test (Treadmill: h/p/cosmos, CORTEX Metalyzer 3B, Germany). The protocol included an initial 3-min warm up at 5 km/h and the speed was increased to 9 km/h. Treadmill speed was then increased by 1 km/h every minute, until the subjects were unable to continue or had reached the VO2max plateau. Subjects then cooled down at 5 km/h for 5 min.
Determination of Running Economy
Running economy was measured twice, before and after 4 weeks of training. The testing equipment was the same as with VO2max test. The protocol included an initial 3-min warm up at 5 km/h, and the speed was increased to 8 km/h and lasted for 8 min until steady O2 uptake had been reached. Then the speed was increased to 10 and 12 km/h individually for testing the running economy and these stages also lasted for 8 min.
Determination of Exhaled Gas and Blood CO Concentration
In the preliminary experiment, the concentrations of exhaled CO and venous blood (HbCO%) were measured pre and post CO inhalation (pre, 0, 1, 2, 4, 6, and 8 h). The CO concentration in exhaled (end-tidal) gas was measured using the Drager Pac 7000 CO (Germany) and HbCO% in blood was analyzed via Radiometer ABL 725 (Radio, United States).
Measurement of EPO
5 mL blood samples were drawn from cubital vein. Serum EPO was measured via ELISA (ELISA kit: Human EPO ELISA Kit, Sigma, United States; xMARK, Bio-rad, United States). The sensitivity of the test was 0.16 mIU/mL. The coefficient variable of intra-assay was 4.0% and the CV of inter-assay was 7.3%.
Determination of Hemoglobin Mass and Total Blood Volume
The measurements of tHb, total blood volume (BV), Plasma volume (PV), and Red cell volume (RCV) were determined through the CO-rebreathing method, according to the Durussel’s protocol (Kjellberg et al., 1949; Fröscher and Uhlmann, 2016). We measured these parameters twice, once 3 days prior to the start of training, and 3 days post the 4-week training intervention.
Statistics
Values are reported as mean ± standard deviation. Statistical analyses were performed by an analysis of variance (ANOVA) for repeated measures (acute experiments) and pre- and post-training intervention (2-way ANOVA). When significant F-ratios were detected, Student-Newman–Keuls post hoc tests were used to identify differences between time points or the INCO and NOCO conditions. A difference of P < 0.05 was classified as significant, and a difference of P < 0.01 was classified as very significant. The magnitude of changes was expressed as standardized mean differences (Effect Size, ES) (Cohen, 1988). ES-values between 0.2 and 0.49 indicated a small difference, between 0.5 and 0.79 as a medium difference, and from 0.8 and above as a large difference. All data analyses were carried out through SPSS 23.0.
Results
Preliminary Experiment
Exhaled CO concentration, blood HbCO% and EPO concentration initially increased after inhalation of CO (Figure 2). 1 and 2 h post inhalation, CO concentrations in exhaled gas were elevated (P < 0.05), returning to baseline 4 h post inhalation (Figure 2A). After initial increase, blood HbCO% (Figure 2B) fell with a clearance half-time of 5 h, but was still elevated 8 h post inhalation (P < 0.05). Serum EPO concentration (Figure 2C) increased significantly 2 h post inhalation (P < 0.05), peaking at 4 h post inhalation at 2.7 mIU/mL (an increase of 42.1% from baseline values), then gradually decreased over time.
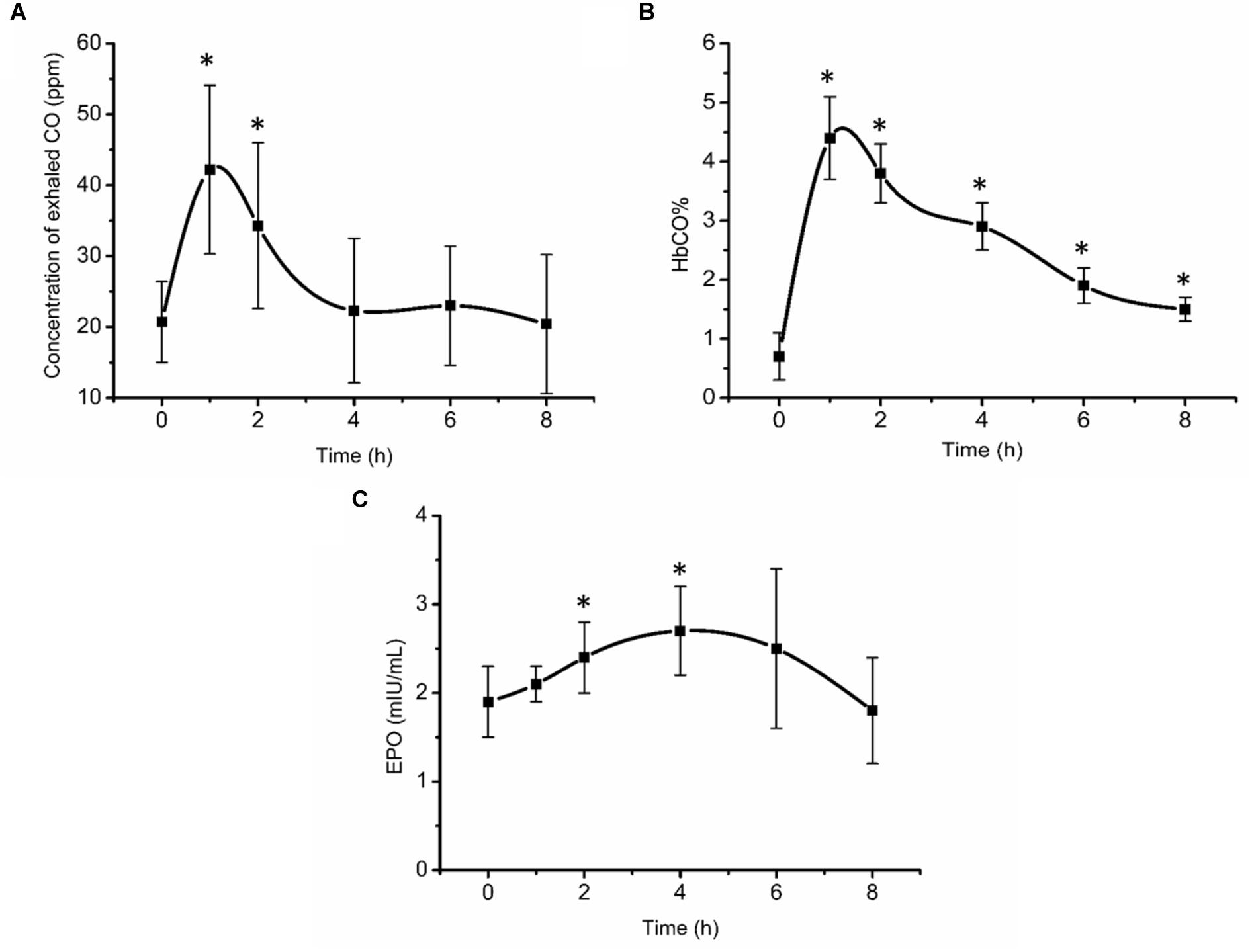
Figure 2. Exhaled CO concentration (A), blood HbCO% (B), and EPO concentration (C) after acute inhalation of CO. ∗Compared to baseline value P < 0.05. The HbCO% and EPO are percentage of carboxy-hemoglobin and erythropoietin hormone, respectively.
Exercise Training and CO Inhalation
Blood parameters in the INCO group and the NOCO group were measured 3 days pre and post of the 4-week training intervention as presented in Table 2. Post-training, a significant (P < 0.05) increase was observed in tHb (3.7%, ES = 0.76), RCV (5.3%, ES = 2.14), PV (6.8%, ES = 6.34), and BV (6.2%, ES = 39.45) in the INCO group. In contrast, NOCO group had no significant differences post-training intervention. Further, the hematological parameters of pre- and post-training intervention are shown in Table 3, and the values of these variables (RBC, Hct, Hb, and MCV) were not significantly different between the two conditions.
Maximal Aerobic Power After 4 Weeks of Training
The absolute and relative values of VO2max in the INCO condition significantly increased by 2.4 and 2.7% (P < 0.05, ES = 0.33 and 0.70), respectively, post 4 weeks of training. The NOCO condition showed no significant differences (0.1%, ES = 0.014). The VO2max in the INCO group was significantly higher (P < 0.05) post-training when compared to the NOCO group. The data are presented in Table 4.
At submaximal running speeds (8, 10, and 12 km/h), absolute/relative O2 uptake of both the INCO and NOCO group appears to be lower post-training, as shown in Table 5. However, the decrease was only significant in the INCO group (3.7, 3.6, and 5.4%, ES = 0.61, 0.48, and 0.63, respectively) (P < 0.05).
Discussion
The results of the present study demonstrated that EPO concentration initially increased after one inhalation of CO (peaking at 4 h post inhalation (42.1%), then gradually decreased over time), also 4-week of sea level treadmill endurance training with an application of 1 mL/kg of CO inhalation 15 min prior to each 50-min training sessions increased subjects’ tHb (3.7%), plasma (6.8%), and whole blood volume (6.2%), VO2max (2.7%), and running economy (4%). Therefore, it is proposed that inhalation of CO is a practical method of inducing the performance enhancing physiological adaptations of hypoxia in well-trained athletes, without the logistical and financial burdens of traveling to altitude.
Acute Effects of CO Inhalation on EPO Secretion
It is well-known that CO binds to Hb stronger than oxygen and is therefore potentially dangerous, especially when concentration is higher than 35 ppm or HbCO percentage of 15–20%. However, it is also widely used agent in medical research. CO re-breathing method is widely used to measure tHb, (Schmidt and Prommer, 2005; Jérôme et al., 2013) radiolabeled CO is used to measure regional tissue blood volume, (Heinonen et al., 2014) and CO is also used in physiological research to address fundamental aspects of cardiovascular regulation (González-Alonso et al., 2002). In the present study acute CO inhalation measures indicated that HbCO% was close to 5% at the highest (below 18 ppm) and gradually decreased to 2% at the 8 h time point. EPO is a glycoprotein secreted by cells in many tissues, mainly the renal cortical cells. When the O2 concentration in the renal cortex decreases, EPO secretion into the blood increases, causing myeloid progenitor stem cells to promote erythropoiesis (Sasaki, 2003; Lee and Percy, 2010). This occurs when low landers travel to altitude, due to the reduction in barometric pressure at altitude, which results in hypoxemia. Training at altitude takes advantage of this response, where hypoxia independent of training, stimulates EPO secretion (Schmidt et al., 1991; Volker, 2013). As a result, Hb increases and O2 delivery to the muscles is enhanced, enabling athletes to perform better on their return to sea level for competition (Geiser et al., 2001). In the preliminary experiments of the present study, approximately 2 h post CO inhalation, EPO concentration increased significantly by 26.9% and later by 42.3% at 4 h post CO inhalation. These results are similar to those previously documented by Eckardt et al. (1989) who determined that in subjects who were exposed to simulated altitude of 3000 and 4000 m, respectively, EPO significantly increased at 114 and 84 min, respectively, with a percentage increase of 40.6 and 67.7%, respectively. Therefore, it can be concluded that the current clinically safe dose of 1 mL/kg BW of CO as also previously utilized by Schmidt and Prommer (2010) is sufficient to induce a hypoxic state in vivo stimulating also EPO release.
It is known that CO binds avidly to Hb, with an affinity approximately 200-fold higher than that of O2 (Karen and Jean, 2007). When CO is inhaled, it readily displaces O2 from its binding sites on Hb. This occurs without a reduction in arterial PO2. While altitude results in reduction in both arterial PO2 and HbO2 concentration, inhalation of CO reduces only arterial HbO2 concentration. Therefore, with HbCO potentially impairing O2 delivery to cells, cellular hypoxia may develop in spite of a normal arterial PO2 as the plasma oxygen carrying capacity is very limited triggering an increased EPO secretion by the kidneys. It has been shown that increased ventilation during exercise may accelerate the removal of CO from the blood, (Zavorsky et al., 2012) but in the present study this was not determined, but should be addressed further in the future.
Training Adaptations as a Result of Repetitive CO Inhalations
VO2max
The increase of VO2max through altitude training is thought to be the result of a combination of factors including better oxygen carrying capacity and enhanced O2 kinetics (Gore et al., 2001; Wehrlin et al., 2006; Kane et al., 2016). Importantly, it should be noted that altitude-induced hypoxemia is well known to stimulate compensatory hyperventilation, causing systemic alkalosis. This results in renal excretion of sodium and bicarbonate in an attempt to normalize pH. The excretion of sodium and bicarbonate increases urinary loss of water, which may result in possible dehydration and an early (EPO-unrelated) increase in Hb and Hct, from the reduction in PV. This may in turn act to impair cardiac output and O2 delivery and athletic performance. In contrast, CO does not stimulate hyperventilation, as there is no basis for dehydration after CO inhalation. As a consequence, BV may be better maintained using CO to simulate altitude in comparison to altitude exposure. Indeed, our results seem to reflect this expectation (Table 4). Further, as by 3 days post the 4-week training intervention tHb increased by 3.7% (ES = 0.76) in the INCO condition and by 2.8% (ES = 0.93) in the control group, effect was evident and indirectly demonstrated the function of EPO. It is suspected that some potential reasons for this may have been due to the elevated tHb and thus prolonged half-time of CO (Zavorsky et al., 2014) as well as an existing elevation in tHb, due to highly trained status of the sample (Kjellberg et al., 1949). While the present study cannot establish cause and effect, the percentage increases in BV were similar to those of the VO2max (Tables 2, 4).
In this study, the increase of both tHb and RCV correspond to the results of Wehrlin et al. (2006) who after 24 days of altitude training showed that Hb mass increased by 5.3% and RCV by 5%. In addition, Friedmann et al. (2005) observed that elite athletes who lived and trained for 3-week at an altitude of 2100–2300 m, showed on average a significant similar increase in tHb (6%). In line with Friedmann et al. (2005) no significant difference in Hct was apparent in this study despite of the increase in tHb, when comparing pre and post-training measurements. It is suggested that the increase in PV may be the reason. However, contradictory to our results utilizing CO inhalation, Wehrlin et al. (2006) found no significant change in PV and BV post-training at altitude. Two of the fundamental factors that directly impact maximal aerobic power are O2 transport through the cardiovascular system and O2 utilization (Saltin and Strange, 1992). In particular O2 transport can be influenced by tHb in the blood, as well as BV (Martino et al., 2002). Hence, high tHb and BV are essential to have a high maximal aerobic power (Green et al., 1999). It has been suggested that a 1 g increase in tHb can increase VO2max by approximately 4 mL/min (Schmidt and Prommer, 2010). Moreover, a higher BV, whether via an increase in PV or erythrocyte mass, causes an increase in stroke volume, cardiac output and thus an elevation in VO2max. A change in BV of 1.0 mL triggers a variation in VO2max by 0.7 mL/g/min (Eastwood et al., 2012). However, if tHb rises excessively there is the chance of blood viscosity increasing and having a negative impact on cardiac output, (Rhodes et al., 2009) nulling any aerobic fitness benefits. It is assumed that in this study the increase in PV likely prevented an increase in blood viscosity and thus the higher tHb mass did not interfere with cardiac output, resulting in improved maximal aerobic performance.
Running Economy
Oxygen uptake marginally, but significantly decreased at submaximal speeds of 8, 10, and 12 km/h, suggesting an improvement in running economy (Figure 3). In accordance, research in hypobaric hypoxia with elite athletes, at submaximal intensities, has demonstrated that altitude training can enhance energetics through stimulating erythropoiesis, causing an increase in VO2max and a reduced energy cost of exercise (Daniel et al., 1986). A major contributories in the present study are thought to be CO’s ability to stimulate mitochondrial biogenesis, (Czuba et al., 2014) which can be partially initiated when CO binds to cytochrome c oxidase and heightens mitochondrial hydrogen peroxide formation (Suliman et al., 2007). Furthermore, CO acts similarly as nitric oxide, which has been shown to reduce skeletal muscle oxygen consumption in humans (Heinonen et al., 2011), which likely translates to benefit also exercising oxygen economy. Additionally, it has also been identified that post CO exposure, major histocompatibility complex class I (MHCI) protein expression is elevated in skeletal muscle, indicating a shift to efficient muscular phenotypes (Czuba et al., 2014). Finally, CO exposure may have an essential role in the preservation of cellular homeostasis at sites of muscle damage through the stabilization of HIF-1α, causing an up-regulation of TGF-α, leading to its pleiotropic effects (Chin et al., 2007). However, due to the premature and novel nature of this research, such mechanistic underpinnings can only be speculated. Nevertheless, it is probable that CO, in vivo, may have a complex multi-disciplinary contribution to improve running economy. Thus, further research is required to determine CO’s mechanistic role in vivo during exercise in the improvement of oxygen utilization economy.
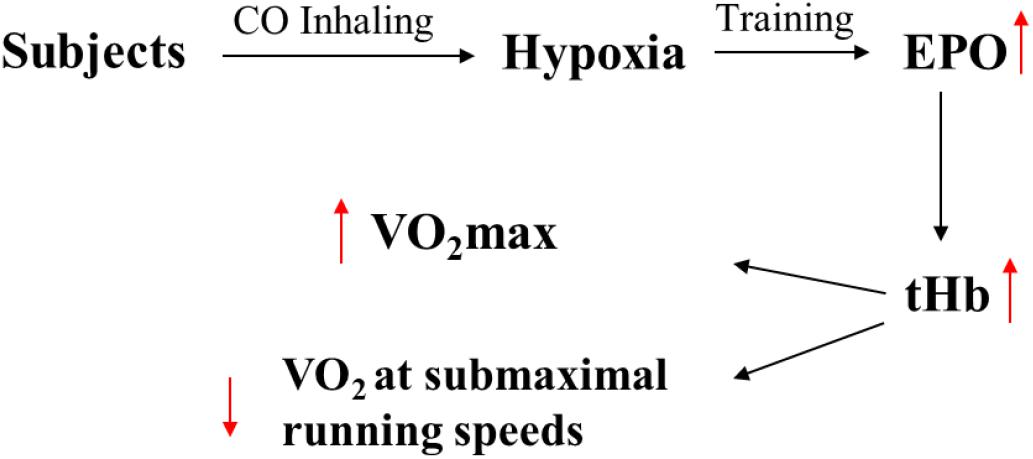
Figure 3. The assumed physiological reasons of improving in performance caused by inhalation of CO. The EPO and tHb are erythropoietin hormone and total hemoglobin.
Limitations
This study included only fairly small number of subjects who all were football players. Therefore, these findings should be extended by studying larger amounts of subjects including also other sport disciplines. Furthermore, as excessive CO breathing can be detrimental and can even lead to death, CO breathing interventions such as presented here should only be done in well-controlled laboratory setting, where both exhaled and blood CO values are always closely monitored and appropriate first aid facilities are readily accessible if needed.
Conclusion
Inhalation of CO (1 mL/kg BW) in normoxia stimulates EPO secretion in well-trained male soccer players. Inhaling 1 mL/kg CO prior to training sessions (five times/week for 4 weeks) increases PV, BV, RBC, tHb, running economy, and VO2max post-training and may thus be an alternative method to living and/or training at altitude, although no direct comparison to altitude training was performed in the present study. Therefore, it is proposed that inhalation of CO is a practical method of inducing the performance enhancing physiological adaptations of hypoxia, without the logistical and financial burdens of traveling to attitude. Furthermore, CO inhalation may also avoid the dehydrating diuresis typical in altitude, which can impair performance. However, it is emphasized that further research is warranted in regards to the ethical concerns of CO inhalation on individual athletes and in competitive sports.
Ethics Statement
This study was carried out in accordance with the recommendations of sport science ethics review of Beijing Sport University, Beijing Sport University Institutional Review Board (BSU IRB), with written informed consent from all subjects. All subjects gave written informed consent in accordance with the Declaration of Helsinki. The protocol was approved by the “BSU IRB.”
Author Contributions
All authors listed have made a substantial, direct and intellectual contribution to the work, and approved it for publication.
Funding
The study was supported by National Programs for Winter Olympics of Ministry of Science and Technology (2018YFF0300800) and special funds for Basic Scientific Research Services of Chinese Central University (2018GJ006).
Conflict of Interest Statement
The authors declare that the research was conducted in the absence of any commercial or financial relationships that could be construed as a potential conflict of interest.
Acknowledgments
The authors wish to thank the subjects from Beijing Sport University.
References
Chapman, R. F., Stray-Gundersen, J., and Levine, B. D. (1998). Individual variation in response to altitude training. J. Appl. Physiol. 85, 1448–1456. doi: 10.1152/jappl.1998.85.4.1448
Chin, B. Y., Jiang, G., Wegiel, B., Wang, H. J., MacDonald, T., Zhang, X. C., et al. (2007). Hypoxia-inducible factor 1α stabilization by by carbon monoxide results in cytoprotective preconditioning. Proc. Natl. Acad. Sci. U.S.A. 104, 5109—-5114.
Cohen, J. (1988). Statistical Power Analysis for the Behavioural Sciences, 2nd Edn. Hillsdale, NJ: Laurence Earlbaum Associates.
Czuba, M., Maszczyk, A., Gerasimuk, D., Roczniok, R., Fidos-Czuba, O., Zając, A., et al. (2014). The effects of hypobaric hypoxia on erythropoiesis, maximal oxygen uptake and energy cost of exercise under normoxia in elite biathletes. J. Sports Sci. Med. 13, 912–920.
Daniel, M. K., Bennett, B., Dawson, A. A., and Rawles, J. M. (1986). Hemoglobin concentration and linear cardiac output, peripheral resistance, and oxygen transport. Br. Med. J. 292, 923–926. doi: 10.1136/bmj.292.6525.923
Eastwood, A., Bourdon, P. C., Norton, K. I., Lewis, N. R., Snowden, K. R., and Gore, C. J. (2012). No change in hemoglobin mass after 40 days of physical activity in previously untrained adults. Scand. Med. Sci. Sports 22, 722–728. doi: 10.1111/j.1600-0838.2011.01310.x
Eckardt, K. U., Boutellier, U., Kurtz, A., Schopen, M., Koller, E. A., and Bauer, C. (1989). Rate of erythropoietin formation in humans in response to acute hypobaric hypoxia. J. Appl. Physiol. 66, 1785–1788. doi: 10.1152/jappl.1989.66.4.1785
Friedmann, B., Frese, F., Menold, E., Kauper, F., Jost, J., and Bartsch, P. (2005). Individual variation in the erythropoietic response to altitude training in elite junior swimmers. Br. J. Sports Med. 39, 148–153. doi: 10.1136/bjsm.2003.011387
Fröscher, W., and Uhlmann, C. (2016). Ten days of intermittent, low-dose carbon monoxide inhalation does not significantly alter hemoglobin mass, aerobic performance predictors, or peak-power exercise tolerance. Int. J. Sports Med. 37, 884–889. doi: 10.1055/s-0042-108197
Geiser, J., Vogt, M., Billeter, R., Zuleger, C., Belforti, F., and Hoppeler, H. (2001). Training high-living low: changes of aerobic performance and muscle structure with training at simulated altitude. Int. J. Sports Med. 22, 579–585. doi: 10.1055/s-2001-18521
González-Alonso, J., Olsen, D. B., and Saltin, B. (2002). Erythrocyte and the regulation of human skeletal muscle blood flow and oxygen delivery: role of circulating ATP. Circ. Res. 91, 1046–1055. doi: 10.1161/01.res.0000044939.73286.e2
Gore, C. J., Hahn, A. G., Aughey, R. J., Martin, D. T., Ashenden, M. J., Clark, S. A., et al. (2001). Live high: train low increases muscle buffer capacity and submaximal cycling efficiency. Acta Physiol. Scand. 173, 275–286. doi: 10.1046/j.1365-201x.2001.00906.x
Green, H. J., Carter, S., Grant, S., Tupling, R., Coates, G., and Ali, M. (1999). Vascular volumes and hematology in male and female runners and cyclists. Eur. Appl. Physiol. 79, 244–250. doi: 10.1007/s004210050502
Heinonen, I., Kudomi, N., Kemppainen, J., Kiviniemi, A., Noponen, T., Luotolahti, M., et al. (2014). Myocardial blood flow and its transit time, oxygen utilization, and efficiency of highly endurance-trained human heart. Basic Res. Cardiol. 109:413. doi: 10.1007/s00395-014-0413-1
Heinonen, I., Saltin, B., Kemppainen, J., Sipilä, H. T., Oikonen, V., Nuutila, P., et al. (2011). Skeletal muscle blood flow and oxygen uptake at rest and during exercise in humans: a PET study with nitric oxide and cyclooxygenase inhibition. Am. J. Physiol. Heart Circ. Physiol. 300, H1510–H1517. doi: 10.1152/ajpheart.00996.2010
Jérôme, D., Ramzy, R., and Prithvi, R. K. (2013). Precision of the optimized carbon monoxide rebreathing method to determine total haemoglobin mass and blood volume. Eur. J. Sport Sci. 13, 68–77. doi: 10.1080/17461391.2011.606843
Kane, L. A., Ryan, B. J., Schmidt, W., and Byrnes, W. C. (2016). Acute, low-dose CO inhalation does not alter energy expenditure during submaximal exercise. Int. J. Sports Med. 37, 19–24. doi: 10.1055/s-0035-1554641
Karen, I. B., and Jean, L. C. (2007). Exogenous acquired metabolic disorders of the nervous system: toxins and illicit drugs. Textbook Clin. Neurol. 32, 865–896. doi: 10.1016/b978-141603618-0.10039-6
Kjellberg, S. R., Rudhe, U., and Sjostr, T. (1949). Increase of the amount of hemoglobin and blood volume in connection with physical training. Acta Physiol. 19, 146–151. doi: 10.1111/j.1748-1716.1949.tb00146.x
Lee, F. S., and Percy, M. J. (2010). The HIF pathway and erythrocytosis. Annu. Rev. Pathol. 6, 165–192. doi: 10.1146/annurev-pathol-011110-130321
Levine, B. D., and Stray-Gundersen, J. (1997). Living high-training low: effect of moderate-altitude acclimatization with low-altitude training on performance. J. Appl. Physiol. 83, 102–112. doi: 10.1152/jappl.1997.83.1.102
Martino, M., Gledhilln, G., and Jamnik, V. (2002). High VO2max with no history of training is primarily due to high blood volume. Med. Sci. Sport. Exer. 34, 966–971. doi: 10.1097/00005768-200206000-00010
Prabhakar, N. R. (1998). Endogenous carbon monoxide in control of respiration. Resp. Physiol. 114, 57–64. doi: 10.1016/s0034-5687(98)00072-3
Rhodes, M. A., Carraway, M. S., Piantadosi, C. A., Reynolds, C. M., Cherry, A. D., Wester, T. E., et al. (2009). Carbon monoxide, skeletal muscle oxidative stress, and mitochondrial biogenesis in humans. Am. J. Physiol. Heart Circ. Physiol. 297, H392–H399. doi: 10.1152/ajpheart.00164.2009
Saltin, B., and Strange, S. (1992). Maximal oxygen uptake: “old” and “new” arguments for a cardiovascular limitation. Med. Sci. Sports Exer. 24, 30–37.
Sasaki, R. (2003). Pleiotropic functions of erythropoietin. Int. Med. 42, 142–149. doi: 10.2169/internalmedicine.42.142
Saunders, P. U., Pyne, D. B., Richard, D., Telford, R. D., and Hawley, J. A. (2004). Factors affecting running economy in trained distance runners. Sports Med. 34, 465–485. doi: 10.2165/00007256-200434070-00005
Schmidt, W., Eckardt, K. U., Hilgendorf, A., Strauch, S., and Bauer, C. (1991). Effects of maximal and submaximal exercise under normoxic and hypoxic conditions on serum erythropoietin level. Int. J. Sports Med. 12, 457–461. doi: 10.1055/s-2007-1024713
Schmidt, W., and Prommer, N. (2005). The optimised CO-rebreathing method: a new tool to determine total haemoglobin mass routinely. Eur. J. Appl. Physiol. 95, 486–495. doi: 10.1007/s00421-005-0050-3
Schmidt, W., and Prommer, N. (2010). Impact of alterations in total hemoglobin mass on VO2max. Exerc. Sport Sci. Rev. 38, 68–75. doi: 10.1097/JES.0b013e3181d4957a
Suliman, H. B., Carraway, M. S., Tatro, L. G., and Piantadosi, C. A. (2007). A new activating role for CO in cardiac mitochondrial biogenesis. J. Cell Sci. 120, 299–308. doi: 10.1242/jcs.03318
Volker, H. H. (2013). Regulation of erythropoiesis by hypoxia-inducible factors. Blood Rev. 27, 41–53. doi: 10.1016/j.blre.2012.12.003
Wehrlin, J. P., Zuest, P., Hallen, J., and Marti, B. (2006). Live high-train low for 24 days increases hemoglobin mass and red cell volume in elite endurance athletes. J. Appl. Physiol. 100, 1938–1945. doi: 10.1152/japplphysiol.01284.2005
Zavorsky, G. S., Smoliga, J. M., Longo, L. D., Uhranowsky, K. A., Cadman, C. R., Duffin, J., et al. (2012). Increased carbon monoxide clearance during exercise in humans. Med. Sci. Sport Exer. 44, 2118–2124. doi: 10.1249/mss.0b013e3182602a00
Keywords: carbon monoxide, endurance training, EPO, total hemoglobin mass, running economy, maximal aerobic power
Citation: Wang J, Ji Y, Zhou L, Xiang Y, Heinonen I and Zhang P (2019) A New Method to Improve Running Economy and Maximal Aerobic Power in Athletes: Endurance Training With Periodic Carbon Monoxide Inhalation. Front. Physiol. 10:701. doi: 10.3389/fphys.2019.00701
Received: 10 January 2019; Accepted: 20 May 2019;
Published: 06 June 2019.
Edited by:
Leonardo Alexandre Peyré-Tartaruga, Federal University of Rio Grande do Sul, BrazilReviewed by:
Simona Mrakic-Sposta, Institute of Bioimaging and Molecular Physiology, National Research Council, ItalyMarcelo Coertjens, Federal University of Piauí, Brazil
Copyright © 2019 Wang, Ji, Zhou, Xiang, Heinonen and Zhang. This is an open-access article distributed under the terms of the Creative Commons Attribution License (CC BY). The use, distribution or reproduction in other forums is permitted, provided the original author(s) and the copyright owner(s) are credited and that the original publication in this journal is cited, in accordance with accepted academic practice. No use, distribution or reproduction is permitted which does not comply with these terms.
*Correspondence: Jun Wang, MTU3NzE1MjMzQHFxLmNvbQ==; d2o0MzAwNzlAMTI2LmNvbQ==; Yang Xiang, eDM3ODY4MTA4NEAxNjMuY29t