- 1Physiology Section, Department of Cell Biology, Physiology and Immunology, Faculty of Biology, Universitat de Barcelona, Barcelona, Spain
- 2Exercise Physiology Unit, Department of Physiological Sciences, Faculty of Medicine and Health Sciences, Universitat de Barcelona, L'Hospitalet de Llobregat, Barcelona, Spain
In recent years, the altitude acclimatization responses elicited by short-term intermittent exposure to hypoxia have been subject to renewed attention. The main goal of short-term intermittent hypobaric hypoxia exposure programs was originally to improve the aerobic capacity of athletes or to accelerate the altitude acclimatization response in alpinists, since such programs induce an increase in erythrocyte mass. Several model programs of intermittent exposure to hypoxia have presented efficiency with respect to this goal, without any of the inconveniences or negative consequences associated with permanent stays at moderate or high altitudes. Artificial intermittent exposure to normobaric hypoxia systems have seen a rapid rise in popularity among recreational and professional athletes, not only due to their unbeatable cost/efficiency ratio, but also because they help prevent common inconveniences associated with high-altitude stays such as social isolation, nutritional limitations, and other minor health and comfort-related annoyances. Today, intermittent exposure to hypobaric hypoxia is known to elicit other physiological response types in several organs and body systems. These responses range from alterations in the ventilatory pattern to modulation of the mitochondrial function. The central role played by hypoxia-inducible factor (HIF) in activating a signaling molecular cascade after hypoxia exposure is well known. Among these targets, several growth factors that upregulate the capillary bed by inducing angiogenesis and promoting oxidative metabolism merit special attention. Applying intermittent hypobaric hypoxia to promote the action of some molecules, such as angiogenic factors, could improve repair and recovery in many tissue types. This article uses a comprehensive approach to examine data obtained in recent years. We consider evidence collected from different tissues, including myocardial capillarization, skeletal muscle fiber types and fiber size changes induced by intermittent hypoxia exposure, and discuss the evidence that points to beneficial interventions in applied fields such as sport science. Short-term intermittent hypoxia may not only be useful for healthy people, but could also be considered a promising tool to be applied, with due caution, to some pathophysiological states.
Intermittent Hypoxia: Concept and Historical Background
The term “intermittent hypoxia” is widely used and applies to a wide spectrum of situations that range from alpine expeditions to obstructive sleep apnea (OSA). However, in physiological terms, there are often few similarities between these conditions (Viscor et al., 2014). Although the same physiological responses are elicited by the same sensors and signaling pathways, the varied intensity and duration of the hypoxia switches different mechanisms on and off at different times, thus making the final physiological changes induced in the whole organism highly variable. In addition, a high variability in human tolerance to hypoxia has been reported, and it is now known to vary throughout the lives of individuals (Canouï-Poitrine et al., 2014; Richalet and Lhuissier, 2015). As a consequence, specialists have engaged in an interesting discussion about how to measure and define hypoxic “dosage” (Serebrovskaya et al., 2008; Garvican-Lewis et al., 2016). In general, three different types of intermittent hypoxia can be considered:
a) Episodic intermittent hypoxia, which consists of successive short episodes of hypoxia with variable intensity. This may be present in permanent situations such as OSA, in transitory situations such as surgical ischemia-reperfusion (to one or several organs) and in some sporting activities such as parachuting and extreme skiing, in which the subject may not even notice the hypoxia.
b) Intervallic intermittent hypoxia, which is characterized by long periods of normoxia interspersed with periods of hypoxia, as seen in frequent alpine expeditions and trekking at altitude, mountain rescue teams, regular intercontinental commercial flight crews (when in flight, commercial airplane cabins are usually regulated at pressures equivalent to a moderate altitude of about 2,000 m above sea level), and even astronauts at the International Space Station or future extraplanetary missions.
c) Chronic intermittent hypoxia, which affects individuals who work under shift systems characterized by work at moderate or high altitudes alternating with periods of rest at sea level. This last model is common in the Andes region and in the Central Asian mining industry, but is also found in contractors for astronomical observatories, the military and customs, and police and border control personnel in many high-elevation countries.
The concept of “hypoxic training” was coined during the 1930s in the academic environment of the former Soviet Union, and was considered a useful therapeutic tool after it was shown to have beneficial effects on a number of different pathologies, even though the mechanisms involved in these favorable effects were unclear (Agadzhanyan and Torshin, 1986; Serebrovskaya, 2002). For instance, it was reported to have a beneficial effect on hypertension and cardiovascular diseases (Aleshin et al., 1993). Later, new experimental studies corroborated some of these findings and provided a fresh insight through enhanced plasma lipid profiles (Tin'kov and Aksenov, 2002). Nowadays in former Soviet States, hypoxic training is systematically applied as a non-pharmacological strategy for treating a wide range of alterations, including chronic lung disease, bronchial asthma, hypertension, diabetes mellitus, Parkinson's disease, emotional disorders and radiation toxicity, and for the prophylactic treatment of some occupational diseases (Ge et al., 1994; Xi and Serebrovskaya, 2012). In Western countries, intermittent hypoxia exposure programs were first applied in the field of sports medicine to improve aerobic capacity and for pre-acclimatization to altitude (Richalet et al., 1992; Levine and Stray-Gundersen, 1997; Rodríguez et al., 1999; Stray-Gundersen and Levine, 1999; Casas M. et al., 2000; Ricart et al., 2000).
Our group took an in-depth look at the physiological responses to intermittent exposure to hypobaric hypoxia (IEHH) in hypobaric (low barometric pressure) chambers. A detailed study of the precise mechanisms that underlie these adaptive responses (erythropoiesis, angiogenesis and the release of circulating stem cells) in humans and in an experimental rodent model encouraged us to explore the possibilities of applying IEHH programs with biomedical and therapeutic purposes (Panisello et al., 2007, 2008; Esteva et al., 2009; Viscor et al., 2009; Corral et al., 2014b). Thus, we recently demonstrated the efficacy of applying IEHH programs (passive exposure only or combined with exercise protocols) in the recovery of a range of injuries (Corral et al., 2014a; Núñez-Espinosa et al., 2014; Rizo-Roca et al., 2017a,b). These results are consistent with the new paradigm that proposes biphasic effects in the response to hypoxia (hormesis); that is, its harmful or beneficial effects depend on the frequency and severity of the hypoxic challenge to the organism or tissue in question (Navarrete-Opazo and Mitchell, 2014).
Biological Effects of Intermittent Hypoxia Exposure
The molecular mechanisms involved in the response to hypoxia at the cellular level are relatively well understood (Fábián et al., 2016; Bhattarai et al., 2017; Koyasu et al., 2017). However, the complexity of the interactions between the divergent signaling pathways and the time frame of the various processes on different tissues and organs, together with the significant individual variability in humans' susceptibility to hypoxia (Rathat et al., 1992), pose formidable challenges to researching the potential benefits of regular programs involving exposure to real or simulated altitudes. This paper presents a series of examples of how intermittent exposure to hypoxia can benefit certain patients, although it is not intended to be an exhaustive list. Obviously, these programs must always be applied with due caution and under rigorous clinical controls, as with any pharmacological treatment.
Erythropoiesis
Table 1 lists several representative studies of the favorable effects of intermittent hypoxia exposure by increasing erythropoiesis. A detailed report on the rate of erythropoietin (EPO) formation and plasma lifetime in humans in response to acute hypobaric hypoxia exposure in a hypobaric chamber was first provided by the group led by Christian Bauer in Zurich (Eckardt et al., 1989). Later, the pivotal role of hypoxia-inducible factor 1 (HIF-1) in the transcriptional response of EPO to hypoxia was also described (Wang and Semenza, 1993). These seminal works and many subsequent studies sparked an interest in applying IEHH programs to increase erythrocyte mass as a way of improving the aerobic capacity of athletes. Recombinant human erythropoietin (rHuEPO) opened up a new chapter in the correction of uremic anemia due to chronic renal failure (Egrie et al., 1986; London et al., 1989; Najean et al., 1989), but also led to unethical use in sports medicine. The celebration of the 1968 Summer Olympics held in Mexico City (2,250 m above sea level) aroused interest in studying the effects of altitude on human performance. The reduced performance in sporting events with a high aerobic component was evident, while participants in competitions with a clear anaerobic character saw no decline in their performance, and some even beat their records (Di Prampero et al., 1970). As a consequence, stays at high altitude and other artificial hypoxia exposure strategies in athletes were subject to intense study; a wide range of strategies, from permanent stays at moderate or high geographic altitudes (Antezana et al., 1994; Richalet et al., 1994) to different patterns of intermittent exposure (Levine and Stray-Gundersen, 1997; Chapman et al., 1998; Rodríguez et al., 1999; Casas M. et al., 2000; Karlsen et al., 2001; Stray-Gundersen et al., 2001; Ge et al., 2002; Lundby et al., 2007; Richalet and Gore, 2008) were examined. Noticeable differences in protocols and hypoxia exposure methods led to intense debate on the usefulness of intermittent hypoxia exposure for elite athletes, given that hypoxic dose and interindividual variability represent two of the main constraints (Chapman et al., 1998; Casas H. et al., 2000; Julian et al., 2004; Gore et al., 2006; Wilber et al., 2007; Truijens et al., 2008; Rodríguez et al., 2015). In parallel, there was rising interest in understanding the non-erythropoietic effects of EPO. The discovery of multi-tissue erythropoietin receptor expression provided an insight into erythropoietin (EPO) activity that went beyond its role in the regulation of red blood cell production, including a key role in cardioprotection, brain development and neuroprotection, through a coordinated response against tissue oxygen shortage (Noguchi et al., 2007; Arcasoy, 2008; Burger et al., 2009; Chateauvieux et al., 2011; Jia et al., 2012; Zhang Y. et al., 2014b), thus contributing to ischemic preconditioning, an interesting and important property of organ survival that could also prove very useful for new biomedical applications.
Angiogenesis and Muscle Capillarization
In Table 2, some examples of the effects of intermittent hypoxia exposure on angiogenesis, vascular remodeling, muscle capillarization and hypertension are presented. In addition to its erythropoietic role, HIF-1 is the main mediator of angiogenesis in response to hypoxic conditions (Rey and Semenza, 2010) and has been considered a potential therapeutic target in many diseases. Two different strategies have been applied: HIF-1 upregulation for ischemic diseases (Li et al., 2014) and HIF-1 inhibition for cancer and endometriosis (Zhou et al., 2012; Bhattarai et al., 2017). Multiple angiogenic factors have been tested in the past; however, therapies that use only one proangiogenic agent to elicit angiogenesis were shown to be insufficient (Hirota and Semenza, 2006). Therefore, the addition of non-pharmacological treatments based on hypoxia-induced angiogenesis may be a successful strategy (Zimna and Kurpisz, 2015).
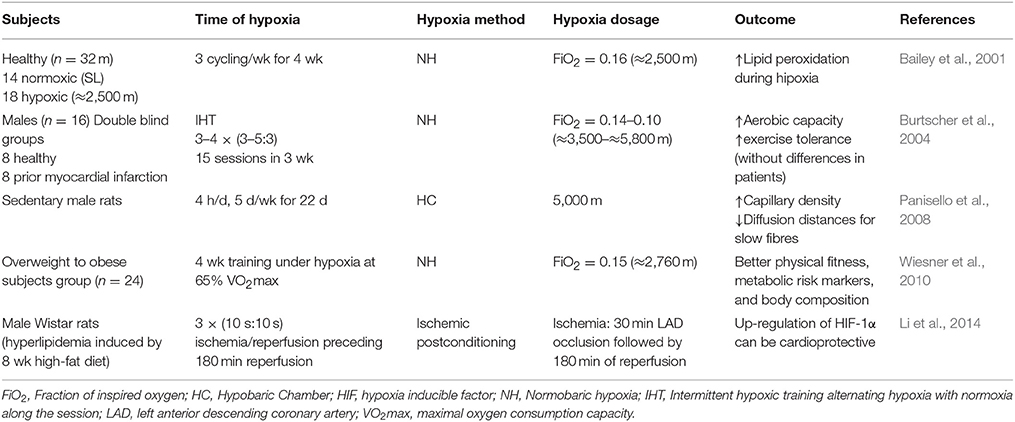
Table 2. Examples of the effects of intermittent hypoxia exposure on angiogenesis, vascular remodeling, muscle capillarization, and hypertension.
The physiological response elicited by hypoxia at moderate altitude exposure (1,800–3,000 m) is low, but increases when combined with exercise, with additional specific responses that are not observed when similar exercise levels are carried out in normoxia (Bärtsch et al., 2008). Moreover, even greater adaptations are obtained when the hypoxic intervention is accompanied by high-intensity training (Faiss et al., 2013; Sanchez and Borrani, 2018).
Although altitude is generally associated with increased health risks in most patients and elderly individuals, several studies have reported therapeutic benefits associated with exercising in mild hypoxia in a variety of alterations (Bailey et al., 2001; Burtscher et al., 2004; Wiesner et al., 2010). As exercising in moderate hypoxia seems to play a valuable role as an additional “therapeutic strategy,” albeit one with both benefits and risks, new insights on this paradigm are now increasing. Some critical analysis and guidelines for hypertensive, obese and elderly individuals have recently been proposed (Millet et al., 2016). These concluded that intermittent hypoxic training seems to be well tolerated by most patients, in a similar way to healthy individuals, and that hypoxia and exercise may have additive or synergistic effects, probably mediated by several factors, including nitric oxide, angiogenesis and “altitude anorexia,” thereby paving the way for researchers to identify the optimal individual combination of exercise and hypoxia.
Cardiac Remodeling
Aerobic exercise activities have traditionally been widely recommended for preventing disease and promoting health. Today, resistance training is usually included in physical activity counseling, even for older adults and people with a range of cardiac conditions (Haskell et al., 2007; Nelson et al., 2007; Heuschmann et al., 2010), and there is solid evidence of different echocardiographic repercussions (Kenney et al., 2012). The cardioprotective effects of chronic intermittent hypoxia have been extensively studied, and their positive effect has shown to be related to preservation of mitochondrial function and inhibition of potassium channels sensitive to ATP (mitoKATP) present in the sarcoplasmic and mitochondrial membranes (Ostádal et al., 1989; Asemu et al., 1999, 2000; Chouabe et al., 2004; Ostadal and Kolar, 2007). Additional myocardial remodeling data were reported by our group using a model of IEHH (Panisello et al., 2007). Both chronic and intermittent exposure models supported the potential beneficial effects of acute exposure in coronary patients reported in pioneering studies conducted by Peruvian cardiologist Emilio Marticorena (Marticorena, 1993; Marticorena et al., 2001; Reynafarje and Marticorena, 2002; Valle et al., 2006). In rodents, it has been demonstrated that endurance exercise training and IEHH modulate cardiac mitochondria to a protective phenotype characterized by decreased induction of mitochondrial permeability transition pore and apoptotic signaling (Magalhães et al., 2013, 2014). However, there are no studies on humans on cardiac remodeling that combine hypoxia and exercise, other than those dealing with OSA models. There have been extensive studies on patients suffering from this syndrome, and several pieces of evidence may be found in the literature: the progression and reversibility of atrial remodeling following stretch release may help prevent atrial fibrillation (Thanigaimani et al., 2017), the important prognostic information of right-sided heart dysfunction (Kusunose et al., 2016) and the evidence that OSA is associated independently with decreasing left ventricular systolic function and reduced right ventricular function (Korcarz et al., 2016). Nevertheless, this cardiac remodeling in OSA patients –individuals characterized by sustained systemic acidosis, hypercapnia and cerebral vasodilation– might not be present during intermittent hypoxia exposure in healthy subjects, in which alkalosis and hypocapnia, both induced by the hyperventilation caused by adrenergic drive, are evident and probably lead to cerebral vascular constriction and reduced effects of hypoxic insult (Viscor et al., 2014). Consequently, some of the changes and/or adaptive responses found in these pathological conditions must be interpreted with caution. In conclusion, there is no solid evidence for pernicious cardiac remodeling, but rather the opposite, after intermittent hypoxia in healthy individuals, whether accompanied by physical exercise or not. Table 3 contrasts the deteriorated cardiac function in OSA patients in comparison to several studies demonstrating, both in experimental animal models and human coronary patients, the positive effects of intermittent hypoxia exposure on cardiac function.
Treatment of Bronchial Asthma
Despite modern advances in the treatments, bronchial asthma continues being a potentially severe illness. All treatments focus on the improvement of bronchial obstruction, but nowadays we do not have an etiological definitive treatment. Bronchial asthma generates great dependence on a variety of medications and therefore frequently submits the patient to derived complications (Chiu et al., 1981; Fairfax et al., 1999; van der Woude et al., 2001; Salpeter et al., 2006). The Experts Committee of the United States Food & Drug Administration published a consensus document about the risks of the antiasthma medications, some of them potentially lethal (DeNoon, 2008).
For that reason, every procedure that could diminish pharmacological dependence among asthmatic patients should be considered a benefit. IEHH programs represent a realistic possibility to apply a minimally aggressive non-pharmacological approach that would reduce bronchial obstruction and pharmacological dependence in these patients.
As early as the nineteenth century there was social wisdom and medical knowledge that respiratory illnesses may improve in the mountains. The sanatoriums for respiratory patients, traditionally, were located at moderate altitude in the mountains. Notable examples were the Dutch Asthma Center, Davos, Clavadel, at 1,686 m over the sea level (Switzerland) and the Istituto Pio XII, Misurina, Auronzo at 1,756 m (Italy) devoted to childhood bronchial asthma. The first medical reference we found about bronchial asthma and altitude is an inquiry between the doctors of Davos referring that 133 among their 143 patients with bronchial asthma that spent their holidays in this mountain town, did not present any acute episode of asthma and that 81% reported persistent improvement of the illness (Turban and Spengler, 1906).
Moreover, different epidemiological studies showed the beneficial effects of living at moderate altitude in the prevalence and severity of bronchial asthma (van Velzen et al., 1996; Yangzong et al., 2006; Droma et al., 2007; Kiechl-Kohlendorfer et al., 2007; Sy et al., 2007). However, acute hypoxia exposure, as occurs with acute altitude exposure, as in many other stress situations, can induce an asthmatic episode of bronchoconstriction. On the other hand, when the acclimatization process advances, the asthmatic illness improved or even disappeared (Allegra et al., 1995; Christie et al., 1995; Cogo et al., 1997, 2004; Gourgoulianis et al., 2001; Karagiannidis et al., 2006; Schultze-Werninghaus, 2006, 2008). Regrettably, this improvement vanished upon returning to the sea level.
The triad altitude exposure-hypoxia-acclimatization produces a number of physiological changes, some of which are accepted as related to the improvement of bronchial asthma: (a) a different breathing control pattern (Harrison et al., 2002; Serebrovskaya et al., 2003), (b) mitochondrial changes that optimize oxygen metabolism during the normal acclimatization process (Levett et al., 2012), and (c) the decrease in free radicals and the associated anti-inflammatory and immunosuppressive effects (Meehan, 1987; Simon et al., 1994; Serebrovskaya et al., 2003; Ohta et al., 2011; Oliver et al., 2013).
Since bronchial asthma improves with acclimatization to altitude and IEHH stimulates the acclimatization process (Rodríguez et al., 1999, 2000; Casas H. et al., 2000; Casas M. et al., 2000; Ibáñez et al., 2000; Ricart et al., 2000), it could be hypothesized that IEHH improves bronchial asthma. In fact, some medical studies have shown the usefulness of intermittent hypoxia exposure as a treatment for bronchial asthma (Harrison et al., 2002; Serebrovskaya et al., 2003). However, due to the different techniques and protocols used to produce the hypoxia and the wide dispersion of data, further research is required to design more effective protocols for intermittent hypoxia exposure that could prove useful in treating this disease. The ultimate objective of such treatments must be to reduce bronchial obstruction and the dependence on potentially dangerous drugs. Moreover, if protocols demonstrate a good response in bronchial asthma mitigation, they could also be useful for treating other illnesses with inflammatory backdrop. Table 4 summarizes some of the results that demonstrate a favorable effect of exposure to hypoxia on the symptoms of bronchial asthma.
Neurological Impact of Hypoxic Exposure
Hypobaric hypoxic exposure at altitude, usually long-term, results in several pathophysiological and psychological conditions associated with the nervous system. The term high altitude deterioration (HAD) was first used by members of early Mount Everest expeditions to denote the deterioration in mental and physical condition due to prolonged time spent at high altitudes (Ward, 1954). It is well known among climbers that staying at extreme altitudes for long periods is deleterious (Milledge, 2003). Manifestations vary depending on the altitude reached and the individual's hypoxia tolerance, but include acute and chronic mountain sickness, memory loss and high-altitude cerebral edema (Lieberman et al., 1994; Hornbein, 2001; West et al., 2013). Acute mountain sickness generally occurs 6 to 12 h after an unacclimatized person ascends to 2,500 m or higher (Bärtsch and Swenson, 2013). As a result, cognitive function may be impaired under hypoxia (Virués-Ortega et al., 2006), although the physiological changes that occur during acclimatization prevent mountain sickness. Given the acclimatization-like responses triggered by intermittent hypoxic exposure, it offers protection against severe hypoxia exposure damage and has been reported to produce beneficial effects (Kushwah et al., 2016). Our group reported how short-term (3-h sessions on three consecutive days) IEHH with surface muscle electrostimulation increased the concentration of circulating progenitor cells in the peripheral blood of humans (Viscor et al., 2009). However, we were unable to reproduce these results later in healthy patients and those with traumatic brain injuries (Corral et al., 2014a,b), thus raising doubts about the potential role of hypoxia exposure in the release of stem cells to circulation and its involvement in the tissue regeneration process. In any case, the translation of the physiological effects of IEHH to humans is not straightforward in the field of neurology.
The brain's protective mechanisms involved in intermittent exposure to hypoxia have been widely studied using experimental animal models, and numerous beneficial effects have been reported. Intermittent hypoxia facilitates the proliferation of neural stem cells in situ in the subventricular zone and dentate gyrus of rat brains (Zhu et al., 2005; Ross et al., 2012). Xu et al. (2007) described a time-dependent migration of neural progenitor cells (NPC), promoted by hypoxia-induced astrocytes, thereby suggesting a role for astrocytes in NPC replacement therapy in the central nervous system. Intermittent hypoxia stimulated hippocampal angiogenesis and neurogenesis and improved short-term memory indices in control mice; and, in brain-injured mice, it reduced injury size and prevented memory impairments (Bouslama et al., 2015). It was recently reported that activation of HIF-1 is involved in hyperglycemia-aggravated blood-brain barrier disruption in an ischemic stroke model (Zhang et al., 2016b). Moreover, glycemic control by insulin abolished HIF-1α upregulation in diabetic animals and reduced blood-brain barrier permeability and brain infarction (Zhang et al., 2016b). Acute intermittent hypoxia can trigger spinal plasticity associated with sustained increases in respiratory, somatic and/or autonomic motor output (Streeter et al., 2017).
In rats, intermittent hypobaric hypoxia preconditioning caused a reduction in the degree of brain injury following ischemia-reperfusion by reducing hippocampal neuronal apoptosis by local upregulation of neuroglobin and Bcl-2 expression (Wu et al., 2015). Neuroglobin is an intracellular monomer hemoprotein that was discovered by Burmester et al. (2000) and is expressed in the central and peripheral nervous system, cerebrospinal fluid, retina and some endocrine areas of the brain (Burmester and Hankeln, 2004). It reversibly binds oxygen with a higher affinity than normal adult hemoglobin, and plays a critical role in brain tissue protection facing a possible oxygen delivery shortage (Ascenzi et al., 2016). Bcl-2 is an anti-apoptotic protein localized in the outer membrane of the mitochondria; overexpression of Bcl-2 in neurons can inhibit neuron apoptosis induced by ischemia-reperfusion injury by maintaining the integrity of mitochondria (Xing et al., 2008; Zhang et al., 2008).
Kushwah et al. (2016) also explored the ameliorating potential of intermittent hypoxia against the detrimental effects of unpredictable chronic mild stress (UCMS) on anxiety and depression-like behavior in rats, through the enhancement of neurogenesis in the hippocampus, a response mediated by brain derived neurotrophic factor (BDNF). In the postischemic rat brain, intermittent hypoxia intervention rescued ischemia-induced spatial learning and memory impairment by inducing hippocampal neurogenesis and functional synaptogenesis via BDNF expression (Tsai et al., 2013).
Nowadays, intermittent hypoxia exposure is known to enhance neurogenesis at multiple stages. Notch1 is a transcription factor in the neuron's membrane that regulates several stages of neurogenesis and promotes differentiation of progenitor cells into astroglia. Notch1 is activated by hypoxia in vivo, and such activation has been shown to be required for hypoxia-induced neurogenesis (Zhang K. et al., 2014a). Chronic IEHH pretreatment can reduce cerebral ischemic injury, which, as similarly reported for myocardium (see above), is mediated through upregulation of the expression and activity of mitochondrial membrane ATP-sensitive potassium channel (mitoKATP) (Zhang et al., 2016a). As is well known, hypoxia inducible factor-1 (HIF-1) is the key transcription factor that controls early adaptive responses to the lack of oxygen in mammalian cells. HIF-1α and HIF-1β expression was measured during acclimatization to hypobaric hypoxia in the rat cerebral cortex, and neurons, astrocytes, ependymal cells and possibly endothelial cells were the cell types that expressed HIF-1α (Chávez et al., 2000). Thus, the vascular remodeling and metabolic changes triggered during prolonged hypoxia may restore normal oxygen delivery levels to brain tissue (Agani et al., 2002; Chavez and LaManna, 2002).
Finally, there is solid evidence of the beneficial effects of intermittent hypoxia exposure on spinal cord neural tissue repair. Complete or incomplete spinal cord injuries are characterized by spared synaptic pathways below the level of the injury. Intermittent hypoxia elicits plasticity in the spinal cord and strengthens these spared synaptic pathways, expressed as respiratory and somatic functional recovery in both experimental animals and humans with traumatic spinal cord injury (Navarrete-Opazo et al., 2015, 2017a,b; Dougherty et al., 2017; Trumbower et al., 2017). Table 5 lists studies reporting beneficial neurological impact after a wide range of intermittent hypoxia exposure protocols.
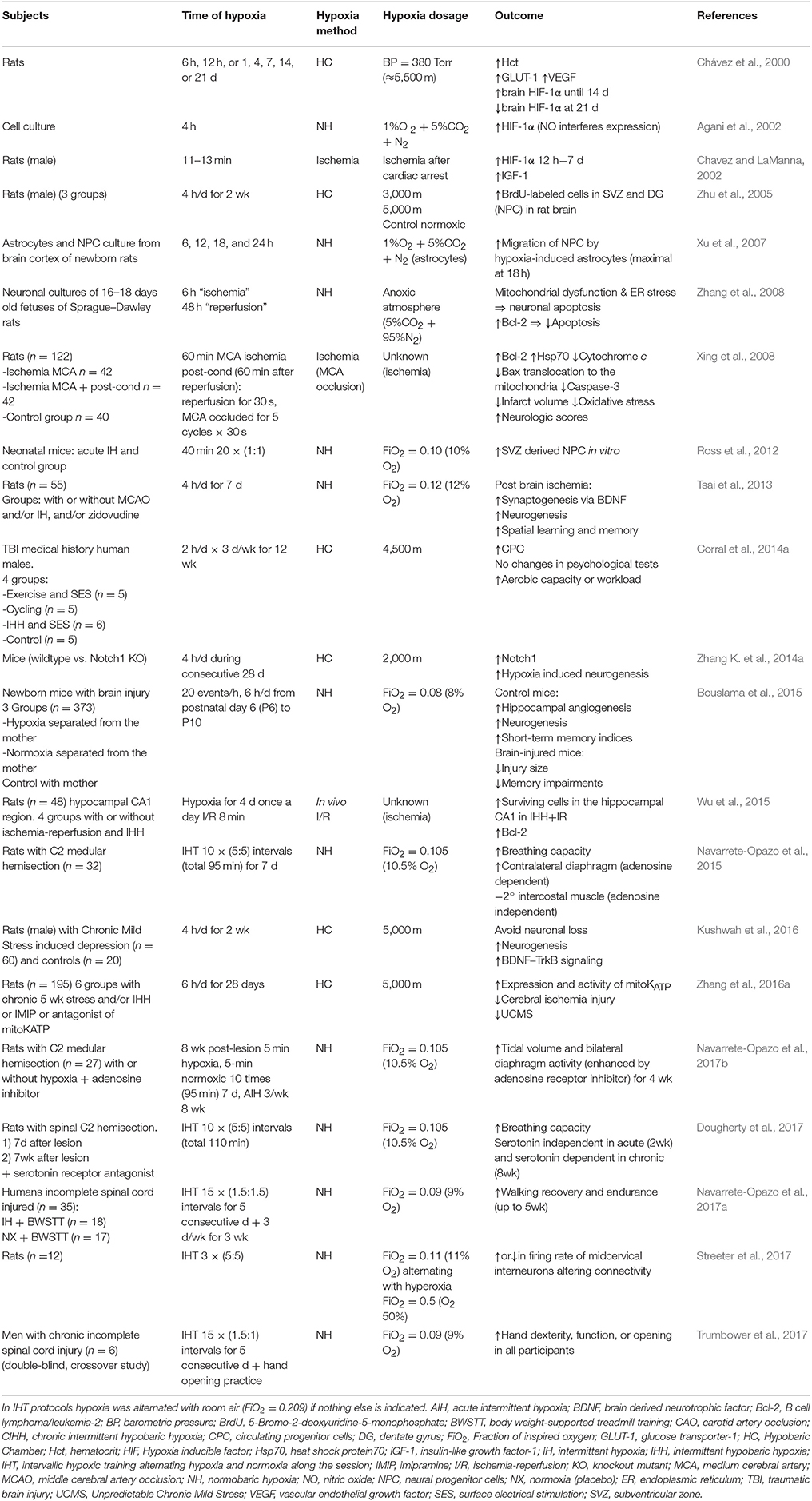
Table 5. Examples of the effects of intermittent hypoxia exposure with favorable neurological impact.
Other Pathological Conditions Where the Use of Intermittent Hypoxia Exposure Has Potential Therapeutic Value
Since the altitude-hypoxia-acclimatization triad is known to have some antioxidant, anti-inflammatory and immunosuppressive effects (Meehan, 1987; Meehan et al., 1988; Ohta et al., 2011; Oliver et al., 2013), a benefit in some other pathologies related to immune response, such as psoriasis, atopy, arthritis or autoimmune pneumonitis can be expected. This is still a controversial field with no extensive clinical studies available, although some medical descriptive studies point to potential future research opportunities (Singh et al., 1977; Vocks et al., 1999; Engst and Vocks, 2000; Steiner, 2009).
Intermittent exposure to both normobaric and hypobaric hypoxia has been related to some protective effects (Cai et al., 2003; Costa et al., 2013) and beneficial outcomes in several pathological conditions, especially in those related to metabolic syndrome (Marquez et al., 2013; Leone and Lalande, 2017; Serebrovska et al., 2017). The possible role of intermittent hypoxia on body weight control has also attracted considerable attention. In addition to improving exercise performance and diet control, intermittent exposure protocols to normobaric and hypobaric hypoxia have been applied in an attempt to potentiate weight loss, showing in some cases positive short-term results (Haufe et al., 2008; Netzer et al., 2008; Lippl et al., 2010; Wiesner et al., 2010; Cabrera-Aguilera et al., in press). However, a long-term study failed to demonstrate permanent body weight reduction after IEHH (Gatterer et al., 2015), suggesting that additional research is needed to clarify the discrepancies reported in this field.
In summary, recent reports call for increased attention to the potential benefits of the application of intermittent hypoxia protocols in several clinical areas (Dale et al., 2014; Mateika et al., 2015). It is likely that future studies will yield important new information regarding potential therapeutic uses of intermittent hypoxia. Table 6 lists a non-exhaustive but representative sample of studies reporting favorable impact of intermittent hypoxia exposure in other pathological conditions.
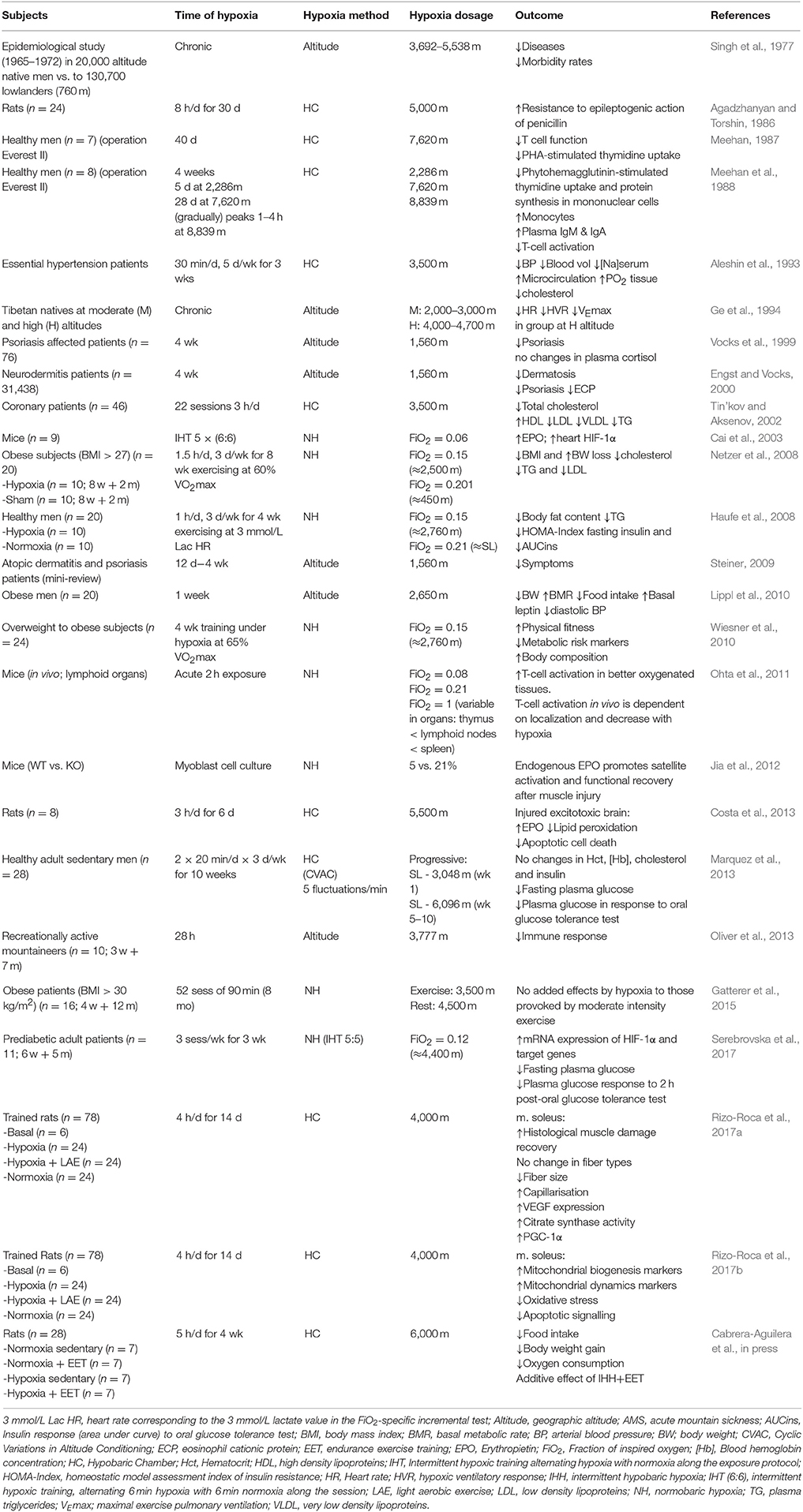
Table 6. Examples of the effects of intermittent hypoxia exposure with favorable impact in other pathological conditions.
Intermittent Hypoxia in Sport
The benefits of intermittent hypoxia programs in competitive sport are still a subject of scientific debate. Table 7 shows several examples of intermittent hypoxia exposure effect on the improvement of human physical performance. A prior consideration to bear in mind when dealing with this topic is that two inherent factors justify the diversity of results in the field of elite sport: (a) the narrow margin of improvement detectable in elite athletes; and (b) the limitation in the sample size when performing studies with this population. Both factors contribute to reduced statistical power in most of these studies.
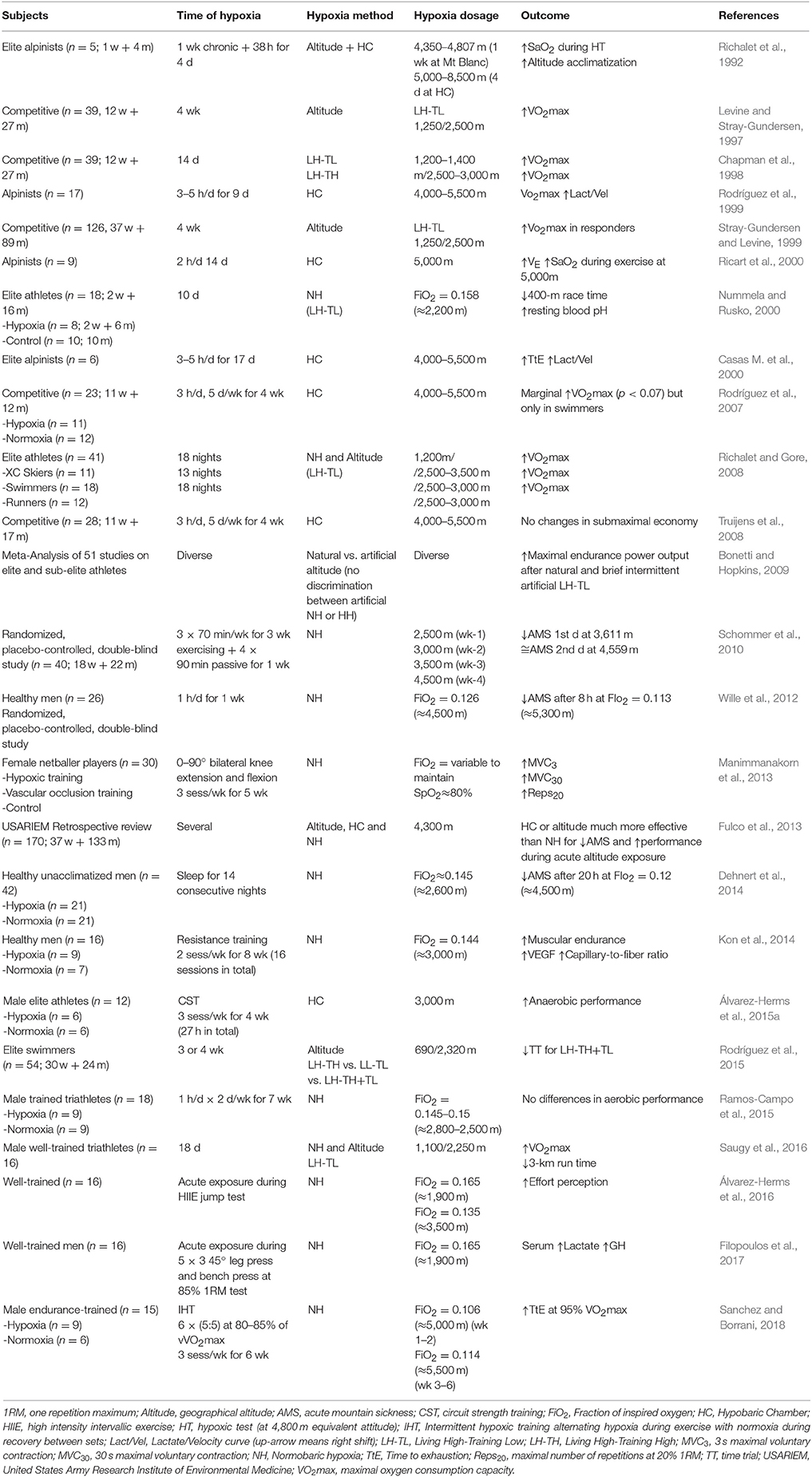
Table 7. Examples of the intermittent hypoxia exposure on the improvement of human physical performance.
Other sources of variability are the wide range of exposure patterns, differing hypoxic doses (or altitude), and the kind of hypoxia (hypobaric or normobaric), as is discussed below. The living high-training low (LH-TL) pattern (Levine and Stray-Gundersen, 1997) is the most widespread training schedule, although sometimes the reverse model, living low-training-high (LL-TH), is also applied, especially when artificial hypoxia is used. Moreover, studies evaluating training at altitude during permanent stays are also very usual.
In addition to other reports cited in precedent sections, a high number of studies have consistently found positive effects of IEHH programs to improve exercise performance. Thus, 4 weeks of LH-TL improved sea-level running performance in trained runners (Levine and Stray-Gundersen, 1997). Short-term intermittent hypobaric hypoxia (in a hypobaric chamber) improved the aerobic performance capacity in healthy subjects (Rodríguez et al., 1999). Intermittent hypobaric hypoxia combined with low-intensity exercise induced altitude acclimation, improved lactate threshold and ventilatory anaerobic threshold in healthy subjects (Casas M. et al., 2000). Normobaric hypoxia increased the growth hormone response to maximal resistance exercise in trained men (Filopoulos et al., 2017). Finally, a reduction in the severity of acute mountain sickness was also reported after several intermittent normobaric hypoxia protocols (Schommer et al., 2010; Wille et al., 2012; Dehnert et al., 2014).
In contrast, other studies did not detect significant improvements in exercise performance. Four weeks of IEHH did not improve oxygen transport in trained swimmers and runners (Rodríguez et al., 2007) nor did it change the submaximal economy in a group of well-trained athletes (Truijens et al., 2008). Seven weeks of normobaric hypoxia training in triathletes, caused an improvement in hematological parameters but not in the aerobic performance (Ramos-Campo et al., 2015). Finally, a recent systematic review and meta-analysis did not reveal a significant benefit of resistance training in hypoxia compared to the same training in normoxia (Ramos-Campo et al., 2018).
In general, it is commonly accepted that the application of intermittent hypoxia exposure has beneficial effects for competitive sport in the same way as for the biomedical field. As discussed above, the wide difference in effects that have been reported in the literature can be explained by individual susceptibility and the diversity of intermittent hypoxia patterns applied (Debevec and Mekljavic, 2013). The adaptive or maladaptive responses can be due to differences in the frequency, severity or duration of hypoxic episodes (Serebrovska et al., 2016). Factors such as age, sex or genotypic variability may also contribute to varying results (Almendros et al., 2014). A review of relevant publications between 1980 and 2015 concluded that evidence regarding the effects of altitude training on athletic performance is weak but that the natural stay at altitude combined with a live high-train low training strategy may provide the best protocol for enhancing endurance performance in elite and subelite athletes (Khodaee et al., 2016), thus confirming similar findings in a previous study (Bonetti and Hopkins, 2009). Finally, a recently published meta-analysis on the effect of natural or simulated altitude training in team-sport athletes conclude that hypoxic intervention appears to be a worthwhile training strategy for improvement in team-sport athletes, with enhanced performance over control groups persisting for at least 4 weeks post-intervention (Hamlin et al., 2018). Also, our recent data indicate that contractile activity seems to be necessary to trigger in skeletal muscle the adaptive responses induced by intermittent exposure to hypoxia (Rizo-Roca et al., 2018).
Although most of the expected effects of altitude training and IEHH programs have been mainly aimed at improving aerobic capacity (Stray-Gundersen and Levine, 1999; Wilber, 2004), some reports also have described benefits for strength training at moderate hypoxia levels (Nummela and Rusko, 2000; Manimmanakorn et al., 2013; Álvarez-Herms et al., 2015b, 2016). The underlying mechanism of these responses remain to be clarified, but potential psychological benefits may be derived of the perception of increased effort during hypoxic training (Álvarez-Herms et al., 2016). In addition, training during hypoxia may result in a greater increase in muscular endurance than the same training load performed in normoxia, probably because of increased angiogenesis in skeletal muscle level (Kon et al., 2014), can be involved in those improvements.
Normobaric vs. Hypobaric Hypoxia: the Same Stimulus?
When artificial methods of producing hypoxia exposure were first developed, no attention was paid to the differences between hypobaric (low barometric pressure) and normobaric (low oxygen content in an inhaled gas mixture) hypoxia. It seems evident that the same physiological effects are expected for a determined alveolar oxygen partial pressure, regardless of the technical means used. However, data in the literature present consistent discrepancies after applying hypobaric and normobaric hypoxia, which could be attributed to alterations in other environmental parameters that affect alveolar gas composition, such as carbon dioxide partial pressure, humidity and temperature. For instance, even for the same level of oxygen partial pressure, the atmospheric composition in a small hypoxic tent with low air turnover (limited by the flow capacity of the hypoxic device) can be very different from the air composition in a hypobaric chamber. Generally, the use of powerful vacuum pumps in hypobaric chamber systems guarantees sufficient renewal of the air inside the room, thus preventing carbon dioxide accumulation and a rise in air temperature and humidity, factors that could become unbalanced in small volume hypoxic tents, especially with exercising subjects inside. In a comparative study of hypobaric (low pressure chamber) and normobaric hypoxia (hypoxic tent) during a submaximal exercise test in the same subjects, differences were observed in some cardiorespiratory and heart rate variability parameters between the two artificial hypoxia systems used (Basualto-Alarcón et al., 2012).
An interesting and dynamic debate, which is beyond the scope of this review, is currently under way among altitude researchers concerning whether or not hypobaric hypoxia induces different responses from normobaric hypoxia (Girard, 2012; Millet et al., 2012; Hauser et al., 2016; Saugy et al., 2016). Some evidences demonstrate that there are different physiological responses and outcomes between exposure to normobaric and hypobaric hypoxia conditions (Savourey et al., 2003; Fulco et al., 2013; Millet et al., 2013; Beidleman et al., 2014; Debevec and Millet, 2014; Boos et al., 2016; DiPasquale, 2017). For instance, it has been reported that the decrease in air density that accompanies the partial pressure drop of oxygen at geographic altitude affects the way in which explosive actions are executed and increases movement velocity and power during force-velocity bench presses in comparison to normobaric hypoxia (Feriche et al., 2014).
Conclusions
A growing body of knowledge supports the beneficial effects of natural or simulated altitude techniques on health outcomes (Navarrete-Opazo and Mitchell, 2014; Millet et al., 2016; Lizamore and Hamlin, 2017). Future research should be oriented to: (1) gain more in-depth knowledge of the subcellular mechanisms involved in the hypoxic response at different tissue levels, (2) standardize hypoxia exposure methods and establish a universal method for measuring, and repeatedly applying, hypoxic dosage, (3) improve predictions of individual hypoxia tolerance to prevent possible negative consequences, (4) apply this new knowledge to the selection and education of altitude workers, (5) improve altitude acclimatization, altitude training camps and altitude competition events to benefit mountaineers, athletes and coaches, and finally (6) cautiously explore the application of IEHH in pathological conditions.
Author Contributions
GV, JV, AR, and LC contributed to the initial draft of the manuscript; GV and JT edited the document after contributions from all authors; All authors reviewed and approved the final version.
Conflict of Interest Statement
The authors declare that the research was conducted in the absence of any commercial or financial relationships that could be construed as a potential conflict of interest.
Acknowledgments
This study was supported in part by research grants DEP2010-22205-C02-01, DEP2010-2205-C02-02, DEP2013 48334-C2-1P, and DEP2013-48334-C2-2-P from the Plan Nacional de I+D+i (Spain's Ministry of Economy, Industry and Competitiveness). The authors thank Christopher Evans and Paula Weiss (Language Advisory Service, Universitat de Barcelona) for their help editing the manuscript.
References
Agadzhanyan, N. A., and Torshin, V. I. (1986). Effect of adaptation to hypoxia on resistance of rats to the epileptogenic action of penicillin. Bull. Exp. Biol. Med. 102, 1669–1671. doi: 10.1007/BF00840791
Agani, F. H., Puchowicz, M., Chavez, J. C., Pichiule, P., and LaManna, J. (2002). Role of nitric oxide in the regulation of HIF-1α expression during hypoxia. Am. J. Physiol. Cell Physiol. 283, C178–C186. doi: 10.1152/ajpcell.00381.2001
Aleshin, I. A., Kots, I. I., Tverdokhlib, V. P., Galiautdinov, G. S., Vdovenko, L. G., Zabirov, M. R., et al. (1993). [The nondrug treatment of hypertension patients by their adaptation to periodic hypoxia in a barochamber]. Ter. Arkh. 65, 23–29.
Allegra, L., Cogo, A., Legnani, D., Diano, P. L., Fasano, V., and Negretto, G. G. (1995). High altitude exposure reduces bronchial responsiveness to hypo-osmolar aerosol in lowland asthmatics. Eur. Respir. J. 8, 1842–1846. doi: 10.1183/09031936.95.08111842
Almendros, I., Wang, Y., and Gozal, D. (2014). The polymorphic and contradictory aspects of intermittent hypoxia. Am. J. Physiol. Lung Cell. Mol. Physiol. 307, L129–L140. doi: 10.1152/ajplung.00089.2014
Álvarez-Herms, J., Julià-Sánchez, S., Corbi, F., Pagès, T., and Viscor, G. (2015a). A program of circuit resistance training under hypobaric hypoxia conditions improves the anaerobic performance of athletes. Sci. Sports 31, 78–87. doi: 10.1016/j.scispo.2015.08.005
Álvarez-Herms, J., Julià-Sánchez, S., Gatterer, H., Blank, C., Corbi, F., Pagès, T., et al. (2016). Anaerobic training in hypoxia: A new approach to stimulate the rating of effort perception. Physiol. Behav. 163, 37–42. doi: 10.1016/j.physbeh.2016.04.035
Álvarez-Herms, J., Julià-Sánchez, S., Hamlin, M., and Viscor, G. (2015b). Strength training under hypoxic conditions. Physiol. Rep. 3:e12227. doi: 10.14814/phy2.12227
Antezana, A. M., Kacimi, R., Le Trong, J. L., Marchal, M., Abousahl, I., Dubray, C., et al. (1994). Adrenergic status of humans during prolonged exposure to the altitude of 6,542 m. J. Appl. Physiol. 76, 1055–1059. doi: 10.1152/jappl.1994.76.3.1055
Arcasoy, M. O. (2008). The non-haematopoietic biological effects of erythropoietin. Br. J. Haematol. 141, 14–31. doi: 10.1111/j.1365-2141.2008.07014.x
Ascenzi, P., di Masi, A., Leboffe, L., Fiocchetti, M., Nuzzo, M. T., Brunori, M., et al. (2016). Neuroglobin: From structure to function in health and disease. Mol. Aspects Med. 52, 1–48. doi: 10.1016/j.mam.2016.10.004
Asemu, G., Neckár, J., Szárszoi, O., Papousek, F., Ostádal, B., and Kolar, F. (2000). Effects of adaptation to intermittent high altitude hypoxia on ischemic ventricular arrhythmias in rats. Physiol. Res. 49, 597–606. Available online at: http://www.biomed.cas.cz/physiolres/pdf/49/49_597.pdf
Asemu, G., Papousek, F., Ostádal, B., and Kolár, F. (1999). Adaptation to high altitude hypoxia protects the rat heart against ischemia-induced arrhythmias. Involvement of mitochondrial K(ATP) channel. J. Mol. Cell. Cardiol. 31, 1821–1831. doi: 10.1006/jmcc.1999.1013
Bailey, D. M., Davies, B., and Young, I. S. (2001). Intermittent hypoxic training: implications for lipid peroxidation induced by acute normoxic exercise in active men. Clin. Sci. 101, 465–475. doi: 10.1042/cs1010465
Bärtsch, P., and Swenson, E. R. (2013). Clinical practice: acute high-altitude illnesses. N. Engl. J. Med. 368, 2294–2302. doi: 10.1056/NEJMcp1214870
Bärtsch, P., Dehnert, C., Friedmann-Bette, B., and Tadibi, V. (2008). Intermittent hypoxia at rest for improvement of athletic performance. Scand. J. Med. Sci. Sports 18(Suppl. 1), 50–56. doi: 10.1111/j.1600-0838.2008.00832.x
Basualto-Alarcón, C., Rodas, G., Galilea, P. A., Riera, J., Pagés, T., Ricart, A., et al. (2012). Cardiorespiratory parameters during submaximal exercise under acute exposure to normobaric and hypobaric hypoxia. Apunt. Med. l'Esport 47, 65–72. doi: 10.1016/j.apunts.2011.11.005
Beidleman, B. A., Fulco, C. S., Staab, J. E., Andrew, S. P., and Muza, S. R. (2014). Cycling performance decrement is greater in hypobaric versus normobaric hypoxia. Extrem. Physiol. Med. 3:8. doi: 10.1186/2046-7648-3-8
Bhattarai, D., Xu, X., and Lee, K. (2017). Hypoxia-inducible factor-1 (HIF-1) inhibitors from the last decade (2007 to 2016): A “structure-activity relationship” perspective. Med. Res. Rev. 38, 1–39. doi: 10.1002/med.21477
Bonetti, D. L., and Hopkins, W. G. (2009). Sea-level exercise performance following adaptation to hypoxia: a meta-analysis. Sports Med. 39, 107–127. doi: 10.2165/00007256-200939020-00002
Boos, C. J., O'Hara, J. P., Mellor, A., Hodkinson, P. D., Tsakirides, C., Reeve, N., et al. (2016). A four-way comparison of cardiac function with normobaric normoxia, normobaric hypoxia, hypobaric hypoxia and genuine high altitude. PLoS ONE 11:e0152868. doi: 10.1371/journal.pone.0152868
Bouslama, M., Adla-Biassette, H., Ramanantsoa, N., Bourgeois, T., Bollen, B., Brissaud, O., et al. (2015). Protective effects of intermittent hypoxia on brain and memory in a mouse model of apnea of prematurity. Front. Physiol. 6:313. doi: 10.3389/fphys.2015.00313
Burger, D., Xenocostas, A., and Feng, Q. P. (2009). Molecular basis of cardioprotection by erythropoietin. Curr. Mol. Pharmacol. 2, 56–69. doi: 10.2174/1874467210902010056
Burmester, T., and Hankeln, T. (2004). Neuroglobin: a respiratory protein of the nervous system. News Physiol. Sci. 19, 110–113. doi: 10.1152/nips.01513.2003
Burmester, T., Weich, B., Reinhardt, S., and Hankeln, T. (2000). A vertebrate globin expressed in the brain. Nature 407, 520–523. doi: 10.1038/35035093
Burtscher, M., Pachinger, O., Ehrenbourg, I., Günther, M., Faulhaber, M., Reinhard, P., et al. (2004). Intermittent hypoxia increases exercise tolerance in elderly men with and without coronary artery disease. Int J Cardiol. 96, 247–254. doi: 10.1016/j.ijcard.2003.07.021
Cabrera-Aguilera, I., Rizo-Roca, D., Marques, E., Santocildes, G., Pagès, T., Viscor, G, et al. (in press). Additive effects of intermittent hypobaric hypoxia endurance training on body weight, food intake, oxygen consumption in rats. High Alt. Med. Biol. doi: 10.1089/ham.2018.0013.
Cai, Z., Manalo, D. J., Wei, G., Rodriguez, E. R., Fox-Talbot, K., Lu, H., et al. (2003). Hearts from rodents exposed to intermittent hypoxia or erythropoietin are protected against ischemia-reperfusion injury. Circulation 108, 79–85. doi: 10.1161/01.CIR.0000078635.89229.8A
Canouï-Poitrine, F., Veerabudun, K., Larmignat, P., Letournel, M., Bastuji-Garin, S., and Richalet, J.-P. (2014). Risk prediction score for severe high altitude illness: a cohort study. PLoS ONE 9:e100642. doi: 10.1371/journal.pone.0100642
Casas, H., Casas, M., Ricart, A., Rama, R., Ibáñez, J., Palacios, L., et al. (2000). Efectiveness of three short intermittent hypobaric hypoxia protocols: hematological responses. J. Exerc. Physiol. 3, 38–45. Available online at: http://www.asep.org/asep/asep/PAGES.PDF
Casas, M., Casas, H., Pagés, T., Rama, R., Ricart, A., Ventura, J. L., et al. (2000). Intermittent hypobaric hypoxia induces altitude acclimation and improves the lactate threshold. Aviat. Space. Environ. Med. 71, 125–30.
Chapman, R. F., Stray-Gundersen, J., and Levine, B. D. (1998). Individual variation in response to altitude training. J. Appl. Physiol. 85, 1448–1456. doi: 10.1152/jappl.1998.85.4.1448
Chateauvieux, S., Grigorakaki, C., Morceau, F., Dicato, M., and Diederich, M. (2011). Erythropoietin, erythropoiesis and beyond. Biochem. Pharmacol. 82, 1291–1303. doi: 10.1016/j.bcp.2011.06.045
Chávez, J. C., Agani, F., Pichiule, P., and LaManna, J. C. (2000). Expression of hypoxia-inducible factor-1alpha in the brain of rats during chronic hypoxia. J. Appl. Physiol. 89, 1937–1942. doi: 10.1152/jappl.2000.89.5.1937
Chavez, J. C., and LaManna, J. C. (2002). Activation of hypoxia-inducible factor-1 in the rat cerebral cortex after transient global ischemia: potential role of insulin-like growth factor-1. J. Neurosci. 22, 8922–8931. doi: 10.1523/JNEUROSCI.22-20-08922.2002
Chiu, J. T., Wells, I., and Novey, H. S. (1981). Incidence of fungal precipitins in patients treated with beclomethasone dipropionate aerosol. Ann. Allergy 46, 137–9.
Chouabe, C., Ricci, E., Amsellem, J., Blaineau, S., Dalmaz, Y., Favier, R., et al. (2004). Effects of aging on the cardiac remodeling induced by chronic high-altitude hypoxia in rat. Am. J. Physiol. Heart Circ. Physiol. 287, H1246–H1253. doi: 10.1152/ajpheart.00199.2004
Christie, P. E., Yntema, J. L., Tagari, P., Ysselstijn, H., Ford-Hutchinson, A. W., and Lee, T. H. (1995). Effect of altitude on urinary leukotriene (LT) E4 excretion and airway responsiveness to histamine in children with atopic asthma. Eur. Respir. J. 8, 357–363. doi: 10.1183/09031936.95.08030357
Cogo, A., Basnyat, B., Legnani, D., and Allegra, L. (1997). Bronchial asthma and airway hyperresponsiveness at high altitude. Respiration. 64, 444–449. doi: 10.1159/000196721
Cogo, A., Fischer, R., and Schoene, R. (2004). Respiratory diseases and high altitude. High Alt. Med. Biol. 5, 435–444. doi: 10.1089/ham.2004.5.435
Corral, L., Conde, L., Guillam,ó, E., Blasi, J., Juncadella, M., Javierre, C., et al. (2014a). Circulating progenitor cells during exercise, muscle electro-stimulation and intermittent hypobaric hypoxia in patients with traumatic brain injury: a pilot study. NeuroRehabilitation 35, 763–769. doi: 10.3233/NRE-141172
Corral, L., Javierre, C., Blasi, J., Viscor, G., Ricart, A., and Ventura, J. L. (2014b). Combined intermittent hypobaric hypoxia and muscle electro-stimulation: a method to increase circulating progenitor cell concentration? J. Transl. Med. 12:174. doi: 10.1186/1479-5876-12-174
Costa, D. C., Alva, N., Trigueros, L., Gamez, A., Carbonell, T., and Rama, R. (2013). Intermittent hypobaric hypoxia induces neuroprotection in kainate-induced oxidative stress in rats. J. Mol. Neurosci. 50, 402–410. doi: 10.1007/s12031-012-9945-8
Dale, E. A., Ben Mabrouk, F., and Mitchell, G. S. (2014). Unexpected benefits of intermittent hypoxia: enhanced respiratory and nonrespiratory motor function. Physiology (Bethesda). 29, 39–48. doi: 10.1152/physiol.00012.2013
Debevec, T., and Mekljavic, I. B. (2013). Short intermittent hypoxia for improvement of athletic performance: reality or a placebo? / Kratkotrajna intermitentna hipoksija za izboljsanje sportne sposobnosti: realnost ali placebo? Kinesiol. Slov. 19, 5–28. Available online at: https://search.proquest.com/openview/22ce1795696590210cf7a98ba0253a14/1?
Debevec, T., and Millet, G. (2014). Discerning normobaric and hypobaric hypoxia: significance of exposure duration. J. Appl. Physiol. 116:1255. doi: 10.1152/japplphysiol.00319.2013.5
Dehnert, C., Böhm, A., Grigoriev, I., Menold, E., and Bärtsch, P. (2014). Sleeping in moderate hypoxia at home for prevention of acute mountain sickness (AMS): a placebo-controlled, randomized double-blind study. Wilderness Environ. Med. 25, 263–271. doi: 10.1016/j.wem.2014.04.004
DeNoon, D. J. (2008). FDA panel asks: are asthma drugs safe? Medscape WebMD, December 10th. Available online at: https://www.medscape.com/viewarticle/585129 [Accessed December 27, 2017]
Di Prampero, P. E., Piñera Limas, F., and Sassi, G. (1970). Maximal muscular power, aerobic and anaerobic, in 116 athletes performing at the XIXth olympic games in Mexico. Ergonomics 13, 665–674. doi: 10.1080/00140137008931192
DiPasquale, D. M. (2017). Moving the debate forward: are normobaric and hypobaric hypoxia interchangeable in the study of altitude? Curr. Sports Med. Rep. 16, 68–70. doi: 10.1249/JSR.0000000000000337
Dougherty, B. J., Terada, J., Springborn, S. R., Vinit, S., MacFarlane, P. M., and Mitchell, G. S. (2017). Daily acute intermittent hypoxia improves breathing function with acute and chronic spinal injury via distinct mechanisms. Respir. Physiol. Neurobiol. doi: 10.1016/j.resp.2017.05.004. [Epub ahead of print].
Droma, Y., Kunii, O., Yangzom, Y., Shan, M., Pingzo, L., and Song, P. (2007). Prevalence and severity of asthma and allergies in schoolchildren in Lhasa, Tibet. Clin. Exp. Allergy 37, 1326–1333. doi: 10.1111/j.1365-2222.2007.02781.x
Eckardt, K. U., Boutellier, U., Kurtz, A., Schopen, M., Koller, E. A., and Bauer, C. (1989). Rate of erythropoietin formation in humans in response to acute hypobaric hypoxia. J Appl Physiol. 66, 1785–1788. doi: 10.1152/jappl.1989.66.4.1785
Egrie, J. C., Strickland, T. W., Lane, J., Aoki, K., Cohen, A. M., Smalling, R., et al. (1986). Characterization and biological effects of recombinant human erythropoietin. Immunobiology 172, 213–224. doi: 10.1016/S0171-2985(86)80101-2
Engst, R., and Vocks, E. (2000). [High-mountain climate therapy for skin diseases and allergies - Mode of action, therapeutic results, and immunologic effects]. Rehabilitation 39, 215–222. doi: 10.1055/s-2000-5897
Esteva, S., Panisello, P., Torrella, J. R., Pagés, T., and Viscor, G. (2009). Blood rheology adjustments in rats after a program of intermittent exposure to hypobaric hypoxia. High Alt. Med. Biol. 10, 275–281. doi: 10.1089/ham.2008.1086
Fábián, Z., Taylor, C. T., and Nguyen, L. K. (2016). Understanding complexity in the HIF signaling pathway using systems biology and mathematical modeling. J. Mol. Med. (Berl). 94, 377–390. doi: 10.1007/s00109-016-1383-6
Fairfax, A. J., David, V., and Douce, G. (1999). Laryngeal aspergillosis following high dose inhaled fluticasone therapy for asthma. Thorax 54, 860–861. doi: 10.1136/thx.54.9.860
Faiss, R., Girard, O., and Millet, G. P. (2013). Advancing hypoxic training in team sports: from intermittent hypoxic training to repeated sprint training in hypoxia. Br. J. Sports Med. 47 (Suppl. 1), i45–i50. doi: 10.1136/bjsports-2013-092741
Feriche, B., García-Ramos, A., Calderón-Soto, C., Drobnic, F., Bonitch-Góngora, J. G., Galilea, P. A., et al. (2014). Effect of acute exposure to moderate altitude on muscle power: hypobaric hypoxia vs. normobaric hypoxia. PLoS ONE 9:e114072. doi: 10.1371/journal.pone.0114072
Filopoulos, D., Cormack, S. J., and Whyte, D. G. (2017). Normobaric hypoxia increases the growth hormone response to maximal resistance exercise in trained men. Eur. J. Sport Sci. 17, 821–829. doi: 10.1080/17461391.2017.1317834
Fulco, C. S., Beidleman, B. A., and Muza, S. R. (2013). Effectiveness of preacclimatization strategies for high-altitude exposure. Exerc. Sport Sci. Rev. 41, 55–63. doi: 10.1097/JES.0b013e31825eaa33
Garvican-Lewis, L. A., Sharpe, K., and Gore, C. J. (2016). Time for a new metric for hypoxic dose? J. Appl. Physiol. 121, 352–355. doi: 10.1152/japplphysiol.00579.2015
Gatterer, H., Haacke, S., Burtscher, M., Faulhaber, M., Melmer, A., Ebenbichler, C., et al. (2015). Normobaric Intermittent Hypoxia over 8 Months Does Not Reduce Body Weight and Metabolic Risk Factors-a Randomized, Single Blind, Placebo-Controlled Study in Normobaric Hypoxia and Normobaric Sham Hypoxia. Obes. Facts 8, 200–209. doi: 10.1159/000431157
Ge, R. L., Chen, Q. H., and He, L. G. (1994). [Characteristics of hypoxic ventilatory response in Tibetan living at moderate and high altitudes]. Zhonghua Jie He He Hu Xi Za Zhi 17, 364–6, 384.
Ge, R., Witkowski, S., Zhang, Y., Alfrey, C., Sivieri, M., Karlsen, T., et al. (2002). Determinants of erythropoietin release in response to short-term hypobaric hypoxia. J. Appl. Physiol. 92, 2361–2367. doi: 10.1152/japplphysiol.00684.2001
Girard, O. (2012). Comments on point:counterpoint: hypobaric hypoxia induces / does not induce different responses from normobaric hypoxia - Reduced air resistance with terrestrial altitude alters run sprint performance. J. Appl. Physiol. (Bethesda, MD 1985) 112:1788. doi: 10.1152/japplphysiol.00356.2012
Gore, C. J., Rodríguez, F. A., Truijens, M. J., Townsend, N. E., Stray-Gundersen, J., and Levine, B. D. (2006). Increased serum erythropoietin but not red cell production after 4 wk of intermittent hypobaric hypoxia (4,000-5,500 m). J. Appl. Physiol. 101, 1386–1393. doi: 10.1152/japplphysiol.00342.2006
Gourgoulianis, K. I., Brelas, N., Hatziparasides, G., Papayianni, M., and Molyvdas, P. A. (2001). The influence of altitude in bronchial asthma. Arch. Med. Res. 32, 429–431. doi: 10.1016/S0188-4409(01)00302-2
Hamlin, M. J., Lizamore, C. A., and Hopkins, W. G. (2018). The effect of natural or simulated altitude training on high-intensity intermittent running performance in team-sport athletes: a meta-analysis. Sports Med. 48, 431–446. doi: 10.1007/s40279-017-0809-9
Harrison, C. C., Fleming, J. M., and Giles, L. C. (2002). Does interval hypoxic training affect the lung function of asthmatic athletes. New Zeal. J. Sport. Med. 30, 64–67. Available online at: http://blm-altitude.com/blm/uploads/images/Gallery/Applications/Health-and-Wellness/Lung-Function-Asthmatic-Athletes.pdf
Haskell, W. L., Lee, I.-M., Pate, R. R., Powell, K. E., Blair, S. N., Franklin, B. A., et al. (2007). Physical activity and public health: updated recommendation for adults from the American College of Sports Medicine and the American Heart Association. Med. Sci. Sports Exerc. 39, 1423–1434. doi: 10.1161/CIRCULATIONAHA.107.185649
Haufe, S., Wiesner, S., Engeli, S., Luft, F. C., and Jordan, J. (2008). Influences of normobaric hypoxia training on metabolic risk markers in human subjects. Med. Sci. Sports Exerc. 40, 1939–1944. doi: 10.1249/MSS.0b013e31817f1988
Hauser, A., Schmitt, L., Troesch, S., Saugy, J. J., Cejuela-Anta, R., Faiss, R., et al. (2016). Similar hemoglobin mass response in hypobaric and normobaric hypoxia in athletes. Med. Sci. Sports Exerc. 48, 734–741. doi: 10.1249/MSS.0000000000000808
Heuschmann, P., Hoffmann, U., Piepoli, M. F., Verschuren, M., Halcox, J., Reviewers, D., et al. (2010). Secondary prevention through cardiac rehabilitation : physical activity counselling and exercise training Key components of the position paper from the Cardiac rehabilitation section of the European Association of Cardiovascular Prevention and Rehabilitation. Eur Heart J. 31, 1967–1976. doi: 10.1093/eurheartj/ehq236
Hirota, K., and Semenza, G. L. (2006). Regulation of angiogenesis by hypoxia-inducible factor 1. Crit. Rev. Oncol. Hematol. 59, 15–26. doi: 10.1016/j.critrevonc.2005.12.003
Hornbein, T. F. (2001). The high-altitude brain. J. Exp. Biol. 204, 3129–3132. Available online at: http://jeb.biologists.org/content/204/18/3129.full.pdf
Ibáñez, J., Casas, H., Casas, M., Rama, R., Ricart, A., Ventura, J. L., et al. (2000). L'exposition intermittente à l'hypoxie en caisson hypobarique: effets physiologiques et applications. Médecine du Sport 74, 32–34
Jia, Y., Suzuki, N., Yamamoto, M., Gassmann, M., and Noguchi, C. T. (2012). Endogenous erythropoietin signaling facilitates skeletal muscle repair and recovery following pharmacologically induced damage. FASEB J. 26, 2847–2858. doi: 10.1096/fj.11-196618
Julian, C. G., Gore, C. J., Wilber, R. L., Daniels, J. T., Fredericson, M., Stray-Gundersen, J., et al. (2004). Intermittent normobaric hypoxia does not alter performance or erythropoietic markers in highly trained distance runners. J. Appl. Physiol. 96, 1800–1807. doi: 10.1016/j.obhdp.2010.11.002
Karagiannidis, C., Hense, G., Rueckert, B., Mantel, P. Y., Ichters, B., Blaser, K., et al. (2006). High-altitude climate therapy reduces local airway inflammation and modulates lymphocyte activation. Scand. J. Immunol. 63, 304–310. doi: 10.1111/j.1365-3083.2006.01739.x
Karlsen, T., Resaland, G. K., Ri-Li, G., Sivieri, M., Witowski, S., Yates, R., et al. (2001). Epo response to 24 hrs of artificial hypobaric hypoxia predicts epo response to natural altitude. Med. Sci. Sport. Exerc. 33:S98. Available online at: https://journals.lww.com/acsm-msse/Fulltext/2001/05001/EPO_RESPONSE_TO_24_HRS_OF_ARTIFICIAL_HYPOBARIC.556.aspx
Kenney, W. L., Wilmore, J. H., Costill, D. L., and Wilmore, J. H. (2012). Physiology of Sport and Exercise. 5th Edn. Champaign IL: Human Kinetics
Khodaee, M., Grothe, H. L., Seyfert, J. H., and VanBaak, K. (2016). Athletes at high altitude. Sports Health 8, 126–132. doi: 10.1177/1941738116630948
Kiechl-Kohlendorfer, U., Horak, E., Mueller, W., Strobl, R., Haberland, C., Fink, F. M., et al. (2007). Living at high altitude and risk of hospitalisation for atopic asthma in children: results from a large prospective birth-cohort study. Arch. Dis. Child. 92, 339–342. doi: 10.1136/adc.2006.106278
Kon, M., Ohiwa, N., Honda, A., Matsubayashi, T., Ikeda, T., Akimoto, T., et al. (2014). Effects of systemic hypoxia on human muscular adaptations to resistance exercise training. Physiol. Rep. 2, 1–13. doi: 10.14814/phy2.12033
Korcarz, C. E., Peppard, P. E., Young, T. B., Chapman, C. B., Hla, K. M., Barnet, J. H., et al. (2016). Effects of obstructive sleep apnea and obesity on cardiac remodeling: the wisconsin sleep cohort study. Sleep 39, 1187–1195. doi: 10.5665/sleep.5828
Koyasu, S., Kobayashi, M., Goto, Y., Hiraoka, M., and Harada, H. (2017). Regulatory mechanisms of hypoxia-inducible factor 1 activity: two decades of knowledge. Cancer Sci. 109, 560–571. doi: 10.1111/cas.13483
Kushwah, N., Jain, V., Deep, S., Prasad, D., Singh, S. B., and Khan, N. (2016). Neuroprotective role of intermittent hypobaric hypoxia in unpredictable chronic mild stress induced depression in rats. PLoS ONE 11:e0149309. doi: 10.1371/journal.pone.0149309
Kusunose, K., Phelan, D., Seicean, S., Seicean, A., Collier, P., Boden, K. A., et al. (2016). Relation of echocardiographic characteristics of the right-sided heart with incident heart failure and mortality in patients with sleep-disordered breathing and preserved left ventricular ejection fraction. Am. J. Cardiol. 118, 1268–1273. doi: 10.1016/j.amjcard.2016.07.024
Leone, R. J., and Lalande, S. (2017). Intermittent hypoxia as a means to improve aerobic capacity in type 2 diabetes. Med. Hypotheses 100, 59–63. doi: 10.1016/j.mehy.2017.01.010
Levett, D. Z., Radford, E. J., Menassa, D. A., Graber, E. F., Morash, A. J., Hoppeler, H., et al. (2012). Acclimatization of skeletal muscle mitochondria to high-altitude hypoxia during an ascent of Everest. FASEB J. 26, 1431–1441. doi: 10.1096/fj.11-197772
Levine, B. D., and Stray-Gundersen, J. (1997). “Living high-training low”: effect of moderate-altitude acclimatization with low-altitude training on performance. J. Appl. Physiol. 83, 102–112. doi: 10.1152/jappl.1997.83.1.102
Li, X., Zhao, H., Wu, Y., Zhang, S., Zhao, X., Zhang, Y., et al. (2014). Up-regulation of hypoxia-inducible factor-1alpha enhanced the cardioprotective effects of ischemic postconditioning in hyperlipidemic rats. Acta Biochim Biophys Sin 46, 112–118. doi: 10.1093/abbs/gmt132
Lieberman, P., Protopapas, A., Reed, E., Youngs, J. W., and Kanki, B. G. (1994). Cognitive defects at altitude. Nature 372:325. doi: 10.1038/372325a0
Lippl, F. J., Neubauer, S., Schipfer, S., Lichter, N., Tufman, A., Otto, B., et al. (2010). Hypobaric hypoxia causes body weight reduction in obese subjects. Obesity 18, 675–681. doi: 10.1038/oby.2009.509
Lizamore, C. A., and Hamlin, M. J. (2017). The use of simulated altitude techniques for beneficial cardiovascular health outcomes in nonathletic, sedentary, and clinical populations: a literature review. High Alt. Med. Biol. 18, 305–321. doi: 10.1089/ham.2017.0050
London, G. M., Zins, B., Pannier, B., Naret, C., Berthelot, J. M., Jacquot, C., et al. (1989). Vascular changes in hemodialysis patients in response to recombinant human erythropoietin. Kidney Int. 36, 878–882. doi: 10.1038/ki.1989.274
Lundby, C., Calbet, J. A. L., Sander, M., Van Hall, G., Mazzeo, R. S., Stray-Gundersen, J., et al. (2007). Exercise economy does not change after acclimatization to moderate to very high altitude. Scand. J. Med. Sci. Sport. 17, 281–291. doi: 10.1111/j.1600-0838.2006.00530.x
Magalhães, J., Falcão-Pires, I., Gonçalves, I. O., Lumini-Oliveira, J., Marques-Aleixo, I., Dos Passos, E., et al. (2013). Synergistic impact of endurance training and intermittent hypobaric hypoxia on cardiac function and mitochondrial energetic and signaling. Int. J. Cardiol. 168, 5363–5371. doi: 10.1016/j.ijcard.2013.08.001
Magalhães, J., Gonçalves, I. O., Lumini-Oliveira, J., Marques-Aleixo, I., Passos, E., Rocha-Rodrigues, S., et al. (2014). Modulation of cardiac mitochondrial permeability transition and apoptotic signaling by endurance training and intermittent hypobaric hypoxia. Int. J. Cardiol. 173, 40–45. doi: 10.1016/j.ijcard.2014.02.011
Manimmanakorn, A., Manimmanakorn, N., Taylor, R., Draper, N., Billaut, F., Shearman, J. P., et al. (2013). Effects of resistance training combined with vascular occlusion or hypoxia on neuromuscular function in athletes. Eur. J. Appl. Physiol. 113, 1767–1774. doi: 10.1007/s00421-013-2605-z
Marquez, J. L., Rubinstein, S., Fattor, J. A., Shah, O., Hoffman, A. R., and Friedlander, A. L. (2013). Cyclic hypobaric hypoxia improves markers of glucose metabolism in middle-aged men. High Alt. Med. Biol. 14, 263–272. doi: 10.1089/ham.2012.1057
Marticorena, E. A. (1993). Montañismo médico: Rehabilitación de pacientes coronarios con by-pass. Rev. Peru. Cardiol. 19, 25–29.
Marticorena, E. A., Marticorena, J. A., Gutierrez, I., Rodríguez, V., Fernández-Dávila, L., Oyola, L., et al. (2001). Factor relajante del endotelio (ON) en rehabilitación coronaria con cámara hipobárica. Rev. Peru. Cardiol. 27, 148–149. Avavilable online at: http://sisbib.unmsm.edu.pe/bvrevistas/cardiologia/anteriores.htm
Mateika, J. H., El-Chami, M., Shaheen, D., and Ivers, B. (2015). Intermittent hypoxia: a low-risk research tool with therapeutic value in humans: Fig. 1. J. Appl. Physiol. 118, 520–532. doi: 10.1152/japplphysiol.00564.2014
Meehan, R. T. (1987). Immune suppression at high altitude. Ann. Emerg. Med. 16, 974–979. doi: 10.1016/S0196-0644(87)80743-6
Meehan, R., Duncan, U., Neale, L., Taylor, G., Muchmore, H., Scott, N., et al. (1988). Operation Everest II: alterations in the immune system at high altitudes. J. Clin. Immunol. 8, 397–406. doi: 10.1007/BF00917156
Milledge, J. S. (2003). “Altitude deterioration,” in Health and Height (Barcelona: Publicacions Universitat de Barcelona), 173–180. Available online at: http://www.publicacions.ub.edu/ficha.aspx?cod=06036
Millet, G. P., Debevec, T., Brocherie, F., Malatesta, D., and Girard, O. (2016). Therapeutic use of exercising in hypoxia : promises and limitations. Front. Physiol. 7:224. doi: 10.3389/fphys.2016.00224
Millet, G. P., Faiss, R., and Pialoux, V. (2012). Point: Counterpoint: Hypobaric hypoxia induces/does not induce different responses from normobaric hypoxia. J. Appl. Physiol. 112, 1783–1784. doi: 10.1152/japplphysiol.00067.2012
Millet, G. P., Faiss, R., and Pialoux, V. (2013). Evidence for differences between hypobaric and normobaric hypoxia is conclusive. Exerc. Sport Sci. Rev. 41:133. doi: 10.1097/JES.0b013e318271a5e1
Najean, Y., Moynot, A., Deschryver, F., Zins, B., Naret, C., Jacquot, C., et al. (1989). Kinetics of erythropoiesis in dialysis patients receiving recombinant erythropoietin treatment. Nephrol. Dial. Transplant 4, 350–355. doi: 10.1093/oxfordjournals.ndt.a091889
Navarrete-Opazo, A., Alcayaga, J., Sepúlveda, O., Rojas, E., and Astudillo, C. (2017a). Repetitive intermittent hypoxia and locomotor training enhances walking function in incomplete spinal cord injury subjects: a randomized, triple-blind, placebo-controlled clinical trial. J. Neurotrauma 34, 1803–1812. doi: 10.1089/neu.2016.4478
Navarrete-Opazo, A., and Mitchell, G. S. (2014). Therapeutic potential of intermittent hypoxia: a matter of dose. Am. J. Physiol. Regul. Integr. Comp. Physiol. 307, R1181–R1197. doi: 10.1152/ajpregu.00208.2014
Navarrete-Opazo, A., Dougherty, B. J., and Mitchell, G. S. (2017b). Enhanced recovery of breathing capacity from combined adenosine 2A receptor inhibition and daily acute intermittent hypoxia after chronic cervical spinal injury. Exp. Neurol. 287, 93–101. doi: 10.1016/j.expneurol.2016.03.026
Navarrete-Opazo, A., Vinit, S., Dougherty, B. J., and Mitchell, G. S. (2015). Daily acute intermittent hypoxia elicits functional recovery of diaphragm and inspiratory intercostal muscle activity after acute cervical spinal injury. Exp. Neurol. 266, 1–10. doi: 10.1016/j.expneurol.2015.02.007
Nelson, M. E., Rejeski, W. J., Blair, S. N., Duncan, P. W., Judge, J. O., King, A. C., et al. (2007). Physical activity and public health in older adults: recommendation from the American College of Sports Medicine and the American Heart Association. Med. Sci. Sports Exerc. 39, 1435–1445. doi: 10.1249/mss.0b013e3180616aa2
Netzer, N. C., Chytra, R., and Küpper, T. (2008). Low intense physical exercise in normobaric hypoxia leads to more weight loss in obese people than low intense physical exercise in normobaric sham hypoxia. Sleep Breath. 12, 129–134. doi: 10.1007/s11325-007-0149-3
Noguchi, C. T., Asavaritikrai, P., Teng, R., and Jia, Y. (2007). Role of erythropoietin in the brain. 64, 159–171 doi: 10.1016/j.critrevonc.2007.03.001
Nummela, A., and Rusko, H. (2000). Acclimatization to altitude and normoxic training improve 400-m running performance at sea level. J. Sports Sci. 18, 411–419. doi: 10.1080/02640410050074340
Núñez-Espinosa, C., Douziech, A., Ríos-Kristjánsson, J. G., Rizo, D., Torrella, J. R., Pagès, T., et al. (2014). Effect of intermittent hypoxia and exercise on blood rheology and oxygen transport in trained rats. Respir. Physiol. Neurobiol. 192, 112–117. doi: 10.1016/j.resp.2013.12.011
Ohta, A. A., Diwanji, R., Kini, R., Subramanian, M., Ohta, A. A., and Sitkovsky, M. (2011). In vivo T cell activation in lymphoid tissues is inhibited in the oxygen-poor microenvironment. Front. Immunol. 2:27. doi: 10.3389/fimmu.2011.00027
Oliver, S. J., Macdonald, J. H., Harper Smith, A. D., Lawley, J. S., Gallagher, C. a, Di Felice, U., et al. (2013). High altitude impairs in vivo immunity in humans. High Alt. Med. Biol. 14, 144–149. doi: 10.1089/ham.2012.1070
Ostadal, B., and Kolar, F. (2007). Cardiac adaptation to chronic high-altitude hypoxia: beneficial and adverse effects. Respir. Physiol. Neurobiol. 158, 224–236. doi: 10.1016/j.resp.2007.03.005
Ostádal, B., Kolár, F., Pelouch, V., Bass, A., Samánek, M., and Procházka, J. (1989). The effect of chronic hypoxia on the developing cardiopulmonary system. Biomed. Biochim. Acta 48, S58–62.
Panisello, P., Torrella, J. R., Esteva, S., Pagés, T., and Viscor, G. (2008). Capillary supply, fibre types and fibre morphometry in rat tibialis anterior and diaphragm muscles after intermittent exposure to hypobaric hypoxia. Eur. J. Appl. Physiol. 103, 203–213. doi: 10.1007/s00421-008-0691-0
Panisello, P., Torrella, J. R., Pagés, T., and Viscor, G. (2007). Capillary supply and fiber morphometry in rat myocardium after intermittent exposure to hypobaric hypoxia. High Alt. Med. Biol. 8, 322–330. doi: 10.1089/ham.2007.1030
Ramos-Campo, D. J., Martínez-Sánchez, F., Esteban-García, P., Rubio-Arias, J. A., Clemente-Suarez, V. J., and Jiménez-Díaz, J. F. (2015). The effects of intermittent hypoxia training on hematological and aerobic performance in triathletes. Acta Physiol. Hung. 102, 409–418. doi: 10.1556/036.102.2015.4.8
Ramos-Campo, D. J., Scott, B. R., Alcaraz, P. E., and Rubio-Arias, J. A. (2018). The efficacy of resistance training in hypoxia to enhance strength and muscle growth: a systematic review and meta-analysis. Eur. J. Sport Sci. 18, 92–103. doi: 10.1080/17461391.2017.1388850
Rathat, C., Richalet, J. P., Herry, J. P., and Larmignat, P. (1992). Detection of high-risk subjects for high altitude diseases. Int. J. Sports Med. 13 (Suppl. 1), S76–S78. doi: 10.1055/s-2007-1024602
Rey, S., and Semenza, G. L. (2010). Hypoxia-inducible factor-1-dependent mechanisms of vascularization and vascular remodelling. Cardiovasc. Res. 86, 236–242. doi: 10.1093/cvr/cvq045
Reynafarje, B. D., and Marticorena, E. (2002). Bioenergetics of the heart at high altitude: environmental hypoxia imposes profound transformations on the myocardial process of ATP synthesis. J. Bioenerg. Biomembr. 34, 407–412. doi: 10.1023/A:1022597523483
Ricart, A., Casas, H., Casas, M., Pagés, T., Palacios, L., Rama, R., et al. (2000). Acclimatization near home? Early respiratory changes after short-term intermittent exposure to simulated altitude. Wilderness Environ. Med. 11, 84–88. doi: 10.1580/1080-6032(2000)011[0084:ANHERC]2.3.CO;2
Richalet, J. P., Bittel, J., Herry, J. P., Savourey, G., Le Trong, J. L., Auvert, J. F., et al. (1992). Use of a hypobaric chamber for pre-acclimatization before climbing Mount Everest. Int. J. Sports Med. 13 (Suppl. 1), S216–S220. doi: 10.1055/s-2007-1024644
Richalet, J. P., Souberbielle, J. C., Antezana, A. M., Déchaux, M., Le Trong, J. L., Bienvenu, A., et al. (1994). Control of erythropoiesis in humans during prolonged exposure to the altitude of 6,542 m. Am. J. Physiol. 266, R756–R764. doi: 10.1152/ajpregu.1994.266.3.R756
Richalet, J.-P., and Gore, C. J. (2008). Live and/or sleep high:train low, using normobaric hypoxia. Scand. J. Med. Sci. Sports 18 (Suppl. 1), 29–37. doi: 10.1111/j.1600-0838.2008.00830.x
Richalet, J.-P., and Lhuissier, F. J. (2015). Aging, tolerance to high altitude, and cardiorespiratory response to hypoxia. High Alt. Med. Biol. 16:117–124. doi: 10.1089/ham.2015.0030
Rizo-Roca, D., Bonet, J. B., Inal, B., Ríos-Kristjánsson, J. G., Pagès, T., Viscor, G., et al. (2018). Contractile activity is necessary to trigger intermittent hypobaric hypoxia-induced fibre size and vascular adaptations in skeletal muscle. Front. Physiol. 9:481. doi: 10.3389/FPHYS.2018.00481
Rizo-Roca, D., Ríos-Kristjánsson, J. G., Núñez-Espinosa, C., Santos-Alves, E., Gonçalves, I. O., Magalhães, J., et al. (2017a). Intermittent hypobaric hypoxia combined with aerobic exercise improves muscle morphofunctional recovery after eccentric exercise to exhaustion in trained rats. J. Appl. Physiol. 122, 580–592. doi: 10.1152/japplphysiol.00501.2016
Rizo-Roca, D., Ríos-Kristjánsson, J. G., Núñez-Espinosa, C., Santos-Alves, E., Magalhães, J., Ascensão, A., et al. (2017b). Modulation of mitochondrial biomarkers by intermittent hypobaric hypoxia and aerobic exercise after eccentric exercise in trained rats. Appl. Physiol. Nutr. Metab. 42, 683–693. doi: 10.1139/apnm-2016-0526
Rodríguez, F. A., Casas, H., Casas, M., Pagés, T., Rama, R., Ricart, A., et al. (1999). Intermittent hypobaric hypoxia stimulates erythropoiesis and improves aerobic capacity. Med. Sci. Sports Exerc. 31, 264–268. doi: 10.1097/00005768-199902000-00010
Rodríguez, F. A., Iglesias, X., Feriche, B., Calderón-Soto, C., Chaverri, D., Wachsmuth, N. B., et al. (2015). Altitude Training in Elite Swimmers for Sea Level Performance (Altitude Project). Med. Sci. Sports Exerc. 47, 1965–1978. doi: 10.1249/MSS.0000000000000626
Rodríguez, F. A., Truijens, M. J., Townsend, N. E., Gore, C. J., Levine, B. D., Truijens, M. J., et al. (2007). Performance of runners and swimmers after four weeks of intermittent hypobaric hypoxic exposure plus sea level training. J. Appl. Physiol. 103, 1523–1535. doi: 10.1152/japplphysiol.01320.2006
Rodríguez, F. A., Ventura, J. L., Casas, M., Casas, H., Pagés, T., Rama, R., et al. (2000). Erythropoietin acute reaction and haematological adaptations to short, intermittent hypobaric hypoxia. Eur. J. Appl. Physiol. 82, 170–177. doi: 10.1007/s004210050669
Ross, H. H., Sandhu, M. S., Cheung, T. F., Fitzpatrick, G. M., Sher, W. J., Tiemeier, A. J., et al. (2012). In vivo intermittent hypoxia elicits enhanced expansion and neuronal differentiation in cultured neural progenitors. Exp. Neurol. 235, 238–245. doi: 10.1016/j.expneurol.2012.01.027
Salpeter, S. R., Buckley, N. S., Ormiston, T. M., and Salpeter, E. E. (2006). Meta-analysis: effect of long-acting beta-agonists on severe asthma exacerbations and asthma-related deaths. Ann. Intern. Med. 144, 904–912. doi: 10.7326/0003-4819-144-12-200606200-00126
Sanchez, A. M. J., and Borrani, F. (2018). Effects of intermittent hypoxic training performed at high hypoxia level on exercise performance in highly trained runners. J. Sports Sci. 36, 2045–2052. doi: 10.1080/02640414.2018.1434747
Saugy, J. J., Schmitt, L., Hauser, A., Constantin, G., Cejuela, R., Faiss, R., et al. (2016). Same Performance Changes after Live High-Train Low in Normobaric vs. Hypobaric Hypoxia. Front. Physiol. 7:138. doi: 10.3389/fphys.2016.00138
Savourey, G., Launay, J.-C., Besnard, Y., Guinet, A., and Travers, S. (2003). Normo- and hypobaric hypoxia: are there any physiological differences? Eur. J. Appl. Physiol. 89, 122–126. doi: 10.1007/s00421-002-0789-8
Schommer, K., Wiesegart, N., Menold, E., Haas, U., Lahr, K., Buhl, H., et al. (2010). Training in normobaric hypoxia and its effects on acute mountain sickness after rapid ascent to 4559 m. High Alt. Med. Biol. 11, 19–25. doi: 10.1089/ham.2009.1019
Schultze-Werninghaus, G. (2006). Should asthma management include sojourns at high altitude? Chem. Immunol. Allergy 91, 16–29. doi: 10.1159/000090227
Schultze-Werninghaus, G. (2008). [Effects of high altitude on bronchial asthma]. Pneumologie 62, 170–176. doi: 10.1055/s-2007-1016442
Serebrovska, T. V., Portnychenko, A. G., Drevytska, T. I., Portnichenko, V. I., Xi, L., Egorov, E., et al. (2017). Intermittent hypoxia training in prediabetes patients: Beneficial effects on glucose homeostasis, hypoxia tolerance and gene expression. Exp. Biol. Med. (Maywood). 242, 1542–1552. doi: 10.1177/1535370217723578
Serebrovska, T. V., Serebrovska, Z. O., and Egorov, E. (2016). Fitness and therapeutic potential of intermittent hypoxia training: a matter of dose. Fiziol. Zh. 62, 78–91. Available online at: https://fz.kiev.ua/journals/2016_V.62/2016_3/2016_3-78-91.pdf
Serebrovskaya, T. V., Manukhina, E. B., Smith, M. L., Downey, H. F., and Mallet, R. T. (2008). Intermittent hypoxia: cause of or therapy for systemic hypertension? Exp. Biol. Med. (Maywood). 233, 627–650. doi: 10.3181/0710-MR-267
Serebrovskaya, T. V., Swanson, R. J., and Kolesnikova, E. E. (2003). Intermittent hypoxia: mechanisms of action and some applications to bronchial asthma treatment. J. Physiol. Pharmacol. 54 (Suppl. 1), 35–41. doi: 10.318/0710-MR-
Serebrovskaya, T. V (2002). Intermittent hypoxia research in the former Soviet Union and the Commonwealth of Independent States: history and review of the concept and selected applications. High Alt. Med. Biol. 3, 205–221. doi: 10.1089/15270290260131939
Simon, H. U., Grotzer, M., Nikolaizik, W. H., Blaser, K., and Schöni, M. H. (1994). High altitude climate therapy reduces peripheral blood T lymphocyte activation, eosinophilia, and bronchial obstruction in children with house-dust mite allergic asthma. Pediatr. Pulmonol. 17, 304–311. doi: 10.1002/ppul.1950170507
Singh, I., Chohan, I. S., Lal, M., Khanna, P. K., Srivastava, M. C., Nanda, R. B., et al. (1977). Effects of high altitude stay on the incidence of common diseases in man. Int. J. Biometeorol. 21, 93–122. doi: 10.1007/BF01553705
Steiner, C. (2009). [Atopic dermatitis and psoriasis: what is the benefit of in-patient high altitude climate therapy?]. Praxis (Bern. 1994). 98, 1373–1376. doi: 10.1024/1661-8157.98.23.1373
Stray-Gundersen, J., and Levine, B. D. (1999). “Living high and training low” can improve sea level performance in endurance athletes. 33, 150–151. doi: 10.1136/bjsm.33.3.150
Stray-Gundersen, J., Chapman, R. F., and Levine, B. D. (2001). “Living high-training low” altitude training improves sea level performance in male and female elite runners. J. Appl. Physiol. 91, 1113–1120. doi: 10.1152/jappl.2001.91.3.1113
Streeter, K. A., Sunshine, M. D., Patel, S., Gonzalez-Rothi, E. J., Reier, P. J., Baekey, D. M., et al. (2017). Intermittent hypoxia enhances functional connectivity of midcervical spinal interneurons. J. Neurosci. 37, 8349–8362. doi: 10.1523/JNEUROSCI.0992-17.2017
Sy, D. Q., Thanh Binh, M. H., Quoc, N. T., Hung, N. V., Quynh Nhu, D. T., Bao, N. Q., et al. (2007). Prevalence of asthma and asthma-like symptoms in Dalat Highlands, Vietnam. Singapore Med. J. 48, 294–303. Available online at: http://smj.sma.org.sg/4804/4804a4.pdf
Thanigaimani, S., McLennan, E., Linz, D., Mahajan, R., Agbaedeng, T. A., Lee, G., et al. (2017). Progression and reversibility of stretch induced atrial remodeling: characterization and clinical implications. Prog. Biophys. Mol. Biol. 130, 376–386. doi: 10.1016/j.pbiomolbio.2017.07.010
Tin'kov, A. N., and Aksenov, V. A. (2002). Effects of intermittent hypobaric hypoxia on blood lipid concentrations in male coronary heart disease patients. High Alt. Med. Biol. 3, 277–282. doi: 10.1089/152702902320604250
Truijens, M. J., Rodríguez, F. A., Townsend, N. E., Stray-Gundersen, J., Gore, C. J., and Levine, B. D. (2008). The effect of intermittent hypobaric hypoxic exposure and sea level training on submaximal economy in well-trained swimmers and runners. J. Appl. Physiol. 104, 328–337. doi: 10.1152/japplphysiol.01324.2006
Trumbower, R. D., Hayes, H. B., Mitchell, G. S., Wolf, S. L., and Stahl, V. A. (2017). Effects of acute intermittent hypoxia on hand use after spinal cord trauma: a preliminary study. Neurology 89, 1904–1907. doi: 10.1212/WNL.0000000000004596
Tsai, Y.-W., Yang, Y.-R., Sun, S. H., Liang, K.-C., and Wang, R.-Y. (2013). Post ischemia intermittent hypoxia induces hippocampal neurogenesis and synaptic alterations and alleviates long-term memory impairment. J. Cereb. Blood Flow Metab. 33, 764–773. doi: 10.1038/jcbfm.2013.15
Turban, K., and Spengler, L. (1906). Resultate der Asthmabehandlung im Hochgebirge. Jahresbericht der Schweiz Balneol. Gesellschaft 2:72.
Valle, M., del, P., García-Godos, F., Woolcott, O. O., Marticorena, J. M., Rodríguez, V., et al. (2006). Improvement of myocardial perfusion in coronary patients after intermittent hypobaric hypoxia. J. Nucl. Cardiol. 13, 69–74. doi: 10.1016/j.nuclcard.2005.11.008
van der Woude, H. J., Winter, T. H., and Aalbers, R. (2001). Decreased bronchodilating effect of salbutamol in relieving methacholine induced moderate to severe bronchoconstriction during high dose treatment with long acting beta2 agonists. Thorax 56, 529–535. doi: 10.1136/thorax.56.7.529
van Velzen, E., van den Bos, J. W., Benckhuijsen, J. A., van Essel, T., de Bruijn, R., and Aalbers, R. (1996). Effect of allergen avoidance at high altitude on direct and indirect bronchial hyperresponsiveness and markers of inflammation in children with allergic asthma. Thorax 51, 582–584. doi: 10.1136/thx.51.6.582
Virués-Ortega, J., Garrido, E., Javierre, C., and Kloezeman, K. C. (2006). Human behaviour and development under high-altitude conditions. Dev. Sci. 9, 400–410. doi: 10.1111/j.1467-7687.2006.00505.x
Viscor, G., Javierre, C., Pagès, T., Ventura, J.-L., Ricart, A., Martin-Henao, G., et al. (2009). Combined intermittent hypoxia and surface muscle electrostimulation as a method to increase peripheral blood progenitor cell concentration. J. Transl. Med. 7:91. doi: 10.1186/1479-5876-7-91
Viscor, G., Ricart, A., Pagès, T., Corral, L., Javierre, C. F., and Ventura, J. L. (2014). Intermittent hypoxia for obstructive sleep apnea? High Alt. Med. Biol. 15, 520–521. doi: 10.1089/ham.2014.1060
Vocks, E., Schuh, A., Liebich, C., Topperzer, U., and Ring, J. (1999). High altitude stay and plasma cortisol level in psoriasis. Phys. Medizin Rehabil. Kurortmedizin 9, 197–201. doi: 10.1055/s-2008-1061805
Wang, G. L., and Semenza, G. L. (1993). General involvement of hypoxia-inducible factor 1 in transcriptional response to hypoxia. Proc. Natl. Acad. Sci.U.S.A. 90, 4304–4308. doi: 10.1073/pnas.90.9.4304
Ward, M. (1954). High altitude deterioration. Proc. R. Soc. London. Ser. B Biol. Sci. 143, 40–42. doi: 10.2307/83018
West, J. B., Schoene, R. B., Luks, A. M., and Milledge, J. S. (2013). High Altitude Medicine and Physiology. 5th Edn. Boca Raton, FL: CRC Press/Taylor & Francis Group
Wiesner, S., Haufe, S., Engeli, S., Mutschler, H., Haas, U., Luft, F. C., et al. (2010). Influences of normobaric hypoxia training on physical fitness and metabolic risk markers in overweight to obese subjects. Obesity 18, 116–120. doi: 10.1038/oby.2009.193
Wilber, R. L., Stray-Gundersen, J., and Levine, B. D. (2007). Effect of hypoxic “dose” on physiological responses and sea-level performance. Med. Sci. Sports Exerc. 39, 1590–1599. doi: 10.1249/mss.0b013e3180de49bd
Wille, M., Gatterer, H., Mairer, K., Philippe, M., Schwarzenbacher, H., Faulhaber, M., et al. (2012). Short-term intermittent hypoxia reduces the severity of acute mountain sickness. Scand. J. Med. Sci. Sport. 22, 79–85. doi: 10.1111/j.1600-0838.2012.01499.x
Wu, Q., Yu, K. X., Ma, Q. S., and Liu, Y. N. (2015). Effects of intermittent hypobaric hypoxia preconditioning on the expression of neuroglobin and Bcl-2 in the rat hippocampal CA1 area following ischemia-reperfusion. Genet. Mol. Res. 14, 10799–10807. doi: 10.4238/2015.September.9.18
Xi, L., and Serebrovskaya, T. V (eds) (2012). Intermittent Hypoxia and Human Diseases. London: Springer.
Xing, B., Chen, H., Zhang, M., Zhao, D., Jiang, R., Liu, X., et al. (2008). Ischemic postconditioning inhibits apoptosis after focal cerebral ischemia/reperfusion injury in the rat. Stroke 39, 2362–2369. doi: 10.1161/STROKEAHA.107.507939
Xu, Q., Wang, S., Jiang, X., Zhao, Y., Gao, M., Zhang, Y., et al. (2007). Hypoxia-induced astrocytes promote the migration of neural progenitor cells via vascular endothelial factor, stem cell factor, stromal-derived factor-1alpha and monocyte chemoattractant protein-1 upregulation in vitro. Clin. Exp. Pharmacol. Physiol. 34, 624–631. doi: 10.1111/j.1440-1681.2007.04619.x
Yangzong Nafstad, P., Madsen, C., and Bjertness, E. (2006). Childhood asthma under the north face of Mount Everest. J. Asthma 43, 393–398. doi: 10.1080/02770900600710326
Zhang, H., Li, Q., Li, Z., Mei, Y., and Guo, Y. (2008). The protection of Bcl-2 overexpression on rat cortical neuronal injury caused by analogous ischemia/reperfusion in vitro. Neurosci. Res. 62, 140–146. doi: 10.1016/j.neures.2008.07.002
Zhang, K., Zhao, T., Huang, X., Wu, L., Wu, K., Zhu, L., et al. (2014a). Notch1 mediates postnatal neurogenesis in hippocampus enhanced by intermittent hypoxia. Neurobiol. Dis. 64, 66–78. doi: 10.1016/j.nbd.2013.12.010
Zhang, S., Guo, Z., Yang, S., Ma, H., Fu, C., Wang, S., et al. (2016a). Chronic intermittent hybobaric hypoxia protects against cerebral ischemia via modulation of mitoKATP. Neurosci. Lett. 635, 8–16. doi: 10.1016/j.neulet.2016.10.025
Zhang, Y., Wang, L., Dey, S., Alnaeeli, M., Suresh, S., Rogers, H., et al. (2014b). Erythropoietin action in stress response, tissue maintenance and metabolism. Int. J. Mol. Sci. 15, 10296–10333. doi: 10.3390/ijms150610296
Zhang, Z., Yan, J., and Shi, H. (2016b). Role of hypoxia Inducible Factor 1 in hyperglycemia-exacerbated blood-brain barrier disruption in ischemic stroke. Neurobiol. Dis. 95, 82–92. doi: 10.1016/j.nbd.2016.07.012
Zhou, S., Yi, T., Liu, R., Bian, C., Qi, X., He, X., et al. (2012). Proteomics identification of annexin A2 as a key mediator in the metastasis and proangiogenesis of endometrial cells in human adenomyosis. Mol. Cell. Proteomics 11:M112.017988. doi: 10.1074/mcp.M112.017988
Zhu, L., Zhao, T., Li, H., Zhao, H., Wu, L.-Y., Ding, A., et al. (2005). Neurogenesis in the adult rat brain after intermittent hypoxia. Brain Res. 1055, 1–6. doi: 10.1016/j.brainres.2005.04.075
Keywords: intermittent hypoxia, erythropoiesis, angiogenesis, cardioprotection, bronchial asthma, neuroprotection, circulating stem cells, regenerative medicine
Citation: Viscor G, Torrella JR, Corral L, Ricart A, Javierre C, Pages T and Ventura JL (2018) Physiological and Biological Responses to Short-Term Intermittent Hypobaric Hypoxia Exposure: From Sports and Mountain Medicine to New Biomedical Applications. Front. Physiol. 9:814. doi: 10.3389/fphys.2018.00814
Received: 16 February 2018; Accepted: 11 June 2018;
Published: 09 July 2018.
Edited by:
Rodrigo Del Rio, Pontificia Universidad Católica de Chile, ChileReviewed by:
Cheryl Heesch, University of Missouri, United StatesSimona Mrakic-Sposta, Istituto di Bioimmagini e Fisiologia Molecolare, Italy
Copyright © 2018 Viscor, Torrella, Corral, Ricart, Javierre, Pages and Ventura. This is an open-access article distributed under the terms of the Creative Commons Attribution License (CC BY). The use, distribution or reproduction in other forums is permitted, provided the original author(s) and the copyright owner(s) are credited and that the original publication in this journal is cited, in accordance with accepted academic practice. No use, distribution or reproduction is permitted which does not comply with these terms.
*Correspondence: Ginés Viscor, Z3Zpc2NvckB1Yi5lZHU=