- 1MARA-CABI Joint Laboratory for Bio-safety, Institute of Plant Protection, Chinese Academy of Agricultural Sciences, Beijing, China
- 2College of Plant Protection, Yunnan Agricultural University, Kunming, China
- 3State Key Laboratory of Integrated Management of Pest Insects and Rodents, Institute of Zoology, Chinese Academy of Sciences, Beijing, China
- 4Centre for Agriculture and Biosciences International (CABI) East Asia, Beijing, China
- 5Life Science College, Anhui Normal University, Wuhu, China
Halyomorpha halys is a major herbivore insect in the fruit orchards of China that has become a devastating invasive pest in North America and Europe since its accidental introductions in the 1990s and 2000s, respectively. Like other hemipteran insects, H. halys releases defensive chemicals against natural enemies, including (E)-2-decenal, which is an aldehyde associated with alarm pheromones. In this study, a series of electrophysiological and behavioral tests were conducted to characterize the alarm functions of (E)-2-decenal among H. halys adults and nymphs. An antennal transcriptome was obtained from a Chinese H. halys population, and 44 odorant-binding protein (OBP) genes were annotated. Among them, five putative alarm pheromone-binding proteins were screened and were extremely consistent with their homologs from US populations. These five OBPs were later expressed in a heterologous expression system, harvested, and then challenged with (E)-2-decenal in a binding assay. All five OBPs showed high binding activities to (E)-2-decenal, which demonstrated its behavioral significance as a defensive component in H. halys, as well as being the first report of its olfactory reception. These findings will help develop behavioral-mediating tools as part of integrated pest management approaches to control this invasive pest.
Introduction
Stink bugs (Hemiptera: Pentatomidae) employ a spectrum of volatile compounds that have ecologically significant roles in colonization, courtship, immunity, and chemical defense (Noge et al., 2015; Weber et al., 2017b). Among these compounds, alarm pheromones, which mostly consist of short-chain aliphatic aldehydes, alcohols and esters, alkanes, terpenes, and phenolics, are well-known signature odorant cues in stink bugs (Aldrich, 1988; Millar, 2005; Moraes et al., 2008; Noge and Becerra, 2012). These unpleasant and pungent secretions are stored in the dorsal abdominal glands of nymphs and the metathoracic scent glands of adults and are released under aggressive environmental stimuli to mediate behavioral consequences among individual pentatomids, predators, and natural enemies (Borges and Aldrich, 1992; Weber et al., 2017b). To date, a wide variety of hydrocarbons, aldehydes, and oxo-aldehydes are thought to be defensive compounds involved in the dispersal process (Aldrich, 1988; Tada et al., 2001a,b; Noge and Becerra, 2012; Weber et al., 2017b). Previous studies described the proportions and release biases among species, genders, and developmental stages (Kuhar and Kamminga, 2017; Weber et al., 2017b). A number of key components separated from several stink bug species have multiple functions, including prompting generalist predator avoidance (Noge and Becerra, 2012), delivering antifungal and antibacterial substances (Lopes et al., 2015; Sagun et al., 2016), and accelerating the locating of hosts by parasitoids (Zhong et al., 2017).
Halyomorpha halys, the brown marmorated stink bug, is a well-studied stink bug species in the field of chemical ecology (Weber et al., 2017b). Native to Eastern Asia, H. halys was accidently introduced into North America and Europe in the 1990s and 2000s, respectively (Haye and Weber, 2017; Valentin et al., 2017; Leskey and Nielsen, 2018). It is now been considered a very damaging invasive insect pest globally (Haye and Weber, 2017). As a highly polyphagous pentatomid herbivore with over 120 host plants, H. halys has caused serious annual losses in economically important fruit crops, vegetables, ornamentals, shrubs, and forest trees (Lee et al., 2013). Research has emphasized the identification of its aggregation pheromone, its synergism with the pheromone of Plautia stali, and the development of pheromone-based traps for field applications (Borges and Aldrich, 1992; Tada et al., 2001a; Lee et al., 2013; Kuhar and Kamminga, 2017). Additionally, chemical communications among this pest, its host plants, and its natural enemies have been studied to develop biological control based integrated pest management strategies (Bernays and Chapman, 1994; Schoonhoven et al., 2005; Noge and Becerra, 2012; Zhang et al., 2014b; Tognon et al., 2016; Zhong et al., 2017). The defensive chemicals n-tridecane, n-dodecane, (E)-2-decenal, and (E)-2-octenal have been identified from H. halys volatiles (Kitamura et al., 2008; Noge and Becerra, 2012; Harris et al., 2015; Zhong et al., 2017). In particular, the emission patterns of (E)-2-decenal have been investigated in both H. halys sexes and 3rd instar nymphs (Harris et al., 2015; Zhong et al., 2017), and it is considered as an alarm pheromone component (Harris et al., 2015; Weber et al., 2017a). However, its alarm effects on H. halys, and the molecular basis for the olfactory perception behind its effects, have not been revealed.
H. halys senses these semiochemicals through its olfactory organs on the antennae, and odorant-binding proteins (OBPs) play an important role in the olfactory process (Vosshall and Stensmyr, 2005; Leal, 2013; Wicher, 2015; Paula et al., 2016). OBP responses are the first step at the peripheral olfactory level for delivering odorant cues to the transmembrane odorant receptors in the aqueous lumen of chemosensilla on insect antennae (Vogt and Riddiford, 1981). Since the report of the first hemipteran OBP family in Lygus lineolaris in 1995, OBPs have been isolated and functionally characterized from over 32 hemipteran species (Dickens et al., 1995; Paula et al., 2016). A recent break-through in H. halys reported the annotation of 33 OBPs in its US population, and an olfactory plasticity was observed under alarm pheromone stimulation when males or females were forced to release the alarm pheromone in contained small arena bioassays (Paula et al., 2016). The quantitative assessment of expression changes in OBP genes revealed multiple OBPs might be involved in detection of alarm pheromone stimulus in H. halys. Nevertheless, which genes are responsible for binding alarm pheromone compounds, such as (E)-2-decenal, remains unknown. Revealing the existence of (E)-2-decenal-sensing OBPs may contribute to new solutions and approaches to control H. halys through behavioral interference, such the use of RNA interference technology (Mogilicherla et al., 2018).
It is still critical to understand and demonstrate clear behavioral responses of different developmental stages of H. halys to alarm pheromones and their corresponding molecular olfactory mechanisms. (E)-2-Decenal is an abundant defensive compound released by H. halys adults, and it can strongly repel male and female H. halys in Y-tube assays (Zhong et al., 2017). In the present study, we investigated the alarm effects of (E)-2-decenal and its olfactory preferences in H. halys by conducting electroantennogram (EAG) tests, behavioral assays with grouped or individual H. halys, antennal transcriptome analyses, and binding activity assays with putative OBPs. This work provides strong behavioral evidence and molecular olfactory bases to demonstrate the function of (E)-2-decenal as an alarm pheromone component in H. halys.
Materials and Methods
Insects
Nymphs and adults of H. halys were obtained from laboratory colonies at the MARA-CABI Joint Laboratory, Beijing. The initial laboratory colony was established from wild H. halys populations collected in Beijing, China (N40°01′53″; E116°15′32″). They were continuously reared on a diet of organic green beans (Phaseolus vulgaris L.) and corn (Zea mays L.) in rearing cages (60 × 60 × 60 cm) at 25 ± 1°C, with 65% ± 5% relative humidity and a 16 L:8 D photoperiod (Zhong et al., 2017). Egg masses were collected daily and maintained in separate rearing cages until completion of the nymphal stages. Newly emerged adults were removed and maintained in separate rearing cages as described above. The nymphs and adults of H. halys used for the experiments were reared for 2-3 generations in the laboratory.
Chemicals
n-Hexane (95%, Sigma-Aldrich, St. Louis, MO, USA) was used as the control and solvent, and (E)-2-decenal (95%, Sigma-Aldrich, St. Louis, MO, USA) was used as stimulus chemical.
EAG Recordings
EAG recordings were used to identify electrophysiological activities of H. halys at each developmental stage (female and male adults, and nymphal larvae). Each antenna of the brown marmorated stink bug was prepared following the standard procedures of cutting its tip and base and immediately mounting the excised antenna between two glass capillary Ag/AgCl electrodes filled with Kaissling saline (Tang et al., 2016). The electrode at the distal end of the antenna was connected through an interface box to a signal acquisition interface board (IDAD; Syntech, Netherlands).
n-Hexane was used as the control, and 1 μg/μl (E)-2-decenal was used as the test solution. The measure dose was 10 μg, and the order of antennal exposure was air, n-hexane, and (E)-2-decenal. In total, 10 replicates were used. The direct voltage was 2 mv, the continuous flow velocity was 150 ml/min, the stimulated flow velocity was 20 ml/min, the stimulation time was 0.1 s, and the stimulus intervals were 10 s.
The following equation was used to calculate the EAG recording result:
Relative response value = sample response value − control response value.
Behavioral Assays
A group test was performed first to assess the repellency of (E)-2-decenal to H. halys adults. In total, 10–20 adults were collected and moved into a plastic rearing box for 1 h to let the adults aggregate and rest before the test. A filter paper loaded with 10 μg (E)-2-decenal was then put into a syringe, and the volatile was released into the air at a distance of 1 cm from the aggregated H. halys adults. The test lasted for 60 s, and the number of dispersed H. halys were counted and recorded. n-Hexane was used as the control following the same protocol. In total, 10 replicates were performed for each treatment.
To identify the alarm pheromone activity in each developmental stage of H. halys (female and male adults, and 1st to 5th instar nymphs), we performed a behavioral test (Noge et al., 2015) using a gradient of (E)-2-decenal solutions (10 μg/μl, 1 μg/μl, 100 ng/μl, 10 ng/μl, and 1 ng/μl) for the nymphs and the last four doses for both genders of adults. A circular track was made by joining the ends of a silicone tube (100 cm long, 1.2 cm inner diameter), and the track was placed on a tripod stand in the behavioral testing laboratory (25°C ± 1°C, relative humidity = 50% ± 10%). An individual H. halys was placed gently on the track and left for 5 min until it was motionless. Different 10-μl concentrations of (E)-2-decenal were loaded onto a filter paper disk (2 cm diameter), and the disk was then placed 2 mm from the stink bug's antenna. The time taken for the nymph or adult stink bug to change direction and the distance it moved away from the starting point within 15 s were observed and recorded. If the stink bug did not change direction or stayed still for 15 s, the distance and time were recorded as 0 cm and 15 s, respectively. n-Hexane was used as the control. Every developmental stage of H. halys for each chemical treatment was tested 15 times.
Antennal Transcriptome Analysis and Putative OBP Annotations
Antennae from H. halys were collected, pooled into female and male groups and then used in a standard Illumina HiSeq2000 platform pipeline for de novo RNA sequencing at BGI Tech. Co., Beijing, China. Clean reads were obtained using the FastQC tool and then assembled with SOAP. OBP annotations were performed using BLAST algorithm-based searches against the NR, NT, Swiss-Prot, KEGG, COG, and GO databases. Translated amino acid sequences of OBPs from H. halys, Apolygus lucorum, and Drosophila melanogaster were first aligned with MUSCLE, and then, a phylogenetic tree was developed using the neighbor-joining method (Saitou and Nei, 1987) in MEGA 7.0.14 software (Kumar et al., 2016). Protein structures of OBPs were predicted with the Swiss-model to locate potential plus-C domains. Alignments of OBP25, OBP30, OBP16, OBP8, and OBP4 between the Chinese and the US populations were performed using the PRALINE multiple sequence alignment online tools.
Expression and Purification of OBP Proteins
We chose five H. halys OPBs based on the results of a previous study (Paula et al., 2016), which showed that OBP25, OBP30, OBP16, OPB8, and OBP4 were highly expressed after exposure to an alarm pheromone, and the increases in the ranges were ~100,000-, 10,000-, 1,000-, 100-, and 10-fold. We synthesized the genes and heterologously expressed these five OPBs. The prokaryotic expression was used to identify the target proteins. In detail, the exact gene sequences from transcriptome analysis were synthesized by the Genscript Biology Company (Nanjing, China) and subcloned into target vector for Escherichia coli expression (Table 1).
For each OBP gene, recombinant pET30a vectors were transferred into the E. coli BL21 (DE3) competent cell strain and allowed to duplicate for 60 min. E. coli were then plated on LB agar dishes containing 50 μg/ml kanamycin. Three single cloned colonies were isolated and reared in LB medium until the OD600 was 0.6–0.8. Isopropyl β-D-1-thiogalactopyranoside at the final concentration of 1.0 mM was added to two of the three liquid cultures for induction at 15°C for 16 h and at 37°C for 4 h, respectively, with the third sample acting as the negative control. Pilot expression levels of each OBP were detected with SDS-PAGE and western blotting, respectively.
OBP protein samples for later tests were prepared from isopropyl β-D-1-thiogalactopyranoside-induced liquid cultures. The cells were harvested by centrifugation at 12,000 rpm for 20 min at 4°C. Insoluble inclusion cell bodies were dissolved in 8 M urea in 20 mM Tris-HCl buffer at pH 7.4. Raw solutions were then purified through an affinity chromatography XK 16/20 column filled with Ni Sepharose High Performance (GE Healthcare, Little Chalfont, Buckinghamshire, UK). Laddered urea solutions were used for protein renaturation and extensive dialysis. Harvested proteins were then treated with enterokinase (Genscript Biology Company) to cleave His-tag residues. The cleavage proteins were purified following the same protocol as above and then desalted by extensive dialysis, lyophilized, and stored at −70°C until use.
Competitive Fluorescence-Binding Assay
Emission fluorescence spectra were measured on a HORIBA FluoromMax®-4 fluorescence spectrophotometer in a right-angle configuration with a 1 cm light path quartz cuvette. The protein was dissolved in 50 mM Tris-HCl buffer, pH 7.4, while all ligands used in binding experiments were used as 1 mM methanol solutions. The excitation wavelength was 337 nm, and the range of the scanning emission wavelength was 380–450 nm.
The binding constants of OBPs with probe N-phenyl-1-naphtylamine (1-NPN) were determined. We first added 2 ml 50 mM Tris-HCl solution to the cuvette, then added the OBP until the concentration reached 2 μM, measured and recorded the emission spectrum, continued titration, and recorded the highest stable fluorescence intensity. The Scatchard equation was used to record the binding constant of each OPB with 1-NPN.
The binding abilities of the OBPs and (E)-2-decenal were later investigated. Fluorescence intensities were automatically subtracted from blank fluorescence values when harvested from instrument. The excitation wavelength was set at 337 nm, and the range of the scanning emission wavelength was 380–450 nm. First, 2 mL 50-mM Tris-HCl solution was added, and then the OBP and 1-NPN were added until the concentration was 2 μM. The highest stable fluorescence intensity was record. Then, we added the (E)-2-decenal, which was dissolved in methanol in the cuvette, and continued the titration from 2 μM until the fluorescence intensity was stable. The highest stable fluorescence intensity was recorded. Each OBP was tested three times. The dissociation constant was calculated based on the formula Kd = [IC50]/(1 + [1-NPN]/K1−NPN), where IC50 represents the (E)-2-decenal concentration when the fluorescence intensity of [OBP/1-NPN] declined to the half of the highest value, [1-NPN] represents the dissociative concentration of 1-NPN, and K1−NPN represents the dissociation constant of OBP/1-NPN.
Statistical Analysis
All statistics were done in IBM SPSS Statistics 22.0.0 (SPSS, Chicago, IL, USA). Parametric tests (either t tests for 2 treatments or GLM for > 2 treatments) were used to compare difference among means for EAG and behavioral assays. Tukey HSD was used for multiple comparison among treatments. For competitive fluorescence-binding assays, correlations and curves were done using Prism 5 for Windows ver. 5.01 (GraphPad software, San Diego, CA, USA). All significance was accepted at the α = 0.05 level. Three replicates were done for binding assays, and means were used for the statistics.
Data Viability
Original EAG voltage data sheets were provided as Data S1. Original competitive fluorescence-binding assay data sheets were provided as Data S2.
Results
(E)-2-Decenal Elicited Significant Electrophysiological Responses From H. halys
We first conducted EAG tests to investigate the biological activities of (E)-2-decenal at the antennal level. Among all of the H. halys' developmental stages, we observed significantly greater electrophysiological responses compared with the n-hexane control, except for the 1st instar nymphs (Figure 1). Antennae of adults had greater EAG responses than nymphs. Moreover, (E)-2-decenal stimulated significantly greater EAG responses in male adults compared with all nymphs, but there was no significant difference between the sexes of adults. Female H. halys showed significantly greater responses compared with 1st and 2nd instar nymphs (Figure 1, Data S1).
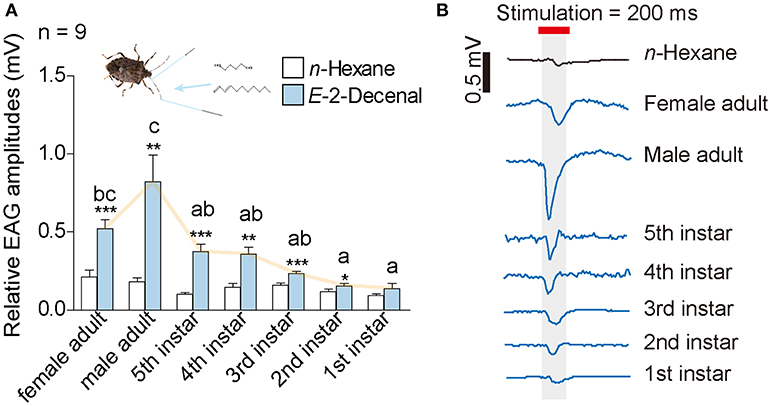
Figure 1. Results from EAG tests of H. halys. (A) A dosage of 10 μg (E)-2-decenal was used as treatment, and 10 μl n-hexan was used as control. Lower case letters indicate significant differences among H. halys stages in terms of EAG responses toward (E)-2-decenal [GLM and Tukey HSD, F(6, 61) = 10.09, P < 0.0001]. Asterisks indicate significant higher EAG responses of H. halys antennae toward (E)-2-decenal compared with n-hexane (t-tests, female adult: t = 7.7, P < 0.0001; male adult: t = 3.9, P = 0.0036; fifth instar nymph: t = 6.0, P = 0.0002; fourth instar nymph: t = 3.4, P = 0.0086; third instar nymph: t = 5.3, P = 0.0005; second instar nymph: t = 2.6, P = 0.03; first instar nymph: t = 1.9, P = 0.091). (B) Example traces of EAG responses from each stage and gender of H. halys. Original voltage data can be found in Data S1.
(E)-2-Decenal Dispersed All of the Developmental Stages of H. halys in a Temporospatial Manner
In a grouped dispersal tests with 30 H. halys adults, (E)-2-decenal caused significantly greater dispersal rates among tested replicates (Movie S1) than the n-hexane control, while the group treated with the latter showed little behavioral responses within 1 min (Movie S2). (E)-2-Decenal significantly influenced the behaviors of H. halys adults compared with n-hexane control (Figure 2A).
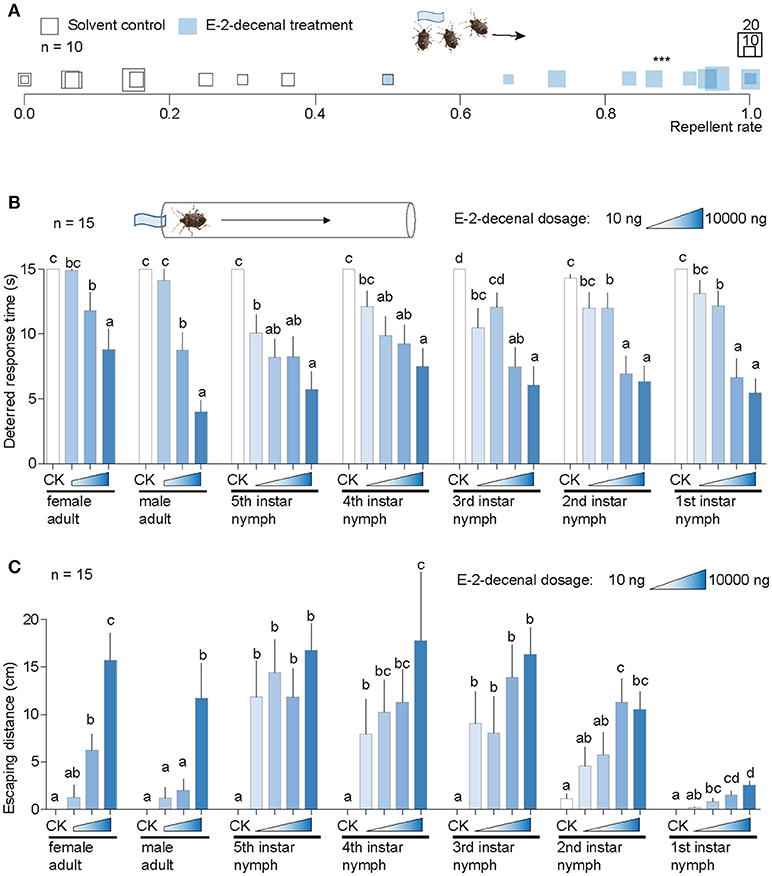
Figure 2. Behavioral responses of H. halys to (E)-2-decenal. (A) Results from a grouped test with 30 H. halys for each replicate. (E)-2-decenal was used at a dosage of 10 μg and solvent n-hexane was used as control. Square sizes indicate aggregated numbers of H. halys adults. Repellent rates for (E)-2-decenal and control were 84.1 ± 5.14% and 18.47 ± 5.25%, respectively. ***Indicates significant difference was observed between (E)-2-decenal and control [student's t-test, t(18) = 2.1, P < 0.001]. (B) Comparison of deterred response times of single H. halys in each developmental stage among (E)-2-decenal dosages. Solvent was used as control (CK). Different lower case letters indicate significant differences were observed among dosages in each developmental stage of H. halys [GLM and Tukey HSD, female adults: F(3, 86) = 15.0, P < 0.0001; male adults: F(3, 86) = 63.03, P < 0.0001; fifth instar nymphs: F(4, 115) = 26.2, P < 0.0001; fourth instar nymphs: F(4, 115) = 18.17, P < 0.0001; third instar nymphs: F(4, 115) = 24.88, P < 0.0001; second instar nymphs: F(4, 115) = 20.98, P < 0.0001; first instar nymphs: F(4, 115) = 40.11, P < 0.0001]. Error bars indicate + s.e.m. (C) Comparison of escaping distances of single H. halys in each developmental stage among (E)-2-decenal dosages. Solvent was used as control (CK). Different lower case letters indicate significant differences were observed among dosages in each developmental stage of H. halys [GLM and Tukey HSD, female adults: F(3, 86) = 32.15, P < 0.0001; male adults: F(3, 86) = 13.36, P < 0.0001; fifth instar nymphs: F(4, 115) = 18.5, P < 0.0001; fourth instar nymphs: F(4, 115) = 13.62, P < 0.0001; third instar nymphs: F(4, 115) = 14.55, P < 0.0001; second instar nymphs: F(4, 112) = 11.05, P < 0.0001; first instar nymphs: F(4, 115) = 22.78, P < 0.0001]. Error bars indicate + s.e.m.
We then used a route test to assess in detail the behavioral responses of H. halys individuals toward (E)-2-decenal. H. halys at each developmental stage were sensitive to this chemical, with the repellent effect starting at a tested dose of 10 ng to 5th instar nymphs (Figure 2B). All stages of H. halys were significantly repelled by 1 μg and 10 μg (E)-2-decenal compared with the n-hexane control. The exposure time prior to the initiation of escape behaviors significantly decreased as the dose of (E)-2-decenal increased in all tested stink bug stages (Figure 2B).
Halyomorpha halys nymphs moved farther to escape the chemical source compared with adults, excepted for the 1st instar nymphs. In particular, significantly longer moving distances were observed in 3rd to 5th instar nymphs for all tested doses of (E)-2-decenal compared with the n-hexane control (Figure 2C). Moving distances generally increased as the tested (E)-2-decenal concentration increased (Figure 2C). In comparison with other developmental stages, the 1st instar nymphs moved shorter distances at all tested doses (Figure 2C).
Five OBPs Were Screened as Putative Alarm Pheromone-Binding Proteins
In total, 44 OBPs were annotated in the H. halys antennal transcriptome. A phylogenic tree was established using OBPs from both Chinese and US populations of H. halys (Paula et al., 2016), D. melanogaster (Hekmat-Scafe et al., 2002), and A. lucorum (Yuan et al., 2015). In total, 14 OBPs, OBP8a, OBP31, OBP32, OBP33, OBP34, OBP35, OBP36, OBP37, OBP38, OBP39, OBP40, OBP46, OBP83, and OBP99, were newly identified in the Chinese H. halys populations (Figure 3). By aligning the amino acid sequences of H. halys OBPs, we found that 36 out of 44 OBPs contained the normal six conserved Cys residues, indicating that the predicted C-pattern motif was indicative of a classic OBP in insects (Xu et al., 2009). OBP46 and seven other previously reported OBPs (Paula et al., 2016) had additional conserved Cys residues and were classified as Plus-C OBPs (Figure 3).
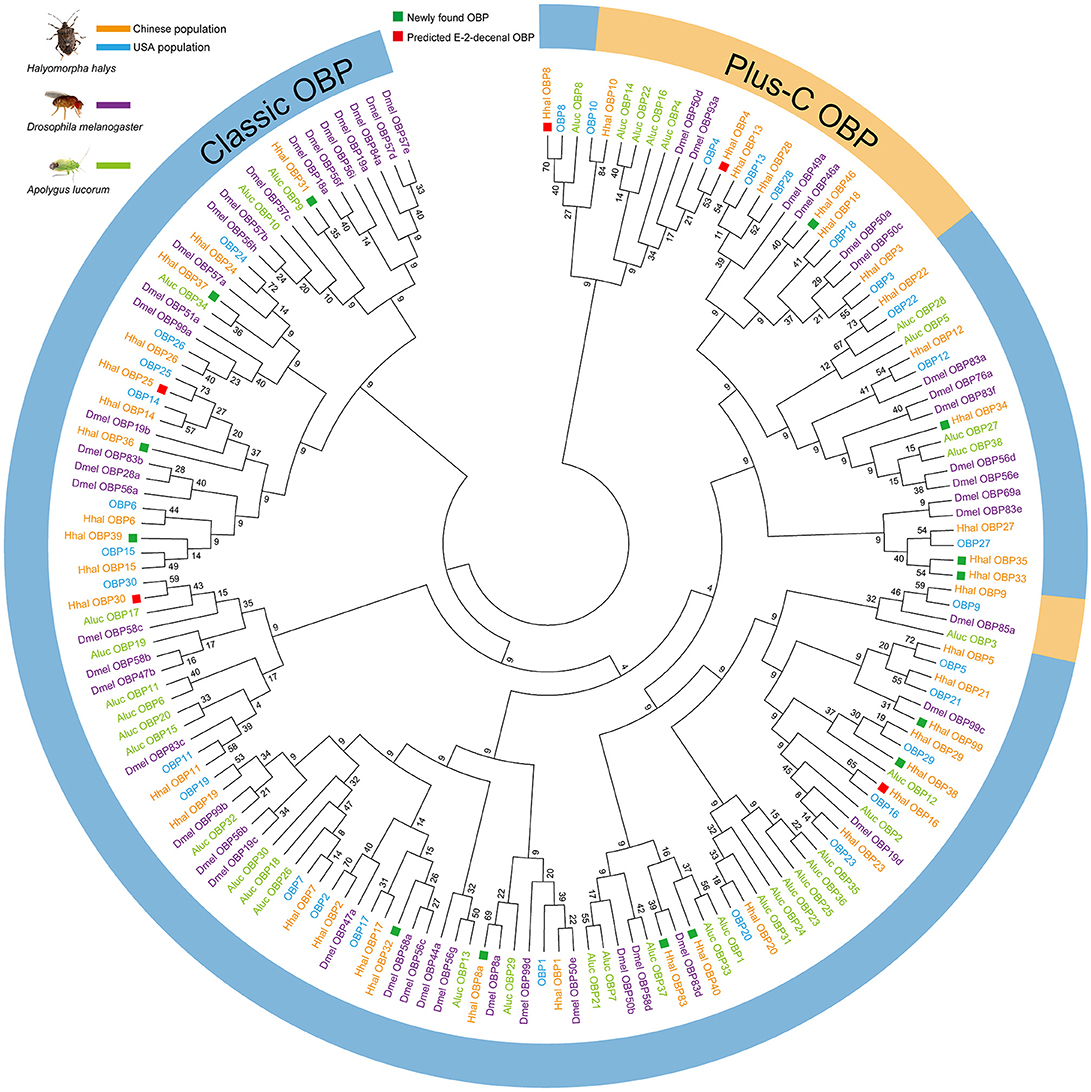
Figure 3. Neighbor-Joining phylogenetic tree established from candidate OBP sequences from two H. halys populations and other two insect species. The evolutionary history was inferred using the Neighbor-Joining method. The optimal tree with the sum of branch length = 95.69757843 is shown. The evolutionary distances were computed using the number of differences method and are in the units of the number of amino acid differences per sequence. The analysis involved 163 amino acid sequences. All positions containing gaps and missing data were eliminated. There were a total of 4 positions in the final dataset. Evolutionary analyses were conducted in MEGA7. Green squares showed 14 newly annotated OBPs in antennal transcriptome from Chinese strain of H. halys. Red squares indicate putative (E)-2-decenal binding OBPs (HhalOBP25, HhalOBP30, HhalOBP16, HhalOBP8, and HhalOBP4). Included are OBP genes identified from: Halyomorpha halys (Hhal: Chinese strain; OBP: USA strain), Apolygus lucorum (Aluc), and Drosophila melanogaster (Dmel). Outside circle shows predicted classic OBPs and plus-C OBPs.
The expression levels of OBP25, OBP30, OBP16, OBP8, and OBP4 in US H. halys populations greatly increase after exposure to an alarm pheromone (Paula et al., 2016). We successfully identified homologs for all five of these OBPs in Chinese populations (Figure 3). Moreover, all five OBPs had conserved amino acid sequences between US and Chinese populations, with identity levels > 99% (Figure 4). The highly conserved amino acid sequences of these five OBPs indicated that they may also be functionally conserved among different populations. Both behavioral assays and chemical analyses revealed that (E)-2-decenal is the main functional alarm ingredient (Zhong et al., 2017), which suggests that OBP25, OBP30, OBP16, OBP8, and OBP4 in H. halys may contribute to the olfactory reception of (E)-2-decenal. We selected these five OBPs to further study their functional characteristics and binding activities to this chemical.
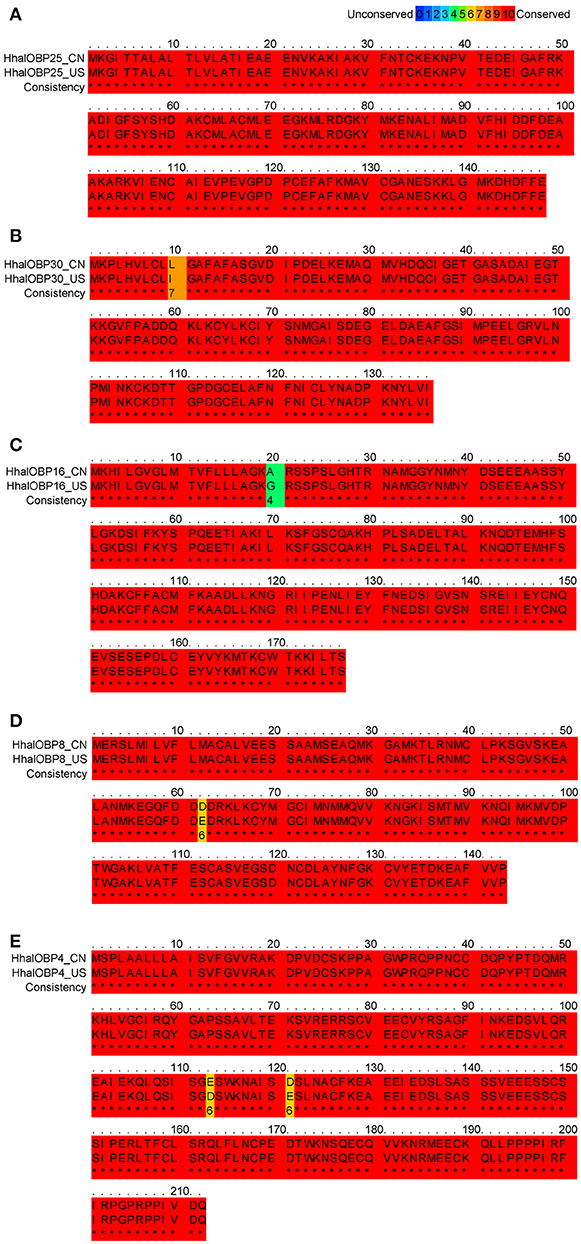
Figure 4. Multiple alignments of H. halys OBP25, OBP30, OBP16, OBP8, and OBP4. (A) Alignment of H. halys OBP25 amino acid sequences between the Chinese (CN) and the American (US) populations. The amino acid identity of the two populations was 100%. (B) Alignment of H. halys OBP30 amino acid sequences between the Chinese (CN) and the American (US) population. The amino acid identity of the two populations was 99%. (C) Alignment of H. halys OBP16 amino acid sequences between the Chinese (CN) and the American (US) populations. The amino acid identity of the two populations was 99%. (D) Alignment of H. halys OBP8 amino acid sequences between the Chinese (CN) and the American (US) populations. The amino acid identity of the two populations was 99%. (E) Alignment of H. halys OBP4 amino acid sequences between the Chinese (CN) and the American (US) populations. The amino acid identity of the two populations was 99%. Letters in red boxes (*) represent fully conserved amino acids while unconserved residues were shown by different colors.
Expression and Purification of OBP25, OBP30, OBP16, OBP8, and OBP4
The chosen five OBP genes were duplicated in vitro through a bacterial expression system. The purification was performed following a standard protocol (Prestwich, 1993; Calvello et al., 2003). After the cleavage of the His-tag and purification, sodium dodecyl sulfate-polyacrylamide gel electrophoresis (SDS-PAGE) and western blotting showed that the molecular masses of OBP25, OBP30, and OBP8 were ~15 kDa, while those of OBP16 and OBP4 were ~18 and 28 kDa, respectively. The molecular mass information obtained from SDS-PAGE and western blot tests were consistent with the amino acid lengths predicted from the H. halys antennal transcriptome (Figure 5).
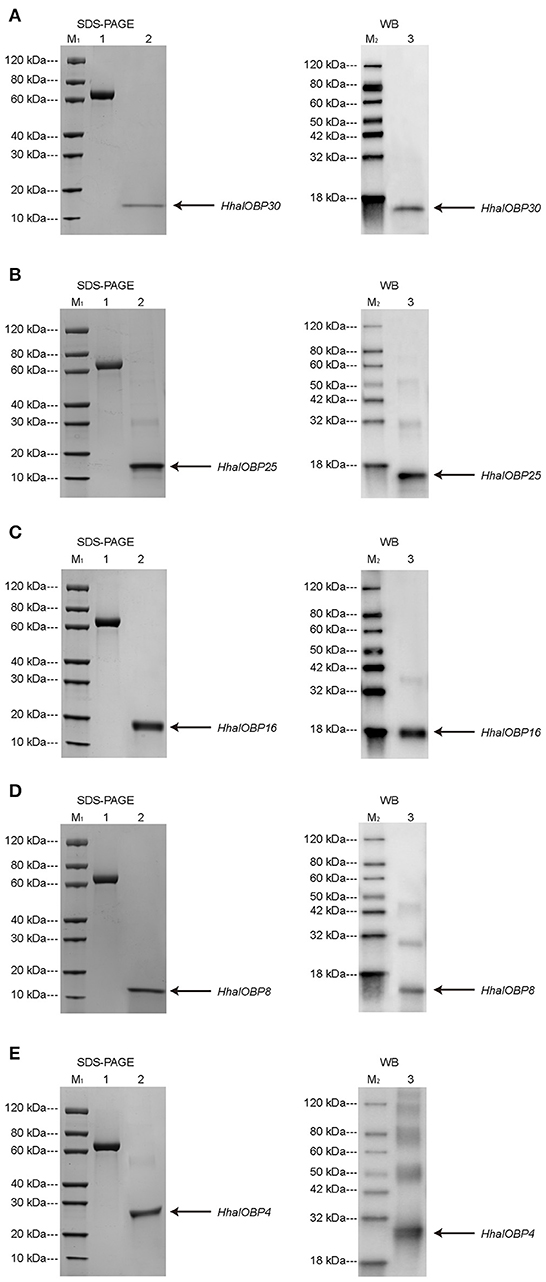
Figure 5. SDS-PAGE (left) and Western blot (right) analysis for purified H. halys OBP30 (A), H. halys OBP25 (B), H. halys OBP16 (C), H. halys OBP8 (D), and H. halys OBP4 (E) expression. Arrows indicate the protein bands. M1 and M2 were molecular markers. 1: bovine serum protein as a reference. 2 & 3: purified OBPs.
Binding Activities of OBP25, OBP30, OBP16, OBP8, and OBP4 to (E)-2-Decenal
The fluorescent probe 1-NPN was first used to bind the OBPs. Significant increases in fluorescence intensity levels were observed when the OBPs were added. Among the tested OBPs, OBP25 revealed the highest slope, yet was saturated at the lowest intensity. The 1-NPN dissociation constants for the OBPs were calculated from correlation curves as shown in Table 2 (Figures 6A–E, left column, Data S2).
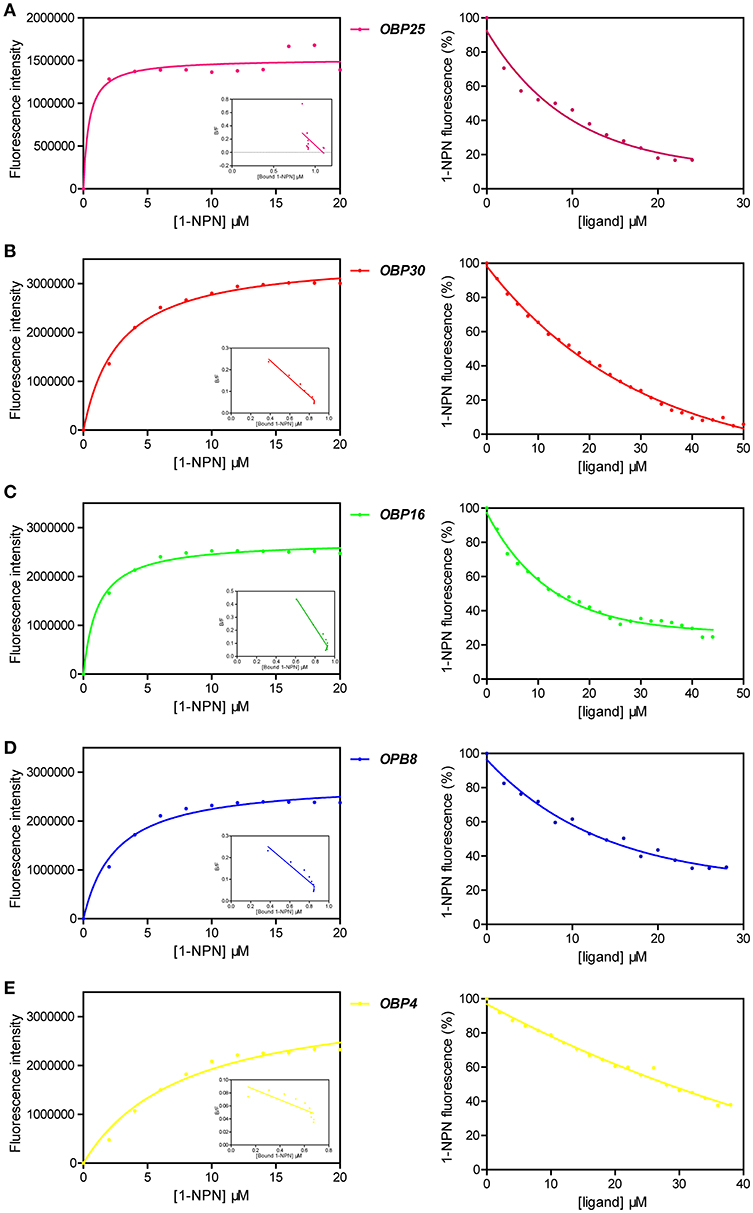
Figure 6. Competitive fluorescence binding assays of H. halys OBPs for (E)-2-decenal ligand, with 1-NPN as the fluorescent probe. Detailed calculation for key measurements were listed in Table 2. Means from 3 replicates were used in the plotting and original data sheets were provided in Data S2. (A) Left: binding curve of 1-NPN to H. halys OBP25 and the resulting Scatchard plot. Right: competitive binding curve of (E)-2-decenal to OBP25. (B) Left: binding curve of 1-NPN to H. halys OBP30 and the resulting Scatchard plot. Right: competitive binding curve of (E)-2-decenal to OBP30. (C) Left: binding curve of 1-NPN to H. halys OBP16 and the resulting Scatchard plot. Right: competitive binding curve of (E)-2-decenal to OBP16. (D) Left: binding curve of 1-NPN to H. halys OBP8 and the resulting Scatchard plot. Right: competitive binding curve of (E)-2-decenal to OBP8. (E) Left: binding curve of 1-NPN to H. halys OBP4 and the resulting Scatchard plot. Right: competitive binding curve of (E)-2-decenal to OBP4.
We then challenged each OBP/1-NPN complex with (E)-2- decenal to test their binding affinities to this alarm pheromone component. All five OBPs bound to (E)-2-decenal (Table 2, Figure 6). The top four highly expressed OBPs increased from 100,000- to 100-fold (Paula et al., 2016) when H. halys was treated with the alarm pheromone mixture, and they all showed excellent binding activities to (E)-2-decenal, with IC50 values < 32 μM and Kd values < 20 μM. However, OBP4 increased only by 10-fold and did not show a good binding activity to (E)-2-decenal. Thus, (E)-2-decenal is likely a major ligand of OBP25, OBP30, OBP16, and/or OBP8, but OBP4 may be a general-binding protein that uses (E)-2-decenal as only a minor ligand. The functions of these five OBPs may be essential for H. halys to successfully initially detect (E)-2-decenal as an alarm signal.
Discussion
In the current study, behavioral responses of H. halys elicited by (E)-2-decenal as an alarm pheromone were revealed through comprehensive electrophysiological tests and laboratory bioassays. However, although greater EAG responses were observed in adult H. halys, they did not show a corresponding greater behavioral sensitivity to low doses in comparison with nymphs. This may result from differences in peripheral or central coding patterns among the developmental stages of the insect (Hansson and Stensmyr, 2011; Junker et al., 2017; Haverkamp et al., 2018). (E)-2-Decenal may be sensed through combinatorial coding that decides its final behavioral effects on adult H. halys, which suggests that it may have other functional roles in adults (Haverkamp et al., 2018). Because EAG tests can only record the overall electrophysiological responses from the whole antenna, further investigations on (E)-2-decenal sensilla's sensing diversity and distributional mapping will be needed. Moreover, compared with adults, H. halys nymphs were more sensitive to (E)-2-decenal as assessed by reaction times and escape distances from the chemical source. The exceptional was 1st instar nymphs, which might be attributed to their incomplete neurological systems development (Fu et al., 2013). According to previously reported works, 3rd instar nymphs emit a greater level of (E)-2-decenal than adult males or females (Harris et al., 2015). Studies on other pentatomids indicate that nymphal defensive secretions are enriched in high-molecular weight constituents, presumably because the flightless immature stages require longer-lasting protection (Aldrich, 1988). Thus, we hypothesized that the greater sensitivity of nymphal H. halys, except 1st instars, to (E)-2-decenal helps them disperse quickly and avoid threats from natural enemies in the environment, resulting in higher survival rates.
(E)-2-Decenal has also been identified as a component of defensive secretions in other pentatomids, such as Nezara viridula (Lockwood and Story, 1987), Erthesina fullo (Kou et al., 1989), Chinavia impicticornis, and Chinavia ubica (Pareja et al., 2007). However, (E)-2-decenal had no alarm effects on adult N. viridula as assessed by the olfactometer assay (Lockwood and Story, 1987), and to our knowledge, its exact alarm function has not been clarified in other pentatomids, except our present study. Exposure to (E)-2-decenal acted as a strong feeding deterrent for H. halys in laboratory trials when it was combined with tridecane (Zhang et al., 2014a), which is a major component of the defensive chemicals emitted by H. halys (Harris et al., 2015; Zhong et al., 2017). Moreover, (E)-2-decenal has a fungistatic effect and inhibits spore germination in entomopathogenic fungi (Weber et al., 2017b).
In the antennal transcriptome analysis, we found 14 more OBP genes in the Chinese H. halys populations. As the pest originated from East Asia, this result is not surprising because geographically ancient populations usually maintain a higher divergence in the relevant functional protein abundance owing to the longer evolutionary process and a broader genomic variation in populations (Gariepy et al., 2014; Zhu et al., 2016). Genetic analysis also showed much lower genetic diversity in US H. halys populations compared to Chinese populations (Xu et al., 2013). Interestingly, the screened amino acid sequences of OBP25, OBP30, OBP16, OBP8, and OBP4 were highly conserved between a Chinese and US population but not completely the same. However, because (E)-2-decenal exists in both populations (Harris et al., 2015; Zhong et al., 2017), we expect that these OBPs will be functionally conserved in both populations.
According to the slopes of the competitive binding assays, the order of the tested OBPs binding activities to (E)-2-decenal was OBP25 > OBP16 > OBP 30 > OBP 8 > OBP4. As OBP25 was observed in the binding assays to have the highest affinity for (E)-2-decenal, this protein may be involved in a rapid response pathway that drives the initial escape behavior of H. halys within a short time period after exposure to low levels of (E)-2-decenal. The other four OBPs, which have weaker affinities for (E)-2-decenal, are therefore less sensitive, and they could be involved in detecting higher levels of (E)-2-decenal.
In addition, OBPs usually do not act exclusively as odorant receptors and may have a spectrum of chemical ligands (Leal, 2013; Larter et al., 2016). The OBPs with lower increases in expression, such as OBP16, OBP8, and OBP4, may also serve as more general carriers that deal with multiple semiochemicals, such as other components in the alarm pheromone blends. Moreover, the newly annotated 14 OBPs may contribute to (E)-2-decenal olfactory sensing; therefore, it is desirable to further investigate their olfactory functions.
In summary, our results have revealed the clear behavioral effects of (E)-2-decenal on adult H. halys of both sexes and the nymphal stages, and this alarm pheromone component was sensed by olfactory reception through OBP25, OBP30, OBP16, OBP8, and OBP4. (E)-2-Decenal mediates the host-finding and oviposition behaviors of Trissolcus japonicas, an egg parasitoid of H. halys (Zhong et al., 2017). Therefore, the information regarding these five H. halys OBPs provides insights that can be used to identify potential (E)-2-decenal OBPs in T. japonicas wasps. Because of the higher tolerance of adult H. halys to (E)-2-decenal and the discovery of diversified candidate OBPs, we hypothesize that the chemosensitization of H. halys to (E)-2-decenal through its olfactory system is complex. The current study contributes to the understanding of H. halys' olfactory perception of its alarm pheromone components, but further studies are needed to determine the complete olfactory mechanism. Such knowledge of an alarm pheromone will be potentially useful in developing behavioral-mediating tools as part of integrated pest management programs to control this pest in native and invaded regions.
Author Contributions
FZ, G-HC, J-PZ, and RT designed the study. Y-ZZ and J-PZ conducted the behavioral assays. Y-ZZ and RT conducted the electrophysiological and molecular tests. K-LH and Z-YW helped in carrying out the molecular work. S-YY and RT conducted the antennal transcriptome analysis. Y-ZZ, RT, and FZ analyzed the data and drafted the manuscript with inputs provided from all.
Funding
This research was supported by China's Donation to the CABI Development Fund, the Belt and Road Plant Protection Project of CIAR-CAAS, and the National Key Research and Development Program of China (2016YFC1200600).
Conflict of Interest Statement
The authors declare that the research was conducted in the absence of any commercial or financial relationships that could be construed as a potential conflict of interest.
Acknowledgments
CABI is an international intergovernmental organization and we gratefully acknowledge the core financial support from our member countries (and lead agencies) including the United Kingdom (Department for International Development), China (Chinese Ministry of Agriculture and Rural Affairs), Australia (Australian Centre for International Agricultural Research), Canada (Agriculture and Agri-Food Canada), Netherlands (Directorate-General for International Cooperation), and Switzerland (Swiss Agency for Development and Cooperation). See https://www.cabi.org/about-cabi/who-we-work-with/key-donors/ for full details. We thank B.F.A. Xiao-Qian Bao for technical support on development of insect graphics.
Supplementary Material
The Supplementary Material for this article can be found online at: https://www.frontiersin.org/articles/10.3389/fphys.2018.01610/full#supplementary-material
References
Aldrich, J. (1988). Chemical ecology of the Heteroptera. Ann. Rev. Entomol. 33, 211–238. doi: 10.1146/annurev.en.33.010188.001235
Bernays, E. A., and Chapman, R. E. (1994). Host-Plant Selection by Phytophagous Insects. Springer US 1754–1756. doi: 10.1007/b102508
Borges, M., and Aldrich, J. R. (1992). Instar-specific defensive secretions of stink bugs (Heteroptera: Pentatomidae). Experientia 48, 893–896. doi: 10.1007/BF02118429
Calvello, M., Guerra, N., Brandazza, A., D'Ambrosio, C., Scaloni, A., Dani, F. R., et al. (2003). Soluble proteins of chemical communication in the social wasp Polistes dominulus. Cell. Mol. Life Sci. 60, 1933–1943. doi: 10.1007/s00018-003-3186-5
Dickens, J. C., Callahan, F. E., Wergin, W. P., and Erbe, E. F. (1995). Olfaction in a hemimetabolous insect - antennal-specific protein in adult Lygus lineolaris (Heteroptera, Miridae). J. Insect Physiol. 41, 857–867. doi: 10.1016/0022-1910(95)00038-V
Fu, B. X., Zhu, Z. R., Rong, N. H., Hong, J., Zhang, C. X., and Cheng, J. A. (2013). Phase-related developmental characteristics of antennal sensilla of nymphal Laodelphax striatellus (Hemiptera: Delphacidae), a serious virus-transmitting insect vector of graminaceous crops. Ann. Entomol. Soc. Am. 106, 626–636. doi: 10.1603/AN13019
Gariepy, T. D., Haye, T., Fraser, H., and Zhang, J. (2014). Occurrence, genetic diversity, and potential pathways of entry of Halyomorpha halys in newly invaded areas of Canada and Switzerland. J. Pest. Sci. 87, 17–28. doi: 10.1007/s10340-013-0529-3
Hansson, B., and Stensmyr, M. (2011). Evolution of insect olfaction. Neuron 72, 698–711. doi: 10.1016/j.neuron.2011.11.003
Harris, C., Abubeker, S., Yu, M., Leskey, T., and Zhang, A. (2015). Semiochemical production and laboratory behavior response of the brown marmorated stink bug, Halyomorpha Halys. PLoS ONE 10:e0140876. doi: 10.1371/journal.pone.0140876
Haverkamp, A., Hansson, B. S., and Knaden, M. (2018). Combinatorial codes and labeled lines: how insects use olfactory cues to find and judge food, mates, and oviposition sites in complex environments. Front. Physiol. 9:49. doi: 10.3389/fphys.2018.00049
Haye, T., and Weber, D. C. (2017). Special issue on the brown marmorated stink bug, Halyomorpha halys: an emerging pest of global concern. J. Pest. Sci. 90, 987–988. doi: 10.1007/s10340-017-0897-1
Hekmat-Scafe, D. S., Scafe, C. R., McKinney, A. J., and Tanouye, M. A. (2002). Genome-wide analysis of the odorant-binding protein gene family in Drosophila melanogaster. Genome Res. 12, 1357–1369. doi: 10.1101/gr.239402
Junker, R. R., Kuppler, J., Amo, L., Blande, J. D., Borges, R. M., van Dam, N. M., et al. (2017). Covariation and phenotypic integration in chemical communication displays: biosynthetic constraints and eco-evolutionary implications. N. Phytol. 220, 739–749. doi: 10.1111/nph.14505
Kitamura, C., Wakamura, S., and Takahashi, S. (2008). Identification and functions of ventral glands secretion of some Heteroptera. Appl. Entomol. Zool. 19, 33–41. doi: 10.1303/aez.19.33
Kou, R., Tang, D. S., and Chow, Y. S. (1989). Alarm pheromone of pentatomid bug, Erthesina fullo Thunberg (Hemiptera: Pentatomidae). J. Chem. Ecol. 15, 2695–2702. doi: 10.1007/BF01014726
Kuhar, T. P., and Kamminga, K. (2017). Review of the chemical control research on Halyomorpha halys in the USA. J. Pest. Sci. 90, 1021–1031. doi: 10.1007/s10340-017-0859-7
Kumar, S., Stecher, G., and Tamura, K. (2016). MEGA7: molecular evolutionary genetics analysis version 7.0 for bigger datasets. Mol. Biol. Evol. 33, 1870–1874. doi: 10.1093/molbev/msw054
Larter, N. K., Sun, J. S., and Carlson, J. R. (2016). Organization and function of Drosophila odorant binding proteins. Elife 5:e20242. doi: 10.7554/eLife.20242
Leal, W. S. (2013). Odorant reception in insects: roles of receptors, binding proteins, and degrading enzymes. Annu. Rev. Entomol. 58, 373–391. doi: 10.1146/annurev-ento-120811-153635
Lee, D., Short, B. D., Joseph, S. V., Christopher, J., Leskey, T. C., and Bergh, J. C. (2013). Review of the biology, ecology, and management of Halyomorpha halys (Hemiptera: Pentatomidae) in China, Japan, and the Republic of Korea. Environ. Entomol. 42, 627–641. doi: 10.1603/EN13006
Leskey, T. C., and Nielsen, A. L. (2018). Impact of the invasive brown marmorated stink bug in North America and Europe: history, biology, ecology, and management. Annu. Rev. Entomol. 63, 599–618. doi: 10.1146/annurev-ento-020117-043226
Lockwood, J. A., and Story, R. N. (1987). Defensive secretion of the southern green stink bug (Hemiptera: Pentatomidae) as an alarm pheromone. Ann. Entomol. Soc. Am. 80, 686–691. doi: 10.1093/aesa/80.5.686
Lopes, R. B., Laumann, R. A., Blassioli-Moraes, M. C., Borges, M., and Faria, M. (2015). The fungistatic and fungicidal effects of volatiles from metathoracic glands of soybean-attacking stink bugs (Heteroptera: Pentatomidae) on the entomopathogen Beauveria bassiana. J. Invert. Pathol. 132, 77–85. doi: 10.1016/j.jip.2015.08.011
Mogilicherla, K., Howell, J. L., and Palli, S. R. (2018). Improving RNAi in the brown marmorated stink bug: identification of target genes and reference genes for RT-qPCR. Sci. Rep. 8:3720 doi: 10.1038/s41598-018-22035-z
Moraes, M. C., Pareja, M., Laumann, R. A., and Borges, M. (2008). The chemical volatiles (semiochemicals) produced by neotropical stink bugs (Hemiptera: Pentatomidae). Neotrop. Entomol. 37, 489–505. doi: 10.1590/S1519-566X2008000500001
Noge, K., and Becerra, J. X. (2012). Defensive roles of (E)-2-alkenals and related compounds in Heteroptera. J. Chem. Ecol. 38, 1050–1056. doi: 10.1007/s10886-012-0166-y
Noge, K., Kakuda, T., Abe, M., and Tamogami, S. (2015). Identification of the alarm pheromone of Hygia lativentris and changes in composition during development. J. Chem. Ecol. 41, 757–765. doi: 10.1007/s10886-015-0607-5
Pareja, M., Borges, M., Laumann, R. A., and Moraes, M. C. B. (2007). Inter- and intraspecific variation in defensive compounds produced by five neotropical stink bug species (Hemiptera: Pentatomidae). J. Insect. Physiol. 53, 639–648. doi: 10.1016/j.jinsphys.2007.04.004
Paula, D. P., Togawa, R. C., Costa, M. M., Grynberg, P., Martins, N. F., and Andow, D. A. (2016). Identification and expression profile of odorant-binding proteins in Halyomorpha halys (Hemiptera: Pentatomidae). Insect. Mol. Biol. 25, 580–594. doi: 10.1111/imb.12243
Prestwich, G. D. (1993). Bacterial expression and photoaffinity labeling of a pheromone binding protein. Protein Sci. 2, 420–428. doi: 10.1002/pro.5560020314
Sagun, S., Collins, E., Martin, C., Nolan, E. J., and Horzempa, J. (2016). Alarm odor compounds of the brown marmorated stink bug exhibit antibacterial activity. J. Pharmacogn. Nat. Prod. 2:119. doi: 10.4172/2472-0992.1000119
Saitou, N., and Nei, M. (1987). The Neighbor-joining method: a new method for reconstructing phylogenetic trees. Mol. Biol. Evol. 4, 406–425.
Schoonhoven, L. M., Loon, J. J. A. V., and Dicke, M. (2005). Insect-Plant Biology. Oxford: Oxford University Press.
Tada, N., Yoshida, M., and Sato, Y. (2001a). Monitoring of forecasting for stink bugs in apple. 1. Characteristics of attraction to aggregation pheromone in Iwate Prefecture. Ann. Rep. Soc. Plant. Protec. Nor. Jpn. 2001, 224–226. doi: 10.11455/kitanihon1966.2001.224
Tada, N., Yoshida, M., and Sato, Y. (2001b). Monitoring of forecasting for stink bugs in apple: 2. The possibility of forecasting with aggregation pheromone. Ann. Rep. Soc. Plant. Protec. Nor. Jpn. 2001, 227–229. doi: 10.11455/kitanihon1966.2001.227
Tang, R., Zhang, F., Kone, N. G., Chen, J. H., Zhu, F., Han, R. C., et al. (2016). Identification and testing of oviposition attractant chemical compounds for Musca domestica. Sci. Rep. 6:33017. doi: 10.1038/srep33017
Tognon, R., Sant'Ana, J., Zhang, Q. H., Millar, J. G., Aldrich, J. R., and Zalom, F. G. (2016). Volatiles mediating parasitism of euschistus conspersus and Halyomorpha halys eggs by Telenomus podisi and Trissolcus erugatus. J. Chem. Ecol. 42, 1–12. doi: 10.1007/s10886-016-0754-3
Valentin, R. E., Nielsen, A. L., Wiman, N. G., Lee, D. H., and Fonseca, D. M. (2017). Global invasion network of the brown marmorated stink bug, Halyomorpha halys. Sci. Rep. 7:9866 doi: 10.1038/s41598-017-10315-z
Vogt, R. G., and Riddiford, L. M. (1981). Pheromone binding and inactivation by moth antennae. Nature 293, 161–163. doi: 10.1038/293161a0
Vosshall, L. B., and Stensmyr, M. C. (2005). Wake up and smell the pheromones. Neuron 45, 179–181. doi: 10.1016/j.neuron.2005.01.001
Weber, D. C., Khrimian, A., Blassioli-Moraes, M., and Millar, J. (2017a). Semiochemistry of Pentatomoidea, Chapter 15. Invasive Stink Bugs and Related Species: Biology, Higher Systematics, Semiochemistry, and Management. Boca Raton, FL: CRC Press.
Weber, D. C., Morrison, W. R., Khrimian, A., Rice, K. B., Leskey, T. C., Rodriguez-Saona, C., et al. (2017b). Chemical ecology of Halyomorpha halys: discoveries and applications. J. Pest. Sci. 90, 989–1008. doi: 10.1007/s10340-017-0876-6
Wicher, D. (2015). Olfactory signaling in insects. Prog. Mol. Biol. Transl. Sci. 130, 37–54. doi: 10.1016/bs.pmbts.2014.11.002
Xu, J., Fonseca, D. M., Hamilton, G. C., Hoelmer, K. A., and Nielsen, A. L. (2013). Tracing the origin of US brown marmorated stink bugs, Halyomorpha halys. Biol. Invasions. 16, 153–166. doi: 10.1007/s10530-013-0510-3
Xu, Y. L., He, P., Zhang, L., Fang, S. Q., Dong, S. L., Zhang, Y. J., et al. (2009). Large-scale identification of odorant-binding proteins and chemosensory proteins from expressed sequence tags in insects. BMC Genom. 10:632. doi: 10.1186/1471-2164-10-632
Yuan, H. B., Ding, Y. X., Gu, S. H., Sun, L., Zhu, X. Q., Liu, H. W., et al. (2015). Molecular characterization and expression profiling of odorant-binding proteins in Apolygus lucorum. PLoS ONE 10:e0140562. doi: 10.1371/journal.pone.0140562
Zhang, A., Harris, C., and Leskey, T. C. (2014a). Methods and Compositions for Deterring Feeding/Repelling the Brown Marmorated Stink Bug (BMSB), Halyomorpha halys. United State Patent US 20140256825 A1.
Zhang, Q. H., Schneidmiller, R. G., Hoover, D. R., Zhou, G., Margaryan, A., and Bryant, P. (2014b). Essential oils as spatial repellents for the brown marmorated stink bug, Halyomorpha halys (Stål) (Hemiptera: Pentatomidae). J. Appl. Entomol. 138, 490–499. doi: 10.1111/jen.12101
Zhong, Y. Z., Zhang, J. P., Ren, L. L., Tang, R., Zhan, H. X., Chen, G. H., et al. (2017). Behavioral responses of the egg parasitoid Trissolcus japonicus to volatiles from adults of its stink bug host, Halyomorpha halys. J. Pest. Sci. 90, 1097–1105. doi: 10.1007/s10340-017-0884-6
Keywords: brown marmorated stink bug, (E)-2-decenal, dispersal, olfaction, transcriptome, odorant-binding protein
Citation: Zhong Y-Z, Tang R, Zhang J-P, Yang S-Y, Chen G-H, He K-L, Wang Z-Y and Zhang F (2018) Behavioral Evidence and Olfactory Reception of a Single Alarm Pheromone Component in Halyomorpha halys. Front. Physiol. 9:1610. doi: 10.3389/fphys.2018.01610
Received: 16 July 2018; Accepted: 25 October 2018;
Published: 13 November 2018.
Edited by:
Shigehiro Namiki, The University of Tokyo, JapanReviewed by:
Robert Renthal, University of Texas at San Antonio, United StatesMaria Carolina Blassioli Moraes, Empresa Brasileira de Pesquisa Agropecuária (EMBRAPA), Brazil
Copyright © 2018 Zhong, Tang, Zhang, Yang, Chen, He, Wang and Zhang. This is an open-access article distributed under the terms of the Creative Commons Attribution License (CC BY). The use, distribution or reproduction in other forums is permitted, provided the original author(s) and the copyright owner(s) are credited and that the original publication in this journal is cited, in accordance with accepted academic practice. No use, distribution or reproduction is permitted which does not comply with these terms.
*Correspondence: Feng Zhang, f.zhang@cabi.org http://orcid.org/0000-0002-7812-6645
†Rui Tang http://orcid.org/0000-0002-9313-0802
‡These authors have contributed equally to this work