- 1Division of Nutritional Sciences, Cornell University, Ithaca, NY, United States
- 2Department of Kinesiology, Southwestern University, Georgetown, TX, United States
- 3Department of Biomedical Sciences, Cornell University, Ithaca, NY, United States
Peptide YY (PYY) is considered a gut peptide with roles in post-prandial appetite and glucose regulation. Circulating PYY protein levels increase during aerobic exercise. Furthermore, people who have greater increases in muscle progenitor cells (hMPCs), the adult stem cell population responsible for skeletal muscle (SkM) repair, after resistance training have higher PYY transcript levels in SkM prior to training. Currently, examination of PYY expression patterns in SkM and/or hMPCs is lacking. Our objective was to identify the expression patterns of PYY in SkM and hMPCs. PYY and the associated Y receptors were analyzed in SkM biopsy tissue and cultured hMPCs from young and old human participants. Additional experiments to assess the role and regulation of PYY in hMPCs were performed. In SkM, PYY and one of the three Y receptors (Y1r) were detectable, but expression patterns were not affected by age. In expanding hMPCs, PYY and all three Y receptor (Y1r, Y2r, and Y5r) proteins were expressed in a temporal fashion with young hMPCs having greater levels of Y receptors at various time points. Exogenous PYY did not affect hMPC population expansion. hMPC PYY levels increased following the metabolic stimulus, 5-Aminoimidazole-4-carboxamide ribonucleotide (AICAR), but were not affected by the inflammatory stimulus, tumor necrosis factor alpha (TNFα). In conclusion, PYY and Y receptor expression are not impacted by age in SkM tissue but are reduced in old vs. young expanding hMPCs. Furthermore, endogenous PYY production is stimulated by low energy states and thus may be integral for skeletal muscle and hMPC responses to metabolic stimuli.
Introduction
Peptide YY (PYY), a gut peptide released after food intake (De Silva et al., 2011), increases during aerobic exercise (Broom et al., 2008). The relationship between exercise and plasma PYY depends on exercise type and form of PYY measured (i.e., total PYY or PYY3-36) (Hazell et al., 2016). Post-exercise increases in PYY are posited to be from pancreatic or intestinal secretion via an undescribed mechanism (Hazell et al., 2016). There is evidence, however, skeletal muscle (SkM) may be a source of PYY. Basal PYY gene expression in SkM is greater in extreme hypertrophic responders to resistance exercise training compared to non-responders who displayed no/minimal hypertrophy (Thalacker-Mercer et al., 2013). The extreme responders have more muscle progenitor cells (MPCs), the adult stem cell population responsible for SkM repair (Petrella et al., 2008). In humans, PYY acts through the Y family of Gαi-protein coupled receptors (Y1r, Y2r, and Y5r). PYY exists in two forms, PYY1-36 has affinity for all three receptors while PYY3-36, the cleaved form of PYY1-36, has increased affinity for Y2r (Berglund et al., 2003). Expression of the Y receptors is documented in rodent myoblasts (Zhang et al., 2010) but, to our knowledge, their expression in human MPCs (hMPCs) and SkM has not been identified.
In this report, we provide the first thorough examination of PYY and Y receptor expression in human SkM and hMPCs. Additionally, we elucidate temporal and age-related differences in the expression of PYY and the Y receptors throughout hMPC population expansion. Finally, we identify that PYY levels in hMPCs are altered by compounds that affect hMPC metabolism and consequently population expansion such as the activator, 5-Aminoimidazole-4-carboxamide ribonucleotide (AICAR).
Materials and Methods
Participants
Young (21–40 years) and old adults (65–80 years) were recruited from the Ithaca, New York and Boone, North Carolina areas (participant characteristics in Supplementary Table S1) in a manner previously published (Riddle et al., 2018a,b). The Cornell University and Appalachian State University Institutional Review Boards approved the protocols. All subjects gave written informed consent in accordance with the Declaration of Helsinki.
Skeletal Muscle Biopsies and Primary hMPC Culture
Skeletal muscle tissue and primary hMPCs were obtained using previously published methods (Riddle et al., 2018b). Briefly, SkM tissue was taken from the vastus lateralis using the percutaneous biopsy technique. Tissue was snap-frozen in liquid nitrogen and stored at -80°C (SkM) or stored in Hibernate®-A medium (Invitrogen) at 4°C until tissue disassociation. hMPCs were isolated as previously described (Xu et al., 2015; Riddle et al., 2018b) and were verified to be positive for the MPC specific transcription factor MyoD (Supplementary Figure S1). Passage six hMPCs were cultured in a 5% CO2 atmosphere at 37°C on collagen (Type I, Rat Tail, Corning) coated plates. Only female hMPCs were used due to sample availability.
SkM RNA and Protein Extraction
RNA was extracted from SkM tissue using Trizol Reagent (Ambion). SkM tissue was homogenized in RIPA buffer containing protease and phosphatase inhibitors (cOmplete and PhosStop, Roche) to isolate protein.
Radioimmunoassay
Total human PYY in SkM protein lysate was measured using a commercially available radioimmunoassay (EMD Millipore, PYYT-66HK). PYY values were normalized to the wet weight (mg) of tissue.
Quantitative RT-PCR
Gene expression for PYY and the Y receptors was measured using quantitative RT-PCR. The Taqman Gene Expression System (Applied Biosystems) was used to measure mRNA expression levels of: PYY (HS00358823), Y1r (Hs00268565), Y2r (Hs01921296), Y5r (Hs01883189), and IL-6 (Hs00985639). All samples were normalized to 18S (Hs99999901) expression. Data were expressed as delta Cp values.
Immunoblotting
Y receptor protein levels in SkM were determined by immunoblotting. Protein lysates were subjected to deglycosylation under denaturing reaction conditions according to manufacturer’s instructions (P0704, New England Biolabs). Following deglycosylation, lysates were heated at 60°C and cooled to room temperature. 20 μg of protein was loaded in 10% SDS gels and transferred to PVDF membranes using Stain-Free technology (Bio-Rad). Membranes were incubated in primary antibodies Y1r, Y2r, and Y5r (NBP1-59008, NB100-56480, NB100-1538, respectively, Novus Biologicals) diluted (1:1000) in a chemiluminescent blocking buffer (blØkTM – CH, Millipore) overnight at 4°C. After the overnight incubation, membranes were washed with TBST before incubation with appropriate secondary antibody (anti-rabbit, Proteintech or anti-goat, Thermo Scientific) at a 1:25000 dilution in blocking buffer at room temperature. Membranes were visualized after incubation in SuperSignalTM West Femto (Thermo Scientific) on the Bio-Rad ChemiDoc MP.
hMPC Treatments
For hMPC population expansion experiments, recombinant human PYY1-36, PYY3-36 (Phoenix Pharmaceuticals), or vehicle control (sterile H2O) was administered every 24. For TNFα (Millipore Sigma) and AICAR (Cell Signaling Technology) experiments, hMPCs were treated with the compound or vehicle control (sterile H2O or DMSO) for the specified time, then fixed with 4% formaldehyde for In-Cell Western analysis.
The number of live cells was measured every 24 h by co-staining cells with Hoechst 33342 (to identify number of nuclei) and Propidium Iodide (to identify dead cells).
In-Cell Western Assay
Fixed hMPCs were permeabilized with 0.1% Triton in PBS. Cells were incubated in primary antibodies, specific for PYY (ab22663, Abcam, Supplementary Figure S2) and Y1r, Y2r, and Y5r diluted (PYY, 1:1000; Y receptors, 1:100) in Odyssey Blocking Buffer (LI-COR), overnight at 4°C. Cells were washed with 0.1% PBST and incubated at room temperature in secondary antibody (IRDye® 800CW anti-rabbit, LI-COR or IRDye® 800CW anti-goat, LI-COR) diluted 1:400 in Odyssey Blocking Buffer. Fluorescence intensity, representing protein levels, was measured using the Odyssey Imaging System (LI-COR). Protein levels were normalized to number of cells via Hoechst 33342 staining and counting using the Celigo® Imaging Cytometer (Nexcelom Bioscience).
Immunocytochemistry
Fixed hMPCs were permeabilized with 0.1% Triton in PBS and incubated in primary embryonic myosin heavy chain (eMHC) antibody (DSHB Hybridoma Product F1.652, F1.652 was deposited to the DSHB by Blau, Helen M.) (Webster et al., 1988) and either primary Y2r or Y5r antibodies diluted (1:100) in eMHC supernatant, overnight at 4°C. Cells were washed with PBS and incubated in secondary antibody (AlexFluor® 568 anti-mouse and AlexFluor® 488 anti-rabbit, Life Technologies) at 37°C, diluted 1:1000 in 1% goat serum. Nuclei were counterstained with Hoechst 33342.
Statistics
All statistical analyses were performed in GraphPad 6.0. Protein and gene expression between young and old, were compared using an unpaired t-test. A two-way analysis of variance (ANOVA), with main factors of age and time, was used to discern differences in protein levels in hMPCs. To compare live cell count after treatment a two-way, repeated measures ANOVA with treatment and time being the main factors was conducted. To evaluate the effect of TNFα and AICAR on hMPC PYY levels, either a two-way ANOVA (treatment and age) or a one-way repeated measures ANOVA (treatment) was performed. When the interaction term was significant, a Tukey HSD post hoc test was performed.
Results
PYY and Y Receptor Expression in Human SkM and hMPCs
Peptide YY protein and Y1r mRNA and protein, but not Y2r and Y5r (data not shown), were detectable in SkM, but were not different between young and old individuals (Figures 1A–C). To validate that Y2r and Y5r are not expressed in SkM, we differentiated hMPCs for 7 days, until eMHC was expressed, and used immunocytochemistry to visualize Y2r and Y5r expression (Figure 1D). Y2r could not be visualized in myotubes, however, Y5r was detectable and colocalized with eMHC.
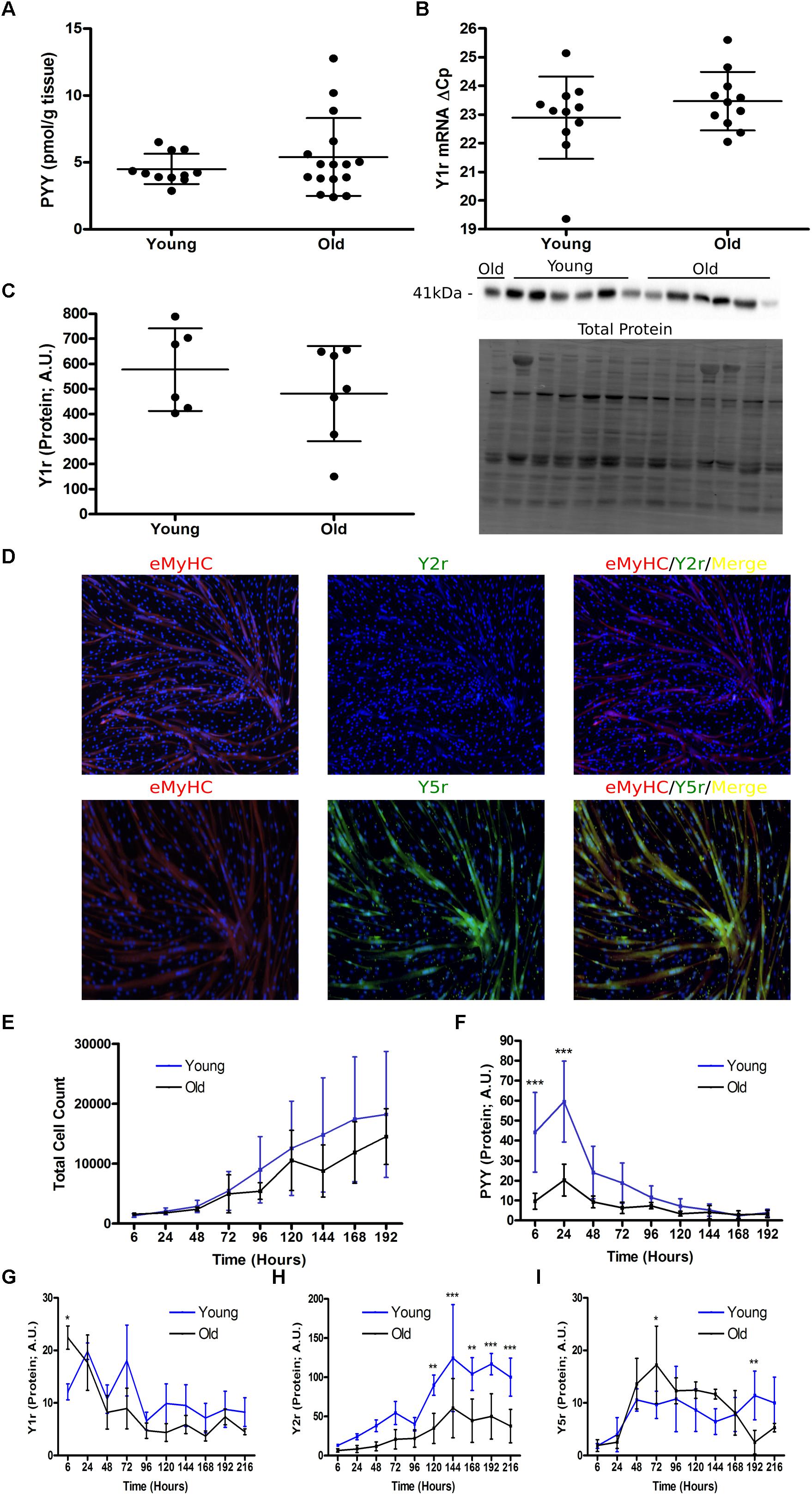
Figure 1. Characterization of PYY and the Y receptors in human skeletal muscle and expanding human muscle progenitor cells. Expression of (A) PYY protein expression in SkM of young (n = 11) and old (n = 16) individuals. SkM Y1r expression at the (B) gene (n = 27) and (C) protein level (n = 11). (D) Representative microscopy images of hMPCs co-stained with a DNA stain (Hoechst 33342, blue), Y2r or Y5r (green), and embryonic myosin (red). (E) Expansion curves of cultured hMPCs isolated from young (n = 5) and old (n = 5) donors. Protein expression of (F) PYY, (G) Y1r, (H) Y2r, and (I) Y5r in expanding young (n = 5) and old (n = 5) hMPC cultures. A–C: unpaired t-test. E–I: Two-way ANOVA, main effect of age, time, age × time interaction (stars denote results of Tukey’s HSD post hoc test performed when interaction term was significant) ∗p < 0.05, ∗∗p < 0.01, and ∗∗∗p < 0.001. Data are expressed as mean ± SEM. SkM, skeletal muscle; PYY, Peptide YY; Yr, Y receptor; hMPC, human muscle progenitor cell.
In young (n = 5) and old (n = 5) hMPCs, PYY was detectable and declined throughout 192 h of population expansion (p < 0.05, Figure 1F). PYY protein levels were greater in hMPCs from young (vs. old) at 6 h and 24 h after seeding (p < 0.05, Figure 1F). Y1r protein levels declined throughout population expansion (p < 0.05, Figure 1G). Young hMPCs had greater levels of Y1r protein than old hMPCs at 6 h after seeding (p < 0.05, Figure 1G). Y2r protein levels increased with population expansion (Figure 1H). Young (vs. old) hMPCs had higher levels of Y2r from 120 to 216 h after seeding (p < 0.01, Figure 1H). Compared to old hMPCs, young hMPCs expressed greater levels of Y5r protein at 72 h (p < 0.05) and 192 h after seeding (p < 0.01, Figure 1I). Collectively these data demonstrate that SkM tissue PYY levels do not differ between young and old; however, PYY and the Y receptors are higher in young compared with old hMPCs. This age difference between SkM and cultured hMPCs may be related to differences only observed during dynamic conditions (i.e., population expansion).
Role and Regulation of PYY During hMPC Population Expansion
Noting the transient expression of PYY and the Y receptors during hMPC population expansion, we hypothesized that exogenous PYY promotes hMPC population expansion. Basic fibroblast growth factor (bFGF) and the PYY-related peptide, neuropeptide Y, have been shown to have similar, but not necessarily additive, effects on human embryonic cell proliferation (Son et al., 2011); therefore, for some of these experiments, bFGF was removed. To determine the effect of PYY on hMPC population expansion, hMPCs from young donors (n = 5) were cultured with or without bFGF and supplemented with varying doses of PYY1-36 or PYY3-36. hMPCs grown in the presence of PYY1-36 (Figure 2A) or PYY3-36 (Figure 2B) and without bFGF showed no increase in population expansion compared to a no bFGF control. Therefore, exogenous PYY does not appear to regulate hMPC population expansion.
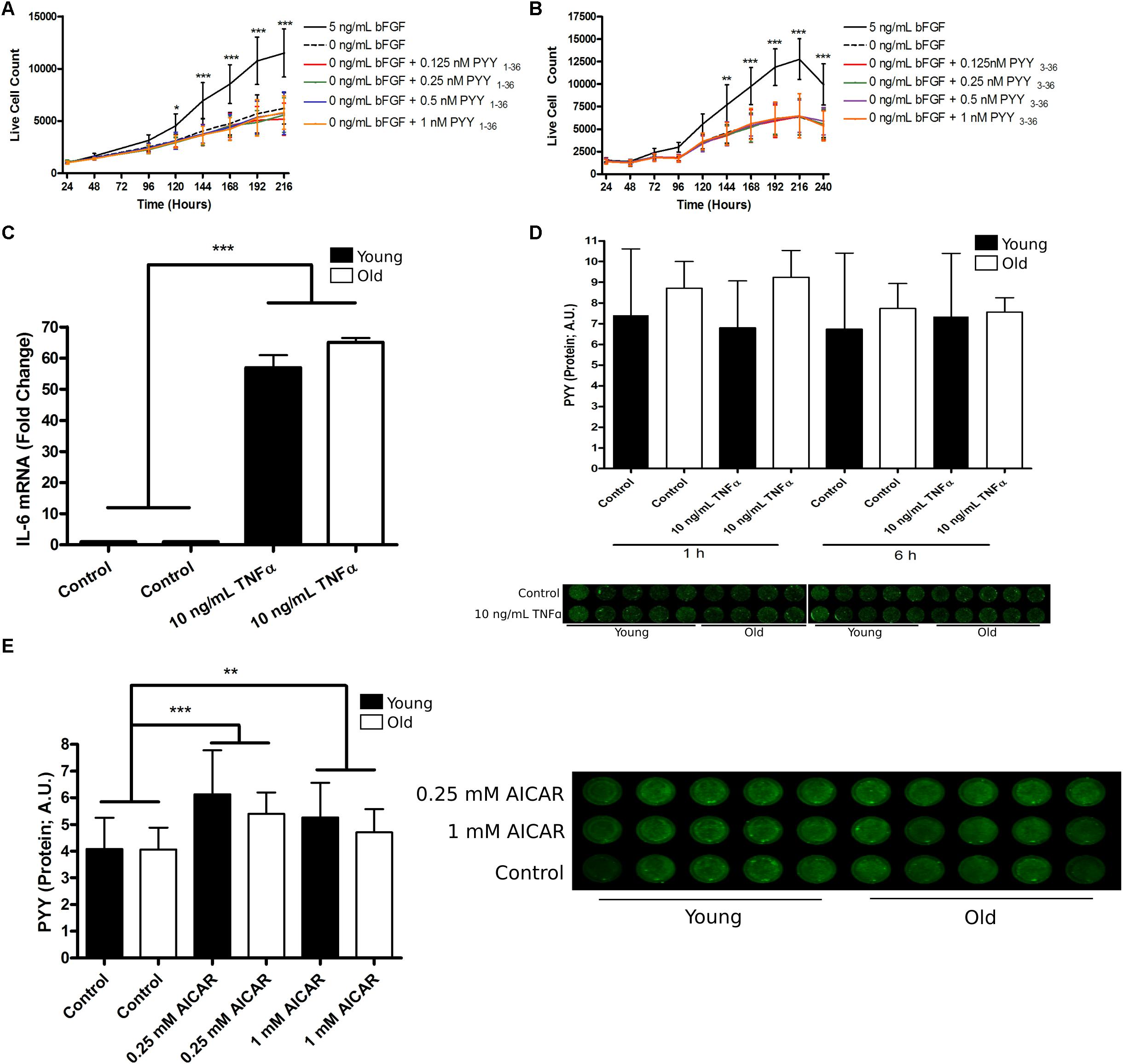
Figure 2. The role and regulation of PYY in human muscle progenitor cells. (A) PYY1-36 or (B) PYY3-36 do not affect hMPC expansion. (C) The effect of 6 h of 10 ng/mL TNFα treatment on IL-6 mRNA levels in young (n = 5, black bars) and old (n = 5, open bars) hMPCs. (D) PYY protein levels in young (n = 5) and old (n = 5) hMPCs after 1 and 6 h of 10 ng/mL TNFα exposure. (E) PYY protein levels in young (n = 5) and old (n = 5) hMPCs after 6 h of 0.25 or 1 mM AICAR treatment. A,B: Two-way ANOVA, main-effect of treatment, time, and treatment-by-time interaction and C–E: Two-way ANOVA, main-effect of treatment, age, and treatment-by-age interaction (asterisks denote results of Tukey’s HSD post hoc test performed when interaction term was significant). ∗p < 0.05, ∗∗p < 0.01, and ∗∗∗p < 0.001. Data are expressed as mean ± SEM. PYY, Peptide YY; Yr, Y receptor; hMPC, human muscle progenitor cell; TNFα, tumor necrosis factor alpha; AICAR, 5-Aminoimidazole-4-carboxamide ribonucleotide.
Alternatively, we hypothesized that because PYY and Y receptor levels were highest during most rapid population expansion (Figures 1E–I), endogenous PYY levels may be regulated by factors that affect this process. First, we considered TNFα, an inflammatory cytokine that promotes hMPC population expansion (Miller et al., 1988). After hMPCs from young (n = 4) and old donors (n = 5) entered exponential growth (96 h after seeding) they were treated with 10 ng/mL of TNFα. This treatment increased IL-6 mRNA levels by ∼50-fold (Figure 2C) within 6 h. TNFα exposure for 1 h (p = 0.88) or 6 h (p = 0.66) had no effect on hMPC PYY levels regardless of age (Figure 2D).
Next we treated hMPCs with AICAR, an activator of AMPKα; AMPKα activity has been implicated in the regulation of MPC population expansion (Pavlidou et al., 2017). hMPCs were treated with either 0.25 or 1 mM AICAR for 6 h after cells entered exponential growth (96 h after seeding). 6 h of 0.25 mM AICAR increased hMPC PYY levels by 42% (p = 0.0001) and 1 mM produced a 30% increase (p = 0.02, Figure 2E). We observed no age-related differences.
Discussion
This report presents the first examination of PYY and Y receptor expression in human skeletal muscle, using two systems: SkM and hMPCs. Under resting conditions PYY and Y1r, but not Y2r or Y5r, are expressed in SkM. Our data suggest that hMPC PYY expression is regulated by metabolic state. Circulating PYY concentrations increase after exercise to a modest degree (Hazell et al., 2016), but the source of this PYY is unknown. Our results support that SkM-derived PYY could be one contributor. The lack of difference between PYY and Y receptor levels in young and old SkM suggests no age-related difference in the activity of this peptide during steady state conditions, however, we detected age-related differences in expanding hMPCs. Therefore, it is likely age-related differences exist during dynamic conditions such as during regeneration. Y1r, Y2r, and Y5r were expressed during hMPC expansion, only Y1r and Y5r were detectable in either SkM tissue or differentiated hMPCs, suggesting PYY signaling depends on state of differentiation.
hMPC PYY levels were shown to be unaffected by a pro-expansion stimulus (TNFα) but were increased by an anti-expansion stimulus (AICAR). The increase in hMPC PYY levels could be due to intracellular PYY synthesis and storage. Alternatively, increased hMPC PYY levels could be due to increased binding of PYY from the media to the Y receptors on the cell surface. This explanation is unlikely as bovine PYY differs from human PYY (71% homology) and differences were seen between young and old hMPCs under similar culture conditions. Alternatively, as PYY is traditionally considered a secretory peptide (Psichas et al., 2015) it could be posited that AICAR treatment resulted in the synthesis, secretion, and binding of PYY in an autocrine manner.
Conclusion
In conclusion, we present the most comprehensive evidence to date that PYY is produced by post-mitotic SkM tissue and expanding hMPCs. Furthermore, we identify AICAR treatment, which negatively affects hMPC population expansion, as an inducer of PYY expression in hMPCs. We propose that hMPC endogenous PYY and Y receptor activity may be integral for skeletal muscle and hMPC responses to metabolic stimuli.
Data Availability
All datasets generated for this study are included in the manuscript and/or the Supplementary Files.
Author Contributions
BG and AT-M designed the experiments. BG, JB, EM, BC, and AT-M collected, analyzed, and interpreted the data.
Funding
This study was funded by President’s Council of Cornell Women to AT-M, Cornell Division of Nutritional Sciences funds to AT-M, and a grant from the US Highbush Blueberry Council to EM. BG was funded by a Canadian Institutes of Health Research Doctoral Foreign Study Award.
Conflict of Interest Statement
The authors declare that the research was conducted in the absence of any commercial or financial relationships that could be construed as a potential conflict of interest.
Acknowledgments
We thank Heather Roman, M. S. for her assistance with maintaining the hMPC cultures. We also thank Melinda Lem for her help with participant recruitment. Finally, we also thank the participants for their time and participation in the study.
Supplementary Material
The Supplementary Material for this article can be found online at: https://www.frontiersin.org/articles/10.3389/fphys.2019.00188/full#supplementary-material
FIGURE S1 | Passage 6 hMPCs are MyoD positive. Representative microscopy images of passage 6 hMPCs co-stained with a DNA stain (Hoechst 33342, blue) and the MPC-specific transcription factor MyoD (red).
FIGURE S2 | Validation of the PYY antibody for the In-Cell Western assay. In-Cell Western images demonstrating the specificity of the PYY antibody used for all experiments to recombinant PYY and not the closely related NPY.
TABLE S1 | Baseline participant characteristics.
References
Berglund, M. M., Hipskind, P. A., and Gehlert, D. R. (2003). Recent developments in our understanding of the physiological role of PP-fold peptide receptor subtypes. Exp. Biol. Med. 228, 217–244. doi: 10.1177/153537020322800301
Broom, D. R., Batterham, R. L., King, J. A., and Stensel, D. J. (2008). Influence of resistance and aerobic exercise on hunger, circulating levels of acylated ghrelin, and peptide YY in healthy males. AJP Regul. Integr. Comp. Physiol. 296, R29–R35. doi: 10.1152/ajpregu.90706.2008
De Silva, A., Salem, V., Long, C. J., Makwana, A., Newbould, R. D., Rabiner, E. A., et al. (2011). The gut hormones PYY 3-36 and GLP-1 7-36 amide reduce food intake and modulate brain activity in appetite centers in humans. Cell Metab. 14, 700–706. doi: 10.1016/j.cmet.2011.09.010
Hazell, T. J., Islam, H., Townsend, L. K., Schmale, M. S., and Copeland, J. L. (2016). Effects of exercise intensity on plasma concentrations of appetite-regulating hormones: potential mechanisms. Appetite 98, 80–88. doi: 10.1016/j.appet.2015.12.016
Miller, S. C., Ito, H., Blau, H. M., and Torti, F. M. (1988). Tumor necrosis factor inhibits human myogenesis in vitro. Mol. Cell. Biol. 8, 2295–2301. doi: 10.1128/MCB.8.6.2295
Pavlidou, T., Rosina, M., Fuoco, C., Gerini, G., Gargioli, C., Castagnoli, L., et al. (2017). Regulation of myoblast differentiation by metabolic perturbations induced by metformin. PLoS One 12:e0182475. doi: 10.1371/journal.pone.0182475
Petrella, J. K., Kim, J.-S., Mayhew, D. L., Cross, J. M., and Bamman, M. M. (2008). Potent myofiber hypertrophy during resistance training in humans is associated with satellite cell-mediated myonuclear addition: a cluster analysis. J. Appl. Physiol. 104, 1736–1742. doi: 10.1152/japplphysiol.01215.2007
Psichas, A., Sleeth, M. L., Murphy, K. G., Brooks, L., Bewick, G. A., Hanyaloglu, A. C., et al. (2015). The short chain fatty acid propionate stimulates GLP-1 and PYY secretion via free fatty acid receptor 2 in rodents. Int. J. Obes. 39, 424–429. doi: 10.1038/ijo.2014.153
Riddle, E. S., Bender, E. L., and Thalacker-Mercer, A. E. (2018a). Expansion capacity of human muscle progenitor cells differs by age, sex, and metabolic fuel preference. Am. J. Physiol. Cell Physiol. 315, C643–C652. doi: 10.1152/ajpcell.00135.2018
Riddle, E. S., Bender, E. L., and Thalacker-Mercer, A. E. (2018b). Transcript profile distinguishes variability in human myogenic progenitor cell expansion capacity. Physiol. Genomics 50, 817–827. doi: 10.1152/physiolgenomics.00041.2018
Son, M. Y., Kim, M. J., Yu, K., Koo, D. B., and Cho, Y. S. (2011). Involvement of neuropeptide Y and its Y1 and Y5 receptors in maintaining self-renewal and proliferation of human embryonic stem cells. J. Cell. Mol. Med. 15, 152–165. doi: 10.1111/j.1582-4934.2009.00956.x
Thalacker-Mercer, A., Stec, M., Cui, X., Cross, J., Windham, S., and Bamman, M. (2013). Cluster analysis reveals differential transcript profiles associated with resistance training-induced human skeletal muscle hypertrophy. Physiol. Genomics 45, 499–507. doi: 10.1152/physiolgenomics.00167.2012
Webster, C., Silberstein, L., Hays, A. P., and Blau, H. M. (1988). Fast muscle fibers are preferentially affected in Duchenne muscular dystrophy. Cell 52, 503–513. doi: 10.1016/0092-8674(88)90463-1
Xu, X., Wilschut, K. J., Kouklis, G., Tian, H., Hesse, R., Garland, C., et al. (2015). Human satellite cell transplantation and regeneration from diverse skeletal muscles. Stem Cell Rep. 5, 419–434. doi: 10.1016/j.stemcr.2015.07.016
Keywords: satellite cells, aging, stem cell, inflammation, metabolism, Peptide YY, muscle progenitor cells, skeletal muscle
Citation: Gheller BJ, Blum JE, Merritt EK, Cummings BP and Thalacker-Mercer AE (2019) Peptide YY (PYY) Is Expressed in Human Skeletal Muscle Tissue and Expanding Human Muscle Progenitor Cells. Front. Physiol. 10:188. doi: 10.3389/fphys.2019.00188
Received: 17 November 2018; Accepted: 14 February 2019;
Published: 05 March 2019.
Edited by:
Stefano Biressi, University of Trento, ItalyReviewed by:
David C. Hughes, The University of Iowa, United StatesMin Du, Washington State University, United States
Copyright © 2019 Gheller, Blum, Merritt, Cummings and Thalacker-Mercer. This is an open-access article distributed under the terms of the Creative Commons Attribution License (CC BY). The use, distribution or reproduction in other forums is permitted, provided the original author(s) and the copyright owner(s) are credited and that the original publication in this journal is cited, in accordance with accepted academic practice. No use, distribution or reproduction is permitted which does not comply with these terms.
*Correspondence: Anna E. Thalacker-Mercer, QWV0NzRAY29ybmVsbC5lZHU=