- 1RF SSC – Institute of Biomedical Problems, Russian Academy of Sciences, Moscow, Russia
- 2Center for Neuroregeneration, Houston Methodist Research Institute, Houston, TX, United States
Dry immersion (DI) is one of the most widely used ground models of microgravity. DI accurately and rapidly reproduces most of physiological effects of short-term space flights. The model simulates such factors of space flight as lack of support, mechanical and axial unloading as well as physical inactivity. The current manuscript gathers the results of physiological studies performed from the time of the model’s development. This review describes the changes induced by DI of different duration (from few hours to 56 days) in the neuromuscular, sensory-motor, cardiorespiratory, digestive and excretory, and immune systems, as well as in the metabolism and hemodynamics. DI reproduces practically the full spectrum of changes in the body systems during the exposure to microgravity. The numerous publications from Russian researchers, which until present were mostly inaccessible for scientists from other countries are summarized in this work. These data demonstrated and validated DI as a ground-based model for simulation of physiological effects of weightlessness. The magnitude and rate of physiological changes during DI makes this method advantageous as compared with other ground-based microgravity models. The actual and potential uses of the model are discussed in the context of fundamental studies and applications for Earth medicine.
Introduction
Observations performed after space flights of different durations have shown that weightlessness causes body deconditioning, which is demonstrated by changes in most of the physiological systems (Gazenko et al., 1986; Kornilova, 1987; Kozlovskaya et al., 1988, 1990; Berger et al., 1992; Grigoriev and Egorov, 1992; Edgerton and Roy, 1996; Reschke et al., 1998; Clement et al., 2003). The necessity to study the mechanisms of hypogravitational effects on the human body led to the exploration of experimental models of weightlessness.
Immersion into a liquid analogous by density to human body tissues (Gazenko et al., 1972; Shulzhenko and Vill-Villiams, 1975) and antiorthostatic hypokinesia (head down tilt bedrest, HDBR) (Kakurin, 1968; Genin and Sorokin, 1969) have been proposed to be the most appropriate environment for the physiological studies of hypogravity effects.
Support withdrawal, local load elimination and the proximity of biomechanical conditions of motor activity organization to those in weightlessness from the beginning of space era determined that immersion would be chosen as the best model for training of working operations in weightlessness.
In the early 1960s, scientists started to study the physiological effects of immersion to determine its capacity to simulate the effects of weightlessness (Beckman et al., 1961; Graveline et al., 1961). It has been shown that immersion reproduces microgravity-induced changes in the human body’s motor (Ovsyannikov, 1972) and cardio-vascular (Shulzhenko and Vill-Villiams, 1976) systems and affects other physiological functions. The usage of immersion as a valuable model was limited only by discomfort and possible risks of long term skin contact with the liquid environment.
History of DI Model Development
At the beginning of the 1970s two investigators from the Institute of Biomedical Problems (Russia), K. B. Shulzhenko and I. F. Vil-Villiams, developed a method of long term immersion, dry immersion (DI), with the use of special waterproof and highly elastic fabric (Shulzhenko, 1975; Shulzhenko and Vill-Villiams, 1975). A test subject wearing a shirt and trunks was put on waterproof fabric and immersed into a deep bath up to the neck level, in a supine position. The area of the fabric’s surface considerably exceeded the area of the water surface. The folds of the waterproof fabric allowed the person’s body to be enveloped from all sides freely (Figure 1).
The high elasticity properties of the fabric artificially created conditions similar to zero gravity via floatation. Thus, DI reproduced three effects of weightlessness: physical inactivity, support withdrawal and elimination of the vertical vascular gradient. Since its development, DI has been the main model in Russia for studying the effects of weightlessness lasting 5–7 days, similar to the duration of short-term flights on orbital space stations.
Most of the results described in this review come from studies where healthy male volunteers were placed, individually or in pairs, in a supine position in a bath with dimensions of 200 × 100 × 100 (300 × 300 × 200) cm. The bath was filled with water at a temperature that was kept constant at 33 ± 0.5°C. The daily routine was specified in accordance with the schedule of studies, countermeasure procedures (if the experiment included them), including 8 h of sleep, 3–4 meals, a medical supervision program and experimental studies. The research participants were taken out of DI for 15–20 min each day for sanitary and hygienic procedures with the usage of a special lift rising from the bottom of the bath.
In 1974, E. B. Shulzhenko and I. F. Vil-Villiams performed the longest 56-day DI experiment with two volunteers, which convincingly proved the applicability and safety of the DI model for reproducing long-term microgravity effects. The results of this experiment showed that 56 days of DI decreased the redundant capabilities of the blood circulation system, providing deconditioning of the body as a whole. The changes of the functional state of the blood circulation system demonstrated signs of an adaptation processes with the predominance of parasympathetic tone and decline of resistance to g-loading in the “head-hip” direction and low body negative pressure (Shulzhenko and Vill-Villiams, 1976; Vil-Villiams and Shulzhenko, 1978).
In early years of the DI’s use, the main direction of research was a description of its effects on different functions of the human body. Attention of the researchers was primarily focused on vital functions, including the activities of the cardiovascular and respiratory systems and metabolism. These studies revealed similarities in the depth, dynamics and direction of DI-induced physiological changes to those observed in space flights (Kamenskiy et al., 1976; Aleksandrova et al., 1980; Fomin, 1981; Ivanov et al., 1983; Kozinets et al., 1983; Orlov, 1985; Yarullin et al., 1987; Genin and Galichiy, 1995; Mikhailov et al., 1995).
It has been shown that the subjective experience of exposure to DI at its initial stage is perceived as a comfortable and pleasant state of relaxation (Shulzhenko and Vill-Villiams, 1976; Shulzhenko et al., 1983a; At’kov and Bednenko, 1987; Navasiolava et al., 2011a; Tomilovskaya, 2013a,b). However, heaviness in the head and nasal congestion in some cases were observed later (presumably due to a redistribution of the body’s fluids in the cranial direction). During the first 2–3 days of DI, most of test subjects reported the development of abdominal pain (Rukavishnikov et al., 2014b) and back pain of differing intensity (Rukavishnikov et al., 2017a,b); on the 3rd or 4th day of DI, all of these signs disappeared. Sleep disorders (mostly due to back and abdominal pain), loss of appetite, meteorism and constipation are also observed in the initial stages of DI (Shulzhenko, 1975; Fomin, 1981; Shulzhenko et al., 1984; Tomilovskaya, 2013a,b). Under conditions of “double baths,” when 2 subjects shared the same water pool, body movements of one subject were sometimes followed by the symptoms of space motion sickness for the other one (Barmin et al., 1983; Struhal et al., 2002; Kreidich et al., 2007), similar to those registered during the 1st days of space flight (Kornilova, 1997; Williams et al., 2009) and bedrest (Greenleaf, 1984; Baum and Essfeld, 1999).
In the 1970s the water immersion was used broadly as a fast and convenient model for assessing the efficacy of countermeasure means and methods. Those studies were performed at the Russian Scientific Testing Institute of Aviation and Space Medicine by A. M. Genin, I. D. Pestov, V. I. Stepantsov, A. V. Eremin and other specialists of space mission medical support. Later, this research was entrusted to the newly created Institute of Biomedical Problems. However, the impossibility of long term stay in the water immersion imposed serious restrictions to the range of tested countermeasure means. The DI method opened the possibility for long-duration exposures and started a new line of studies.
Similarity of DI- and HDBR-Induced Effects
In parallel to the DI experiments, HDBR research has been developing and the model has achieved worldwide popularity (Watenpaugh, 2016). This is not surprising because the organization of HDBR is relatively easier than DI, since the test subjects are available for the studies for almost 24 h a day at all the stages of experiment. Comparison of the data has shown similarity of the changes observed in these two models (Aleksandrova et al., 1980; Chaika et al., 1982; Grigoriev et al., 2004). However, the dynamics and the depth of these changes significantly differ (Kozlovskaya et al., 1984; Grigoriev et al., 2004). For instance, according to Shenkman et al. (1997), the decrease in size of the slow and fast types of muscle fibers reached 15–18% by the 7th day of DI; similar changes were observed only after 60 days of HDBR. Very recent studies of cardiovascular, postural and neuromuscular changes under conditions of 21 days of HDBR and 3 days of DI revealed the similarity of their effects, suggesting that DI has an influence that is seven times stronger than HDBR (Tomilovskaya et al., 2018).
HDBR and DI reproduce the lack of axial loads, redistribution of body fluids in a cranial direction (to a greater extent in HDBR), hypokinesia and immobilization. The only substantial difference between these models is the level of support deprivation: in DI, support loads are totally eliminated, while HDBR provides the redistribution of support loads from the soles of the feet to the larger areas of the back and sides of the torso. The data from an experiment with combined exposure to HDBR and DI (alternating 12 h of HDBR and 12 h of DI) revealed significantly greater changes in periods of DI and their smoothing during HDBR sessions, confirming the leading role of support afferentation in the tonic postural system and motor acts regulation (Kozlovskaya et al., 1984).
Therefore, at the end of the 1970s, the DI model became the core component of studies focused on the effects of microgravity on the sensory-motor system. The motor system is considered to be the most gravity-dependent system of the human body. One of the main gravity-depended functions of the motor system is the maintenance of vertical posture and the position of body segments in the gravitational field. These functions are provided in mammals including humans by the tonic (postural) muscle system. The absence or sharp decline of gravity, eliminating the necessity of the aforementioned activities in weightlessness, greatly alters the functional and structural properties of tonic muscles. Orbital space flights have confirmed this suggestion and revealed the main factors which contribute to these effects of microgravity (Kozlovskaya, 2007). These factors are: a decrease of mechanical loads, a decline of axial and support loads, changes in the biomechanics of movements and changes in sensory system activities. The DI model reproduces accurately and to a full extent axial and support unloading, and significantly changes the sensory system’s activity. Exposure to DI eliminates the factor of support but at the same time does not directly affect the other sensory systems, which provides a definite advantage in comparison to other microgravity models and allows to study the role of support afferentation in the body systems’ activities.
In recent years the model of DI has gained popularity: a number of investigators from different countries (DLR in Germany, Angers University and Caen University in France) have taken part in the Russian DI research at IBMP. The results of these studies confirmed the supposition of the effects of support withdrawal on the activities of almost all body systems.
DI Effects on the Neuromuscular System
Exposure to DI results in dramatic decline in muscle force-velocity properties, as well in muscle tone, and composition. Muscle effects, in particular a decrease in force-velocity properties, have been revealed even in very short term space flights (Kakurin et al., 1971; Bryanov et al., 1976; Kozlovskaya et al., 1984, 1988; Bachl et al., 1992); however, only under DI conditions their metrics and temporal dynamics could be studied in detail. Comparisons between the depth of changes after 7 days of DI and space flights of the same duration have revealed their close similarity (Grigorieva and Kozlovskaya, 1983; Kozlovskaya et al., 1984). DI experiments allowed not only to describe these phenomena but to get a better understanding about the nature of the decline in force-velocity properties of skeletal muscles (up to 30%), which cannot be explained by the muscle atrophy requiring much greater exposure times.
Subsequent studies of evoked and voluntary maximal contraction (MVC) strength of shin muscles confirmed this suggestion (Koryak, 1998, 1999, 2001). The results of the research have shown that MVC amplitude decreases similarly after 7 days of space flight, 7 days of DI and 4 months of HDBR. However, the amplitude of evoked responses declined insignificantly, indicating that the decrease of muscle force-velocity properties was caused by lower intensity of central efferent command (Koryak, 1998; Koryak, 2001).
One of the acute responses to transition to weightlessness is a flexor posture, which points to a decrease of the extensor muscle activity involved in the maintenance of vertical posture on Earth (Thornton, 1987; Reschke et al., 1998; Kozlovskaya, 2007). In fact, neurological observations performed immediately after short-term space flights in crew members of the first space expeditions revealed distinct extensor atonia (Bryanov et al., 1976). The results of studies of shin muscle transverse stiffness performed after 7-day space flights revealed a 15–20% decrease of muscle tone in m.gastrocnemius and m.soleus on the 2nd day after landing (Kozlovskaya et al., 1988).
This phenomenon was studied in detail in DI experiments on the ground, demonstrating the rapid decrease (up to 40–50%) of transverse stiffness in all three heads of the shin extensor — the lateral and medial m.gastrocnemius and most prominent – in m.soleus during the first 6 h of exposure (Gevlich et al., 1983; Kozlovskaya et al., 1988; Miller et al., 2004, 2005, 2010). The decrease of transverse stiffness of single muscle fibers was also revealed in m.soleus after 7-day DI (Ogneva et al., 2011). Studies of transverse stiffness in the shin flexor (m.tibialis anterior) did not reveal significant changes under DI conditions (Gevlich et al., 1983; Kozlovskaya et al., 1988; Miller et al., 2010).
Based on the above-mentioned data, the reflex nature of the muscle tone decline and its connection to the changes in motor units’ activity was suggested by Kirenskaya et al. (1986). This was confirmed in the studies of the recruitment order of motor units (MUs) in a task involving small effort plantar flexion (10–12% from maximal voluntary contraction) under conditions of 7 days of DI and 120 days of HDBR (Kirenskaya et al., 1986; Khristova et al., 1988; Kozlovskaya et al., 1988). The results of these studies allowed to conclude that support unloading causes the changes of recruitment order of shin extensor MUs through suppression of small (tonic) MUs recruitment and facilitation of recruitment of large (phasic) ones. Later, this suggestion was also confirmed in studies of DI of different duration (Shigueva et al., 2015b).
It is worth noting that revealed phenomena indicate that Henneman’s law of the MUs’ recruitment order is reversed under microgravity conditions. Classic Henneman’s law (or size principle) states that under load, motor units are recruited from smallest to largest. In practice, this means that slow-twitch, low-force, fatigue-resistant muscle fibers are activated before fast-twitch, high-force, less fatigue-resistant muscle fibers (Henneman et al., 1965). During DI, however, a motoneuron size is not the only factor defining the recruitment order.
In parallel to the neurophysiological research, a wide program of muscle morphological studies has been performed under DI conditions. Decreases in the size of both slow and fast types of muscle fibers reached 5–9% after 3 days and 15–18% after 7 days of DI (Shenkman et al., 1999; Shenkman, 2016; Shenkman et al., 2017). Meanwhile, the analogous changes after 60 days of HDBR reached 14.2 and 12.4% for slow and fast types of fibers, respectively (Shenkman et al., 2004a). Thus, the depth of size reduction of muscle fibers under conditions of short-term DI was commensurate to that under conditions of long term HDBR. This fact indicates the important role of support withdrawal in the genesis of hypogravitational muscle atrophy. It is important to note that the possibility of support-dependent control of atrophic processes has been discussed in a number of scientific papers (De-Doncker et al., 2000; Nemirovskaya and Shenkman, 2002; Ilyina-Kakueva and Kaplansky, 2005). However, this hypothesis was not validated in direct human experiments with stimulation of the support input. Oganov et al. (1980) first demonstrated the shift in the ratio of slow and fast myosin forms toward the fast ones under conditions of gravitational unloading, although these changes were not so evident in HDBR (Ilyina-Kakueva et al., 1979; Kuznetsov and Stepantsov, 1989, 1990; Berg, 1996).
More recent studies performed under strict conditions of maintenance of subjects’ supine position reported significant atrophy (up to 10%) of type I muscle fibers and increased portion of hybrid, typeI/II muscle fibers after 3 days of DI (Demangel et al., 2017). Molecular studies have also revealed changes in muscle signaling responses at the early stages of gravitational unloading (Vilchinskaya et al., 2015, 2016).
In summary, the neuromuscular effects of DI include:
1. Decrease in muscle force-velocity properties,
2. Decrease of tone in extensor muscles,
3. Suppression of small MUs recruitment and facilitation of recruitment of large ones,
4. Decreases in the size of both slow and fast types of muscle fibers.
Does Support Withdrawal Triggers Hypogravitational Motor Syndrome Development?
The DI studies created a new branch in motor physiology research which can be termed as gravitational motor physiology. Studies of the functions and segments of the motor system both at the cellular and systemic levels have shown that gravity is deeply influential in the motor system. Primarily it affects muscles, or the effector system. The data from cell together with neurophysiological studies allowed scientists to suggest that gravity is a factor which affects the tonic postural system development. The deep skin sensitivity represented by clusters of Fatter-Paccini bodies is the trigger of this system which provides information on the presence or absence of gravity (Otelin et al., 1976).
Since there are no any other direct afferent changes in DI, support withdrawal is what promotes the sharp development of both cellular and systematic changes. This statement was illustrated by the scheme suggested by I. B. Kozlovskaya and B. S. Shenkman (Figure 2).
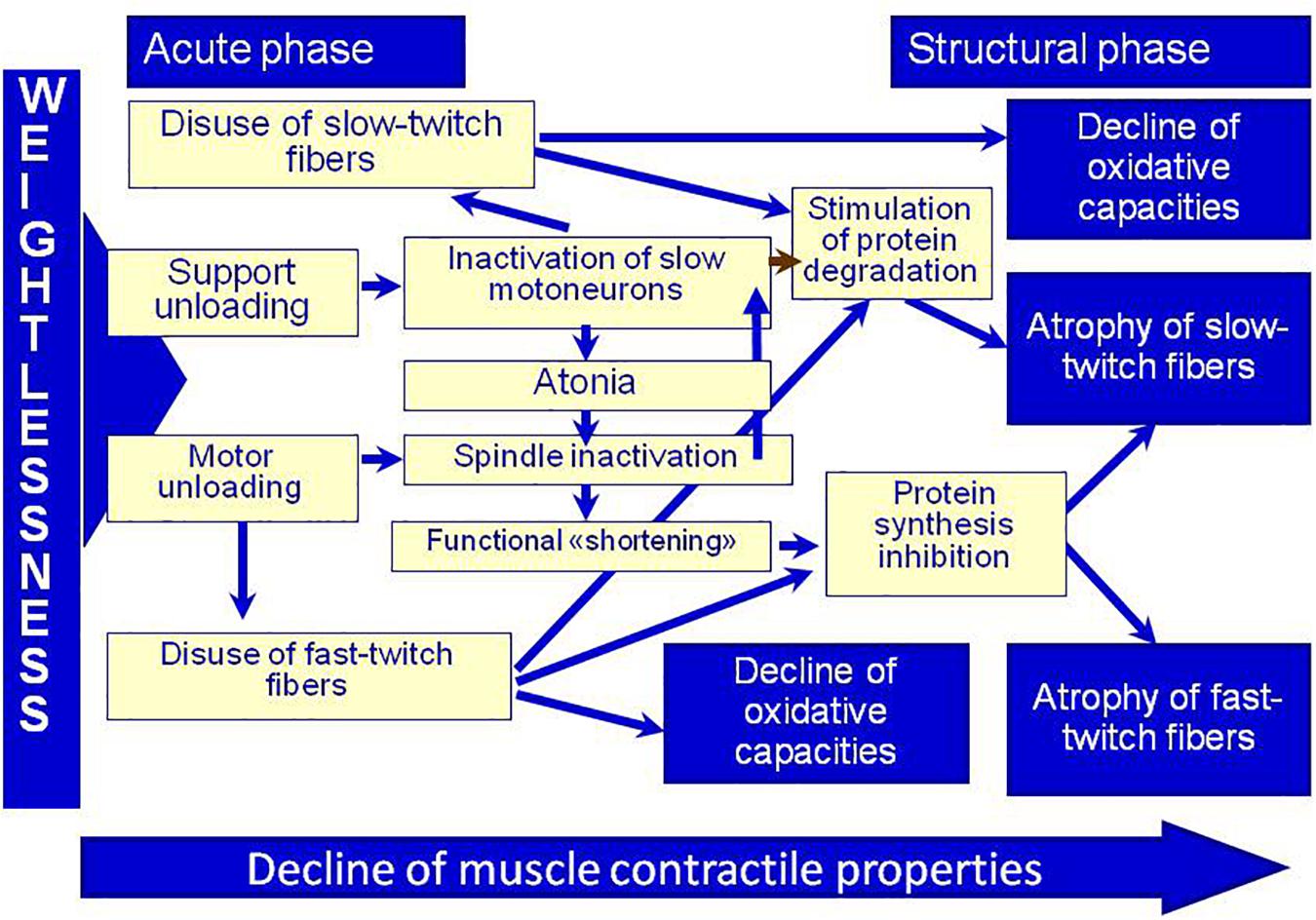
Figure 2. Physiological control of muscle plasticity in weightlessness (by Kozlovskaya I. B. and Shenkman B. S.). Adapted from Kozlovskaya, 2007.
To confirm the trigger role of support afferentation in the control of the structural-functional properties of the tonic muscle system, the influence of mechanical stimulation of the soles support zones in locomotor regimens (75 and 120 steps per minute, pressure of 40 kPa in each sole) was tested. Stimulation was provided by Compensator of Support Unloading (CSU) developed jointly by IBMP, “Zvezda” and “VIT.” CSU provides alternating pressure of 0.5 kg/cm2 (40 kPa) on the support zones of the soles (heel and metatarsal areas).
The results of these studies confirmed the hypothesis that support afferentation plays the trigger role in the system of postural tonic control. The aforementioned changes in leg muscle functions were not observed in participants in whom the mechanical stimulation of the soles support zones during DI exposure was applied (Kozlovskaya et al., 2007a,b).
A decrease of transverse stiffness in m.soleus in this group reached significance only by the 6th day of DI; at the same time decrease of EMG amplitude at rest and maximal isokinetic force of m.triceps surae as well as signs of hyperreflexia and changes in MU recruitment order were not registered at all (Grigoriev et al., 2004; Khusnutdinova et al., 2004; Miller et al., 2004, 2005, 2010; Netreba et al., 2004, 2005, 2006; Kozlovskaya et al., 2007a,b; Shigueva et al., 2015a; Zakirova et al., 2015). Analogous effects of mechanical stimulation of the soles support zones were demonstrated in the structure of the muscular apparatus: no decrease of myofibrils’ sensitivity to free calcium ions, which is regularly observed under conditions of gravitational unloading; no changes in the cross sectional area of muscle fibers; and no decrease of the number of fibers containing slow isoforms of heavy myosin chains (Grigoriev et al., 2004; Moukhina et al., 2004; Shenkman et al., 2004a,b).
The natural consequence of the results described above was an offer to include the method of artificial support stimulation into the space flight countermeasure system for counteracting the early, acute motor system changes in weightlessness. Therefore, the device for mechanical stimulation of the soles support zones was modified in accordance with ISS requirements (the “CSU” device manufactured by IBMP and “Zvezda”), and at the end of 2017, it was delivered to the space station.
DI Effects on the Sensory-Motor System
The DI studies have discovered that sensory-motor control systems are deeply affected when unloaded. First, the accuracy of movement control decreases (Repin, 1981; Kreidich et al., 1982; Grigorieva and Kozlovskaya, 1985; Kirenskaya et al., 1985; Sosnina et al., 2016). It has also been shown that the cortical organization of voluntary movements is changed (Kirenskaya et al., 2006; Tomilovskaya et al., 2008), and that the systems of posture and locomotion control suffer greatly (Melnik et al., 2006; Shpakov et al., 2008; Sayenko et al., 2016; Amirova et al., 2017). Similarly, studies of resting state brain activity revealed the changes similar to those obtained in a real space flight, indicating that support withdrawal is the main contributor to brain activity alterations and decline in the function of corticospinal tract function in weightlessness (Kuznetsova et al., 2015; Lazarev et al., 2018). Interestingly, support stimulation under DI conditions also eliminated these changes, although to a lesser extent than peripheral ones (Kozlovskaya et al., 2007a,b).
Vestibular function and visual-manual tracking is also significantly changed under DI conditions despite the absence of any direct influence on the vestibular system (Repin, 1981; Kreidich et al., 1982; Barmin et al., 1983; Kornilova et al., 2004, 2008, 2011a,b, 2013, 2016; Zobova et al., 2008).
For instance, in the studies of the static torsional otolith-cervical-ocular reflex (OCOR), dynamic vestibular-cervical-ocular reactions (VCOR), vestibular reactivity (VR), and spontaneous eye movements after 5- and 7-day DI a significant decrease in OCOR (gOCOR was 0.1, compared to the background gOCOR value of 0.25) was detected alongside a simultaneous significant increase in the VCOR/VR parameters in 28% of subjects on next day after DI. The findings have demonstrated that the support withdraw and the deficit of proprioception affected rather the accuracy of visual tracking than manual tracking. The obtained results confirm the development of sensory deprivation (and afferent deficit) under the DI exposure (Kornilova et al., 2016).
At the same time, the sensitivity to vestibular signals is sharply increased: the thresholds of oculographic response to galvanic stimulation decreased to 0.68–0.73 mA on the day 2 after 7-day DI in comparison to 0,92–1,05 mA before DI (Repin, 1981). Similar findings were observed in DI experiments performed in primates (Eron et al., 2000; Badakva et al., 2007). It is worth noting that no signs of motion sickness were reported during DI (Tomilovskaya, 2013b).
The sensitivity to proprioceptive signals was also changed: exposure to DI was associated with the development of H-reflex system hypersensitivity, which was demonstrated by a decrease of the soleus H-reflex thresholds and an increase of relative H-reflex amplitudes (Kozlovskaya et al., 2007a; Saenko, 2007; Zakirova et al., 2015).
As mentioned above, motion sickness was not observed during DI (Tomilovskaya, 2013b). Authors suggested that this may be due to rather motionless state of the participants. To test this, researchers began to investigate another type of water immersion – “suit immersion” (SI) — developed by IBMP specialists at the end of the 1980s (Genin et al., 1988a,b). SI, which used a thin-walled wetsuit “Forel” (“Trout” in Russian) equipped with an inflatable neck pillow to create postural, vestibular and operational loads directly during immersion, differed from DI by exclusion of strict hypokinesia and maintenance of vertical posture (Genin et al., 1988a,b; Larina et al., 1999; Larina and Lakota, 2000).
Although there is a difference in the body position between DI and SI, that is supine vs. vertical position, respectively, hemodynamics and water-salt exchange parameters have been shown to be similar (Genin et al., 1988a,b; Larina et al., 1999). However, during the first hours of SI, the development of vestibulo-autonomic syndrome, characterized by perception of body and head movements/rotations, was revealed (Genin et al., 1988b). In addition, the wide spectrum of illusions up to short-term loss of the awareness of body orientation were observed during SI. Following SI, a decrease of passive orthostatic probe tolerance, impaired postural control, an increase of EMG cost of standard motor tasks, and a significant decrease of physical and operator capacity were observed (Kreidich et al., 2007).
In summary, the effects of DI on the sensory-motor system include:
1. Decrease in accuracy of movement control,
2. Decline in descending regulation of voluntary movements,
3. Increased sensitivity to vestibular signals,
4. Decline in the accuracy of visual tracking,
5. Increased excitability of the soleus H-reflex.
DI Effects on the Cardiorespiratory System
Studies of respiratory function have shown an increase of respiratory resistance, greater inspiratory and reduced expiratory reserve volumes, as well as changes in the thoracic-abdominal ratio in breathing motions and shifts in voluntary respiration regulation (Dyachenko et al., 2010; Mikhailovskaya et al., 2011; Popova et al., 2011). In addition, increased amplitude of breathing motions with reduced frequency has been noted, together with distinct breathing-related heart rate fluctuations (Semenov et al., 2011).
Furthermore, after 5 days of DI a significant increase in the markers of oxidative stress such as expired amines, chiefly butylamine, 2-cyanacetimide, some aldehydes, polyols, phenol, phenylacetylene, ketones, butyl acetate and a significant decrease in fatty acids has been observed (Mardanov et al., 2011). The mentioned changes indicate the alterations in metabolism and weakening of antioxidant system.
Studies of cardiovascular system changes in DI have revealed the subsequent involvement of electrical (increase of amplitude of QRS complex) and then – energy metabolic (decrease of heart rate and water-electrolyte balance alterations) processes changes in the myocardium; the most prominent changes were registered on the 5th day of DI (Ivanov et al., 2011). Autonomic regulation also appears to shift in the direction of the sympathetic component (Iwase et al., 2000; Eshmanova et al., 2008, 2009; Kabulova and Eshmanova, 2008).
Exposure to DI even of short duration (7 h) decreases orthostatic tolerance, and as the period of immersion is prolonged, the proportion of participants with orthostatic hypotension increases (Iwase et al., 2000; Vinogradova et al., 2002b; Eshmanova et al., 2009; Navasiolava et al., 2011a). All the studies revealed an increase in heart rate during vertical stance after DI of different duration (Navasiolava et al., 2011a,b). Recent studies performed after 3-days DI have shown decrease of systolic blood pressure, stroke volume and baroreflex sensitivity, pronounced tachycardia during low body negative pressure (LBNP) tilt test (De Abreu et al., 2017). The time of orthostatic tolerance in this study dropped from 27 to 9 min after DI; 9 of 12 subjects were unable to complete the test. Maximal LBNP value in the control studies reached -60 mmHg; 9 of 12 subjects have completed the test at the level of -30 mmHg. After DI exposure the LBNP level of -30 mmHg was reached by only 1 subject of 12 (De Abreu et al., 2017).
The relationship between changes in the water-electrolyte balance and cardiovascular responses revealed changes in electrophysiological propagation of myocardial excitation, and an increased variance of natural small oscillations of the electric potential of the heart (Larina et al., 2011). At the same time there was registered the increase of the brain natriuretic peptide BNP (as well as inactive NTproBNP secreted in an equimolar ratio), which is released by cardiomyocytes in response to stretching upon an increase in the ventricular pressure. The authors suggest that the increased level of NTproBNP during the recovery period following DI, reflects the degree of heart deconditioning during DI. The revealed intense tachycardia during orthostasis after DI, as well as the revealed changes in the electrophysiological characteristics of the myocardium and hypovolemia (one of the factors of development of the cardiovascular deconditioning under microgravity) during DI support the hypothesis of deconditioning induced increase in the heart load in the period of recovery. Within the first 24 h, the weight loss, rapid loss of fluid and sodium, and increase in the free cortisol content in urine were also observed. The changes in the water–electrolyte balance could cause metabolic–energy shifts that required activation of the respective regulatory mechanisms. A considerable (more than twofold) growth of the centralization index at the end of the experiment shows that inclusion of the central regulation mechanisms in the adaptation processes was an appropriate response aimed at compensation of the primary changes in the water–electrolyte balance and hemodynamics. The described changes appeared within the first 24 h of DI, and were manifested in the following days of the experiment. The changes in autonomic regulation and the electrophysiology of the myocardium during the experiment gradually increased, and reached maximum at the end of the 7th day of DI. Thus, it has been shown that the structures regulating the metabolic processes and, potentially, higher autonomic centers, from molecular–cellular to systemic levels, may be involved in the cardiorespiratory response occurring during the first 24 h of DI. The revealed alterations in the myocardial excitation warrants further research (Larina et al., 2011).
In summary, the effects of DI on the cardiorespiratory system include:
1. Increased respiratory resistance,
2. Greater inspiratory reserve volume
3. Reduced expiratory reserve volume,
4. Increase of the abdominal component during breathing,
5. Weakening of antioxidant system,
6. Increase of amplitude of QRS complex,
7. Decrease of heart rate,
8. Orthostatic hypotension,
9. Changes in the water-electrolyte balance and cardiovascular responses.
Hemodynamic Changes in DI
Exposure to DI has also been associated with endothelial dysfunction, including an increase in circulating endothelial microparticles (Larina et al., 2008; Navasiolava et al., 2010, 2011b). Ultrasound analysis of peripheral hemodynamics have shown that the linear velocity of blood flow along main arteries and low extremity veins decelerates in the course of DI (Moreva, 2008; Moreva et al., 2018). At the same time, increased intensity of tissue perfusion and a higher number of capillaries in the upper extremities during 5-day DI were registered (Suvorov et al., 2017). Other authors, however, reported an increase of middle cerebral vein velocity and jugular vein, portal vein and thyroid volume, but these findings were obtained only during the first 2 h of DI (Arbeille et al., 2017). Another recent study failed to reveal changes in blood flow in either cerebral artery, but internal carotid and vertebral arteries conductance decreased significantly on the 2nd and 3rd day of DI (Ogoh et al., 2017).
It was also shown that DI significantly affects blood supply in working muscles. The peak bloodstream in the calf muscles decreases 7–20% after DI exposure, while post-contraction hyperemia noticeably increases (Vinogradova et al., 2002b). In studies of the blood flow of the tibia performed under conditions of physical loading, it was suggested that exposure to DI strengthened the concurrent relationships between local working hyperemia and central vasoconstrictive effects directed toward the maintenance of circulatory homeostasis (Stoida et al., 1998). Physical loading after 7-day DI was also followed by changes in energy and metabolism as indicated by decrease of creatine phosphokinase activity, and the changes of levels of cortisol, triglycerides, insulin and inorganic phosphate in blood plasma (Buravkova et al., 2003). However, no changes in metabolic reflex regulation of hemodynamic parameters under conditions of local static muscle work were registered (Bravy et al., 2008).
In summary, the effects of DI on hemodynamics include:
1. Endothelial dysfunction,
2. Decrease in the linear velocity of blood flow along main arteries and low extremity veins,
3. Decrease in blood supply of lower extremity muscles.
DI Effects on Intracranial Pressure (ICP)
Since DI was proposed to reproduce distribution of body fluids similar to that during space flight, some research has been dedicated to the assessment of intracranial pressure. However, the data of ultrasound studies were characterized by high variability, and did not lead to definite conclusions (Arbeille et al., 2017). Use of the otoacoustic emission method through registration of electrical response of outer hair cells of the cochlea — DPMC (Phase Shift of Microphonic Cochlear Potential) — showed that body position and DI do affect the DPMC (and thus ICP). Changes in ICP were similar during a supine-to-DI position change and supine-to-antiorthostatic position change. Following DI, a tendency to decreased phase shift of the otoacoustic response during transition of the body position from a vertical to antiorthostatic one, was revealed, which could be associated with the ICP increase (Avan et al., 2013; Rukavishnikov et al., 2013).
A study of optic nerve sheath diameter (ONSD) and cerebral autoregulation revealed a strong negative correlation between these two parameters. The authors suggested that a persistent elevation of ICP can be attributed to poor cerebral autoregulation recovery during DI (Kermorgant et al., 2017).
DI Effects on Renal and Digestive Systems
Studies of the excretory function of the kidneys together with the classical increase of dieresis revealed an increase of protein and glucose excretion, although not exceeding physiological standards (Vorontsov et al., 2011, 2014). No shifts of glomerular filtration or tubular reabsorption were registered (Nesterovskaya et al., 2008). At the same time, the impedance measurement revealed a decrease of general body fluid and the volume of extracellular fluid as well as the volume of circulating plasma (Larina et al., 2008; Noskov et al., 2011).
Other studies report that exposure to DI causes rapid fluid and sodium loss, which increases the Na/K ratio in urine. The accompanying body weight loss is due to a decrease in the level of body hydration. An increase in the level of free cortisol in urine only on the 1st day of DI may be due to adaptation to the new conditions. The most pronounced changes were observed on the 1st day of DI. On the following days, a new level of water–electrolyte balance is established. The absence of changes in the renin or aldosterone level on days 3 and 7 was evidence that the major redistribution of fluids had been completed by that time and regulation of the water–electrolyte balance had stabilized. The decrease in urine osmolality during DI characterizes an enhanced excretion of water but not electrolytes and shows that the regulatory mechanisms perceive water consumption during DI as excessive. In addition, this suggests a decrease in the concentration capacity of the kidneys (Larina et al., 2011).
Studies of the digestive system have revealed deceleration in the hepatic venous flow and signs of plethora in the abdominal venous system (Solovieva et al., 2016). Elevated levels of several substances were detected in the blood: pepsinogen, pancreatic amylase, bilirubin total (due to its unconjugated fraction), insulin, and C-peptide. The 13C-methacetin breath test has shown a slowdown in the rate of 13C-methacetin inactivation and a reduction in the hepatic metabolic capacity (Solovieva et al., 2016). The study of the liquid food evacuation during immersion has shown that its rate did not change substantially, therefore, reductions in the rates of methacetin inactivation and metabolism during immersion could be associated, mainly, with a slower assimilation of the preparation and a decrease in the venous blood flow of the liver. A reduction in the metabolic capacity of the liver in the conditions of immersion reflects a decrease in the inactivation of toxic metabolites, including unconjugated bilirubin, which may explain the increase of its content in blood.
Further studies have demonstrated the stability of liquid evacuation from the stomach and acceleration of the chymus transit along the small intestine hinder evacuation of the large intestine content, which is the primary cause for inhibition of gastrointestinal evacuatory activity in DI (Afonin and Goncharova, 2009; Afonin et al., 2013). The elevated gastrointestinal electrical activity may be related to the increased gastric secretion and elevated intestine tone in fasting test subjects, and displayed a close similarity to the changes induced by caffeine stimulation, long-term bed rest or space flight (Afonin and Sedova, 2012). At the same time, clear signs of dysbiotic changes such as significant reduce of fecal lactoflora and developed shifts in the microbial landscape (increase of the number of staphylococcus, yeast microflora and Gram-negative Bacillus) have been revealed (Ilyin et al., 2008; Solovieva et al., 2011).
In summary, the effects of DI on the renal and digestive systems include:
1. Decrease of general body fluid and the volume of extracellular fluid as well as the volume of circulating plasma,
2. Changes associated with rapid fluid and sodium loss,
3. Changes in the hepatic and the abdominal venous systems,
4. Reduction in the metabolic capacity of the liver,
5. Inhibition of gastrointestinal evacuatory activity in DI,
6. Dysbiotic changes.
DI Effects on Biochemical Parameters of Blood and Urine
Biochemical tests revealed changes in blood and urine protein content (Trifonova et al., 2010; Pastushkova et al., 2011; Vorontsov et al., 2014). Specifically, there is a decrease of muscle and cardiac constellation ferments activity, increase of lipid metabolism derivatives, increase of erythrocytes concentration, decrease in glucose tolerance and hypercholesterolemia, and increase of insulin and serum NT-proBNP levels, which is a proxy measure for brain natriuretic peptide (Ivanova et al., 2008; Markin et al., 2008; Navasiolava et al., 2011c; Coupe et al., 2013; Pastushkova et al., 2014; De Abreu et al., 2017). The new series of DI studies introduced the analysis of blood plasma proteome (Pastushkova et al., 2012). On DI days 2 and 3, growth of peak areas was observed in fragments of complement system proteins C3 and C4, high-molecular kininogen and fibrinogen. Significant increases of the peak area of apolipoprotein CI (reduced form with segregated threonine and proline) and C4 enzymes of the complement system, and fibrinogen on the 1st day after the experiment can be related to changes in motor activities of the subjects.
Analysis of the hemostasis system did not reveal any significant changes, although a tendency in decrease of antithrombin III activity (ATIII), protein C and plasminogen after exposure to 7-day DI was observed (Kuzichkin et al., 2010). Studies of antioxidant protection and lipid peroxidation rates did not reveal changes in their values during adaptation to DI. However, the return to normal activity after DI was associated with the development of a significant stress reaction, as evidenced by the strengthening of the lipoperoxidation, and a decrease in the functional activity of the antioxidant defense system (Zhuravleva et al., 2012).
In summary, the effects of DI on the biochemical composition of blood include: changes in the concentration of protein and lipid derivatives.
DI Effects on Skeletal System
It is not surprising that there are not many studies dedicated to bone marker changes in DI, given that the skeletal system is quite inert and changes occurring throughout a relatively short exposure to DI, such as 7 days, are not expected. There were no effects of 7-day DI on bone formation or increase of bone resorption in the study of Kopp (2008). A recent study performed during 3-day DI, however, revealed significant decrement of bone formation markers, namely total procollagen type I N- and C-terminal propetides and osteoprotegerin (Linossier et al., 2017).
DI Effects on Immune System
Studies of the DI effects on innate and acquired immunity revealed high variability of changes during DI (Berendeeva et al., 2011; Ponomarev et al., 2013). Observed negative shifts in congenital immunity at the end of 5-day DI and during recovery, suggest that there is an increased risk of infectious diseases, for instance caused by changes in normal microflora which normally does not cause pathological effects (Ponomarev et al., 2013). There were changes in the content of immunoglobulin (sIgA, IgA, and IgM) in the gingival fluid, which can reflect inflammation process in the periodontal tissue. Presence in the oral cavity of periodontopathogenic type of bacteria, namely, Prevotella intermedia, Tannerella forsythia, Treponema denticola, Aggregatibacter actinomycetemcomitans, Porphyromonas gingivalis, was also noted (Nosovsky et al., 2017).
A study of the neuroendocrine regulation during DI has demonstrated a decrease of triiodothyronine and cortisol levels, as well as an increase of prolactin and thyroxine concentration (Nichiporuk et al., 2011; De Abreu et al., 2017). The rate of changes in immunity parameters among different subjects demonstrated the highest frequency in non-anxious and extravert individuals on day-5 in DI (Nichiporuk et al., 2011).
Effects of DI on Thermoregulation
Exposure to short and long-term space flights is followed by gradual increase of core body temperature by 1°C over 2.5 months of flight, which was suggested to be associated with augmented concentrations of interleukin-1 receptor antagonist, a key anti-inflammatory protein (Stahn et al., 2017). Unfortunately, there no published studies on thermoregulation during DI, except for one report on the lack of significant changes in the body temperature on the day 3 of DI (De Abreu et al., 2017).
DI Results in the Back Pain Phenomenon
A recent series of DI experiments explored the potential mechanisms of the back pain which is regularly observed at the beginning of space missions and under ground-based conditions. Almost all DI study participants report back pain during the first 2–3 days of DI (Rukavishnikov et al., 2014a,b, 2015, 2017a,b; Kozlovskaya et al., 2015; Treffel et al., 2017). Retrospective analysis of the data from nine of the most recent DI experiments conducted at IBMP (2006–2018) demonstrated that 70 out of 87 participants reported back pain with an intensity ranging from 4–5 to 9–10 points on a ten-mark subjective scale (see Figure 3) (Rukavishnikov et al., 2014b).
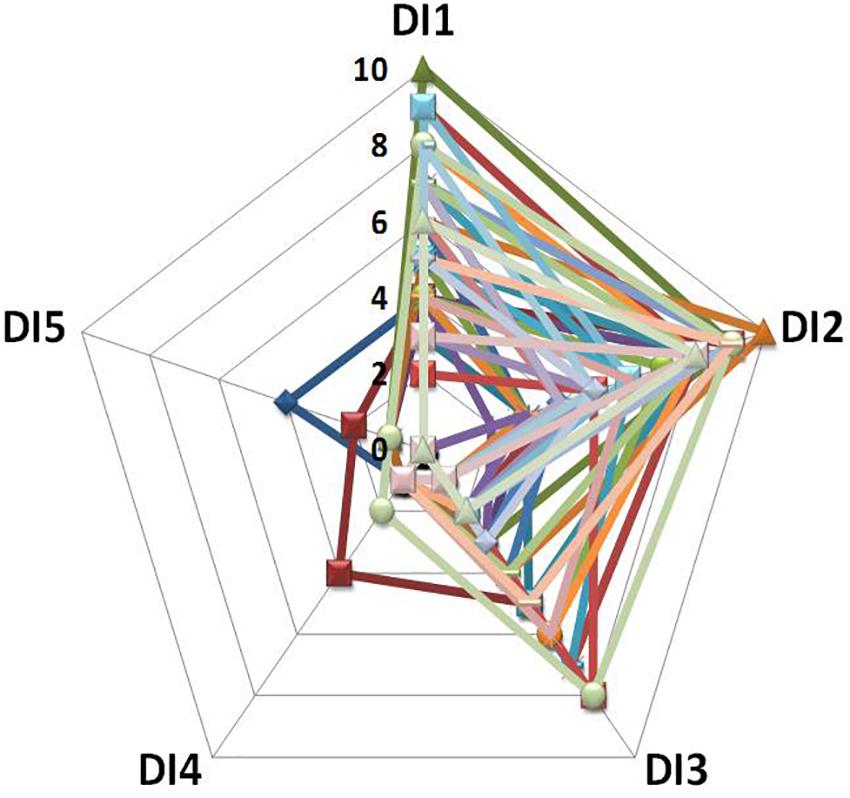
Figure 3. Intensity and duration of back pain during DI: data from 39 participants (Rukavishnikov et al., 2014b). X-axis: day of DI; Y-axis: pain intensity on a 10-point subjective scale.
The findings also revealed a decrease of back extensor transverse stiffness (Rukavishnikov et al., 2017a,b, 2018). Data obtained during magnetic resonance imaging (MRI) also showed that vertebral column length significantly increased in the neck, thoracic and lumbar parts of the spine (Kozlovskaya et al., 2015; Rukavishnikov et al., 2015; Treffel et al., 2016a,b). At the same time, the height of intervertebral disks increased, the disks swelling was observed, the neck and thoracic kyphosis were flattened, as were the thoracic and lumbar lordosis. MRI analysis revealed a decreased cross sectional area of mm. quadratus lumborum, multifidus, and erector spinae at the level of L4–L5 (Rukavishnikov et al., 2017b). It has been proposed that each of these factors can contribute to the hypogravitational back pain, including intervertebral disk swelling, spine lengthening, influence on the yellow ligament of the spine and back muscle atonia (Treffel et al., 2016b; Rukavishnikov et al., 2017b).
Contribution of DI Model to Space Flight Countermeasure System Development
As indicated at the beginning of this review, DI has been developed to investigate in depth the mechanisms of the effects of microgravity on the human body. Logically, DI has become instrumental in the development of a countermeasure system to prevent negative physiological effects of space flights. The history and extent of the studies employing the DI model and leading to the tests, comparison, and optimization of various countermeasure approaches, worth to be discussed in a separate review. In this section, we focus on the major outcomes which originated the design of the current countermeasure programs for use on board the International Space Station.
In the late 1970s, studies of short arm centrifuge were performed (Shulzhenko and Vill-Villiams, 1976; Grigoriev and Shulzhenko, 1979; Vil-Villiams and Shulzhenko, 1980; Clement and Pavy-Le Traon, 2004). The experiments performed under conditions of 13- and 56-day DI have shown that application of short arm centrifuge (SAC) 0,5–1 Gz rotations in the course of DI was followed by maintenance of back-up capabilities of cardiovascular system and external respiration and increase of resistance to 5 min +3 Gz rotations in “head-pelvis” direction. Combination of SAC sessions with water-salt supplements and 2340 kg physical loads (hand and leg exercises with expanders) increased countermeasure effects in this case (Shulzhenko and Vill-Villiams, 1976; Kokova, 1983).
Later, the number of DI studies aimed to examine the effects of different countermeasure means were performed. For instance, the studies of the efficacy of antigravity suit of bladderless type in maintenance of orthostatic tolerance have been carried out under conditions of 7-day DI (Shulzhenko et al., 1983b; Vil-Villiams et al., 1996). With the suit “Centaur” on, the 20 min tilt test at 70 degrees induced less changes in blood pressure as compared with that without the suit. The maximum heart rate significantly decreased, minimum stroke volume and pulse pressure increased. As such, using DI model the authors revealed that the anti-G suit helps to increase orthostatic tolerance and can be used following space flights.
Early DI studies demonstrated that the application of 25 mm Hg negative pressure to the lower body (LBNP) can counteract the decrease in the orthostatic tolerance (Genin and Pestov, 1974). The water-salt additives combined with LBNP and physical training, increased the capacity of vascular bed and help to retain body fluids, sodium, and chloride during DI (Grigoriev and Shulzhenko, 1979).
The positive effect of hip occlusion cuffs in preventing orthostatic intolerance after 18-h immersion strongly depended on the duration of break between occlusion sessions: the best effect has been demonstrated in the case of 1-min break (the pressure consisted 25 mm Hg, the duration of training session – 7 h) (Genin and Pestov, 1974). The mechanisms of the countermeasure effects of occlusion cuffs revealed in DI experiments, are based on the pooling of blood in vessels of the lower extremities due to the occlusion of superficial veins and hence – decrease of blood volume that is shifted in cranial direction (At’kov and Bednenko, 1987).
During 28-day DI study, the efficacy of isolated and combined effects of SAC and veloergometry have been examined. The study has shown that veloergometry training in the regimen of 600 kg/min (10 min training - 10 min break) for 60 min twice a day was followed by increase of cardiac input and decrease of total peripheral resistance. Combination of veloergometry training with SAC (0,8–1,9 Hz twice a day for 60 min per session) enforced the countermeasure effect which was assessed by cardio-vascular system parameters (Vil-Villiams and Shulzhenko, 1980; Vil-Villiams, 1994).
Effects of low and high-frequency electrostimulation of leg muscles have been studied in the series of DI experiments (Koryak et al., 2008; Kozlovskaya, 2008; Eshmanova et al., 2009; Koryak, 2014, 2018; Solovieva et al., 2016). These studies demonstrated that daily use of low frequency stimulation can counteract the decrease of force-velocity properties in m. triceps surae, especially when the intensity of stimulation is rather high (more than 13 V). Comparative evaluation of the efficacy of low-frequency stimulation of the lower limb muscles versus high frequency stimulation during 7-days DI, showed superiority of the low-frequency stimulation in prevention of motor dysfunctions (Koryak et al., 2008; Kozlovskaya, 2008). During these experiments, positive effects of neuromuscular electrical stimulation were also associated with unloading of the right heart and revealed by HD ECG parameters and growth of the “myocardium” index (Eshmanova et al., 2009). The application of high frequency electromyostimulation prevented elevation in pepsinogen, pancreatic amylase, and bilirubin, predominantly within its unconjugated fraction, as well as an increase in insulin secretion; however, it did not affect the ultrasound patterns of the hemodynamic rearrangement in both the liver and the abdomen (Solovieva et al., 2016).
A wide program of DI studies was dedicated to the effects of mechanical stimulation of the plantar mechanoreceptors in locomotor regimens. These studies confirmed the hypothesis on the trigger role of support (weight bearing) afferentation in the hypogravitational motor syndrome development (Grigoriev et al., 2004; Kozlovskaya et al., 2007b; Layne and Forth, 2008). Application of the plantar mechanostimulation during six 20-min sessions per day (with the stimulation frequency of 75 and 120 steps/min and pressure 40 kPa) eliminated the negative effects of gravitational unloading on sensory-motor and neuromuscular systems, including the decline of extensor muscle stiffness and decrease of the maximal voluntary force; a significant decrease of the absolute force of the isometric contraction of single skinned muscle fibers; a prominent decline of the tonic muscle fibers transversal size; and the transformation of the myosin phenotype from slow to fast one (Popov et al., 2003; Grigoriev et al., 2004; Khusnutdinova et al., 2004; Litvinova et al., 2004; Miller et al., 2004; Moukhina et al., 2004; Shenkman et al., 2004a,b; Netreba et al., 2005; Kozlovskaya et al., 2007a,b). The results of studies of biomechanical characteristics of locomotions brought to the conclusion that the rates of mechanical foot stimulation applied in the experiment did not change energy expenditure in the muscles; however, they moderated the amplitude of angular knee joint movements following 7 days of DI (Melnik et al., 2006). Application of the support stimulation was also effective in preventing venous compliance increase and orthostatic intolerance (Vinogradova et al., 2002a).
Countermeasure effects of the soles support zones stimulation has been also demonstrated in the visual-vestibular function. The group of subjects without support stimulation exhibited marked omnidirectional deviations in the eye tracking parameters, whereas in the group of subjects with support stimulation, these parameters were similar to the baseline values. Support stimulation also stabilized the pursuit function of the eye making it less variant. However, there was no uniformity in the subjects’ reaction to stimulation, which infers that the methods of improving the eye pursuit function should be personally “molded” (Kornilova et al., 2004).
Rather few data can be found in the literature concerning the studies of countermeasure effects of different pharmacological means with use of DI model. In the studies of pain sensitivity under conditions of DI, no reduction in the morning pain sensitivity (which is typical for normal conditions) has been revealed. Such analgesic as ketorolac had no effect on pain sensitivity, when determining the pain threshold by method of thermo algometry. The authors noticed that DI substantially altered the pharmacokinetics of ketorolac, increasing the rate of absorption of the drug and reduction of its relative bioavailability and retention time in the blood. These results indicate that pain therapy schemes may be administered differently during space flight as compared with 1G (Baranov et al., 2015).
In 7-day DI experiments the pharyngeal microflora in 22 healthy volunteers has been studied. For prophylactic pharyngeal dysbiosis, two probiotic drugs were used: oral - “lactobacterin dry” and local -“lactobacterin immobilized on collagen.” Administration of oral probiotic was accompanied with growth of pharyngeal opportunistic microflora preventing translocation of intestinal microflora. Local probiotic, on the contrary, decreased opportunistic microflora in the pharynx, but was associated with gastrointestinal dysbiosis. It was concluded that the combination of topic and oral probiotics provides the maximally effective prophylaxis of pharyngeal dysbiosis during DI (Kryukov et al., 2007; Ilyin et al., 2008). In another 7-DI study, the effects of sydnocarb [3-(beta-phenylisopropyl)-N-phenyl carbamoyl sydnonimine] were investigated. The subjects exercised on a bicycle ergometer before and after water immersion. During exercises, ECG, heart rate, minute respiration volume, oxygen consumption, carbon dioxide production, cardiac output, oxygen pulse were recorded. The subjects who received placebo showed a significant decrease of oxygen consumption at a maximum workload. Those who were given sydnocarb maintained normal oxygen consumption during bicycle ergometry. The drug increased the workload per kg body weight, maintained physical work capacity, and improved the cardiovascular function after DI exposure (Anashkin and Belyaev, 1982). The positive effect of the pharmaceutical – alleviation of myocardium strain – was a result of amlodipin (slow calcium channel inhibitor) assistance to the coronary blood flow; however, it had a negative side-effect on orthostatic stability (Eshmanova et al., 2009).
The DI model was actively used during the development of the “Penguin” axial loading suit (Barer et al., 1998). The studies have revealed increased EMG amplitude in the lower extremities muscles in subjects who wore Penguin suit while exercising on a bicycle ergometer. The same studies revealed increased heart rate and metabolic rate values in the subjects during performing the step loading veloergometric test with Penguin use. Other DI studies have shown that the “Penguin” suit was effective in preventing hypogravitational hyperreflexia, peripheral microcirculation changes, height increase, back pain development and back muscle atonia, and a decrease in force-velocity properties (Barer et al., 1998; Shigueva et al., 2015b; Rukavishnikov et al., 2015; Suvorov et al., 2017).
Contribution of DI Model to the Terrestrial Medicine
The findings on the physiological effects of DI on various systems of the human body, found their clinical application starting from the 1980s. First, the effects of DI in muscle relaxation, decreasing skeletal muscles tone and eliminating muscle spasticity, were noted (Kozlovskaya I. B. and Semenova K. A., unpublished observations).
The DI has been applied in edema therapy, especially intractable ones induced by cardiovascular etiologies as well as by burns, liver cirrhosis, and renal disease. In individuals with the mentioned syndromes, the effects of 1.5–3 times diuresis increase after 4–6 h post DI was maintained during 2 days (Yunusov et al., 1985; Ivanov and Bogomazov, 1988).
During the 1990s, several studies utilized DI in therapy of hypertensive crisis (Kirichenko et al., 1988; Evdokimova et al., 1989; Ivanov and Makarova, 1990). The results revealed a significant and persistent hypotensive effect after 90 min of exposure to DI. The effects were pronounced through two different mechanisms: (a) via a decrease of elevated total peripheral vascular resistance (TPVR) and no changes in cardiac output, or (b) via a decrease of elevated cardiac output. These hemodynamic effects were attributed to sanogenetic mechanisms which optimize blood circulation during hypertension (Evdokimova et al., 1989).
Recent studies (Meigal et al., 2017) have revealed positive effect of short-term, up to 6 h, DI sessions in patients with either Parkinson’s disease or parkinsonism: the score of subscale I of Wein’s inventory for autonomic disorders has decreased by 45%, and of the subscale II – by 23%. In addition, the time of the choice reaction has significantly improved. These effects were also observed 2 weeks post the course of DI.
The DI is widely used in pediatrics as a component of rehabilitation programs for premature children, to normalize hemodynamics and prevent brain edema. Exposure to DI is also used for the rehabilitation of children with central nervous system disorders, such as perinatal encephalopathy and other perinatal pathology (Kazanskaya, 2008; Khan et al., 2015). The method is also widely used in children undergoing cerebral palsy therapy, since it is effective in lowering hypertonus and hyperactivity, and in reflex facilitation (Yatsuk, 2002; Titarenko et al., 2015).
On the contrary to negative effects of 3–7 days DI on immune system (see above), the positive effect of 40–50 min DI on immunological parameters were revealed. Namely, promoted normalization of the functional activity of T- helpers and the lower adhesive properties of lymphocytes, as well as reduction in the incidence and severity of neonatal infectious and inflammatory diseases have been demonstrated (Chasha et al., 2008).
The diagnostic value of DI in the early detection of neurological pathologies under normal conditions was suggested in joint Russian–Austrian experiments. After 24, 48, and 72 h of DI, some healthy participants showed neurological symptoms (such as cerebellar disintegration, deterioration in the function of peripheral nerves, posterior tract, as well as pyramid and extrapyramidal systems) (Gerstenbrand and Kozlovskaya, 1990; Berger et al., 2001). This phenomenon may be explained by the fact that some people have latent neurological deficiency (congenital or acquired as a result of injuries, infections, etc.), but the central nervous system’s high plasticity typically provides compensation for these conditions. The sensory conflict created by DI (changing the support and proprioceptive afferent inflow) removes compensatory mechanisms that are ineffective in the new conditions, and hidden neurological disorders are revealed.
Due to its relaxation effects, DI was also used in sports medicine for recovery of athletes after high intensity training (Radzievskiy and Radziyevskaya, 2007).
There are also medical contraindications to the use of DI. According to various experts, they include myocardial infarction, severe cardiac arrhythmia, chronic respiratory diseases with pulmonary heart decompensation, thrombophlebitis, severe shortness of breath at rest (Ivanov and Makarova, 1990), acute inflammatory processes and generalized lesions of the skin (Yatsuk, 2002). However, these considerations are mostly theoretical and no quantitative results have been reported.
Conclusion
Dry immersion reproduces to some extent many of the changes associated with spaceflight. DI can serve as a valid ground-based model for simulation of physiological effects of weightlessness for some systems. The magnitude and rate of physiological changes during DI makes this method advantageous as compared with other ground-based microgravity models. DI is instrumental in the design of countermeasure programs for use in space, especially, for passive countermeasures which do not require intensive motor activity of the subjects. DI may also have a range of clinical applications that warrant further study.
Author Contributions
ET wrote the draft of the manuscript and made its revisions. TS prepared the part on DI effects in sensory-motor system and designed the figures. DS contributed in the global revision and reorganizing of the manuscript. IR prepared the part on back pain phenomenon and implementation of the DI model in Earth medicine. IK made the revision of the manuscript and prepared the part of use of DI for countermeasures development.
Funding
This work was supported by the Russian Academy of Sciences (63.1) and grant RFBR 16-29-08320.
Conflict of Interest Statement
The authors declare that the research was conducted in the absence of any commercial or financial relationships that could be construed as a potential conflict of interest.
Acknowledgments
The authors thank the volunteers who participated in Dry Immersion experiments and organizer team of the experiments.
References
Afonin, B. V., and Goncharova, N. P. (2009). Condition of the digestive system when modeling the effects of weightlessness in the human body in conditions of immersion. Aviakosm Ekolog Med. 1, 11–14.
Afonin, B. V., and Sedova, E. A. (2012). Digestive system functioning during simulation of microgravity effects on humans by means of immersion. Hum. Physiol. 38, 776–780. doi: 10.1134/S036211971207002X
Afonin, B. V., Sedova, E. A., Gontcharova, N. P., and Solovieva, A. A. (2013). Investigation of the evacuation function of the gastrointestinal tract during a five-day Dry Immersion. Hum. Physiol. 39, 787–791. doi: 10.1134/S0362119713070013
Aleksandrova, E. A., Gogolev, K. I., and Shulzhenko, E. B. (1980). Comparative assessment of changes in the human body when antiorthostatic hypokinesia and immersion. Fiziologia Cheloveka 6, 978–983.
Amirova, L. E., Shishkin, N. V., Kitov, V. V., Saveko, A. A., Tomilovskaya, E. S., and Kozlovskaya, I. B. (2017). The role of visual feedback in control of human vertical stability before and after a 5-day Dry Immersion. Aviakosm Ekolog Med. 7, 31–37.
Anashkin, O. D., and Belyaev, S. M. (1982). Effect of sidnocarb in 7-day conditions of water immersion on condition of cardiorespiratory system during physical exercise. Space. Biol. Aerospace Med. 6, 28–31.
Arbeille, P., Avan, P., Treffel, L., Zuj, K., Normand, H., and Denise, P. (2017). Jugular and portal vein volume, middle cerebral vein velocity, and intracranial pressure in dry immersion. Aerosp. Med. Hum. Perform. 88, 1–6. doi: 10.3357/AMHP.4762.2017
At’kov, O. Y., and Bednenko, B. C. (1987). Hypokinesia, Weightlessness: Clinical and Physiological Aspects. Moscow: Nauka.
Avan, P., Rukavishnikov, I., Madero, B., Tomilovskaya, E., Normand, H., Kozlovskaya, I., et al. (2013). “Assessment of intracranial pressure changes with otoacoustic emission under conditions of simulated microgravity,” in Proceedings of 19th IAA Humans in Space Symposium, Cologne.
Bachl, N., Baron, R., Tschan, H., et al. (1992). “Development and implementation of the Motomir experiments on the Mir station,” in Proceedings of the Intern Space Year Conference (Munich: ESA), 147–151. doi: 10.1007/978-3-7091-9260-3_10
Badakva, A. M., Miller, N. V., and Eron, Y. N. (2007). Influence of water immersion to install eye in monkeys. Aviakosm Ekolog Med. 2, 49–53.
Baranov, M. V., Kovalev, A. S., Perfilov, D. F., Chernogorov, R. V., and Repenkova, L. G. (2015). Influence of simulated microgravity on the threshold of pain sensitivity in humans with single dose of ketorolac. Patol. Fiziol. Eksp. Ter. 59, 23–28.
Barer, A. S., Kozlovskaya, I. B., Tikhomirov, E. P., Sinigin, V. M., and Letkova, L. I. (1998). Effects of countermeasure loading suit Penguin on human metabolism during movements performance. Aviakosm Ekolog Med. 32, 4–8.
Barmin, V. A., Kreidich, Y. V., and Kozlovskaya, I. B. (1983). Influence of optokinetic stimulation and immersion on eye-head coordination in man. Physiologist 26, 35–40.
Baum, K., and Essfeld, D. (1999). Origin of back pain during bed rest: a new hypothesis. Eur. J. Med. Res. 4, 389–393.
Beckman, E. L., Koburn, K. R., Chambers, R. M. Deforest, Re, Augerson WS, and Benson, V. G. (1961). Physiologic changes observed in human subjects during zero G-simulation by immersion in water up to neck level. Aerospace Med. 32, 1031–1041.
Berendeeva, T. A., Rykova, M. P., Antropova, E. N., Larina, I. M., and Morukov, B. V. (2011). State of the immune system after 7-day Dry Immersion in human. Hum. Physiol. 37, 840–845. doi: 10.1134/S0362119711070073
Berg, H. (1996). Effects of Unloading on Skeletal Muscle Mass and Function in Man. Stockholm: Karolinska Institut.
Berger, M., Gerstenbrand, F., Kozlovskaya, I. B., Burlatchkova, N., Muigg, A., Sokolov, A., et al. (1992). “Eye, head and arm coordination and spinal reflexes in weightlessness – Monimir Experiment,” in Austrian Society for Aerospace Medicine (ASM), ed. Health from Space Research (Wien, NY: Springer),135–199. doi: 10.1007/978-3-7091-9260-3_9
Berger, M., Lechner-Steinleitner, S., and Kozlovskaya, I. (2001). “Neurological aspects in real and simulated spaceflight,” in 10 Years Space Biomedical Research and Development in Austria, ed. H. Hinghofer-Szalkay (Wien: Facultas Verlag), 19–34.
Bravy, Y. R., Bersenev, E. Y., and Missina, S. S. (2008). The influence of Dry Immersion on the mechanisms of metaboreflex regulation of hemodynamics with muscular work. Aviakosm Ekolog Med. 42, 40–45.
Bryanov, I. I., Kozerenko, O. P., and Kakurin, L. I. (1976). Space Flight on the Soyuz ships. Moscow: Science Publishers.
Buravkova, L. B., Larina, I. M., and Popova, I. A. (2003). Specific features of metabolism in humans performing a physical exercise test after 7-day Dry Immersion. Fiziologia Cheloveka 29, 82–89.
Chaika, A. M., Balahovsky, I. O., Legenkov, V. I., and Kiselev, R. K. (1982). Volume change of plasma and extracellular fluid mass of plasma proteins in the course of bedrest and immersion. Space Biol. Aerospace Med. 6, 22–28.
Chasha, T. V., Belikova, M. E., Sotnticova, N. Y., and Kudryashova, A. V. (2008). Impact of dry immersion on neonatal immunity. Russ. Pediatr. J. 4, 30–33.
Clement, G., Angerer, O., and Schmitt, D. (2003). Life in a spin: what has been learnt from space. ESA Bull. 116, 38–42.
Clement, G., and Pavy-Le Traon, A. (2004). Centrifugation as a countermeasure during actual and simulated microgravity: a review. Eur. J. Appl. Physiol. 92, 235–248. doi: 10.1007/s00421-004-1118-1
Coupe, M., Tomilovskaya, E., Larcher, F., Diquet, B., Pastushkova, L., Kozlovskaya, I., et al. (2013). Body fluid changes, cardiovascular deconditioning and metabolic impairment are reversed 24 hours after a 5-day Dry Immersion. Open J. Nephrol. 3, 13–24. doi: 10.4236/ojneph.2013.31004
De Abreu, S., Amirova, L., Murphy, R., Wallace, R., Twomey, L., Gauquelin-Koch, G., et al. (2017). Multi-system deconditioning in 3-day Dry Immersion without daily raise. Front. Physiol. 8:799. doi: 10.3389/fphys.2017.00799
De-Doncker, L., Picquet, F., and Falempin, M. (2000). Effects of cutaneous receptor stimulation on muscular atrophy developed in hindlimb unloading condition. J. Appl. Physiol. 89, 2344–2351. doi: 10.1152/jappl.2000.89.6.2344
Demangel, R., Treffel, L., Py, G., Brioche, T., Pagano, A. F., Bareille, M.-P., et al. (2017). Early structural and functional signature of 3-day human skeletal muscle disuse using the Dry Immersion model. J. Physiol. 595, 4301–4315. doi: 10.1113/JP273895
Dyachenko, A. I., Mehedova, E. A., and Shulagin, E. B. (2010). Respiratory impedance change in conditions of water immersion. Russ. J. Biomech. 1, 86–94.
Edgerton, V. R., and Roy, R. R. (1996). Neuromuscular adaptation to actual and simulated spaceflight. Handbook of Physiology. Environ. Physiol. The Gravitational Environment. New York, NY: Oxford University Press, 721–763.
Eron, Y. N., Miller, N. V., and Badakva, A. M. (2000). “Change of gaze fixation reaction characteristics in monkeys during the one-day water immersion,” in Proceeding of the conference “Physiology of Muscle Activity”, Glendale, AZ, 85–87.
Eshmanova, A. K., Ivanov, A. Z., and Kabulova, A. Z. (2009). ECG high resolution and dispersion ECG mapping under the influence of amlodipine and myostimulation against a background of a 7-day Dry Immersion. Aviakosm Ekolog Med. 43, 40–43.
Eshmanova, A. K., Luchitskaya, E. S., and Bayevsky, R. M. (2008). Study of vegetative regulation of blood circulation when exposed to 7-day Dry Immersion. Aviakosmichaskaia Ekolog Med. 5, 45–51.
Evdokimova, A. G., Radzevich, A. E., and Solovyov, F. V. (1989). Study of the orthostatic sustainability and condition central and peripheral hemodynamics in mode 7-day Dry Immersion in human with circulatory dystonia on hypertensive type. Space Biol. Aerospace Med. 5, 62–65.
Fomin, A. N. (1981). Fibrinogen of blood in 7-day water immersion and short-term space flight. Space Biol. Aerospace Med. 5, 83–85.
Gazenko, O. G., Genin, A. M., and Malkin, V. B. (1972). Weightlessness is studied on Earth. Science Life 4, 92–97.
Gazenko, O. G., Grigoriev, A. I., and Kozlovskaya, I. B. (1986). Mechanisms of acute and chronic effects of microgravity. Physiologist 29, 48–50.
Genin, A. M., and Galichiy, V. A. (1995). Features external respiratory and pulmonary hyperemia in humans when immersed. Aviakosm Ekolog Med. 4, 28–32.
Genin, A. M., Lakota, N. G., Chikov, I., and Shashkov, V. S. (1988a). Immersion in insulating suit as a new way to simulate the physiological effects of weightlessness. Space. Biol. Aerospace Med. 5, 80–85.
Genin, A. M., Modin, A. Y., and Shashkov, B. C. (1988b). Hemodynamics human water immersion in various postural variants of dives. Space Biol. Aerospace Med. 1, 7–10.
Genin, A. M., and Pestov, I. D. (1974). “Experimental substantiation of some methods of preventing unfavorable effects of weightlessness. Man in space flight,” in Proceedings Of IV Intern Symposium on Main Problems of Human Being in Space Environ (Moscow: Science Publisher), 76–90.
Genin, A. M., and Sorokin, P. A. (1969). Prolonged Restriction of Mobility as a Model of the Effects of Weightlessness on the Human Body. Problems of Space Biology. Moscow: Science Publishers.
Gerstenbrand, F., and Kozlovskaya, I. B. (1990). “Neurological findings after 72-hours exposure to water immersion,” in Life Science Research in Space, ed. V. David (Paris: ESA), 117–120.
Gevlich, G. I., Kozlovskaya, I. B., Zenija, R., and Valdis, K. (1983). Assessment of skeletal muscles tone using the method of transverse stiffness recording. Space Biol. Aviaspace Med. 5, 86–89.
Graveline, D. E., Balke, B., Mckenzie, R. E., and Hartman, B. (1961). Psychological effects of water-immersion induced hypodynamia. Aerospace Med. 32, 387–440.
Greenleaf, J. E. (1984). Physiological responses to prolonged bed rest and fluid immersion in humans. J. Appl. Physiol. 57, 619–633. doi: 10.1152/jappl.1984.57.3.619
Grigoriev, A. I., and Egorov, A. D. (1992). Physiological aspects of adaptation of main human body systems during and after space flight. Adv. Space Biol. Med. 2, 43–82. doi: 10.1016/S1569-2574(08)60017-9
Grigoriev, A. I., Kozlovskaya, I. B., and Shenkman, B. S. (2004). Role of support afferentation in organizing the tonic muscular system. Russ. Physiol. 5, 508–521.
Grigoriev, A. I., and Shulzhenko, E. B. (1979). Effect small gravitational loads on water-salt metabolism and renal function in humans after long-term immersion. Space Biol. Aerospace Med. 6, 27–31.
Grigorieva, H. P., and Kozlovskaya, I. B. (1983). Influence 7-day immersion unloading on speed-strength properties of skeletal muscle. Space Biol. Aerospace Med. 4, 21–25.
Grigorieva, H. P., and Kozlovskaya, I. B. (1985). Influence 7-day immersion hypokinesia on the characteristics of the precision movements. Space Biol. Aerospace Med. 19, 38–42.
Henneman, E., Somjen, G., and Carpenter, D. O. (1965). Functional significance of cell size in spinal motoneurons. J. Neurophysiol. 28, 560–580. doi: 10.1152/jn.1965.28.3.560
Ilyin, V. K., Kryukov, A. I, and Volozhin, A. I (2008). Study of disbiosis, developing in a Dry Immersion, and the methods of its correction. Aviakosm Ekolog Med. 5, 70–75.
Ilyina-Kakueva, E. I, Portugalov, V. V., and Krivenkova, N. P. (1979). Influence of physical exercise and electrical stimulation on metabolism in soleus muscle and its structure during hypokinesia. Russ. Biol. Aerospace Med. 2, 35–38.
Ilyina-Kakueva, E. I., and Kaplansky, A. S. (2005). Muscle atrophy in microgravity and modeling. Aerospace Biol. Environ. Med. 5, 43–49.
Ivanov, A. P., Potters, I. B., Davidkin, A. F., and Lavrov, V. I. (1983). Changes some of the blood rheology in experiments that simulate weightlessness. Space Biol. Aerospace Med. 6, 25–30.
Ivanov, G. G., Baevsky, R. M., Bersenev, E. Y., Rusanova, V. B., Larina, I. M., and Pastushkova, L. K. H. (2011). Indices of electrocardiogram dispersion mapping during 5-day exposure in Dry Immersion. Aviakosm Ekolog Med. 6, 44–49.
Ivanov, S. G., and Bogomazov, E. E. (1988). Dry immersion and prospects of its application in the clinic. Space Biol. Aerospace Med. 5, 4–7.
Ivanov, S. G., and Makarova, L. I. (1990). Application of the method of Dry Immersion in treating hypertensive crisis. Space Biol. Aerospace Med. 1, 40–42.
Ivanova, S. M., Morukov, B. V., Labetskaya, O. I., and Yarlykova, Y. V. (2008). Morphofunctional properties of red blood cells in humans under conditions of 5-day immersion. Aviakosm Ekolog Med. 5, 49–51.
Iwase, S., Sugiyama, Y., Miwa, C., Kamiya, A., Mano, T., Ohira, Y., et al. (2000). Effects of three days of dry immersion on muscle sympathetic nerve activity and arterial blood pressure in humans. J. Auton. Nerv. Syst. 79, 156–164. doi: 10.1016/S0165-1838(99)00076-4
Kabulova, A. Z., and Eshmanova, A. K. (2008). “Comparison of changes in ECG and HRV BP in experiments with 7-day and 7-day immersion antiorthostatic hypokinesia,” in Proceedings 4 Russian Symposium with International Participation ”The Variability of Heart Rhythm: Theoretical Aspects and Practical Application, Moscow 110–113.
Kakurin, I., Cherepahin, M. A., and Pervushin, V. I (1971). Influence of short-term space flights on the State of human neuromuscular apparatus. Russ. Biol. Aerospace Med. 6, 53–56.
Kakurin, L. I. (1968). Influence of limited muscle activity in physiological systems of organism. Russ. Biol. Aerospace Med. 2, 59–71.
Kamenskiy, Y. N., Shulzhenko, E. B., and Andreev, V. (1976). Influence of systematic gravitational effects on respiratory function in conditions of prolonged immersion. Space Biol. Aerospace Med. 4, 40–45.
Kazanskaya, E. V. (2008). Physical rehabilitation in rehabilitation saying sounded at the preterm infants with perinatal CNS injuries. Sci. Notes P.f. Lesgaft. 12, 21–25.
Kermorgant, M., Leca, F., Nasr, N., Custaud, M.-A., Geeraets, T., Czosnyka, M., et al. (2017). Impacts of simulated weightlessness by Dry Immersion on optic nerve sheath diameter and cerebral autoregulation. Front. Physiol. 8:780. doi: 10.3389/fphys.2017.00780
Khan, M. A., Kuyantseva, L. V., and Novikova, E. V. (2015). Non-drug technologies of medical rehabilitation of children with perinatal pathology. Vestnik Vosstanovitel’noi Meditsiny 6, 22–26.
Khristova, L. G., Gidikov, A. A., and Aslanova, I. F. (1988). Influence of immersion hypokinesia on potentials of human muscle motor units. Space Biol. Aerospace Med. 4, 39–43.
Khusnutdinova, D., Netreba, A., and Kozlovskaya, I. (2004). Mechanic stimulation of the soles support zones as a countermeasure of the contractile properties decline under microgravity conditions. J. Gravit. Physiol. 11, 141–142.
Kirenskaya, A. V., Kozlovskaya, I. B., and Sirota, M. G. (1985). Influence of immersion on the characteristics of hypokinesia arbitrary movements software type. Space Biol. Aerospace Med. 6, 27–31.
Kirenskaya, A. V., Kozlovskaya, I. B., and Sirota, M. G. (1986). Influence of immersion on the characteristics of hypokinesia rhythmic activity of soleus muscle motor units. Hum. Physiol. 1, 617–632.
Kirenskaya, A. V., Tomilovskaya, E. S., Novototsky-Vlasov, V. Y., and Kozlovskaya, I. B. (2006). Influence of microgravity on characteristics of slow presaccadic potentials. Hum. Physiol. 2, 1–10.
Kirichenko, L., Masenko, V. P., Raskurazheva, A. B., and Evdokimova, A. G. (1988). Indicators homeostasis in patients with circulatory dystonia under Dry Immersion conditions. Space Biol. Aerospace Med. 1, 10–13.
Kokova, N. I. (1983). Influence water-salt supplements to the diet on portability overloads direction head-pelvis after 7-day Dry Immersion and in normal mode motor activity. Space Biol. Aerospace Med. 4, 33–37.
Kopp, O. (2008). Effects of Dry Water Immersion on Bone Metabolism (Auswirkungen der trockenen Wasserimmersion auf den Knochenstoffwechsel). Ph.D. thesis, University of Bonn, Germany.
Kornilova, L. N. (1997). Vestibular function and sensory interaction in altered gravity. Adv. Space Biol. Med. 6, 275–313. doi: 10.1016/S1569-2574(08)60087-8
Kornilova, L. N., Alyochina, M. I., Temnikova, V. V., and Azarov, K. A. (2004). Pursuit function of the eye during 7-day dry immersion without and with a support stimulator. Aviakosm Ekolog Med. 38, 41–48.
Kornilova, L. N., Glukhih, D. O., Naumov, I. A., Habarova, E. V., Ekimovskiy, G. A., Pavlova, A. S., et al. (2016). Effect of optokinetic stimulation on visual–manual tracking under the conditions of support-proprioceptive deprivation. Hum. Physiol. 42, 508–519. doi: 10.1134/S0362119716040071
Kornilova, L. N., Naumov, I. A., and Gluchih, D. O. (2011a). Visually-manual function eyes in the 5-day immersion. Aviakosm Ekolog Med. 6, 8–13.
Kornilova, L. N., Naumov, I. A., and Makarova, S. M. (2011b). Effect of real and simulated weightlessness on the characteristics of the static otolith reflex. Hum. Physiol. 37, 85–92. doi: 10.1134/S0362119711010075
Kornilova, L. N., Naumov, I. A., Glukhih, D. O., Habarova, E. V., and Kozlovskaya, I. B. (2013). The effects of support-proprioceptive deprivation on visual-manual tracking and vestibular function. Hum. Physiol. 39, 462–471.
Kornilova, L. N., Naumov, I. A., Mazurenko, A. Y., and Kozlovskaya, I. B. (2008). Visual-Manual tracking and vestibular function during 7-day Dry Immersion. Aviakosm Ekolog Med. 42, 8–13. doi: 10.1134/S0362119713050071
Koryak, Y. (1998). Electromyographic study of the contractile and electrical properties of the human triceps surae muscle in a simulated microgravity environment. J. Physiol. 510, 287–295. doi: 10.1111/j.1469-7793.1998.287bz.x
Koryak, Y. (1999). Electrical and contractile parameters of muscle in man: effects of 7-day ”dry” water immersion. Aviat. Space Environ. Med. 70, 459–464.
Koryak, Y. A. (2014). Architectural and functional features of human triceps surae muscles during electro stimulation training in conditions of mechanical unweighting. Vestnik RAEN 1, 106–113.
Koryak, Y. A., Kozlovskaya, I. B., and Khimoroda, N. N. (2008). Low-frequency neuromuscular electrical stimulation training of human skeletal muscles in conditions of gravitational unloading. Eur. J. Nat. Hist. 1, 86–90.
Koryak, Y. U. (2001). Electrically evoked and voluntary properties of the human triceps surae muscle: effects of long-term spaceflights. Acta Physiol. Pharmacol. Bulg. 26, 21–27.
Koryak, Y. A. (2018). Neuromuscular electrical stimulation in conditions of gravitational unloading. Sci. Eur. 23, 3–10.
Kozinets, G. I., Belakovsky, M. S., Ushakov, A. S., Bykova, I. A., and Matveenko, V. P. (1983). Structural and functional changes of erythrocytes and leukocytes in human terms 7-day immersion hypokinesia. Space Biol. Aerospace Med. 3, 48–51.
Kozlovskaya, I., Dmitrieva, I., Grigorieva, L., et al. (1988). “Gravitational mechanisms in the motor system. Studies in real and simulated weightlessness,” in Stance and Motion, eds V. S. Gurfinkel, M. Y. Ioffe, and J. Massion (New York, NY: Plenum), 37–48.
Kozlovskaya, I. B. (2007). Gravitational Mechanisms in the Motor System. Modern Classical Physiology Course. Moscow: Geotar-Media.
Kozlovskaya, I. B. (2008). Fundamental and applied problems of immersion studies. Aviakosm Ekolog Med. 42, 3–7.
Kozlovskaya, I. B., Barmin, V. A., Stepantsov, V. I., and Kharitonov, N. M. (1990). Results of studies of motor functions in long-term space flights. Physiologist 33, S1–S3.
Kozlovskaya, I. B., Grigoriev, H. P., and Gevlich, A. I. (1984). Comparative analysis of the influences of weightlessness and its models on speed-strength properties and skeletal muscle tone. Russ. Biol. Aerospace Med. 6, 22–25.
Kozlovskaya, I. B., Maximov, D. A., Voronkov, Y. I, Sun, I., Ardashev, V. N., Dorogan-Suschev, I. G., et al. (2015). Changes in lumbar segment of the spine and back pain under exposure to 3-day Dry Immersion. Kremlevskaya Med. Klinicheskiy Vestnik 2, 87–90.
Kozlovskaya, I. B., Sayenko, I. V., and Miller, T. F. (2007a). Erratum to: New approaches to counter measures of the negative effects of micro-gravity in long-term space flights. Acta Astronaut. 60, 783–789. doi: 10.1016/j.actaastro.2006.09.038
Kozlovskaya, I. B., Sayenko, I. V., Sayenko, D. G., et al. (2007b). Role of support afferentation in control of the tonic muscle activity. Acta Astronaut. 60, 285–294. doi: 10.1016/j.actaastro.2006.08.010
Kreidich, Y. V., Gazenko, O. G., Kozlovskaya, I. B., and Gregorian, R. A. (2007). Motor and vestibulomotor effects of microgravity induced by water immersion. Herald Natl. Acad. Sci. Belarus 1, 93–109.
Kreidich, Y. V., Repin, A. A., Barmin, V. A., and Kozlovskaya, I. B. (1982). Effect of immersion on the characteristics of hypokinesia, eye movements and head when carrying out the reaction in a person with sight installation. Russ. Biol. Aerospace Med. 5, 41–45.
Kryukov, A. I, Ilyin, V. K., and Volozhin, A. I (2007). Experimental substantiation of application of probiotic preparations in the face of hydroweightlessness. News Otorhinolaryngol. 5, 30–33.
Kuzichkin, D. S., Morukov, B. V., Markin, A. A., Juravlyova, O. A., Zabolotskaya, I. V., and Vostrikova, L. V. (2010). Hemostasis system indices after short-term space flights and during 7-day “dry” immersion. Hum. Physiol. 36, 478–482. doi: 10.1134/S0362119710040158
Kuznetsov, S. L., and Stepantsov, V. V. (1989). Ischerchennogo Reaction of human skeletal muscle fibers during long Antiorthostatic hypokinesia. Arch. Anat. Gistol. Jembriol. 7, 53–58.
Kuznetsov, S. L., and Stepantsov, V. V. (1990). Reaction fibers of skeletal muscle man on the 370-daily antiorthostatic hypokinesia, mixed with the physically demanding. Fam. Biol. Aerospace Med. 5, 34–38.
Kuznetsova, G. D., Gabova, A. V., Lazarev, I. E., Obukhov, Y. V., Obukhov, K. Y., Morozov, A. A., et al. (2015). Frequency-temporal structure of human electroencephalogram in the condition of artificial hypogravity: Dry Immersion model. Aviakosm Ekolog Med. 49, 25–32.
Larina, I. M., Baevsky, R. M., Pastushkova, L. K., Eshmanova, A. K., Luchitskaya, E. S., Navasiolava, N. M., et al. (2011). Seven-day dry immersion: interrelationship between the changes in the water-electrolyte balance and cardiovascular responses. Hum. Physiol. 37, 602–609. doi: 10.1134/S0362119711050124
Larina, I. M., Custaud, M. A., and Pastushkova, L. H. (2008). Status of water-electrolyte exchange, kidney and skin of the Microcirculatory bed surveyed during the 7-day Dry Immersion. Aviakosm Ekolog Med. 5, 29–34.
Larina, I. M., and Lakota, N. G. (2000). Role individual heat and water-electrolytic sensitivity in conditions of water immersion. Aviakos Ekolog Med. 6, 16–22.
Larina, I. M., Sukhanov, Y. V., and Lakota, N. G. (1999). Mechanisms early water-electrolyte exchange reactions in humans in different ground models of the effects of microgravity. Aviakosm Ekolog Med. 4, 17–23.
Layne, C. S., and Forth, K. E. (2008). Plantar stimulation as a possible countermeasure to microgravity-induced neuromotor degradation. Aviat Space Environ. Med. 79, 787–794. doi: 10.3357/ASEM.2293.2008
Lazarev, I. E., Tomilovskaya, E. S., and Kozlovskaya, I. B. (2018). Resting state brain activity during long-term dry immersion. Aerospace Med. Hum. Perform. 89, 1–6. doi: 10.3357/AMHP.4972.2018
Linossier, M.-T., Amirova, L. E., Thomas, M., Normand, M., Bareille, M.-P., Gauquelin-Koch, G., et al. (2017). Effects of short-term dry immersion on bone remodeling markers, insulin and adipokines. PLoS One 12:e0182970. doi: 10.1371/journal.pone.0182970
Litvinova, K. S., Vikhlyantsev, I. M., Kozlovskaya, I. B., Podlubnaya, Z. A., and Shenkman, B. S. (2004). Effects of artificial support stimulation on fiber and molecular characteristics of soleus muscle in men exposed to 7-day dry immersion. J. Gravit. Physiol. 11, 131–132.
Mardanov, R. G., Orlov, O. I., Tsarkov, D. S., and Mukhamedieva, L. N. (2011). Volatile metabolites in expired air of humans during 5-day Dry Immersion. Aviakosm Ekolog Med. 45, 41–43.
Markin, A. A., Morukov, B. V., and Zhuravleva, O. A. (2008). Dynamics of biochemical parameters of blood in an experiment with 7-day immersion. Aviakosm Ekolog Med. 5, 56–60.
Meigal, A. Y., Gerasimova-Meigal, L. I, Saenko, I. V., Subbotina, N. S., Tret’yakova, O. G., and Chernikova, L. A. (2017). The effect of “dry” immersion as microgravity analogue on neurological symptoms in parkinsonism. Aviakosm Ekolog Med. 51, 53–59.
Melnik, K. A., Artamonov, A. L., Miller, T. F., and Ravens, A. V. (2006). Effects of mechanic stimulation of the foot support zones on locomotion kinematics during 7-day dry immersion. Aviakosm Ekolog Med. 5, 61–65.
Mikhailov, V. M., Reushkin, V. N., Reushkina, G. D., Sebekina, T. V., and Smirnova, T. M. (1995). Using variance analysis for the influence of immersion and individuality on the variability of orthostatic reactions. Aviakosm Ekolog Med. 6, 26–32.
Mikhailovskaya, A. N., Osipova, A. A., Dyachenko, A. I., and Kolesnikov, V. I. (2011). Biomechanics and regulation of the external respiration in the conditions of 5-day dry immersion. Aviakosm Ekolog Med. 6,32–37.
Miller, T., Ivanov, O., Galanov, D., et al. (2005). Mechanic stimulation of the foot support zones as a way to maintain activity of the tonic muscular system during functional support deprivation. J. Gravit. Physiol. 12, 149–150.
Miller, T. F., Saenko, I. V., Popov, D. V., et al. (2004). Effect of mechanical stimulation of the support zones of soles on the muscle stiffness in 7-day dry immersion. J. Gravit. Physiol. 11, 135–136.
Miller, T. F., Saenko, I. V., Popov, D. V., and Vinogradova, O. L. (2010). Effect of support deprivation and stimulation of the feet support zones on the characteristics of cross stiffness and electromyogram of resting muscles of the calf in 7-day immersion. Aviakosm Ekolog Med. 6, 13–18.
Moreva, T. I. (2008). Peripheral hemodynamics Study method of Ultrasonic Doppler in the face 7-day immersion. Aviakosm Ekolog Med. 5, 35–40.
Moreva, T. I., Kriushev, E. S., Moreva, O. V., and Pasekova, O. B. (2018). Effect of dry immersion on blood flow in jugular veins and cerebral venous sinus: ultrasonic methods of investigation. Aviakosm Ekolog Med. 52, 48–54. doi: 10.21687/0233-528X-2018-52-2-48-53
Moukhina, A., Shenkman, B., and Blottner, D. (2004). Effects of support stimulation on human soleus fiber characteristics during exposure to “dry” immersion. J. Gravit. Physiol. 11, 137–138.
Navasiolava, N., Dignat-George, F., Sabatier, F., Larina, I. M., Demiot, C., Fortrat, J.-O., et al. (2010). Enforced physical inactivity increases endothelial microparticle levels in healthy volunteers. Am. J. Physiol. Heart Circ. Physiol. 299, 248–256. doi: 10.1152/ajpheart.00152.2010
Navasiolava, N. M., Custaud, M.-A., Tomilovskaya, E. S., et al. (2011a). Long-term dry immersion: review and prospects. Eur. J. Appl. Physiol. 111, 1235–1261. doi: 10.1007/s00421-010-1750-x
Navasiolava, N. M., de Germain, V., and Levrard, T. (2011b). Skin vascular resistance in the standing position increases significantly after 7 days of dry immersion. Auton. Neurosci. 160, 64–68. doi: 10.1016/j.autneu.2010.10.003
Navasiolava, N., Pajot, P., Gallois, Y., Pastushkova, L., Kulchitsky, V., Gauquelin-Koch, G., et al. (2011c). NT-ProBNP levels, water and sodium homeostasis in healthy men: effects of 7 days of dry immersion. Eur. J. Appl. Physiol. 111, 2229–2231. doi: 10.1007/s00421-011-1858-7
Nemirovskaya, T. L., and Shenkman, B. S. (2002). Effects of support stimulation on unloaded soleus in rat. Eur. J. Appl. Physiol. 87, 120–126. doi: 10.1007/s00421-002-0603-7
Nesterovskaya, A. Y., Morukov, B. V., and Vorontsov, A. (2008). Study on the impact of 7-day immersion in the excretory function of the kidneys. Aviakosm Ekolog Med. 5, 52–56.
Netreba, A. I., Khusnutdinova, D. R., Vinogradova, O. L., and Kozlovskaya, I. B. (2004). Effect of dry immersion in combination with stimulation of foot support zones upon muscle force–velocity characteristics. J. Gravit. Physiol. 11, 129–130.
Netreba, A. I., Khusnutdinova, D. R., Vinogradova, O. L., and Kozlovskaya, I. B. (2005). Effect of 7-days dry immersion in combination with mechanical stimulation of foot support zones upon resistance to fatigue of knee extensors and flexors. J. Gravit. Physiol. 12, 137–138.
Netreba, A. I., Khusnutdinova, D. R., Vinogradova, O. L., and Kozlovskaya, I. B. (2006). Effect of dry immersion of various durations in combination with artificial stimulation of foot support zones upon force–velocity characteristics of knee extensors. J. Gravit. Physiol. 13, 71–72.
Nichiporuk, I. A., Vasilyev, G. Y., and Rykov, M. P. (2011). Analysis of the relationships between the psychophysiological status and system of adaptive immunity in the conditions OF 5-day dry immersion. Aviakosm Ekolog Med. 6, 57–64.
Noskov, V. B., Larina, I. M., and Pastushkova, L. H. (2011). Renal functioning and human body liquids during 5-day dry immersion. Aviakosm Ekolog Med. 6, 22–26.
Nosovsky, A. M., Solovieva, Z. O., and Ilyin, V. K. (2017). Application of the analysis of variance for an estimation of change of immunological parameters in gingival fluid of the cosmonauts at long space flights and subjects in conditions of Dry Immersion. Biol. Sci. 1, 117–122.
Oganov, V. S., Kozlova, V. G., Ilyina, E. A., and Rakhmanov, A. S. (1980). Dynamics of functional state changes of human neuro-muscular system under conditions of prolonged hypokinezia. Actual Probl. Space Biol. Med. 187–189.
Ogneva, I. V., Ponomareva, E. V., Kartashkina, N. L., and Altaeva, E. G. (2011). Decrease of contractile properties and transversal stiffness of single fibers in human soleus after 7-day “dry” immersion. Acta Astronautica 68, 1478–1485. doi: 10.1016/j.actaastro.2010.01.012
Ogoh, S., Hirasawa, A., de Abreu, S., Denise, P., and Normand, H. (2017). Internal carotid, external carotid and vertebral artery blood flow responses to a 3-day of head-out dry immersion. Exp. Physiol. 102, 1278–1287. doi: 10.1113/EP086507
Orlov, V. N. (1985). Influence of Dry Immersion model on the performance of water-salt exchange, aldosterone and cortisol level in plasma in patients with different degree of hydration of the body. Space Biol. Aerospace Med. 4, 42–45.
Otelin, A. A., Mashansky, V. F., and Mirkin, A. S. (1976). Fatér-Pacini bodies: Structure Function Features. Leningrad: Nauka, 176.
Ovsyannikov, A. V. (1972). Features of reorganization in the motor system under water immersion. J. Physiol. USSR 3, 305–310.
Pastushkova, L. H., Paharukova, N. A., Trifonova, O. P., et al. (2011). Direct proteome profiling of human blood serum in the experiment with 5-day dry immersion. Aviakosm Ekolog Med. 6, 13–18.
Pastushkova, L. K., Dobrokhotov, I. V., Veselova, O. M., Larina, I. M., Tiys, E. S., Kononikhin, A. S., et al. (2014). Identification of proteins of cardiovascular system in healthy subjects’ urine during “dry” immersion. Hum. Physiol. 40, 330–339. doi: 10.1134/S0362119714030128
Pastushkova, L. K., Pakharukova, N. A., Novoselova, N. M., Dobrokhotov, I. V., Valeeva, O. A., Custaud, M.-A., et al. (2012). Direct proteomic profiling of human urine and blood serum in an experiment with 5-day dry immersion. Aviakosm Ekolog Med. 46, 31–37.
Ponomarev, S. A., Antropova, E. N., Rykova, M. P., Berendeeva, T. A., and Morukov, B. V. (2013). Human innate immunity under the conditions of 5-day Dry Immersion. Hum. Physiol. 39, 780–786. doi: 10.1134/S0362119713070141
Popov, D. V., Saenko, I. V., Vinogradova, O. L., and Kozlovskaya, I. B. (2003). Mechanical stimulation of foot support zones for preventing unfavourable effects of gravitational unloading. J. Gravit. Physiol. 10, 59–60.
Popova, Y. A., Suvorov, A. V., Dyachenko, A. I, and Kolesnikov, V. I (2011). Biomechanics and regulation of the external respiration in the conditions of 5-day dry immersion. Aviakosma Ekolog Med. 45, 26–32.
Radzievskiy, P. A., and Radziyevskaya, M. P. (2007). Dry Immersion-effective physiotherapeutic procedure in system recovery sportsmen/athletes. Pedagogy Psychol. Med. Biol. Probl. Phys. Train. Sport 10, 116–121.
Repin, A. (1981). Study of Mechanisms of Cerebellum Control Vestibulo-Oculomotor Responses. Master’s thesis, IBMP RAS, Moscow.
Reschke, M. F., Jacob, J., Bloomberg, J. J., et al. (1998). Posture, locomotion, spatial orientation, and motion sickness as a function of space flight. Brain Res. Rev. 28, 102–117. doi: 10.1016/S0165-0173(98)00031-9
Rukavishnikov, I. V., Amirova, L. E., Kitov, V. V., Tomilovskaya, E. S., and Kozlovskaya, I. B. (2018). “MRI study of structural and functional changes of back muscles and spine under conditions of Dry Immersion,” in Proceedings of the 42nd assembly Cospar 2018,Pasadena, CA.
Rukavishnikov, I. V., Amirova, L. E., Kukoba, T. B., Tomilovskaya, E. S., and Kozlovskaya, I. B. (2017a). Effects of gravitational unloading on back muscles tone. Hum. Physiol. 43, 291–300. doi: 10.1134/S0362119717030173
Rukavishnikov, I. V., Amirova, L. E., Tomilovskaya, E. S., and Kozlovskaya, I. B. (2017b). “Structural-functional changes of the spine under conditions of supportlessness,” in Proceedings of XXIII Meeting of Physiological Society, ed. I. P. Pavlov (Voronezh: ≪Istoki≫), 2397.
Rukavishnikov, I. V., Dmitrieva, L. E., Kukoba, T. B., Tomilovskaya, E. S., and Kozlovskaya, I. B. (2014a). “Effects of 6-hours and 3-days support withdrawal on back muscles tone,” in Proceedings of the 39 Academic Conference on Cosmonautics Dedicated to S.P. Korolyov, Moscow.
Rukavishnikov, I. V., Dmitrieva, L. E., Tomilovskaya, E. S., and Kozlovskaya, I. B. (2015). “Possible mechanisms of back pain development in microgravity,” in Proceedings of the 11 International Scientific-Practical Conference “Piloted Space Flights”, Tomsk.
Rukavishnikov, I. V., Tomilovskaya, E. S., and Kozlovskaya, I. B. (2014b). “Peculiarities of medical support in Dry Immersion experiment,” in Proceedings of the Head out Water Immersion Symposium and Baroreflex and Heart Rate Variability Group, April 28th-30th 2014, Angers.
Rukavishnikov, I. V., Tomilovskaya, E. S., Matsnev, E. I., Denise, P., and Avan, P. (2013). Otoacoustic emission as a mediate method of intracranial pressure assesement under conditions of simulation of microgravity physiological effects. Aviakosm Ekolog Med. 47:130.
Saenko, I. (2007). Activity Characteristics of Spinal Mechanisms Under Microgravity Conditions. Master’s thesis, IBMP RAS, Moscow.
Sayenko, D. G., Miller, T. F., Melnik, K. A., Netreba, A. I., Khusnutdinova, D. R., Kitov, V. V., et al. (2016). Acute effects of dry immersion on kinematic characteristics of postural corrective responses. Acta Astronautica 121, 110–115. doi: 10.1016/j.actaastro.2015.12.054
Semenov, Y. S., Popova, Y. A., and Dyachenko, A. I (2011). Characteristics of negative pressure inspiration effect on the cardiorespiratory system of humans in the conditions of 5-day dry immersion. Aviakosm Ekolog Med. 45, 37–41.
Shenkman, B., Nemirovskaya, T., and Cheglova, I. (1999). Morphological characteristics of m. vastus lateralis in a supportive environment. Rep. Russ. Acad. Sci. 4, 563–565.
Shenkman, B. S. (2016). From slow to fast: hypogravity-induced remodeling of muscle fiber myosin phenotype. Acta Nat. 8, 47–59.
Shenkman, B. S., Grigoriev, A. I., and Kozlovskaya, I. B. (2017). Gravity mechanisms in tonic motor system. Neurophysiological and muscle aspects. Hum. Physiol. 43, 578–590. doi: 10.1134/S0362119717050140
Shenkman, B. S., Kozlovskaya, I. B., Nemirovskaya, T. L., and Tcheglova, I. A. (1997). Human muscle atrophy in supportlessness: effects of short-term exposure to dry immersion. J. Gravit. Physiol. 4, 137–138.
Shenkman, B. S., Litvinova, K. S., Nemirovskaya, T. L., et al. (2004a). Afferent and peripheral control of muscle fiber properties during gravitational unloading. J. Gravit. Physiol. 11, 111–114.
Shenkman, B. S., Podlubnaya, Z. A., and Vihlyantsev, I. M. (2004b). Contractile characteristics and sarcomeric cytoskeletal proteins of human soleus fibers in muscle unloading: role of mechanical stimulation from the support surface. Biophysics 5, 881–890.
Shulzhenko, E. B., Gogolev, K. I., and Belyaev, S. M. (1983a). Tolerance to physical activity after exposure to water immersion. Russ. Biol. Aerospace Med. 17, 40–45.
Shigueva, T. A., Zakirova, A. Z., Tomilovskaya, E. S., and Kozlovskaya, I. B. (2015a). Effects of support deprivation on the order of motor unit recruitment. Hum. Physiol. 41, 813–816. doi: 10.1134/S036211971507021X
Shigueva, T. A., Zakirova, A. Z., Tomilovskaya, E. S., and Kozlovskaya, I. B. (2015b). “Effects of axial loading suit “Penguin” on characteristics of spinal reflex under supportlessness conditions,” in Proceedings of the 11 International Scientific-Practical Conference “Piloted Space Flights”, Moscow.
Shpakov, A. V., Artamonov, Voronov, A. A., and Melnik, K. A. (2008). Influence of immersion hypokinesia on kinematic and electromyographic characteristics of human locomotion. Aviakosm Ekolog Med. 5, 24–29.
Shulzhenko, E. (1975). Physiological Effects of Altered Gravity (Model Experiments in Model). Master’s thesis, IBMP RAS, Moscow.
Shulzhenko, E. B., Kozlova, V. G., and Kudrin, K. A. (1983b). Antigravity suit as a tool of increasing orthostatic tolerance after water immersion and acceleration exposure. Space Biol. Aerospace Med. 6, 30–33.
Shulzhenko, E. B., Kozlova, V. G., Aleksandrova, E. A., and Kudrin, K. A. (1984). Adaptive effect of repeated immersion of the impacts on the human body. Space Biol. Aerospace Med. 18, 57–59.
Shulzhenko, E. B., and Vill-Villiams, I. F. (1975). Imitation Detraining Organism by Dry Immersion. Moscow: XI reading K.E. Tsiolkovsky.
Shulzhenko, E. B., and Vill-Villiams, I. F. (1976). The opportunity to conduct long-term water immersion method Dry Immersion. Russ. Biol. Aerospace Med. 9, 82–84.
Solovieva, A., Sedova, E., Tomilovskaya, E., Shigueva, T., and Afonin, B. (2016). Functional activity of the liver under the conditions of immersion and effects of the countermeasures. Hum. Physiol. 42, 740–746. doi: 10.1134/S036211971607015X
Solovieva, Z., Ilyin, V., Nosovsky, A., Batov, A., and Savina, N. (2011). Changes of microbiological parameters of mucous membranes of the top respiratory ways under conditions of Dry Immersion. Technol. Zhivih Syst. 8, 61–64.
Sosnina, I. S., Zelenskiy, K. A., Pomelov, R. S., and Tomilovskaya, E. S. (2016). “Study of the effects of support withdrawal on accuracy of voluntary movements in the task of spatial reproduction of simple figures,” in Proceedings of the 12 International Congress “Neuroscience for Med and Psycol”, Philadelphia, PA, 377–378.
Stahn, A. C., Werner, A., Opatz, O., Maggioni, M. A., Steinach, M., Weller, et al. (2017). Increased core body temperature in astronauts during long-duration space missions. Sci. Rep. 7:16180. doi: 10.1038/s41598-017-15560-w
Stoida, Y. M., Vinogradova, O. L., and Golovachev, A. I (1998). Effect of 3-day Dry Immersion on the blood flow in the tibia during a test. Aviakosm Ekolog Med. 2, 24–27.
Struhal, W., Berger, M., Gerstenbrand, F., Golachewski, S., and Lechner-Steinleitner, S. (2002). “Changes of reflex amplitude in dry water immersion,” in Proceedings of the 5th International Head-Out Water Immersion Symposium: Research Simulations to Model Microgravity, Graz.
Suvorov, A. V., Pamova, A. P., and Fedorovich, A. A. (2017). Specifics of microcirculation in the conditions of “Dry” Immersion. Aviakosm Ekolog Med. 51, 32–37. doi: 10.21687/0233-528X-2017-51-6-32-37
Thornton, W. (1987). “Work, exercise and space flight. 1. Operations, environment and effects of spaceflight,” in Proceedings of the JSC Exercise Conferencce, Houston, TX, 1–8.
Titarenko, N. Y., Levchenkova, V. D., Semenova, K. A., Batysheva, T. T., and Dotsenko, V. I (2015). Current non-surgical approaches to correct motion disorders in cerebral palsy children: the literature review. Child. Adolesc. Rehabil. 2, 71–79.
Tomilovskaya, E. S. (2013a). Experiment with five-day dry immersion: objectives, content, structure of the investigations, and specific methods. Hum. Physiol. 39, 756–761. doi: 10.1134/S0362119713070177
Tomilovskaya, E. S. (2013b). “Experiments under Dry Immersion conditions,” in Book: Space Med & Biol, eds A. I. Grigoriev and I. B. Ushakov (Boca Raton, FL: CRC Press), 544–561.
Tomilovskaya, E. S., Amirova, L. E., Custaud, M.-A., and Kozlovskaya, I. B. (2018). “Neuromuscular and cardiovascular alterations in orthostatic hypotension and postural instability induced by microgravity,” in Proceedings of the 42nd Assembly Cospar 2018, Pasadena, CA.
Tomilovskaya, E. S., Kirenskaya, A. V., and Lazarev, I. E. (2008). Influence of lack of safety on the characteristics of presagade EEG potentials in subjects with different asymmetry profiles. Aviakosm Ekolog Med. 5, 14–18.
Treffel, L., Dmitrieva, L., Gauquelin-Koch, G., Custaud, M.-A., Blanc, S., Gharib, C., et al. (2016a). Craniomandibular system and postural balance after 3-day dry immersion. PLoS One 11:e0150052. doi: 10.1371/journal.pone.0150052
Treffel, L., Massabuau, N., Zuj, K., Custaud, M.-A., Gauquelin-Koch, G., Blanc, S., et al. (2017). Pain and vertebral dysfunction in dry immersion: a model of microgravity simulation different from bed rest studies. Pain Res. Manag. 2017:9602131. doi: 10.1155/2017/9602131
Treffel, L., Mkhitaryan, K., Gellee, S., Gauquelin-Koch, G., Gharib, C., Blanc, S., et al. (2016b). Intervertebral disc swelling demonstrated by 3D and water content magnetic resonance analyses after a 3-day dry immersion simulating microgravity. Front. Physiol. 7:605. doi: 10.3389/fphys.2016.00605
Trifonova, O. P., Pastushkova, L. H., Samenkova, N. F., and Pyatnitskii, M. A. (2010). Change in the protein composition of blood plasma in an experiment with a 7-day Dry Immersion. Aviakosm Ekolog Med. 5, 24–28.
Vilchinskaya, N. A., Mirzoev, T. M., Lomonosova, Y. N., Kozlovskaya, I. B., and Shenkman, B. S. (2015). Human muscle signaling responses to 3-day head-out dry immersion. J. Musculoskelet. Neuronal Interact. 15,286–293.
Vilchinskaya, N. A., Mirzoev, T. M., Lomonosova, Y. N., Kozlovskaya, I. B., and Shenkman, B. S. (2016). Effect of short-term Dry Immersion on protelytic signaling in human soleus muscle. Aviakosm Ekolog Med. 50, 28–34.
Vil-Villiams, I. F. (1994). Principle approaches to selection of the short-arm centrifuge regimens for extended space flight. Acta Astronaut. 33, 221–229. doi: 10.1016/0094-5765(94)90129-5
Vil-Villiams, I. F., Kotovskaya, A. R., Ju, V., et al. (1996). Study of the effectiveness of the “Centaur” anti-G suit during exposure to +Gz accelerations after immersion. J. Gravit. Physiol. 3, 24–25.
Vil-Villiams, I. F., and Shulzhenko, E. B. (1978). Heart arrhythmia when subjected to overloads + Gz after immersion. Space Biol. Aerospace Med. 5, 50–56.
Vil-Villiams, I. F., and Shulzhenko, E. B. (1980). Functional State of the cardiovascular system in conditions of combined effects of 28-day immersion spins on the centrifuge short radius and physical load on the bicycle ergometer. Space Biol. Aerospace Med. 2, 42–45.
Vinogradova, O. L., Popov, D. V., Saenko, I. V., and Kozlovskaya, I. B. (2002a). Effect of foot support zones stimulation on muscle transverse stiffness and venous compliance under conditions of dry immersion. J. Gravit. Physiol. 9, 327–330.
Vinogradova, O. L., Stoida, Y. M., Mano, T., and Iwase, S. (2002b). Influence of gravity load on the blood flow to working muscles. Aviakosm Ekolog Med. 36, 39–46.
Vorontsov, A. L., Morukov, B. V., Markin, A. A., Zhuravleva, O. A., Zabolotskaya, I. V., Vostrikova, L. V., et al. (2014). Protein composition of human urine during five-day immersion. Hum. Physiol. 40, 723–726. doi: 10.1134/S0362119714070263
Vorontsov, A. L., Nesterovskaya, A. Y., Morukov, B. V., Markin, A. A., Zhuravleva, O. A., Zabolotskaya, I. V., et al. (2011). Dynamics of the protein content of human urine during 5-day immersion. Aviakosmich Ekolog Med. 45, 18–21.
Watenpaugh, D. E. (2016). Analogs of microgravity: head-down tilt and water immersion. J. Appl. Physiol. 120, 904–914. doi: 10.1152/japplphysiol.00986.2015
Williams, D., Kuipers, A., Mukai, C., and Thirsk, R. (2009). Acclimation during space flight: effects on human physiology. CMAJ 180, 1317–1323. doi: 10.1503/cmaj.090628
Yarullin, H. H., Simonov, L. G., and Vtorov, S. A. (1987). The second change regional and central hemodynamics during 7-day water immersion. Space Biol. Aerospace Med. 4, 45–50.
Yatsuk, G. V. (2002). Algorithms of Diagnostics, Treatment and Rehabilitation of Perinatal Pathologies In Preterm Children. Moscow: Pedagogy-press.
Yunusov, M. A., Orlov, V. N., and Vinohodova, T. V. (1985). Effect Dry Immersion model on the performance of water-salt Exchange, aldosterone and cortisol level in plasma in patients with varying degrees of hydration of the body. Space Biol. Aerospace Med. 4, 42–45.
Zakirova, A. Z., Shigueva, T. A., Tomilovskaya, E. S., and Kozlovskaya, I. B. (2015). Effects of mechanical stimulation of the sole support zones on the H-reflex characteristics under conditions of support unloading. Hum. Physiol. 41, 150–155. doi: 10.1134/S0362119715020176
Keywords: dry immersion, motor control, gravity unloading, support withdrawal, supportlessness
Citation: Tomilovskaya E, Shigueva T, Sayenko D, Rukavishnikov I and Kozlovskaya I (2019) Dry Immersion as a Ground-Based Model of Microgravity Physiological Effects. Front. Physiol. 10:284. doi: 10.3389/fphys.2019.00284
Received: 21 April 2018; Accepted: 04 March 2019;
Published: 27 March 2019.
Edited by:
Nandu Goswami, Medical University of Graz, AustriaReviewed by:
Hanns-Christian Gunga, Charité – Universitätsmedizin Berlin, GermanyDavid Andrew Green, European Astronaut Centre (EAC), Germany
Copyright © 2019 Tomilovskaya, Shigueva, Sayenko, Rukavishnikov and Kozlovskaya. This is an open-access article distributed under the terms of the Creative Commons Attribution License (CC BY). The use, distribution or reproduction in other forums is permitted, provided the original author(s) and the copyright owner(s) are credited and that the original publication in this journal is cited, in accordance with accepted academic practice. No use, distribution or reproduction is permitted which does not comply with these terms.
*Correspondence: Elena Tomilovskaya, ZmluZWdvbGRAeWFuZGV4LnJ1