- 1Departamento de Ciencias Farmacéuticas y de la Salud, Facultad de Farmacia, Universidad San Pablo-CEU, CEU Universities, Madrid, Spain
- 2Departamento de Farmacología, Facultad de Farmacia, Instituto Pluridisciplinar, Universidad Complutense de Madrid, Madrid, Spain
- 3Departamento de Fisiología, Facultad de Medicina, Universidad Autónoma de Madrid, Madrid, Spain
- 4Servicio de Endocrinología y Nutrición, Facultad de Medicina, Hospital Clínico San Carlos, Instituto de Investigaciones Sanitarias San Carlos, Universidad Complutense de Madrid, Madrid, Spain
- 5Servicio de Cirugía, Facultad de Medicina, Hospital Clínico San Carlos, Instituto de Investigaciones Sanitarias San Carlos, Universidad Complutense de Madrid, Madrid, Spain
- 6Departamento de Bioquímica, Facultad de Farmacia, Universidad Complutense de Madrid, Madrid, Spain
Bariatric surgery (BS) results in sustained weight loss and may reverse inflammation, metabolic alterations, extracellular matrix remodeling and arterial stiffness. We hypothesize that increased stiffening in omental arteries from obese patients might be associated with an increase in MMP activity and a decrease in p-AMPK, together with systemic oxidative stress and inflammation. Moreover, BS could contribute to reversing these alterations. This study was conducted with 38 patients of Caucasian origin: 31 adult patients with morbid obesity (9 men and 22 women; mean age 46 years and BMI = 42.7 ± 1.0 kg/m2) and 7 non-obese subjects (7 women; mean age 45 years and BMI = 22.7 ± 0.6 kg/m2). Seventeen obese patients were studied before and 12 months after BS. The stiffness index β, an index of intrinsic arterial stiffness, was determined in omental arteries and was significantly higher in obese patients. Levels of phosphorylated AMPK (p-AMPKThr-172) and SIRT-1 were significantly lower in peripheral blood mononuclear cells (PBMCs) from obese patients than those from non-obese patients (p < 0.05) and were normalized after BS. Total and active MMP-9 activities, LDH, protein carbonyls and uric acid were higher in obese patients and reduced by BS. Moreover, there was a correlation between plasmatic LDH levels and the stiffness index β. BS has a beneficial effect on abnormal MMP-9, LDH and AMPK activities that might be associated with the development of arterial stiffness in obese patients. Since these parameters are easily measured in blood samples, they could constitute potential biomarkers of cardiovascular risk in morbid obesity.
Introduction
Obesity constitutes a major social problem worldwide and is associated with insulin resistance, diabetes, and a higher risk of cardiovascular disease (CVD) (Morris, 2008). The increased accumulation of adipose tissue, especially in a visceral location, is strongly associated with a systemic pro-inflammatory and pro-oxidative state, leading to endothelial dysfunction, vascular remodeling, and arterial stiffness (Sutton-Tyrrell et al., 2001; Brunner et al., 2015; Morigami et al., 2016). Systemic arterial stiffness, assessed by pulse wave velocity (PWV) or the augmentation index is a classic feature of vascular aging and an independent predictor of cardiovascular morbidity and mortality (Turin et al., 2010; Vlachopoulos et al., 2010). Body fat and central adiposity have been suggested as predictors of accelerated arterial stiffness that develops at younger ages in obese patients (Nemes et al., 2008; Grassi and Diez, 2009; Kangas et al., 2013; Brunner et al., 2015; Satoh-Asahara et al., 2015). Stiffness index β, obtained from the stress–strain relationship (Dobrin, 1978), is currently a well-accepted marker of intrinsic arterial stiffness and it has been determined in several studies performed in human resistance arteries (Savoia et al., 2008; Fonck et al., 2009; Grassi et al., 2010). In addition, the increase of intrinsic stiffness of large arteries is now considered an independent predictor of cardiovascular (CV) diseases (Bouissou et al., 2014) and precedes changes in systemic arterial stiffness (Sehgel et al., 2013).
A variety of pathophysiological mechanisms might underlie the arterial stiffness development associated with obesity. AMP-activated protein kinase (AMPK) is a Ser/Thr protein kinase that senses cell energy status and contributes to restoring energy homeostasis (Hardie, 2007; Hue and Rider, 2007). AMPKα, the catalytic subunit of AMPK that is activated via phosphorylation (Dammanahalli and Sun, 2008; Chen et al., 2015), plays a protective role in vascular function (Garcia-Prieto et al., 2015b,c) and prevents the activation of mechanisms implicated in the development of arterial stiffness (for review see Garcia-Prieto et al., 2015a; Salt and Hardie, 2017). Dysregulation of AMPK is a pathogenic factor for the development of vascular disease (Lee et al., 2005) in genetic obese animal models (Ruderman et al., 2013) and humans (Gauthier et al., 2011; Ruderman et al., 2013). In severely obese patients before bariatric surgery (BS), the downregulation in AMPK activity in adipose tissue is linked to increased visceral adiposity, oxidative stress and inflammation (Gauthier et al., 2011; Xu et al., 2012).
Sirtuins, SIRT1–SIRT7, comprise a family of NAD+-dependent enzymes. The most studied is SIRT1, an NAD+-dependent protein deacetylase that protects against CVD (for review see Winnik et al., 2015). It has been shown to reduce arterial stiffness and endothelial dysfunction by inhibiting inflammatory and oxidant pathways (Gano et al., 2014; Fry et al., 2016). SIRT1 might be activated by caloric restriction and increased energy expenditure (Cohen et al., 2004; Civitarese et al., 2007; Chen et al., 2008), but downregulated by energy oversupply (Pfluger et al., 2008). In fact, SIRT1 expression is significantly reduced in adipose tissue from obese humans (Xu et al., 2013).
Matrix metalloproteinases (MMPs) are a large family of endopeptidases that can proteolyze all components of the extracellular matrix (ECM). MMP-2 and MMP-9 are involved in the cleavage of denatured collagen (gelatin), elastin and type IV collagen in blood vessels (Spinale, 2007) and in adipogenesis, vascular remodeling, and inflammation (Jaiswal et al., 2011). Therefore, MMP-9 seems to favor arterial stiffness and the development and progression of hypertension (Flamant et al., 2007; Zhou et al., 2007). In obese subjects, both MMP-2 and MMP-9 plasma levels are increased (Derosa et al., 2008; Andrade et al., 2012).
Weight loss is associated with an improvement in cardiometabolic disorders (Sjostrom et al., 2012). Since BS constitutes the most effective strategy for treating morbidly obese patients (Fruhbeck, 2015), it is a useful approach to search for new potential biomarkers of obesity-associated cardiovascular risk. We hypothesize that obese patients show a decrease in p-AMPK and an increase in MMPs activity associated with systemic oxidative stress and inflammation, and that body weight reduction derived from BS could significantly contribute to reversing these alterations. Therefore, the aim of this study was to evaluate the effect of BS on: (i) oxidative and inflammatory markers, (ii) AMPK activity and SIRT-1 expression, (iii) MMP-9 activity and LDH levels, and (iv) the stiffness index β. Changes in these parameters might allow the definition of potential biomarkers for the development of obesity-related arterial stiffness.
Materials and Methods
Study Population
As shown in Figure 1, this study was conducted with 38 subjects of Caucasian origin: 31 adult patients with morbid obesity [9 men and 22 women; mean age 46 years (range 27–62 years) and BMI = 42.7 ± 1.0 kg/m2] and 7 non-obese control subjects [7 women; mean age 45 years (range 33–55 years) and BMI = 22.7 ± 0.6 kg/m2 without other comorbidities]. The obese group was divided into two subgroups: (i) a group not undergoing BS (non-surgery group, 14 patients) and (ii) a group undergoing BS (pre-surgery group, 17 patients). The pre-surgery group was studied before and 12 months after BS. All pre-surgery patients underwent bariatric surgery [BS, elective laparoscopic bypass (53%) or sleeve gastrectomy (47%)] in the same center. The main inclusion criteria for BS was the presentation of more severe comorbidities (poorly controlled diabetes, ischemic heart disease, renal insufficiency or muscular neurological diseases) than in the non-surgery group. Patients were submitted to BS in chronologic order. Patients in the non-surgery group did not undergo BS because they rejected it for personal reasons and they preferred to receive counseling on lifestyle modifications (hypocaloric diet and exercise). They did not receive any pharmacological treatment for losing weight. Exclusion criteria were any past or present history of benign or malignant tumor, an inflammatory or autoimmune disorder and acute infections. Blood samples were obtained before BS and at 12 months follow-up. Information regarding anthropometric, clinical data and laboratory parameters was collected and included: age, body weight (BW), body mass index (BMI), waist circumference (WC), systolic (SBP), and diastolic blood pressure (DBP), complete hemogram, lipid profile, fasting glucose, glycosylated hemoglobin (HbA1c), insulin, homeostasis model assessment of insulin resistance index (HOMA-IR), C-reactive protein (CRP), lactate dehydrogenase (LDH), carbonyls, and uric acid (UA) levels. Small samples of omental adipose tissue were obtained from patients who underwent BS during the intervention. In addition, detailed clinical information was collected, including the patients’ comorbidities and medication. This study was approved by the Ethics Committee of the Hospital Clínico San Carlos and Universidad San Pablo-CEU and was conducted in compliance with the Helsinki Declaration. All patients signed a written informed consent form before they were included in the study.
Blood Sample Collection
Blood samples from obese and non-obese subjects were collected under fasting conditions in citrated-BD Vacutainer Plus plastic serum tubes (Plymouth, United Kingdom). A total of 1 ml of blood was centrifuged at 3000 rpm for 10 min at 4°C to obtain plasma. A total of 5 ml of blood were diluted with PBS (pH = 7.4) (1:1 ratio) and submitted to a Ficoll-Hypaque (Sigma-Aldrich, United States) density gradient. After centrifugation at 1800 rpm for 30 min at 4°C, the mononuclear cell interface was collected, washed with PBS and centrifuged at 1200/1500 rpm for 5 min at 4°C. Both plasma and peripheral blood mononuclear cells (PBMCs) were frozen at -80°C until assay.
Western Blot Analysis
Protein expression was determined in PBMCs by Western blot, as previously described (Somoza et al., 2007). Briefly, 20 μg protein samples were separated by SDS-PAGE gels. Primary antibodies anti p-AMPKα (Thr172) and AMPKα (1:1000 final dilution; Cell Signaling Biotechnology, Germany) and SIRT-1 (D1D7) (1:1000 final dilution; Cell Signaling Technology, United States) were applied overnight at 4°C. After washing, appropriate secondary antibodies (anti-rabbit or anti-mouse IgG-peroxidase conjugated) were applied for 1 h (1:5000 final dilution). Blots were washed, incubated in commercial enhanced chemiluminescence reagents (ECL Prime, Amersham Bioscience, United Kingdom) and bands were analyzed with the ChemiDoc XRS+ Imaging System (Bio-Rad, United States). To prove equal loading of samples, blots were incubated with β-actin antibody (1:5000 final dilution; Sigma-Aldrich, United States). Blots were quantified using Image Lab 3.0 software (Bio-Rad, United States). Expression values of p-AMPKα and SIRT-1 were normalized with AMPKα and β-actin, respectively.
MMP-2 and MMP-9 Activity in Plasma
MMP-2 and MMP-9 gelatinase activity was determined by gelatin zymography. Total protein concentration was measured with the Bradford method (Bradford, 1976). Human plasma samples were diluted 1:1 with sample buffer and subjected to SDS-polyacrylamide gel electrophoresis (PAGE) containing 0.1% gelatin. Subsequently, gels were renatured and incubated for 12 h at 37°C. Gels were stained with Coomassie blue (Bio-Rad, United States) and, after destaining, gelatinolytic activity of MMP-2 and MMP-9 was detected as transparent bands against the background of the blue-stained gels. Transparent bands are the result of the digestion of gelatin, the substrate incorporated in polyacrylamide gel, because of enzymatic activity of MMP-2 and MMP-9. Bands were quantified using Image Lab 3.0 software (Bio-Rad, United States). The active MMP-9 form was determined using a commercial kit and following the manufacturer’s specifications (QuickZyme Biosciences, Netherlands).
Plasma Carbonyls Analysis
Circulating carbonyl levels, as an index of oxidative marker on proteins, were assessed in plasma samples using the 2, 4-dinitrophenylhydrazine (DNPH)-based assay adapted to a microplate reader (Hawkins et al., 2009; Ruiz-Hurtado et al., 2014). Total protein carbonyls concentration was measured using the extinction coefficient of DNPH (ε = 22,000/mol/cm) and expressed as nmol/mg protein.
Study of Vascular Mechanical Properties
Omental arteries were dissected from samples of omental adipose tissue from patients undergoing BS and were carefully cleaned of their surrounding adipose tissue. Mechanical properties were analyzed with a pressure myograph (Model P100, Danish Myo-Tech), as previously described (Briones et al., 2003; Gil-Ortega et al., 2016). Briefly, intraluminal pressure was set for equilibration at 70 mmHg for 30 min at 37°C in calcium-free KH (0Ca2+; omitting calcium and adding 10 mM EGTA) and bubbled with carbogen (95% O2/5% CO2). Afterwards, intraluminal pressure was augmented at 20 mmHg intervals (5, 20, 40, 60, 80, 100, 120, and 140 mmHg) and both external and internal diameters (Di0Ca, De0Ca) were recorded and analyzed with Myoview software. From the De0Ca and Di0Ca values, we calculated: (i) the circumferential wall strain (ε) = (Di0Ca - D00Ca)/D00Ca, where D00Ca is the diameter at 3 mmHg and Di0Ca is the observed internal diameter for a given intravascular pressure in 0Ca2+ KH and (ii) the circumferential wall stress (β = (P × Di0Ca)/(2WT)), where P is the intraluminal pressure (1 mmHg = 1.334 dynes cm-2) (Briones et al., 2003). The stiffness index β, obtained from the stress-strain relationship, is proportional to Young’s incremental elastic modulus and is used to assess the intrinsic arterial stiffness independently of vessel geometry (Dobrin, 1978).
Statistical Analysis
Continuous variables were compared using the Student’s t-test or one-way analysis of variance (ANOVA) following Newman–Keuls test. Data are reported as mean ± SEM. Correlation analyses were performed through linear regression and analyzed by Pearson’s correlation. All statistical analyses were performed using Graph-Pad Prism 7.0 or Origin 9.0 software. Statistical significance was set at P < 0.05.
Results
Clinical Characteristics
Anthropometric and clinical parameters at baseline and at 12 months follow-up are summarized in Table 1. At baseline, the obese group, especially the pre-surgery group, exhibited a higher number of CV risk factors than non-obese subjects, who did not present any comorbidity. In the pre-surgery group, 14 (82.4%) patients were diagnosed with type 2 diabetes mellitus (T2DM, 76.5% treated with metformin therapy), 10 (58.8%) with hypertension (all treated with either an ACEi or ARB) and 11 (64.7%) with dyslipidemia (all treated with statins). In the non-surgery group, no patient was diagnosed with T2DM, 8 (57.1%) patients were diagnosed with hypertension (all treated with ACEi or ARB) and 9 (64.3%) with dyslipidemia (all treated with statins).
Anthropometric parameters including BW, BMI and WC were significantly higher in obese patients than in controls (P < 0.001, Table 1) and significantly reduced 12 months after BS (% of reduction: BW = 29.7%; BMI = 31.6%; and WC = 28.5% in female and 25.8% in male) compared with their corresponding pre-surgery values (P < 0.001 in all cases). BS significantly reduced fasting glucose level and improved insulin sensitivity (HOMA-IR) (P < 0.001). Lower HDL-cholesterol and higher triglyceride (TG) levels were detected in obese patients than in the control group. However, although these parameters were not significantly modified by BS, a reduction in TG levels was observed (P = 0.068 vs. obese). SBP and DBP were similar between groups, which shows proper blood pressure control in obese patients treated with ACEi/ARBs. In addition, as shown in Table 1, the intake of antihypertensive drugs was reduced after BS.
Since no differences were detected between non-surgery and pre-surgery groups in carbonyls, UA, LDH, inflammatory markers, AMPK and MMP-9 activities and SIRT-1 levels (data not shown), these parameters were analyzed altogether, and we will refer to them as the obese group.
Bariatric Surgery Significantly Reduces Protein Oxidative Damage and Inflammatory Markers/Cells in Obese Patients
Levels of plasma carbonyls (Figure 2A; P < 0.001) and UA (Figure 2B; P < 0.05), were significantly higher in obese patients than in controls. After BS, carbonyls and UA levels were significantly reduced (P < 0.05 vs. obese) and correlated positively with BMI (r = 0.633 and P < 0.001; Figure 2C and r = 0.408 and P < 0.01; Figure 2D, respectively).
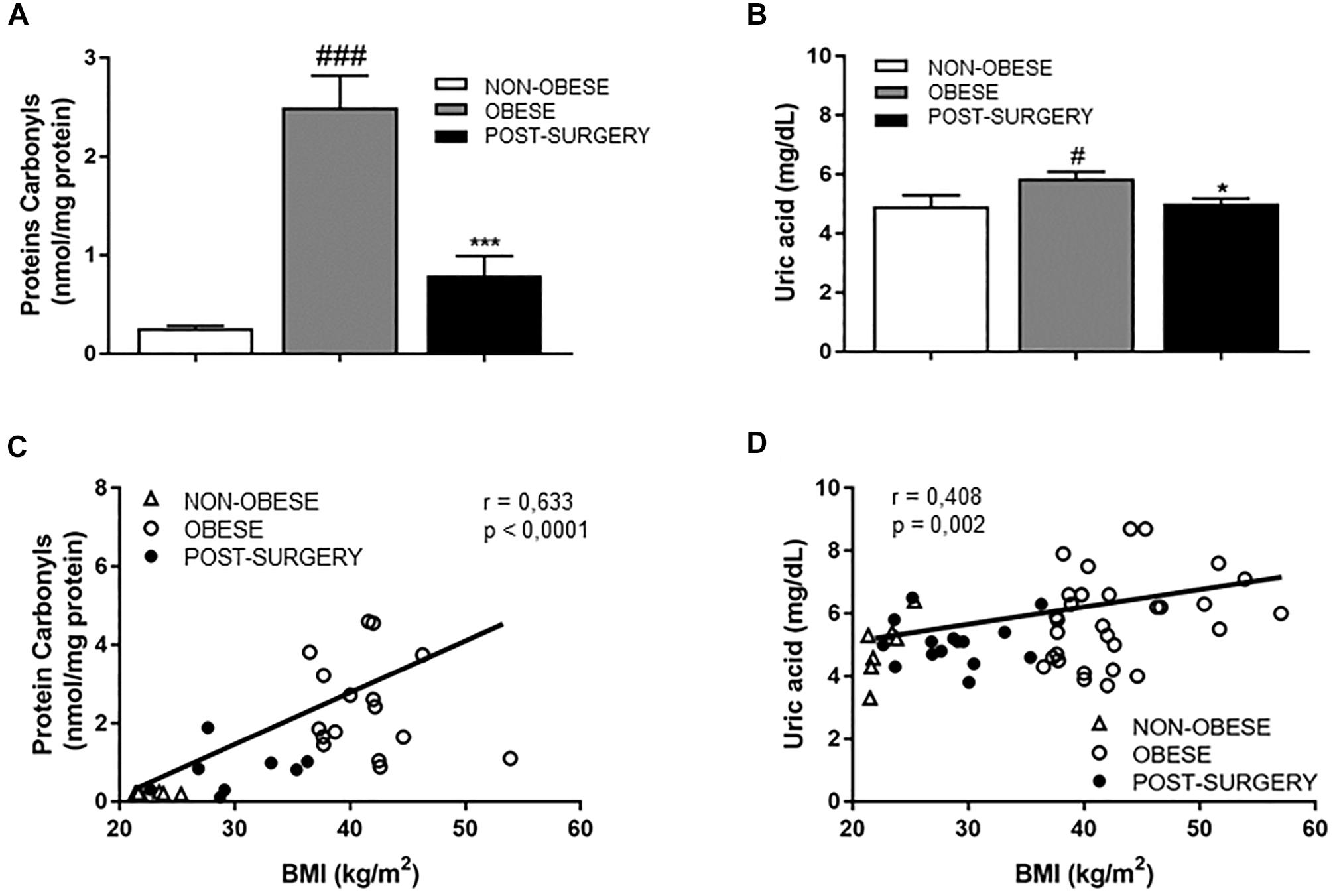
Figure 2. (A,B) Diagram bars shows protein carbonyls (A) and uric acid (UA) concentrations (B) in plasma samples from non-obese (white bar, n = 7), obese (gray bar, n = 31) and post-surgery (black bar, n = 17) patients. (C) Correlation between protein carbonyl concentrations in plasma and BMI. (D) Correlation between plasmatic UA levels and BMI. White triangles correspond to non-obese, white circles correspond to obese and black circles correspond to Post-surgery. Data are expressed as mean ± SEM. #p < 0.05 and ###p < 0.001 compared with the non-obese group. ∗p < 0.05 and ∗∗∗p < 0.001 compared with the obese group.
As shown in Table 2, CRP was over sixfold higher in obese patients than in the non-obese group (P < 0.01), but significantly reduced after BS (P < 0.05). We also quantified in blood samples the cell number of neutrophils, eosinophils, monocytes and lymphocytes. Immune cell number was similar in obese and control subjects. Post-surgery patients exhibited a significant reduction in the amount of these immune cells, except for eosinophils (P < 0.05 vs. obese; Table 2). Simple regression analysis revealed a positive correlation between total leukocytes, lymphocytes, neutrophils, monocytes or eosinophils and BMI (Figure 3).
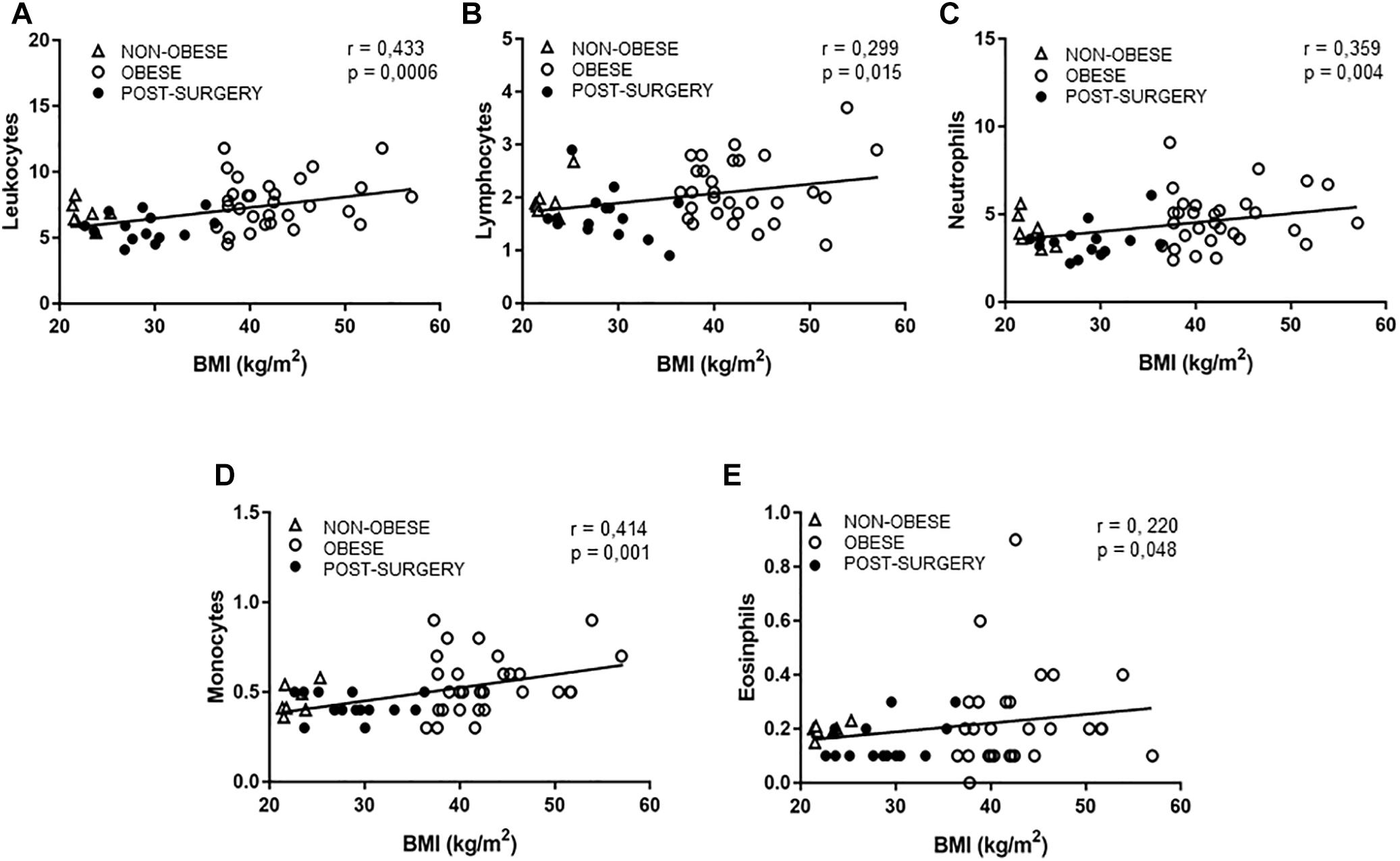
Figure 3. (A–E) Correlations between BMI and total leukocytes (A), lymphocytes (B), neutrophils (C), monocytes (D), and eosinophils (E). White triangles correspond to non-obese, white circles correspond to obese and black circles correspond to Post-surgery.
Bariatric Surgery Improves Both AMPK Activity and SIRT-1 Expression in PBMCs From Obese Patients
p-AMPKαThr172 levels (Figure 4A) were significantly lower in PBMCs from obese patients than in those from the control group (P < 0.01) but were restored to normal values 12 months after surgery (P < 0.001 vs. obese levels). The negative correlation between p-AMPKαThr172 and BMI (Figure 4B; r = 0.655, P < 0.001) evidences greater AMPK activity in post-surgery patients with a lower BMI than in obese patients with a higher BMI. A negative correlation was observed between p-AMPKαThr172 and plasma carbonyls (r = -0.709 and P < 0.001).
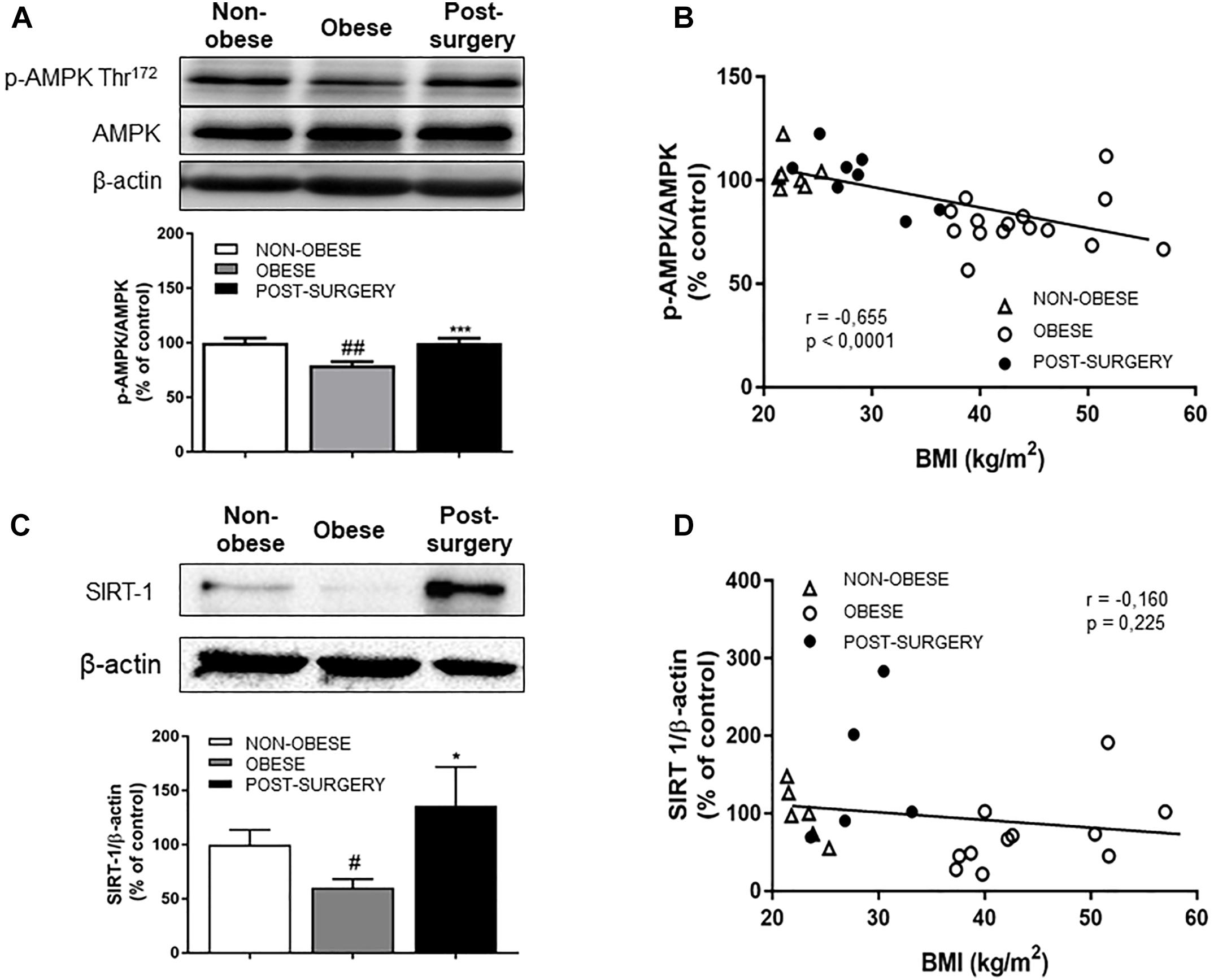
Figure 4. (A) Representative immunoblots of p-AMPK/AMPK and β-actin expression in PBMCs from non-obese, obese and post-surgery patients. Diagram bars show the result of densitometric analysis of p-AMPK/AMPK immunoblots expressed as a percentage of p-AMPK/AMPK in the non-obese group. (B) Correlation between p-AMPK/AMPK and BMI. (C) Representative immunoblots of SIRT1 and β-actin expression in PBMCs from non-obese, obese and post-surgery patients. Diagram bars show the result of densitometric analysis of SIRT1/β-actin immunoblots expressed as a percentage of SIRT1/β-actin in the non-obese group. (D) Correlation between SIRT1/β-actin and BMI. Data are expressed as mean ± SEM of ≥ 7 determinations per group. #p < 0.05 and ##p < 0.01 compared with the non-obese group. ∗p < 0.05 and ∗∗∗p < 0.001 compared with the obese group.
SIRT-1 expression was significantly lower in obese patients (P < 0.05 compared with controls) but reached normal values after BS (P < 0.05; Figure 4C). There was no correlation between SIRT-1 expression and BMI (Figure 4D; r = -0.16, P = 0.225).
Bariatric Surgery Significantly Reduces MMP-9 Activity and Lactate Dehydrogenase Levels in Plasma From Obese Patients
Gelatinase MMP-2 activity was similar in obese and non-obese subjects, but significantly lower after BS (Figure 5A, P < 0.05 vs. obese). Gelatinase MMP-9 activity was significantly higher in obese patients than in controls (Figure 5B; P < 0.01) and normalized after BS (P < 0.001 vs. obese). Likewise, specific measurement of the active MMP-9 form by ELISA revealed a significant reduction in active MMP-9 levels after BS (obese = 0.205 ± 0.01; Post-surgery = 0.153 ± 0.02; P < 0.01), which positively correlated with BMI (Figure 5C; r = 0.527, P < 0.001).
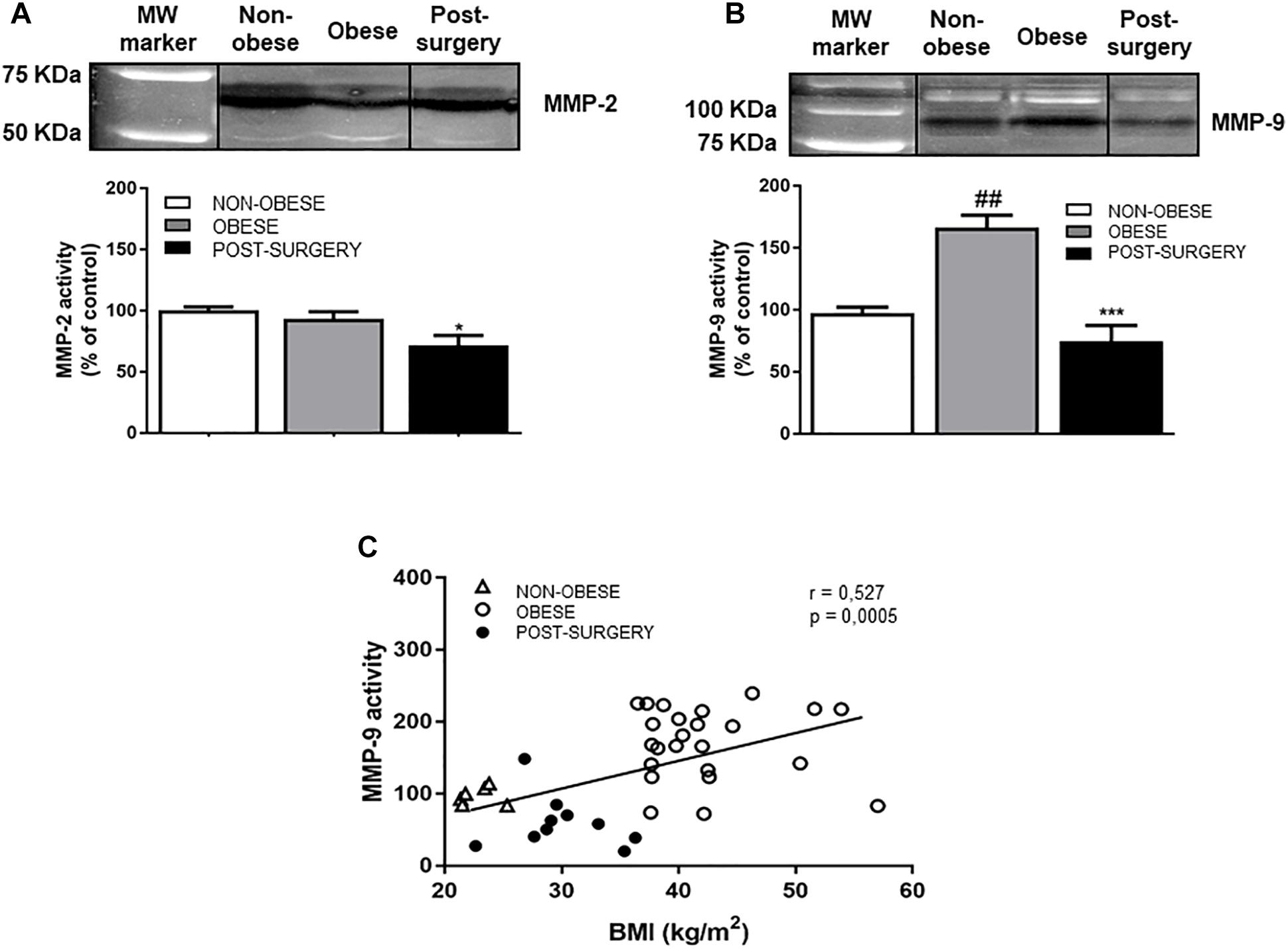
Figure 5. (A) Representative cropped immunoblots of MMP-2 activity in plasma samples from non-obese, obese and Post-surgery patients. Diagram bars show the result of densitometric analysis of MMP-2 activity expressed as a percentage of MMP-2 activity in the non-obese group. (B) Representative cropped immunoblots of MMP-9 activity in plasma samples from non-obese, obese and Post-surgery patients. Diagram bars show the result of densitometric analysis of MMP-9 activity expressed as a percentage of MMP-9 activity in the non-obese group. (C) Correlation between MMP-9 activity and BMI. White triangles correspond to non-obese, white circles correspond to obese and black circles correspond to Post-surgery. Data are expressed as mean ± SEM of ≥ 7 determinations per group. ##p < 0.01 compared with the non-obese group. ∗p < 0.05 and ∗∗∗p < 0.001 compared with the obese group.
There was also a positive correlation between plasmatic MMP-9 activity and protein carbonyls (r = 0.630, P < 0.001), and with leukocyte and neutrophil number (r = 0.300 and P < 0.05 and r = 0.298 and P < 0.05, respectively).
Lactate dehydrogenase (LDH) was significantly higher in obese patients than in controls (P < 0.001) and significantly diminished in the Post-surgery group (Figure 6A, P < 0.01 vs. obese). A positive correlation was observed between LDH levels and BMI (Figure 6B; r = 0.547, P < 0.001).
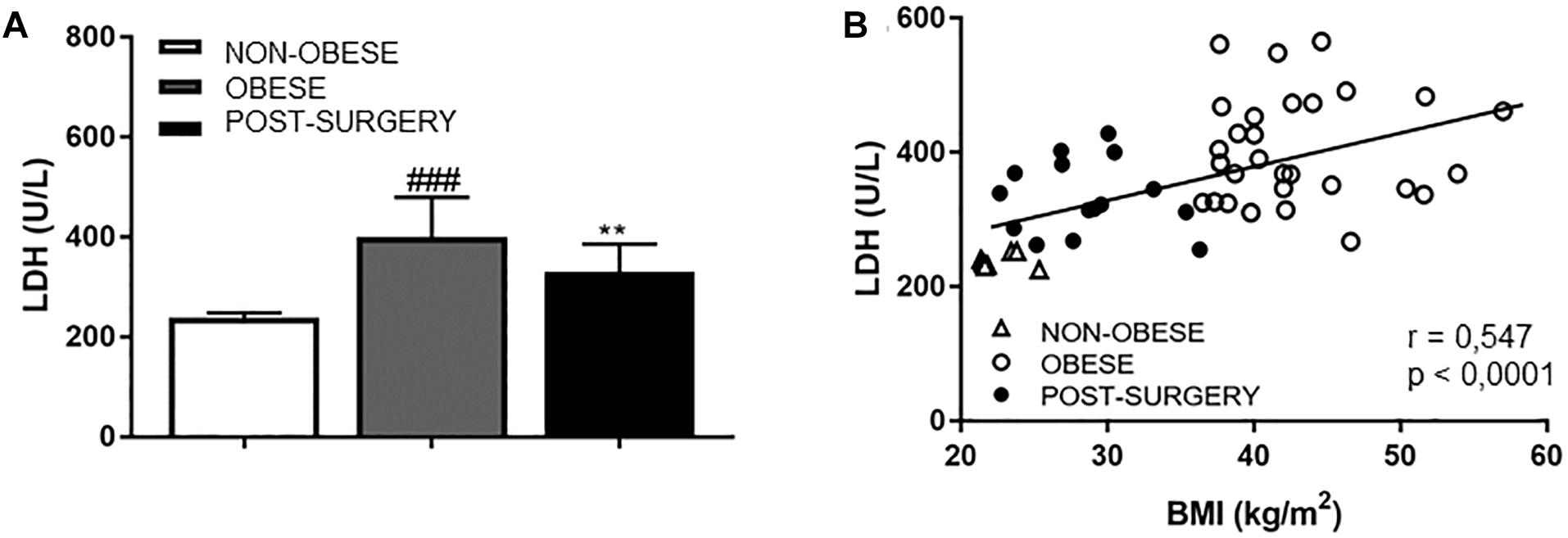
Figure 6. (A) Diagram bars shows LDH concentrations in plasma samples from non-obese, obese and Post-surgery patients. Data are expressed as mean ± SEM of ≥7 determinations per group. ###p < 0.001 compared with the non-obese group. ∗∗p < 0.01 compared with the obese group. (B) Correlation between LDH concentrations and BMI. White triangles correspond to non-obese, white circles correspond to obese and black circles correspond to Post-surgery.
Mechanical studies performed in omental arteries from obese patients evidenced a stiffness index β of 13.9 ± 2.5 (n = 11), as an index of intrinsic arterial stiffness. In obese patients, we found a significant correlation between LDH and the stiffness index β (r = 0.829, P < 0.001).
Discussion
The study demonstrates that both abnormal plasma MMP-9 and PBMCs AMPK activities are reversed by BS. Interestingly, we found a positive correlation between leukocyte and neutrophil number and MMP-9 activity. Moreover, we describe for the first time a relationship between plasmatic LDH levels and the stiffness index β, as a marker of intrinsic arterial stiffness in omental arteries from morbid obese patients, and a correlation between AMPK and MMP-9 activity and LDH values. Finally, as BS has been shown to effectively normalize all these parameters, we suggest that plasma MMP-9 and PBMCs AMPK activities might potentially be used as markers of cardiovascular risk in patients with morbid obesity.
Obesity is an established risk factor for several CVDs and it may be associated with early vascular alterations. The presence of classic CV risk factors (T2DM, hyperlipidemia and hypertension) in obese subjects increases the risk of accelerating arterial stiffness, especially if obesity appears at early ages (Wildman et al., 2003; Woo et al., 2004; Sato et al., 2005; Safar et al., 2006). In our study, the mean age of obese subjects was 46 years and over 70% of them already presented CV risk factors. Insulin resistance and a low-grade inflammatory state are common physiopathological mechanisms in obesity and T2DM. Therefore, we did not detect differences in arterial stiffness-related parameters between obese groups (non-surgery vs. pre-surgery). Moreover, 11 of 17 patients already exhibited an elevated intrinsic stiffness in omental arteries, as shown by stiffness index β over 10.2, which indicates pathological stiffness in this group (Sato et al., 2013) and is a predictor of all-cause mortality (Willum-Hansen et al., 2006; Mitchell et al., 2010; Vlachopoulos et al., 2010). Previous studies also evidenced increased arterial stiffness in obese patients (Dangardt et al., 2013; Pal and Radavelli-Bagatini, 2013; Arner et al., 2015; Cote et al., 2015; Strasser et al., 2015; Manco et al., 2017) assessed by the determination of either the PWV or the augmentation index; parameters that are dependent on blood pressure levels and markers of systemic but not intrinsic arterial stiffness (Matsui et al., 2004). In addition, Savoia et al. (2008), have described an increased stiffness index β in gluteal subcutaneous arteries from hypertensive patients compared to normotensive subjects, thus suggesting a relationship between an increased stiffness and BP in hypertension. Moreover, a recent study from our group performed in an animal model of diet-induced obesity has also shown an association between the stiffness index β in mesenteric resistance arteries and PWV (Gil-Ortega et al., 2016). Nevertheless, a relationship between intrinsic stiffness of omental arteries stated by the stiffness index β and PWV has not been clearly stated yet. Nevertheless, though no direct comparison can be established with our results, changes in intrinsic arterial stiffness precede changes in systemic arterial stiffness (Sehgel et al., 2013). The early identification of patients with altered intrinsic stiffness might thus be key for risk assessment and the prevention of further CV damage.
Several pathophysiological mechanisms like chronic low-grade inflammation and immune activation, oxidative stress, and ECM remodeling are involved in the interaction between obesity and arterial stiffness (Leopold, 2013; Aroor et al., 2018). Moreover, a strong association has been described in women between obesity and increased total lymphocyte counts and white blood cells (Womack et al., 2007). Our obese patients had very high CRP levels, but reached normal levels after BS. CRP directly induces endothelial dysfunction through a reduction in nitric oxide production (Venugopal et al., 2002) and favors oxidative stress. This is in accordance with the oxidative protein damage and increased UA levels observed in the obese group. UA is considered a powerful scavenger of free radicals, which are increased in patients with obesity and insulin resistance, probably as a compensatory mechanism to protect against oxidative damage (Fabbrini et al., 2014). The fact that these parameters reach normal values is in accordance with the beneficial effect of BS on inflammatory and oxidative stress status (Kopp et al., 2005; Sheu et al., 2008). Moreover, there is a reduction in lymphocytes, leukocytes, neutrophils and monocytes in the hemogram of obese patients after BS that correlates with BMI.
Inflammation may induce elastin degradation and changes in the ECM composition through MMP (MMP-2 and MMP-9) activation, thus resulting in an accumulation of stiffer, uncoiled collagen (Galis and Khatri, 2002). Similarly to other studies, our obese patients show a significant increase in MMP-9 activity (Laimer et al., 2005; Derosa et al., 2008; Madsen et al., 2009) that correlates with BMI. Under the influence of pro-inflammatory cytokines, neutrophils induce MMP-9 activity and contribute to microvascular complications (Galis and Khatri, 2002). In this context, we observed a positive, significant relationship in obese patients between neutrophil number and MMP-9 activity, which suggests that neutrophil number may be highlighted as a marker of ECM remodeling. Moreover, the positive correlation between MMP-9 activity and circulating carbonyl levels in obese patients is consistent with the traditional concept that MMP-9 expression is inducible through several mechanisms, the most important of which is the action of reactive oxygen species (ROS) (Kuhad et al., 2015). Pleiotropic effects of statins include the reduction of MMP-9 activity and other inflammation markers (Guerrero-Romero and Rodriguez-Moran, 2005). Therefore, an effect of the treatment on these parameters in addition to BS cannot be excluded.
One of the most interesting results of our study is the increased LDH level in obese patients that is reduced after BS and correlates with BMI. Nutritional diseases such as obesity have been associated with changes in mitochondrial oxidative phosphorylation and changes in the phenotype of vascular smooth muscle cells (VSMCs) (Chiong et al., 2014). The correlation between LDH and the stiffness index β might suggest an association between LDH and arterial stiffness associated with obesity.
Lymphocyte mitochondrial physiology seems to be associated with nutritional status (Briet et al., 2003) and other cell type metabolic alterations. Therefore, it might be considered an interesting biomarker of metabolic status (Cortez et al., 2012). In this context, we used PBMCs to determine both AMPK activity and SIRT-1 expression in controls vs. obese vs. Post-surgery patients. AMPK is a metabolic sensor. It reduces inflammation, oxidative stress and vascular remodeling (Garcia-Prieto et al., 2015a) and is dysregulated in obesity (Gauthier et al., 2011; Xu et al., 2012). In accordance with these studies, we detected a significant reduction in AMPK activity in obese patients that was reversed by BS. Notably, AMPK activation also favors the switch of the synthetic phenotype of VSMCs toward a contractile phenotype (Stone et al., 2013). SIRT-1 can activate AMPK but also has wide-ranging effects on metabolic homeostasis, mainly as a regulator of mitochondrial integrity and by reducing inflammatory and oxidative stress tone (Kida and Goligorsky, 2016). In our study, we found an increase in SIRT-1 expression after BS. Moreover, since it has been suggested that SIRT-1 plays a key role in CV protection, SIRT-1 overexpression could contribute to reducing CV risk after BS.
Altogether, these data demonstrate that alterations in both MMP-9 and AMPK activities might play a key role in the development of arterial stiffness. In addition, other factors that are altered in obesity like LDH, SIRT-1 expression, oxidative stress markers or immune disorders could also contribute to vascular damage.
Conclusion
A limitation of this study is that we did not measure intrinsic arterial stiffness in omental arteries after BS for obvious ethical reasons. Thus, we cannot conclude that modification in abnormal AMPK, LDH, and MMP-9 activities has an impact on intrinsic arterial stiffness in these patients. Further studies are required to answer this question. Nonetheless, several studies have demonstrated the relationship between weight loss (i.e., after lifestyle modifications or surgical interventions) and the reduction of arterial stiffness in both obese animal models and morbid obese patients (Shargorodsky et al., 2006; Rider et al., 2010; Weisbrod et al., 2013; Brunner et al., 2015; Hudson et al., 2017; Manco et al., 2017; Backdahl et al., 2018). In conclusion, since pAMPK, LDH and MMP-9 activities might be easily measured in blood samples, they could be systematically used as potential markers of CV risk and allow for the early identification of patients with an altered intrinsic stiffness and the prevention of further CV damage.
Ethics Statement
This study was carried out in accordance with the recommendations of the Ethics Committee of the Hospital Clínico San Carlos. The protocol was approved by the Ethics Committee of the Hospital Clínico San Carlos.
Author Contributions
CG-P, MG-O, IA, MF-A, and BS participated in the design of the study, the analysis and interpretation of the results obtained, and the main manuscript writing. CG-P and MM-R performed Western blot and gelatin zymography analyses. MG-O performed active MMP-9 determination. DR-C participated in carbonyl determination. EV-M and MG-O performed mechanical studies in omental arteries. EB, AT, AS-P, and MR provided human samples, clinical data, and plasmatic parameters. MF-A, BS, IA, and MR ensured funding. All authors reviewed the manuscript.
Funding
This work has been supported by Fundación Mutua Madrileña, GR921645-Santander, SESCAMET, BFU2011–25303, FUSP-CEU.
Conflict of Interest Statement
The authors declare that the research was conducted in the absence of any commercial or financial relationships that could be construed as a potential conflict of interest.
Abbreviations
AMPK, AMP-dependent protein kinase; BMI, body mass index; BS, bariatric surgery; BW, body weight; CRP, C reactive protein; CVD, cardiovascular disease; DBP, diastolic blood pressure; ECM, extracellular matrix; HOMA-IR, insulin resistance index; LDH, lactate dehydrogenase; MMP, matrix metalloproteinases; ROS, reactive oxygen species; SBP, systolic blood pressure; SIRT, sirtuin; UA, uric acid; VSMCs, vascular smooth muscle cells; WC, waist circumference.
References
Andrade, V. L., Petruceli, E., Belo, V. A., Andrade-Fernandes, C. M., Caetano Russi, C. V., Bosco, A. A., et al. (2012). Evaluation of plasmatic MMP-8, MMP-9, TIMP-1 and MPO levels in obese and lean women. Clin. Biochem. 45, 412–415. doi: 10.1016/j.clinbiochem.2012.01.008
Arner, P., Backdahl, J., Hemmingsson, P., Stenvinkel, P., Eriksson-Hogling, D., Naslund, E., et al. (2015). Regional variations in the relationship between arterial stiffness and adipocyte volume or number in obese subjects. Int J. Obes. 39, 222–227.
Aroor, A. R., Jia, G., and Sowers, J. R. (2018). Cellular mechanisms underlying obesity-induced arterial stiffness. Am. J. Physiol. Regul. Integr. Comp. Physiol. 314, R387–R398. doi: 10.1152/ajpregu.00235.2016
Backdahl, J., Andersson, D. P., Eriksson-Hogling, D., Caidahl, K., Thorell, A., Mileti, E., et al. (2018). Long-term improvement in aortic pulse wave velocity after weight loss can be predicted by white adipose tissue factors. Am. J. Hypertens. 31, 450–457. doi: 10.1093/ajh/hpx201
Bouissou, C., Lacolley, P., Dabire, H., Safar, M. E., Gabella, G., Duchatelle, V., et al. (2014). Increased stiffness and cell-matrix interactions of abdominal aorta in two experimental non-hypertensive models: long-term chemically sympathectomized and sinoaortic denervated rats. J. Hypertens. 32, 652–658. doi: 10.1097/HJH.0000000000000073
Bradford, M. M. (1976). A rapid and sensitive method for the quantitation of microgram quantities of protein utilizing the principle of protein-dye binding. Anal. Biochem. 72, 248–254.
Briet, F., Twomey, C., and Jeejeebhoy, K. N. (2003). Relationship between metabolism and peripheral blood mononuclear cell mitochondrial complex I activity before and after a short-term refeeding in weight-losing cancer patients. Clin. Nutr. 22, 247–253.
Briones, A. M., Gonzalez, J. M., Somoza, B., Giraldo, J., Daly, C. J., Vila, E., et al. (2003). Role of elastin in spontaneously hypertensive rat small mesenteric artery remodelling. J. Physiol. 552, 185–195.
Brunner, E. J., Shipley, M. J., Ahmadi-Abhari, S., Tabak, A. G., Mceniery, C. M., Wilkinson, I. B., et al. (2015). Adiposity, obesity, and arterial aging: longitudinal study of aortic stiffness in the Whitehall II cohort. Hypertension 66, 294–300. doi: 10.1161/HYPERTENSIONAHA.115.05494
Chen, D., Bruno, J., Easlon, E., Lin, S. J., Cheng, H. L., Alt, F. W., et al. (2008). Tissue-specific regulation of SIRT1 by calorie restriction. Genes Dev. 22, 1753–1757. doi: 10.1101/gad.1650608
Chen, K., Zhou, X., and Sun, Z. (2015). Haplodeficiency of klotho gene causes arterial stiffening via upregulation of scleraxis expression and induction of autophagy. Hypertension 66, 1006–1013. doi: 10.1161/HYPERTENSIONAHA.115.06033
Chiong, M., Cartes-Saavedra, B., Norambuena-Soto, I., Mondaca-Ruff, D., Morales, P. E., Garcia-Miguel, M., et al. (2014). Mitochondrial metabolism and the control of vascular smooth muscle cell proliferation. Front. Cell Dev. Biol. 2:72. doi: 10.3389/fcell.2014.00072
Civitarese, A. E., Carling, S., Heilbronn, L. K., Hulver, M. H., Ukropcova, B., Deutsch, W. A., et al. (2007). Calorie restriction increases muscle mitochondrial biogenesis in healthy humans. PLoS Med. 4:e76.
Cohen, H. Y., Miller, C., Bitterman, K. J., Wall, N. R., Hekking, B., Kessler, B., et al. (2004). Calorie restriction promotes mammalian cell survival by inducing the SIRT1 deacetylase. Science 305, 390–392.
Cortez, E., Neves, F. A., Bernardo, A. F., Stumbo, A. C., Carvalho, L., Garcia-Souza, E., et al. (2012). Lymphocytes mitochondrial physiology as biomarker of energy metabolism during fasted and fed conditions. ScientificWorldJournal 2012:629326. doi: 10.1100/2012/629326
Cote, A. T., Phillips, A. A., Harris, K. C., Sandor, G. G., Panagiotopoulos, C., and Devlin, A. M. (2015). Obesity and arterial stiffness in children: systematic review and meta-analysis. Arterioscler. Thromb. Vasc. Biol. 35, 1038–1044. doi: 10.1161/ATVBAHA.114.305062
Dammanahalli, J. K., and Sun, Z. (2008). Endothelin (ET)-1 inhibits nicotinamide adenine dinucleotide phosphate oxidase activity in human abdominal aortic endothelial cells: a novel function of ETB1 receptors. Endocrinology 149, 4979–4987. doi: 10.1210/en.2008-0199
Dangardt, F., Chen, Y., Berggren, K., Osika, W., and Friberg, P. (2013). Increased rate of arterial stiffening with obesity in adolescents: a five-year follow-up study. PLoS One 8:e57454. doi: 10.1371/journal.pone.0057454
Derosa, G., Ferrari, I., D’angelo, A., Tinelli, C., Salvadeo, S. A., Ciccarelli, L., et al. (2008). Matrix metalloproteinase-2 and -9 levels in obese patients. Endothelium 15, 219–224. doi: 10.1080/10623320802228815
Fabbrini, E., Serafini, M., Colic Baric, I., Hazen, S. L., and Klein, S. (2014). Effect of plasma uric acid on antioxidant capacity, oxidative stress, and insulin sensitivity in obese subjects. Diabetes 63, 976–981. doi: 10.2337/db13-1396
Flamant, M., Placier, S., Dubroca, C., Esposito, B., Lopes, I., Chatziantoniou, C., et al. (2007). Role of matrix metalloproteinases in early hypertensive vascular remodeling. Hypertension 50, 212–218.
Fonck, E., Feigl, G. G., Fasel, J., Sage, D., Unser, M., Rufenacht, D. A., et al. (2009). Effect of aging on elastin functionality in human cerebral arteries. Stroke 40, 2552–2556. doi: 10.1161/STROKEAHA.108.528091
Fruhbeck, G. (2015). Bariatric and metabolic surgery: a shift in eligibility and success criteria. Nat. Rev. Endocrinol. 11, 465–477. doi: 10.1038/nrendo.2015.84
Fry, J. L., Al Sayah, L., Weisbrod, R. M., Van Roy, I., Weng, X., Cohen, R. A., et al. (2016). Vascular smooth muscle sirtuin-1 protects against diet-induced aortic stiffness. Hypertension 68, 775–784. doi: 10.1161/HYPERTENSIONAHA.116.07622
Galis, Z. S., and Khatri, J. J. (2002). Matrix metalloproteinases in vascular remodeling and atherogenesis: the good, the bad, and the ugly. Circ. Res. 90, 251–262.
Gano, L. B., Donato, A. J., Pasha, H. M., Hearon, CM Jr, Sindler, A. L., and Seals, D. R. (2014). The SIRT1 activator SRT1720 reverses vascular endothelial dysfunction, excessive superoxide production, and inflammation with aging in mice. Am. J. Physiol. Heart Circ. Physiol. 307, H1754–H1763. doi: 10.1152/ajpheart.00377.2014
Garcia-Prieto, C. F., Gil-Ortega, M., Aranguez, I., Ortiz-Besoain, M., Somoza, B., and Fernandez-Alfonso, M. S. (2015a). Vascular AMPK as an attractive target in the treatment of vascular complications of obesity. Vascul. Pharmacol. 67-69, 10–20. doi: 10.1016/j.vph.2015.02.017
Garcia-Prieto, C. F., Hernandez-Nuno, F., Rio, D. D., Ruiz-Hurtado, G., Aranguez, I., Ruiz-Gayo, M., et al. (2015b). High-fat diet induces endothelial dysfunction through a down-regulation of the endothelial AMPK-PI3K-Akt-eNOS pathway. Mol. Nutr. Food Res. 59, 520–532. doi: 10.1002/mnfr.201400539
Garcia-Prieto, C. F., Pulido-Olmo, H., Ruiz-Hurtado, G., Gil-Ortega, M., Aranguez, I., Rubio, M. A., et al. (2015c). Mild caloric restriction reduces blood pressure and activates endothelial AMPK-PI3K-Akt-eNOS pathway in obese Zucker rats. Vascul. Pharmacol. 65-66, 3–12. doi: 10.1016/j.vph.2014.12.001
Gauthier, M. S., O’brien, E. L., Bigornia, S., Mott, M., Cacicedo, J. M., Xu, X. J., et al. (2011). Decreased AMP-activated protein kinase activity is associated with increased inflammation in visceral adipose tissue and with whole-body insulin resistance in morbidly obese humans. Biochem. Biophys. Res. Commun. 404, 382–387. doi: 10.1016/j.bbrc.2010.11.127
Gil-Ortega, M., Martin-Ramos, M., Arribas, S. M., Gonzalez, M. C., Aranguez, I., Ruiz-Gayo, M., et al. (2016). Arterial stiffness is associated with adipokine dysregulation in non-hypertensive obese mice. Vascul. Pharmacol. 77, 38–47. doi: 10.1016/j.vph.2015.05.012
Grassi, G., and Diez, J. (2009). Obesity-related cardiac and vascular structural alterations: beyond blood pressure overload. J. Hypertens. 27, 1750–1752.
Grassi, G., Seravalle, G., Scopelliti, F., Dell’oro, R., Fattori, L., Quarti-Trevano, F., et al. (2010). Structural and functional alterations of subcutaneous small resistance arteries in severe human obesity. Obesity 18, 92–98. doi: 10.1038/oby.2009.195
Guerrero-Romero, F., and Rodriguez-Moran, M. (2005). Concordance between the 2005 international diabetes federation definition for diagnosing metabolic syndrome with the national cholesterol education program adult treatment panel III and the world health organization definitions. Diabetes Care 28, 2588–2589.
Hardie, D. G. (2007). AMP-activated/SNF1 protein kinases: conserved guardians of cellular energy. Nat. Rev. Mol. Cell. Biol. 8, 774–785.
Hawkins, C. L., Morgan, P. E., and Davies, M. J. (2009). Quantification of protein modification by oxidants. Free Radic. Biol. Med. 46, 965–988. doi: 10.1016/j.freeradbiomed.2009.01.007
Hudson, L., Kinra, S., Wong, I., Cole, T. J., Deanfield, J., and Viner, R. (2017). Is arterial stiffening associated with adiposity, severity of obesity and other contemporary cardiometabolic markers in a community sample of adolescents with obesity in the UK? BMJ Paediatr. Open 1:e000061. doi: 10.1136/bmjpo-2017-000061
Hue, L., and Rider, M. H. (2007). The AMP-activated protein kinase: more than an energy sensor. Essays Biochem. 43, 121–137.
Jaiswal, A., Chhabra, A., Malhotra, U., Kohli, S., and Rani, V. (2011). Comparative analysis of human matrix metalloproteinases: emerging therapeutic targets in diseases. Bioinformation 6, 23–30.
Kangas, P., Tikkakoski, A. J., Tahvanainen, A. M., Leskinen, M. H., Viitala, J. M., Kahonen, M., et al. (2013). Metabolic syndrome may be associated with increased arterial stiffness even in the absence of hypertension: a study in 84 cases and 82 controls. Metabolism 62, 1114–1122. doi: 10.1016/j.metabol.2013.02.009
Kida, Y., and Goligorsky, M. S. (2016). Sirtuins, cell senescence, and vascular aging. Can. J. Cardiol. 32, 634–641. doi: 10.1016/j.cjca.2015.11.022
Kopp, H. P., Krzyzanowska, K., Mohlig, M., Spranger, J., Pfeiffer, A. F., and Schernthaner, G. (2005). Effects of marked weight loss on plasma levels of adiponectin, markers of chronic subclinical inflammation and insulin resistance in morbidly obese women. Int. J. Obes. 29, 766–771.
Kuhad, A., Singh, P., and Chopra, K. (2015). Matrix metalloproteinases: potential therapeutic target for diabetic neuropathic pain. Expert Opin. Ther. Targets 19, 177–185. doi: 10.1517/14728222.2014.960844
Laimer, M., Kaser, S., Kranebitter, M., Sandhofer, A., Muhlmann, G., Schwelberger, H., et al. (2005). Effect of pronounced weight loss on the nontraditional cardiovascular risk marker matrix metalloproteinase-9 in middle-aged morbidly obese women. Int. J. Obes. 29, 498–501.
Lee, W. J., Song, K. H., Koh, E. H., Won, J. C., Kim, H. S., Park, H. S., et al. (2005). Alpha-lipoic acid increases insulin sensitivity by activating AMPK in skeletal muscle. Biochem. Biophys. Res. Commun. 332, 885–891.
Leopold, J. A. (2013). Cellular and molecular mechanisms of arterial stiffness associated with obesity. Hypertension 62, 1003–1004.
Madsen, E. L., Bruun, J. M., Skogstrand, K., Hougaard, D. M., Christiansen, T., and Richelsen, B. (2009). Long-term weight loss decreases the nontraditional cardiovascular risk factors interleukin-18 and matrix metalloproteinase-9 in obese subjects. Metabolism 58, 946–953. doi: 10.1016/j.metabol.2009.02.031
Manco, M., Nobili, V., Alisi, A., Panera, N., and Handberg, A. (2017). Arterial stiffness, thickness and association to suitable novel markers of risk at the origin of cardiovascular disease in obese children. Int. J. Med. Sci. 14, 711–720. doi: 10.7150/ijms.20126
Matsui, Y., Kario, K., Ishikawa, J., Eguchi, K., Hoshide, S., and Shimada, K. (2004). Reproducibility of arterial stiffness indices (pulse wave velocity and augmentation index) simultaneously assessed by automated pulse wave analysis and their associated risk factors in essential hypertensive patients. Hypertens. Res. 27, 851–857.
Mitchell, G. F., Hwang, S. J., Vasan, R. S., Larson, M. G., Pencina, M. J., Hamburg, N. M., et al. (2010). Arterial stiffness and cardiovascular events: the Framingham heart study. Circulation 121, 505–511. doi: 10.1161/CIRCULATIONAHA.109.886655
Morigami, H., Morioka, T., Yamazaki, Y., Imamura, S., Numaguchi, R., Asada, M., et al. (2016). Visceral adiposity is preferentially associated with vascular stiffness rather than thickness in men with type 2 diabetes. J. Atheroscler. Thromb. 23, 1067–1079. doi: 10.5551/jat.33399
Morris, M. J. (2008). Cardiovascular and metabolic effects of obesity. Clin. Exp. Pharmacol. Physiol. 35, 416–419. doi: 10.1111/j.1440-1681.2008.04912.x
Nemes, A., Gavaller, H., Csajbok, E., Forster, T., and Csanady, M. (2008). Obesity is associated with aortic enlargement and increased stiffness: an echocardiographic study. Int. J. Cardiovasc. Imaging 24, 165–171.
Pal, S., and Radavelli-Bagatini, S. (2013). Association of arterial stiffness with obesity in Australian women: a pilot study. J. Clin. Hypertens. 15:304.
Pfluger, P. T., Herranz, D., Velasco-Miguel, S., Serrano, M., and Tschop, M. H. (2008). Sirt1 protects against high-fat diet-induced metabolic damage. Proc. Natl. Acad. Sci. U.S.A. 105, 9793–9798. doi: 10.1073/pnas.0802917105
Rider, O. J., Tayal, U., Francis, J. M., Ali, M. K., Robinson, M. R., Byrne, J. P., et al. (2010). The effect of obesity and weight loss on aortic pulse wave velocity as assessed by magnetic resonance imaging. Obesity 18, 2311–2316. doi: 10.1038/oby.2010.64
Ruderman, N. B., Carling, D., Prentki, M., and Cacicedo, J. M. (2013). AMPK, insulin resistance, and the metabolic syndrome. J. Clin. Invest. 123, 2764–2772. doi: 10.1172/JCI67227
Ruiz-Hurtado, G., Condezo-Hoyos, L., Pulido-Olmo, H., Aranguez, I., Del Carmen Gonzalez, M., Arribas, S., et al. (2014). Development of albuminuria and enhancement of oxidative stress during chronic renin-angiotensin system suppression. J. Hypertens. 32, 2082–2091; discussion 2091. doi: 10.1097/HJH.0000000000000292
Safar, M. E., Czernichow, S., and Blacher, J. (2006). Obesity, arterial stiffness, and cardiovascular risk. J. Am. Soc. Nephrol. 17, S109–S111.
Salt, I. P., and Hardie, D. G. (2017). AMP-activated protein kinase: an ubiquitous signaling pathway with key roles in the cardiovascular system. Circ. Res. 120, 1825–1841.
Sato, H., Hayashi, J., Harashima, K., Shimazu, H., and Kitamoto, K. (2005). A population-based study of arterial stiffness index in relation to cardiovascular risk factors. J. Atheroscler. Thromb. 12, 175–180.
Sato, M., Ogawa, T., Otsuka, K., Ando, Y., and Nitta, K. (2013). Stiffness parameter beta as a predictor of the 4-year all-cause mortality of chronic hemodialysis patients. Clin. Exp. Nephrol. 17, 268–274. doi: 10.1007/s10157-012-0674-7
Satoh-Asahara, N., Kotani, K., Yamakage, H., Yamada, T., Araki, R., Okajima, T., et al. (2015). Cardio-ankle vascular index predicts for the incidence of cardiovascular events in obese patients: a multicenter prospective cohort study (Japan Obesity and Metabolic Syndrome Study: JOMS). Atherosclerosis 242, 461–468. doi: 10.1016/j.atherosclerosis.2015.08.003
Savoia, C., Touyz, R. M., Amiri, F., and Schiffrin, E. L. (2008). Selective mineralocorticoid receptor blocker eplerenone reduces resistance artery stiffness in hypertensive patients. Hypertension 51, 432–439. doi: 10.1161/HYPERTENSIONAHA.107.103267
Sehgel, N. L., Zhu, Y., Sun, Z., Trzeciakowski, J. P., Hong, Z., Hunter, W. C., et al. (2013). Increased vascular smooth muscle cell stiffness: a novel mechanism for aortic stiffness in hypertension. Am. J. Physiol. Heart Circ. Physiol. 305, H1281–H1287. doi: 10.1152/ajpheart.00232.2013
Shargorodsky, M., Fleed, A., Boaz, M., Gavish, D., and Zimlichman, R. (2006). The effect of a rapid weight loss induced by laparoscopic adjustable gastric banding on arterial stiffness, metabolic and inflammatory parameters in patients with morbid obesity. Int. J. Obes. 30, 1632–1638.
Sheu, W. H., Chang, T. M., Lee, W. J., Ou, H. C., Wu, C. M., Tseng, L. N., et al. (2008). Effect of weight loss on proinflammatory state of mononuclear cells in obese women. Obesity 16, 1033–1038. doi: 10.1038/oby.2008.37
Sjostrom, L., Peltonen, M., Jacobson, P., Sjostrom, C. D., Karason, K., Wedel, H., et al. (2012). Bariatric surgery and long-term cardiovascular events. JAMA 307, 56–65. doi: 10.1001/jama.2011.1914
Somoza, B., Guzman, R., Cano, V., Merino, B., Ramos, P., Diez-Fernandez, C., et al. (2007). Induction of cardiac uncoupling protein-2 expression and adenosine 5’-monophosphate-activated protein kinase phosphorylation during early states of diet-induced obesity in mice. Endocrinology 148, 924–931.
Spinale, F. G. (2007). Myocardial matrix remodeling and the matrix metalloproteinases: influence on cardiac form and function. Physiol. Rev. 87, 1285–1342.
Stone, J. D., Narine, A., Shaver, P. R., Fox, J. C., Vuncannon, J. R., and Tulis, D. A. (2013). AMP-activated protein kinase inhibits vascular smooth muscle cell proliferation and migration and vascular remodeling following injury. Am. J. Physiol. Heart Circ. Physiol. 304, H369–H381. doi: 10.1152/ajpheart.00446.2012
Strasser, B., Arvandi, M., Pasha, E. P., Haley, A. P., Stanforth, P., and Tanaka, H. (2015). Abdominal obesity is associated with arterial stiffness in middle-aged adults. Nutr. Metab. Cardiovasc. Dis. 25, 495–502. doi: 10.1016/j.numecd.2015.01.002
Sutton-Tyrrell, K., Newman, A., Simonsick, E. M., Havlik, R., Pahor, M., Lakatta, E., et al. (2001). Aortic stiffness is associated with visceral adiposity in older adults enrolled in the study of health, aging, and body composition. Hypertension 38, 429–433.
Turin, T. C., Kita, Y., Rumana, N., Takashima, N., Kadota, A., Matsui, K., et al. (2010). Brachial-ankle pulse wave velocity predicts all-cause mortality in the general population: findings from the Takashima study. Jpn. Hypertens. Res. 33, 922–925. doi: 10.1038/hr.2010.103
Venugopal, S. K., Devaraj, S., Yuhanna, I., Shaul, P., and Jialal, I. (2002). Demonstration that C-reactive protein decreases eNOS expression and bioactivity in human aortic endothelial cells. Circulation 106, 1439–1441.
Vlachopoulos, C., Aznaouridis, K., and Stefanadis, C. (2010). Prediction of cardiovascular events and all-cause mortality with arterial stiffness: a systematic review and meta-analysis. J. Am. Coll. Cardiol. 55, 1318–1327.
Weisbrod, R. M., Shiang, T., Al Sayah, L., Fry, J. L., Bajpai, S., Reinhart-King, C. A., et al. (2013). Arterial stiffening precedes systolic hypertension in diet-induced obesity. Hypertension 62, 1105–1110. doi: 10.1161/HYPERTENSIONAHA.113.01744
Wildman, R. P., Mackey, R. H., Bostom, A., Thompson, T., and Sutton-Tyrrell, K. (2003). Measures of obesity are associated with vascular stiffness in young and older adults. Hypertension 42, 468–473.
Willum-Hansen, T., Staessen, J. A., Torp-Pedersen, C., Rasmussen, S., Thijs, L., Ibsen, H., et al. (2006). Prognostic value of aortic pulse wave velocity as index of arterial stiffness in the general population. Circulation 113, 664–670.
Winnik, S., Auwerx, J., Sinclair, D. A., and Matter, C. M. (2015). Protective effects of sirtuins in cardiovascular diseases: from bench to bedside. Eur. Heart J. 36, 3404–3412. doi: 10.1093/eurheartj/ehv290
Womack, J., Tien, P. C., Feldman, J., Shin, J. H., Fennie, K., Anastos, K., et al. (2007). Obesity and immune cell counts in women. Metabolism 56, 998–1004.
Woo, K. S., Chook, P., Yu, C. W., Sung, R. Y., Qiao, M., Leung, S. S., et al. (2004). Effects of diet and exercise on obesity-related vascular dysfunction in children. Circulation 109, 1981–1986.
Xu, X. J., Gauthier, M. S., Hess, D. T., Apovian, C. M., Cacicedo, J. M., Gokce, N., et al. (2012). Insulin sensitive and resistant obesity in humans: AMPK activity, oxidative stress, and depot-specific changes in gene expression in adipose tissue. J. Lipid Res. 53, 792–801. doi: 10.1194/jlr.P022905
Xu, X. J., Pories, W. J., Dohm, L. G., and Ruderman, N. B. (2013). What distinguishes adipose tissue of severely obese humans who are insulin sensitive and resistant? Curr. Opin. Lipidol. 24, 49–56. doi: 10.1097/MOL.0b013e32835b465b
Keywords: obesity, bariatric surgery, matrix metalloproteinase-9, AMP-activated protein kinase, lactate dehydrogenase, intrinsic arterial stiffness
Citation: García-Prieto CF, Gil-Ortega M, Vega-Martín E, Ramiro-Cortijo D, Martín-Ramos M, Bordiú E, Sanchez-Pernaute A, Torres A, Aránguez I, Fernández-Alfonso M, Rubio MA and Somoza B (2019) Beneficial Effect of Bariatric Surgery on Abnormal MMP-9 and AMPK Activities: Potential Markers of Obesity-Related CV Risk. Front. Physiol. 10:553. doi: 10.3389/fphys.2019.00553
Received: 27 July 2018; Accepted: 18 April 2019;
Published: 08 May 2019.
Edited by:
Maik Gollasch, Charité Medical University of Berlin, GermanyReviewed by:
Juan Carlos Ruiz De Adana, Hospital Universitario de Getafe, SpainJohannes Baulmann, European Prevention Centre, Germany
Copyright © 2019 García-Prieto, Gil-Ortega, Vega-Martín, Ramiro-Cortijo, Martín-Ramos, Bordiú, Sanchez-Pernaute, Torres, Aránguez, Fernández-Alfonso, Rubio and Somoza. This is an open-access article distributed under the terms of the Creative Commons Attribution License (CC BY). The use, distribution or reproduction in other forums is permitted, provided the original author(s) and the copyright owner(s) are credited and that the original publication in this journal is cited, in accordance with accepted academic practice. No use, distribution or reproduction is permitted which does not comply with these terms.
*Correspondence: Marta Gil-Ortega, mgortega@ceu.es Beatriz Somoza, bsomoza.fcex@ceu.es