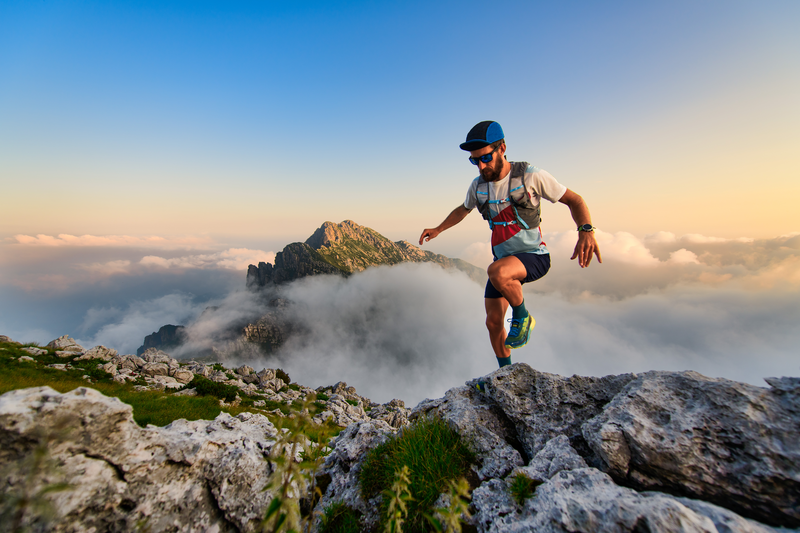
94% of researchers rate our articles as excellent or good
Learn more about the work of our research integrity team to safeguard the quality of each article we publish.
Find out more
ORIGINAL RESEARCH article
Front. Physiol. , 19 June 2018
Sec. Aquatic Physiology
Volume 9 - 2018 | https://doi.org/10.3389/fphys.2018.00757
This article is part of the Research Topic The Physiological and Molecular Stress Responses in Aquatic Species: The Impact on Aquatic Animal Health View all 41 articles
In order to investigate the ecotoxicological effects of nano-ZnO particles and seawater acidification on marine bivalves, the thick shell mussels, Mytilus coruscus were subjected to joint treatments with different nano-ZnO concentrations (0 [control], 2.5 [medium] and 10 mg L-1 [high]) under two pH levels (7.7 [low]and 8.1 [control]) for 14 days. The results showed that respiration rate (RR), absorption efficiency (AE), clearance rate (CR), O:N ratio and scope for growth (SFG) were significantly reduced with nano-ZnO concentration increase, but ammonium excretion rate (ER) was increased. Low pH significantly reduced CR, RR, SFG, and O:N ratio of the mussels especially under high nano-ZnO conditions, and significantly increased ER. Principal component analysis (PCA) showed consistent relationships among most tested parameters, especially among SFG, RR, O:N ratio and CR under the normal pH and 0 nano-ZnO conditions. Therefore, seawater acidification and nano-ZnO interactively impact the ecophysiological responses of mussels and cause more severe effects when they appear concurrently.
Since the beginning of industrial revolution, large amounts of fossil fuels have been burned and the green vegetation has been reduced greatly worldwide, resulting in dramatic increased carbon dioxide (CO2) emissions (Caldeira and Wickett, 2003; Intergovernmental Panel on Climate Change [IPCC], 2014). The ocean plays an important role in the global carbon cycle, and about one-third of the CO2 emitted by human activities is absorbed by the oceans (Feely et al., 2004). The absorption of CO2 in the ocean slows down the trend of rising atmospheric carbon dioxide concentration, but the continuous absorption of CO2 changes the carbon dioxide-carbonate system of seawater, resulting in increased concentrations of hydrogen ions, CO2 and bicarbonate in seawater, and decreased carbonate concentration, ultimately ocean acidification (OA) (Sabine et al., 2004; Wang et al., 2012). According to the current energy use structure, it is estimated that by 2100, the ocean’s pH will be further reduced by 0.3–0.4 units, and by 0.7 units to 2300 years (Orr et al., 2005). According to the exiting evidences, OA can affect the physiological process and fitness of marine animals through acid-base regulation, digestion, metabolism and growth (Fabry et al., 2008; Melzner et al., 2009; Kroeker et al., 2010; Lannig et al., 2010; Fernández-Reiriz et al., 2011; Gazeau et al., 2013; Matoo et al., 2013; Stumpp et al., 2013; Jakubowska and Normant, 2015; Hu et al., 2015; Jansson et al., 2015).
Most nanoparticle contaminants can be deposited in water, posing a threat to aquatic ecosystem (Renzi and Guerranti, 2015). Due to the widespread use of zinc oxide nanoparticles, coastal waters and the ocean would become an ultimate sink of these environmental contaminants (Yung et al., 2014). The application of nanoparticles has attracted more and more attention in aquatic environmental research (Montes et al., 2012; Gagné et al., 2016). It has been found that nanoparticles exert immunotoxic effects on shellfish cells, such as altering DNA structure, transcription level of immune-related genes and expression of related proteins in shellfish, and inhibiting development of shellfish embryos and larvae (Wang et al., 2014). Particularly, nano-ZnO stimulates the respiration rate (RR) and reduces the time of survival of mussels (Hanna et al., 2013). Also, Muller et al. (2014) pointed out that long-term exposure of Mytilus galloprovincialis to nano-ZnO cause accumulation of zinc in mussel tissues, affecting the energy budget. Although nano-ZnO has attracted some attention in ecotoxicological research, there is little research on the combined physiological effects of nano-ZnO associated with additional environmental stressors on marine bivalves. Therefore, it is necessary to explore the combined effects of nano-ZnO and OA on marine species.
Mytilus coruscus is an important marine bivalve species in the East China Sea. The individual is large with poor mobility and long life history, and can accumulate environmental pollutants (such as heavy metals), with strong tolerance to environmental disturbance, thus often serves as an environmental indicator to monitor the changes of coastal environment (Foy et al., 2001; Reeburgh, 2007; Tian et al., 2011; Liu et al., 2014). Scope for growth (SFG), referring to the net energy balance of molluscs, is defined as the energy transferred from the diet into growth by deducting the energy consumed by respiration and excretion, which can be determined by integrating some physiological parameters (i.e., clearance, absorption, respiration, and excretion, Bayne and Widdows, 1978; Widdows and Hawkins, 1989; Smaal and Widdows, 1994; Widdows et al., 2002; Widdows and Staff, 2006; Sarà et al., 2008; Halldórsson et al., 2008; Fernández-Reiriz et al., 2011). These physiological indicators, are not only able to predict the growth rate very well (Bayne et al., 1979), but also good indicators of the health state and the sensitive indexes of the mussels to the environmental changes (Wang et al., 2012). The purpose of this study was to probe the interactive effects of decreased pH and nano-ZnO on the physiological energetic in the mussel M. coruscus. The physiological parameters of the shellfish were determined, including clearance rate (CR), RR, absorption efficiency (AE), ammonium excretion rate (ER), SFG, and O:N ratio.
Nano-ZnO powder (declared purity of 99.9%) was purchased in Horsehead Company, United States. It was made into stock solution for preservation (10 g nano-ZnO L-1). The morphology and particle size of nano-ZnO were measured by transmission electron microscope (Low Voltage Tem5, LVEM5) and scanning electron microscopy (Hitachi JSM-7500F). X-ray diffraction of nano-ZnO was determined by X’Pert PRO X-ray Diffractometer (PANalytical B.V.). Particle size range and zeta potential of nano-ZnO were determined by dynamic light scattering (DLS) using a ZetaSizer Nano ZEN3600 (Malvern, United Kingdom).
Adult mussels (shell length: 8.0 ± 2.0 cm; dry weight 1.6 ± 0.9 g) were collected from the Gouqi island, Zhejiang Province, China, acclimated for 1 week in full aeration seawater (temperature: 20 ± 0.5°C; pH: 8.1; salinity: 25 ± 1 psu), and fed daily with the microalgae Chlorella spp. (2.5 × 105cells mL-1). The handling of experimental mussels was carried on in terms of regulations of the animal welfare for scientific research made by the Institutional Animal Care and Use Committee (IACUC) of Shanghai Ocean University.
The mussels were placed in six treatments: two values of pH [7.7 as the low value of present natural variability at the sampling site (Li et al., 2014), 8.1 as the present average pH] and two doses (mg L-1) of nano-ZnO (0 as control, 2.5 as medium, and 10.0 as high). The concentrations of 1–10 mg L-1 n-ZnO are the most common concentrations currently used in laboratory research, which allow for sub-lethal physiological effects over the exposure period rather than animal mortality (Ciacci et al., 2012; Hao and Chen, 2012). The mussels were divided into six treatments randomly, the six treatments were carried out in exactly the same circulatory system, each system set up three repeated experimental tanks, and each tank contained 30 mussels, with the above mentioned microalgae feed. The low pH value was reduced by adding pure CO2 through a pCO2/pH system (DAQ-M), which was equipped with pH meter (WTW 3310) and pH electrodes (SenTix 41) and operated by CapCTRL software (Loligo Systems Inc.). A multiparameter instrument (model 5200A, YSI, United States) was used to measure seawater salinity. Total alkalinity (TA) was measured by titration method. Other carbonate chemical parameters of seawater (dissolved inorganic carbon (DIC), pCO2, calcite saturation state (Ωcal) and aragonite saturation state (Ωara)) were calculated based on TA and pHNBS using CO2SYS (Lewis et al., 2013).
The thick shell mussels were fasted for 6 h to clear the intestine. They were acclimated for 15 min to ensure they open shells and exchange seawater with the outside environment. Then the microalgae with the initial concentration of 2.5 × 104 cells mL-1 were added, and no pseudo-feces were produced in a preliminary experiment. Three same experimental tanks without mussels were used as the control. At the beginning of the experiment, an initial 20 ml of seawater was taken from the each experimental tank using pipets, after mussels were fed for 60 min, 20 ml of water sample was taken from each experimental tank. The cell concentration in seawater sample was measured with a Coulter Counter (Multisizer 3, Beckman, Irvine, CA, United States). There was no significant decrease in cell concentration during the experiment in the control tanks. CR was computed by the following formula of (Coughlan, 1969):
where CR denotes the CR (L h-1 g-1), V represents the volume of seawater in the tanks (L), C0 is the initial cell concentration (cells mL-1), Ct is the cell concentration at time t (cells mL-1), N is the number of animals in the tank, t is the sampling time (h). CR and other subsequent physiological parameters were standardized to unit dry weight.
After measuring CR, the feces of each replicate tank were collected to calculate AE. Four liters of seawater containing 2.5 × 104 cell mL-1 algae were filtered by 40 mm glass fiber filters (Whatman GF/C), which was ashed and pre-weighed, to determined the organic content of microalgae. The filter papers were rinsed with ammonium formate solution (0.5 M) and dried in the oven (110°C) for 24 h, the first weighing results were recorded. Another weight was measured after ashing the filter papers in a muffle furnace (450°C) for 6 h. The filters were cooled in desiccators in advance. Feces were collected by a pipet from the experimental tanks 8 h after the CR determinations, and the organic content of the feces was measured using the same method as above. AE was computed according to Conover (1966):
Where AE denotes the AE (%), F represents the ratio of organic dry weight: dry weight in the diets, and E is the ratio of organic dry weight: dry weight in the feces.
Ingestion rate (IR) was computed by multiplying CR by POM concentration (particulate organic matter, mg L-1, Hawkins et al., 1998), i.e., the amount of ingested organic food per hour. The POM concentration was transformed to joules using a conversion value of 23 J mg-1 for Chlorella spp (Widdows and Johnson, 1988; Widdows et al., 1990).
The RR of mussels was measured from the corresponding treatment tanks in closed glass respirometer (800 ml) containing air-saturated seawater. To make sure that every mussel was breathing normally, the experiment began after their valves had been open for 15 min and then sealed off the respirometers for 60 min. Two tanks filled only with seawater were used as the control. The decline in oxygen content was measured by an oxygen meter (model 5200A, YSI, United States). Then the initial and final oxygen concentrations of each tank were obtained.
The RR was then computed using the following equation (Wang et al., 2015):
Where RR demotes the RR (mg O2 h-1d final DO levels (mg O2 L-1), respectively, V (L) is the water volume in the respirometer, N is the mussel number, and t (h) is the exposure time. Values for RR were changed to J h-1 using a conversion value of 13.98 J mg O2-1 (Wong and Cheung, 2003).
Excretion rates (ER) were measured after measuring RR of the same mussels. Water samples from each tank were frozen to -20°C until the analysis. The concentration of ammonia was measured by spectrophotometry, referring to the method of phenol-sodium hypochlorite (Solorzano, 1969). ER was calculated on the basis of the difference in the concentration of ammonia in the experimental tank and the blank tank using the following equation (Wang et al., 2015):
where ER denotes the rate of ammonia excretion (mg NH4-N h-1 g-1), Ce is the ammonia concentration (mg L-1) in the experimental sample, Cc is the ammonia concentration (mg L-1) in the control, V is the seawater volume (ml) in the tank, N is the mussel number in the tank and t is the exposure time (h). ER values were changed to J h-1using the conversion value of 1mg NH4-N = 25 J (Elliott and Davison, 1975). The ratio of oxygen consumption to ammonia excretion (O:N) was calculated to reflect the proportion of protein relative to carbohydrates and lipids metabolized under different conditions (Widdows, 1985).
Scope for growth was computed by the energy balance equation given by Smaal and Widdows (1994):
Where SFG denotes SFG (J h-1g-1), Ab represents the total absorbed energy (J h-1 g-1), R is the energy lost for respiration (J h-1g-1), and U is the energy lost for ammonia excretion (J h-1g-1).
Absorption rate (Ab) = IR (J h-1) × AE (%).
The normality and homogeneity of the data were checked by Shapiro-Wilk’s test and Levene’s test (SPSS 19.0), respectively. The effects of pH, nano-ZnO and their interactions were analyzed by two-way analysis of variance (ANOVA). If there is an interaction, the significant effects of nano-ZnO were analyzed by one-way ANOVA at each fixed pH followed by Tukey’s HSD test. Student’s t-test was applied to examine the significant effects of pH at each nano-ZnO concentration. Principal component analysis (PCA) was conducted by XLSTAT®2014. The measured parameters and the observations were listed in a biplot. The significant difference level was considered as P < 0.05.
During the experiment, the salinity remained at 25.1 ± 0.3 psu, the water temperature remained at 20.1 ± 0.3°C, the normal pH was kept at 8.10 ± 0.02, and the low pH was kept at 7.69 ± 0.02 (Table 1). Moreover, Table 1 also summarized the carbonate chemical parameters of seawater for all treatments. All mussels were alive during the experimental period.
The nano-ZnO morphology was observed by both TEM and SEM. The particle diameter of ca. 15–25 nm and spheroid irregular shapes of nano-ZnO were found by TEM and SEM, respectively (Supplementary Figures S1A,B). From the X-ray diffraction patterns (Supplementary Figure S1C), all peaks of the nano-ZnO were well indexed to the hexagonal wurtzite structure of ZnO (JCPDS Card no. 01-089-0510), and there were no other impurity diffraction peaks in the spectrum, so the nanomaterials were pure nano-ZnO. DLS results showed that the particle size of nano-ZnO was affected by pH (Table 2).
CR varied from 1.1 to 4.0 L h-1 g-1 after the mussels were exposed to different treatments of pH and nano-ZnO for 14 days. High concentration of nano-ZnO resulted in a significant reduction of CR throughout the whole experiment. Low pH significantly reduced the CR of the thick shell mussels (p < 0.05) when the nano-ZnO was 10 mgL-1. CR was the lowest when the concentration of nano-ZnO was 10 mg L-1 under pH 7.7 (Figure 1A).
FIGURE 1. (A) Clearance rate (CR), (B) absorption efficiency (AE), (C) respiration rate (RR), (D) ammonia excretion rate (ER), (E) O:N ratio and (F) Scope for growth (SFG) of M. coruscus exposed to different combinations of pH and nano-ZnO for 14 days. The means denoted by different superscripts at each fixed pH are significantly different among three nano-ZnO concentrations at each sampling time (P < 0.05). The means sharing the asterisk between two pH levels at each fixed nano-ZnO are significantly different at each sampling time (P < 0.05).
The AE was low, ranging from 15 to 45%. High concentration of nano-ZnO significantly reduced the AE, but there was no significant difference between the two pH levels. During the 14-day experiment, AE was reduced to the lowest value when the concentration of nano-ZnO was 10 mg L-1 (Figure 1B).
During the experiment, RR was significantly affected by the interaction of nano-ZnO and pH (Table 3 and Figure 1C). Similar to CR, high nano-ZnO significantly reduced the RR of the mussels (p < 0.05). RR was significant reduced (p < 0.05) by low pH when the nano-ZnO is 10 mgL-1 except at day 14. The lowest value of measured RR at each time point was at pH 7.7 and nano-ZnO 10 mg L-1 (Figure 1C).
TABLE 3. Summary of two-way ANOVA results on effects of pH and nano-ZnO on clearance rate (CR), absorption efficiency (AE), respiration rate (RR), excretion rate (ER), O:N ratio, and scope for growth (SFG).
During the whole experiment, low pH significantly increased ER (p < 0.05), and ER was significantly increased with the increment of nano-ZnO concentrations except at day 1. Moreover, the highest values were observed when the mussels were subject to low pH and high nano-ZnO, and interactive effects of nano-ZnO and pH were found at day 14 (Table 3 and Figure 1D).
Nano-ZnO significantly reduced O: N ratio throughout the experiment, and low pH significantly decreased the O: N ratio compared to normal pH 8.1 when nano-ZnO was absent (p < 0.05), and some interactive effects were also observed (Table 3). The effects of both pH and nano-ZnO showed similar trends and resulted in a significant low value at low pH and high nano-ZnO (Figure 1E).
The SFG was significantly decreased (p < 0.05) under high nano-ZnO condition throughout the whole experiment. Moreover, the SFG displayed positive values for all treatments except for nano-ZnO 10 mg L-1 and pH 7.7 at day 14. Low pH significantly decreased the SFG (p < 0.05) when nano-ZnO was 2.5 and 10 mg L-1 at days 7 and 10 (Figure 1F), and the lowest SFG was observed under the combination of high nano-ZnO and low pH.
According to PCA, 93.27% of total variance was represented by the two principal components (Figure 2). PC1 accounted for 78.68% of total variance, the distinct response was the separation between zero concentration and high concentration of nano-ZnO, where the high levels of most physiological activities were observed, especially high levels of SFG associated with CR, O:N and RR under normal pH and 0 nano-ZnO conditions. PC2 representing 14.59% of total variance, differentiated normal pH from low pH exposed mussels, showing that high ER was correlated to low pH treatments.
FIGURE 2. Biplot originating from principal component analysis integrating all measured variables (CR, AE, RR, ER, O:N, SFG) for four sampling times (days: 1, 3, 7, and 14) at six different treatments (■-0 mgL-1 × pH 8.1, □–0mgL-1 × pH 7.7, ♦-2.5 mgL-1 × pH 8.1, ♢-2.5 mgL-1 × pH 7.7 ▲-10 mgL-1 × pH 8.1, Δ-10 mgL-1 × pH 7.7). Both the loadings of the variables (•) and the scores of the experimental conditions were shown.
It was reported that pH has a significant effect on the scattering behavior of ZnO nanoparticles (Bian et al., 2011), and the physical and chemical properties of nanoparticles can be affected by low pH value, thus promoting the polymerization behavior (Huang et al., 2016). The DLS results showed that nano-ZnO can agglomerate to form larger particles at low pH compared to pH 8.1. In terms of the feeding characteristics of mussels, they can ingest more nano-ZnO in the form of aggregation during acidification conditions, resulting in more accumulation of nano-ZnO in the body and leading greater biological toxicity.
From the experimental results, the combination of seawater acidification and nano-ZnO had a direct impact on the physiology of M. coruscus, and some interactive effects were observed. It was found that 14-d nano-ZnO exposure led to significant low CR, RR, AE, SFG, and O: N ratio, but high ER in the thick shell mussel, while the low pH had less effect on the mussels compared to high nano-ZnO. According to the existing research reports, the effect of decreased pH on CR of bivalves is different. Sui et al. (2016) found that the CR of M. coruscus was significantly reduced at low pH. Liu and He (2012) found that low pH resulted in decreased clearance of Chlamys nobilis and Perna viridis. However, low pH made the CR of the pearl oyster Pinctada martensi increased while the filtration activities of the mussel M. galloprovincialis were not affected by the pH reduction (Fernández-Reiriz et al., 2012). For NPs effects, nano-TiO2 exposure reduced CR of M. coruscus (Hu et al., 2017). CR of M. galloprovincialis increased with nano-CeO2 concentration but decreased over time in groups exposed to nano-CeO2 (Conway et al., 2014). In addition, Doyle et al. (2016) found that the adsorption of nano-TiO2 on the gill surface in mussels and oysters resulted in sub-lethal effects, causing gill proliferation and edema and thus affected the respiratory function, damaged mussel filtration function and food intake. In this experiment, it was speculated that nano-ZnO damaged the function of gills of the mussels, thus reducing the CR.
The results showed that the AE of M. coruscus was not affected by low pH. Navarro et al. (2013) found that low pH significantly reduced the AE of M. chilensis. However, at low pH conditions, M. galloprovincialis can show high AE values (Fernández-Reiriz et al., 2012). It is speculated that the low pH may increase the activity of certain digestive enzymes of M. galloprovincialis to promote the nutrient uptake (Areekijseree et al., 2004). From the above results, it can be seen that the effect of acidification on the digestion and absorption is different among species. NPs can enter digestive system and induce oxidative stress and lysosomal membrane changes in digestive gland of mussels (Canesi et al., 2012; Hull et al., 2013). Saggese et al. (2016) found a decreasing AE trend across the silver nanoparticles (AgNPs) concentration gradient in the mussel Brachidontes pharaonis. In the present study, high nano-ZnO significantly reduced AE of the mussels, which may be due to the accumulation of nano-ZnO in the digestive tube of mussels, causing severe stress responses and damage to mussel health. It can be inferred that nano-ZnO has a toxic effect on the digestive physiology of the thick shell mussel.
pH significantly reduced the RR of the mussels when nano-ZnO was 10 mgL-1, indicating the negative effect of acidification on mussels was enhanced under high nano-ZnO. Michaelidis et al. (2005) pointed out that seawater acidification significantly decreased the RR of the mussel M. galloprovincialis. Liu and He (2012) found that the RR of the noble scallop Chlamys nobilis was significantly reduced at pH 7.4. In contrast, researchers have pointed out that some species have a certain adaptability to seawater acidification, thus seawater acidification sometimes increases the metabolic efficiency in some species, such as the mussel M. galloprovincialis and the scallop Pecten maximus (Fernández-Reiriz et al., 2012; Sanders et al., 2013). Throughout the whole experiment, nano-ZnO always negatively affected the RR of the mussels. Due to the toxic effects of nano-ZnO on the gills, the respiration function may be impaired, and ultimately the RR was decreased. However, Hanna et al. (2013) found that after 12 weeks of exposure to nano-ZnO, RR of mussels increased with ZnO concentration, indicating that mussels may adapt nano-ZnO conditions if they were exposed for long term. Muller et al. (2014) confirmed that nano-ZnO accumulated in tissues could impair the RR of M. galloprovincialis.
The ER can be used as an ideal indicator of stress in mussels (Fernández-Reiriz et al., 2011). It is presumed that the stress response of the mussels to acidification leads to an increase in ER. In this study, high nano-ZnO and low pH increased the ER in M. coruscus. There were similar studies made by Thomsen and Melzner (2010) who pointed out that with the increase in pCO2, the ER of M. edulis was increased, while Michaelidis et al. (2005) found the ER of M. galloprovincialis was significantly reduced under acidic conditions. If the oyster Pinctada mazatlanica was exposed to high temperature, the majority of feces is amino acid catabolism products (Saucedo et al., 2004), indicating an amino acid catabolism under stress conditions. It was found that higher ER values under low pH were observed when NPs were present (Hu et al., 2017). In this study, the increase in ER may indicate a sharp increase in amino acid catabolism of the mussels exposed to high nano-ZnO and low pH. Therefore, it can be concluded that high nano-ZnO and low pH can affect the physiological metabolism of M. coruscus.
It is known that normal O:N for molluscs usually is higher than 30 (Fernández-Reiriz et al., 2011). When the amino acid metabolism increases (lack of food or environmental stress) the O:N values may be less than 30 (Fernández-Reiriz et al., 2011). During the whole experiment, O:N ratio was in the range of 4–28, and it was found that the O:N ratio of M. coruscus was negatively affected by high nano-ZnO and low pH. It is therefore speculated that high nano-ZnO and low pH can increase the protein metabolic rate of mussels.
Scope for growth is an important indicator for the impact of environmental stress on mussel physiology (Navarro et al., 2013). In the present study, when M. coruscus was exposed to high nano-ZnO, the SFG value became negative on day 14, which may be due to a significant decrease in CR, since most of the other parameters measured can be affected by the reduced filtration activity of mussels and the time spent on eating or breathing. In addition, high concentration of nano-ZnO significantly reduced the SFG, showing that nano-ZnO obviously damage the growth of M. coruscus. There was a similar study made by Hu et al. (2017) who pointed out that the NPs could significantly reduce SFG of M. coruscus. Acidification also resulted in a decreased SFG of the thick shell mussel at days 7 and 14 when nano-ZnO was 2.5 and 10 mg L-1. Similarly some studies found that long-term acidification exposure significantly reduced the growth of M. galloprovincialis and Crassostrea virginica (Michaelidis et al., 2005; Berge et al., 2006; Beniash et al., 2010). Navarro et al. (2013) pointed out that high levels of pCO2 in seawater had a negative impact on the health of M. galloprovincialis. Duarte et al. (2014) showed that elevated carbon dioxide concentrations had a negative effect on calcium deposition and the weight of M. chilensis. However, Fernández-Reiriz et al. (2011) found acidification caused elevated SFG of M. galloprovincialis, indicating the tolerance to acidification is species specific and even different within species.
The PCA separated non-nano-ZnO treatments from exposed treatments since non- nano-ZnO treatments were at positive side whereas exposed treatments were at negative side by PC1. Under non- nano-ZnO treatments, there are higher values of AE, SFG, RR, O:N, and CR. PC2 reflected pH change of the experiment, as high values of ER was positive, corresponding to the low pH treatments. According to ANOVA and PCA results, the lower CR, RR, AE, O:N ratio and SFG, and higher ER were associated with nano-ZnO exposure treatments. In this study, CR, RR, SFG and O:N ration had positive correlations, if CR, RR and O:N were reduced, SFG was also reduced (Figure 2). In addition, higher ER under high nano-ZnO and low pH indicated the low absorption rate and high protein catabolism, which was harmful to the growth of mussels.
The impact of high nano-ZnO exposure was greater than that of low pH, the physiological parameters of mussels were affected more severely by the combined stress of seawater acidification and high concentration of nano-ZnO, i.e., the low pH enhanced the toxicity of nano-ZnO to the M. coruscus. The results of this study provided new insights for future understanding of the effect of nanomaterials and ocean acidification on marine organisms.
WH and YW designed and led the study. MH, LL, YS, HK, and YL performed the experiments. YS, ZL, FW, and XH analyzed data. YS and YW wrote the manuscript. All authors reviewed the manuscript.
The work described in this paper was supported by a research grant (project no. 31302207) from the National Natural Science Foundation of China, a grant (project no. 17ZR1412900) from the Shanghai Municipal Natural Science Foundation, and sponsored by Shanghai Pujiang Program (18PJ1404000). This study was also supported by the open fund of Guangxi Key Laboratory of Aquatic Genetic Breeding and Healthy Aquaculture.
The authors declare that the research was conducted in the absence of any commercial or financial relationships that could be construed as a potential conflict of interest.
The Supplementary Material for this article can be found online at: https://www.frontiersin.org/articles/10.3389/fphys.2018.00757/full#supplementary-material
Areekijseree, M., Engkagul, A., Kovitvadhi, U., Thongpan, A., Mingmuang, M., Pakkong, P., et al. (2004). Temperature and pH characteristics of amylase and proteinase of adult freshwater pearl mussel, Hyriopsis (Hyriopsis) bialatus Simpson 1900. Aquaculture 234, 575–587. doi: 10.1016/j.aquaculture.2003.12.008
Bayne, B. L., Moore, M. N., Widdows, J., Livingstone, D. R., Salkeld, P., and Crisp, D. J. (1979). The assessment of sublethal effects of pollutants in the sea - Measurement of the responses of individuals to environmental stress and pollution: studies with bivalve molluscs. Philos. Trans. R. Soc. Lond. B 286, 563–581. doi: 10.1098/rstb.1979.0046
Bayne, B. L., and Widdows, J. (1978). The physiological ecology of two populations of Mytilus edulis L. Oecologia 37, 137–162. doi: 10.1007/BF00344987
Beniash, E., Ivanina, A., Lieb, N. S., Kurochkin, I., and Sokolova, I. M. (2010). Elevated level of carbon dioxide affects metabolism and shell formation in oysters Crassostrea virginica. Mar. Ecol. Prog. Ser. 419, 95–108. doi: 10.3354/meps08841
Berge, J. A., Bjerkeng, B., Pettersen, O., Schaanning, M. T., and Øxnevad, S. (2006). Effects of increased sea water concentrations of CO2 on growth of the bivalve Mytilus edulis L. Chemosphere 62, 681–687. doi: 10.1016/j.chemosphere.2005.04.111
Bian, S. W., Mudunkotuwa, I. A., Rupasinghe, T., and Grassian, V. H. (2011). Aggregation and dissolution of 4 nm ZnO nanoparticles in aqueous environments: influence of pH, ionic strength, size, and adsorption of humic acid. Langmuir 27, 6059–6068. doi: 10.1021/la200570n
Caldeira, K., and Wickett, M. E. (2003). Oceanography: anthropogenic carbon and ocean pH. Nature 425, 365–365. doi: 10.1038/425365a
Canesi, L., Ciacci, C., Fabbri, R., Marcomini, A., Pojana, G., and Gallo, G. (2012). Bivalve molluscs as a unique target group for nanoparticle toxicity. Mar. Environ. Res. 76, 16–21. doi: 10.1016/j.marenvres.2011.06.005
Ciacci, C., Canonico, B., Bilaniĉovã, D., Rita, F., Katia, C., Gabriella, G., et al. (2012). Immunomodulation by different types of n-oxides in the hemocytes of the marine bivalve Mytilus galloprovincialis. PLoS One 7:e36937. doi: 10.1371/journal.pone.0036937
Conover, R. J. (1966). Assimilation of organic matter by zooplankton. Limnol. Oceanogr. 11, 338–345. doi: 10.4319/lo.1966.11.3.0338
Conway, J. R., Hanna, S. K., Lenihan, H. S., and Keller, A. A. (2014). Effects and implications of trophic transfer and accumulation of CeO2 nanoparticles in a marine mussel. Environ. Sci. Technol. 48, 1517–1524. doi: 10.1021/es404549u
Coughlan, J. (1969). The estimation of filtering rate from the clearance of suspensions. Mar. Biol. 2, 356–358. doi: 10.1007/bf00355716
Doyle, J. J., Ward, J. E., and Mason, R. (2016). Exposure of bivalve shellfish to titania nanoparticles under an environmental-spill scenario: encounter, ingestion and egestion. J. Mar. Biol. Assoc. U.K. 96, 137–149. doi: 10.1017/S0025315415001174
Duarte, C., Navarro, J. M., Acuña, K., Torres, R., Manríquez, P. H., and Lardies, M. A. (2014). Combined effects of temperature and ocean acidification on the juvenile individuals of the mussel Mytilus chilensis. J. Sea Res. 85, 308–314. doi: 10.1016/j.seares.2013.06.002
Elliott, J. M., and Davison, W. (1975). Energy equivalents of oxygen consumption in animal energetics. Oecologia 19, 195–201. doi: 10.1007/bf00345305
Fabry, V. J., Seibel, B. A., Feely, R. A., and Orr, J. C. (2008). Impacts of ocean acidification on marine fauna and ecosystem processes. ICES J. Mar. Sci. 65, 414–432. doi: 10.1093/icesjms/fsn048
Feely, R. A., Sabine, C. L., Lee, K., Berelson, W., Kleypas, J., Fabry, V. J., et al. (2004). Impact of anthropogenic CO2 on the CaCO3 system in the oceans. Science 305, 362–366. doi: 10.1126/science.1097329
Fernández-Reiriz, M. J., Range, P., Álvarez-Salgado, X. A., Espinosa, J., and Labarta, U. (2012). Tolerance of juvenile Mytilus galloprovincialis to experimental seawater acidification. Mar. Ecol. Prog. Ser. 454, 65–74. doi: 10.3354/meps09660
Fernández-Reiriz, M. J., Range, P., Álvarez-Salgado, X. A., and Labarta, U. (2011). Physiological energetics of juvenile clams Ruditapes decussatus in a high CO2 coastal ocean. Mar. Ecol. Prog. Ser. 433, 97–105. doi: 10.3354/meps09062
Foy, R. H., Lennox, S. D., and Smith, R. V. (2001). Assessing the effectiveness of regulatory controls on farm pollution using chemical and biological indices of water quality and pollution statistics. Water Res. 35, 3004–3012. doi: 10.1016/S0043-1354(00)00587-X
Gagné, F., Auclair, J., Trépanier, S., Turcotte, P., Pilote, M., and Gagnon, C. (2016). The impact of zinc oxide nanoparticles in freshwater mussels exposed to municipal effluents. Invert. Surv. J. 13, 281–290.
Gazeau, F., Parker, L. M., Comeau, S., Gattuso, J. P., O’Connor, W. A., Martin, S., et al. (2013). Impacts of ocean acidification on marine shelled molluscs. Mar. Biol. 160, 2207–2245. doi: 10.1007/s00227-013-2219-3
Halldórsson, H. P., De Pirro, M., Romano, C., Svavarsson, J., and Sarà, G. (2008). Immediate biomarker responses to benzo [a] pyrene in polluted and unpolluted populations of the blue mussel (Mytilus edulis L.) at high-latitudes. Environ. Int. 34, 483–489. doi: 10.1016/j.envint.2007.11.002
Hanna, S. K., Miller, R. J., Muller, E. B., Nisbet, R. M., and Lenihan, H. S. (2013). Impact of engineered zinc oxide nanoparticles on the individual performance of Mytilus galloprovincialis. PLoS One 8:e61800. doi: 10.1371/journal.pone.0061800
Hao, L., and Chen, L. (2012). Oxidative stress responses in different organs of carp (Cyprinus carpio) with exposure to ZnO nanoparticles. Ecotoxicol. Environ. Saf. 80, 103–110. doi: 10.1016/j.ecoenv.2012.02.017
Hawkins, A. J. S., Bayne, B. L., Bougrier, S., Héral, M., Iglesias, J. I. P., Navarro, E., et al. (1998). Some general relationships in comparing the feeding physiology of suspension-feeding bivalve molluscs. J. Exp. Mar. Biol. Ecol. 219, 87–103. doi: 10.1016/S0022-0981(97)00176-7
Hu, M., Li, L., Sui, Y., Li, J., Wang, Y., Lu, W., et al. (2015). Effect of pH and temperature on antioxidant responses of the thick shell mussel Mytilus coruscus. Fish Shellfish Immunol. 46, 573–583. doi: 10.1016/j.fsi.2015.07.025
Hu, M., Lin, D., Shang, Y., Hu, Y., Lu, W., Huang, X., et al. (2017). CO2-induced pH reduction increases physiological toxicity of nano-TiO2 in the mussel Mytilus coruscus. Sci. Rep. 7:40015. doi: 10.1038/srep40015
Huang, X., Lin, D., Ning, K., Sui, Y., Hu, M., Lu, W., et al. (2016). Hemocyte responses of the thick shell mussel Mytilus coruscus exposed to nano-TiO2 and seawater acidification. Aquat. Toxicol. 180, 1–10. doi: 10.1016/j.aquatox.2016.09.008
Hull, M. S., Vikesland, P. J., and Schultz, I. R. (2013). Uptake and retention of metallic nanoparticles in the Mediterranean mussel (Mytilus galloprovincialis). Aquat. Toxicol. 140, 89–97. doi: 10.1016/j.aquatox.2013.05.005
Intergovernmental Panel on Climate Change [IPCC] (2014). Climate Change 2014: Synthesis Report. Contribution of Working Groups I, II and III to the Fifth Assessment Report of the Intergovernmental Panel on Climate Change. Geneva: IPCC. doi: 10.1017/CBO9781107415416
Jakubowska, M., and Normant, M. (2015). Metabolic rate and activity of blue mussel Mytilus edulis trossulus under short-term exposure to carbon dioxide-induced water acidification and oxygen deficiency. Mar. Freshw. Behav. Physiol. 48, 25–39. doi: 10.1080/10236244.2014.986865
Jansson, A., Norkko, J., Dupont, S., and Norkko, A. (2015). Growth and survival in a changing environment: combined effects of moderate hypoxia and low pH on juvenile bivalve Macoma balthica. J. Sea Res. 102, 41–47. doi: 10.1016/j.seares.2015.04.006
Kroeker, K. J., Kordas, R. L., Crim, R. N., and Singh, G. G. (2010). Meta-analysis reveals negative yet variable effects of ocean acidification on marine organisms. Ecol. Lett. 13, 1419–1434. doi: 10.1111/j.1461-0248.2010.01518.x
Lannig, G., Eilers, S., Pörtner, H. O., Sokolova, I. M., and Bock, C. (2010). Impact of ocean acidification on energy metabolism of oyster, Crassostrea gigas-changes in metabolic pathways and thermal response. Mar. Drugs 8, 2318–2339. doi: 10.3390/md8082318
Lewis, C., Clemow, K., and Holt, W. V. (2013). Metal contamination increases the sensitivity of larvae but not gametes to ocean acidification in the polychaete Pomatoceros lamarckii (Quatrefages). Mar. Biol. 160, 2089–2101. doi: 10.1007/s00227-012-2081-8
Li, H. M., Shi, X. Y., Chen, P., and Zhang, C. S. (2014). Effects of pH and DO on the migration and transformation of phosphate in the process of mixing in the Changjiang Estuary. Mar. Environ. Sci. 33, 497–502. doi: 10.13634/j.cnki.mes.2014.04.001
Liu, H. H., He, J. Y., Zhao, R. T., and Xue, C. B. (2014). Molecular expression pattern of glutathione S-transferase gene in Mytilus coruscus exposed to heavy metals. Oceanol. Limnol. Sin. 45, 275–279.
Liu, W., and He, M. (2012). Effects of ocean acidification on the metabolic rates of three species of bivalve from southern coast of China. Chin. J. Oceanol. Limnol. 30, 206–211. doi: 10.1007/s00343-012-1067-1
Matoo, O. B., Ivanina, A. V., Ullstad, C., Beniash, E., and Sokolova, I. M. (2013). Interactive effects of elevated temperature and CO2 levels on metabolism and oxidative stress in two common marine bivalves (Crassostrea virginica and Mercenaria mercenaria). Comp. Biochem. Physiol. Part A 164, 545–553. doi: 10.1016/j.cbpa.2012.12.025
Melzner, F., Gutowska, M. A., Langenbuch, M., Dupont, S., Lucassen, M., Thorndyke, M. C., et al. (2009). Physiological basis for high CO2 tolerance in marine ectothermic animals: pre-adaptation through lifestyle and ontogeny? Biogeosciences 6, 2313–2331. doi: 10.5194/bg-6-2313-2009
Michaelidis, B., Ouzounis, C., Paleras, A., and Pörtner, H. O. (2005). Effects of long-term moderate hypercapnia on acid–base balance and growth rate in marine mussels Mytilus galloprovincialis. Mar. Ecol. Prog. Ser. 293, 109–118. doi: 10.3354/meps293109
Montes, M. O., Hanna, S. K., Lenihan, H. S., and Keller, A. A. (2012). Uptake, accumulation, and biotransformation of metal oxide nanoparticles by a marine suspension-feeder. J. Hazard. Mater. 225, 139–145. doi: 10.1016/j.jhazmat.2012.05.009
Muller, E. B., Hanna, S. K., Lenihan, H. S., Miller, R. J., and Nisbet, R. M. (2014). Impact of engineered zinc oxide nanoparticles on the energy budgets of Mytilus galloprovincialis. J. Sea Res. 94, 29–36. doi: 10.1016/j.seares.2013.12.013
Navarro, J. M., Torres, R., Acuña, K., Duarte, C., Manriquez, P. H., Lardies, M., et al. (2013). Impact of medium-term exposure to elevated pCO2 levels on the physiological energetics of the mussel Mytilus chilensis. Chemosphere 90, 1242–1248. doi: 10.1016/j.chemosphere.2012.09.063
Orr, J. C., Fabry, V. J., Aumont, O., Bopp, L., Doney, S. C., Feely, R. A., et al. (2005). Anthropogenic ocean acidification over the twenty-first century and its impact on calcifying organisms. Nature 437, 681–686. doi: 10.1038/nature04095
Reeburgh, W. S. (2007). Oceanic methane biogeochemistry. Chem. Rev. 107, 486–513. doi: 10.1021/cr050362v
Renzi, M., and Guerranti, C. (2015). Ecotoxicity of nanoparticles in aquatic environments: a review based on multivariate statistics of meta-data. J. Environ. Anal. Chem. 2:149. doi: 10.4172/2380-2391.1000149
Sabine, C. L., Feely, R. A., Gruber, N., Key, R. M., Lee, K., Bullister, J. L., et al. (2004). The oceanic sink for anthropogenic CO2. Science 305, 367–371. doi: 10.1126/science.1097403
Saggese, I., Sarà, G., and Dondero, F. (2016). Silver nanoparticles affect functional bioenergetic traits in the invasive red sea Mussel Brachidontes pharaonis. BioMed. Res. Int. 2016:1872351. doi: 10.1155/2016/1872351
Sanders, M. B., Bean, T. P., Hutchinson, T. H., and Le Quesne, W. J. (2013). Juvenile king scallop, Pecten maximus, is potentially tolerant to low levels of ocean acidification when food is unrestricted. PLoS One 8:e74118. doi: 10.1371/journal.pone.0074118
Sarà, G., Romano, C., Widdows, J., and Staff, F. J. (2008). Effect of salinity and temperature on feeding physiology and scope for growth of an invasive species (Brachidontes pharaonis-Mollusca: Bivalvia) within the Mediterranean Sea. J. Exp. Mar. Biol. Ecol. 363, 130–136. doi: 10.1016/j.jembe.2008.06.030
Saucedo, P. E., Ocampo, L., Monteforte, M., and Bervera, H. (2004). Effect of temperature on oxygen consumption and ammonia excretion in the Calafia mother-of-pearl oyster, Pinctada mazatlanica (Hanley, 1856). Aquaculture 229, 377–387. doi: 10.1016/S0044-8486(03)00327-2
Smaal, A. C., and Widdows, J. (1994). “The scope for growth of bivalves as an integrated response parameter in biological monitoring,” in Biomonitoring of Coastal Waters and Estuaries, ed. K. J. M. Kramer (Boca Raton, FL: CRC Press), 247–268.
Solorzano, L. (1969). Determination of ammonia in natural waters by the phenolhypochlorite method. Limnol. Oceanogr. 14, 799–801. doi: 10.4319/lo.1969.14.5.0799
Stumpp, M., Hu, M., Casties, I., Saborowski, R., Bleich, M., Melzner, F., et al. (2013). Digestion in sea urchin larvae impaired under ocean acidification. Nat. Climate Change 3, 1044–1049. doi: 10.1038/NCLIMATE2028
Sui, Y., Kong, H., Huang, X., Dupont, S., Hu, M., Storch, D., et al. (2016). Combined effects of short-term exposure to elevated CO2 and decreased O2 on the physiology and energy budget of the thick shell mussel Mytilus coruscus. Chemosphere 155, 207–216. doi: 10.1016/j.chemosphere.2016.04.054
Thomsen, J., and Melzner, F. (2010). Moderate seawater acidification does not elicit long-term metabolic depression in the blue mussel Mytilus edulis. Mar. Biol. 157, 2667–2676. doi: 10.1007/s00227-010-1527-0
Tian, X., Ju, M., Shao, C., and Fang, Z. (2011). Developing a new grey dynamic modeling system for evaluation of biology and pollution indicators of the marine environment in coastal areas. Ocean Coast. Manag. 54, 750–759. doi: 10.1016/j.ocecoaman.2011.08.003
Wang, S. R., Yin, K. D., Cai, W. J., and Wang, D. X. (2012). Advances in studies of ecological effects of ocean acidification. Acta Ecol. Sin. 32, 5859–5869. doi: 10.5846/stxb201108131184
Wang, Y., Li, L., Hu, M., and Lu, W. (2015). Physiological energetics of the thick shell mussel Mytilus coruscus exposed to seawater acidification and thermal stress. Sci. Total Environ. 514, 261–272. doi: 10.1016/j.scitotenv.2015.01.092
Wang, Y. J., Li, L. S., Li, Q. Z., and Lü, W. Q. (2014). Research progress on eco-physiological responses of shellfish under ocean acidification and global warming. Acta Ecol. Sin. 34, 3499–3508. doi: 10.5846/stxb201306301803
Widdows, J. (1985). “The effects of stress and pollution on marine animals,” in Physiological Measurements, eds B. L. Bayne, D. A. Brown, K. Burns, D. R. Dixon, A. Ivañovici, D. R. Livingstone, et al. (New York, NY: Praeger Scientific Publications), 3–40.
Widdows, J., Burns, K. A., Menon, N. R., Page, D. S., and Soria, S. (1990). Measurement of physiological energetics (scope for growth) and chemical contaminants in mussels (Arca zebra) transplanted along a contamination gradient in Bermuda. J. Exp. Mar. Biol. Ecol. 138, 99–117. doi: 10.1016/0022-0981(90)90179-g
Widdows, J., Donkin, P., Staff, F. J., Matthiessen, P., Law, R. J., Allen, Y. T., et al. (2002). Measurement of stress effects (scope for growth) and contaminant levels in mussels (Mytilus edulis) collected from the Irish Sea. Mar. Environ. Res. 53, 327–356. doi: 10.1016/S0141-1136(01)00120-9
Widdows, J., and Hawkins, A. J. S. (1989). Partitioning of rate of heat dissipation by Mytilus edulis into maintenance, feeding, and growth components. Physiol. Zool. 62, 764–784. doi: 10.1086/physzool.62.3.30157926
Widdows, J., and Johnson, D. (1988). Physiological energetics of Mytilus edulis: scope for growth. Mar. Ecol. Prog. Ser. 46, 113–121. doi: 10.3354/meps046113
Widdows, J., and Staff, F. (2006). Biological effects of contaminants: measurement of scope for growth in mussels. Copenhagen 40, 1–30.
Wong, W. H., and Cheung, S. G. (2003). Seasonal variation in the feeding physiology and scope for growth of green mussels, Perna viridis in estuarine Ma Wan, Hong Kong. J. Mar. Biol. Assoc. U.K. 83, 543–552. doi: 10.1017/S002531540300746Xh
Keywords: Nano-ZnO, acidification, Mytilus coruscus, physiology, combined effects
Citation: Shang Y, Lan Y, Liu Z, Kong H, Huang X, Wu F, Liu L, Hu M, Huang W and Wang Y (2018) Synergistic Effects of Nano-ZnO and Low pH of Sea Water on the Physiological Energetics of the Thick Shell Mussel Mytilus coruscus. Front. Physiol. 9:757. doi: 10.3389/fphys.2018.00757
Received: 19 March 2018; Accepted: 30 May 2018;
Published: 19 June 2018.
Edited by:
Francesca Carella, Università degli Studi di Napoli Federico II, ItalyReviewed by:
Rui Rosa, Universidade de Lisboa, PortugalCopyright © 2018 Shang, Lan, Liu, Kong, Huang, Wu, Liu, Hu, Huang and Wang. This is an open-access article distributed under the terms of the Creative Commons Attribution License (CC BY). The use, distribution or reproduction in other forums is permitted, provided the original author(s) and the copyright owner are credited and that the original publication in this journal is cited, in accordance with accepted academic practice. No use, distribution or reproduction is permitted which does not comply with these terms.
*Correspondence: Wei Huang, d2lsbGh1YW5nQHNpby5vcmcuY24= Youji Wang, eW91aml3YW5nMkBnbWFpbC5jb20=
†These authors have contributed equally to this work.
Disclaimer: All claims expressed in this article are solely those of the authors and do not necessarily represent those of their affiliated organizations, or those of the publisher, the editors and the reviewers. Any product that may be evaluated in this article or claim that may be made by its manufacturer is not guaranteed or endorsed by the publisher.
Research integrity at Frontiers
Learn more about the work of our research integrity team to safeguard the quality of each article we publish.