- Department of Chemical, Biological, Pharmacological and Environmental Sciences, University of Messina, Messina, Italy
Gastrointestinal function in vertebrates is influenced by stressors, such as fasting and refeeding, different types of diet and hormonal factors. The aim of this paper was to analyze the effect of a Spirulina (Arthrospira platensis) diet, a microalga known for its nutraceutical properties, on the gastrointestinal tract of zebrafish (Danio rerio) regarding expression of oligopeptide transporter 1 (PepT1) and ghrelin (GHR). Food deprivation and refeeding was investigated to elucidate expression of PepT1 and GHR at a gastrointestinal level and the zebrafish compensatory mechanism. PepT1 is responsible for absorbing di- and tripeptides through a brush border membrane of intestinal mucosa. GHR is a brain-gut peptide in fish and mammals, stimulating growth hormone secretion and regulating appetite. Samples were taken after 2 and 5 days of specimen fasting, and 2 and 5 days of refeeding with Sera Spirulina tabs, in which the major constituent is Spirulina sp. (50.2% protein). Morphological and immunohistochemical analysis of PepT1 and GHR were carried out. Control specimen intestinal tract showed normal morphology of the digestive tract. Fasting caused fold structural changes and intestinal lumen constriction. Immunohistochemical analysis showed a PepT1 level reduction after fasting and an increase after refeeding, reaching very high levels after 5 days, compared to controls. GHR levels increased after food deprivation and gradually decreased after refeeding. Increased expression of PepT1 in refeeding fish suggests a compensatory physiological mechanism, as does the increase in GHR levels in fasting fish followed by a reduction after refeeding. A compensatory mechanism may be induced by fasting and refeeding and by a higher protein Spirulina diet. The microalga, for its nutraceutical properties, is an excellent candidate for animal breeding and human diet.
Introduction
It is well known that teleosts in captive conditions are subject to stress phenomena, which may be due to illness or other factors, such as handling, transport, thermal variations, inadequate nutrition, exposure to periods of fasting or different types of diet and harmful agents. Several studies have documented how certain experimental procedures can influence gastrointestinal morphological integrity (Krogdahl and Bakke-McKellep, 2005; Denaro et al., 2006; Lo Cascio et al., 2017), metabolism, change of the enzymatic patterns (Caruso et al., 2011, 2012, 2014; Abolfathi et al., 2012), regulation of gastrointestinal hormones, and peptides transport (Jonsson et al., 2006; Olsson et al., 2008). Studies in zebrafish (Danio rerio, Hamilton, 1822), based on short term food deprivation and re-feeding to evaluate mRNA expression of the oligopeptide transporter (PepT1) and cholecystokinin (CCK), gastrin-releasing peptide (GRP) and ghrelin (GHR), showed that with successive refeeding PepT1 mRNA expression increased reaching values 8 times higher than pre-fasting levels (Koven and Schulte, 2012). PepT1 is a hydrogen ion/peptide cotransporter; it transports oligopeptides but cannot transport peptides with more than three amino acid residues or free amino acids. Its transport action requires proton binding and a negative membrane potential. PepT1 is a 708-amino acid protein with 12 putative membrane-spanning domains. It can absorb a part of ingested protein into the mucosa as di- and tripeptides. GHR is a 28-amino acid peptide, first identified and isolated from the stomach of rats (Kojima et al., 1999), which functions as a neuropeptide (Dickson et al., 2011). It is produced in the gastrointestinal tract by ghrelinergic cells (Sakata and Sakai, 2010) when the stomach is empty, conversely, secretion stops at full stomach. Besides regulating appetite, GHR also plays an important role in regulating energy distribution and rate (Burger and Berner, 2014). Its action on hypothalamic brain cells increases hunger, gastric acid secretion, and gastrointestinal motility (Nakazato et al., 2001; Shousha et al., 2005). It is highly conserved among species (Kaiya et al., 2011). Immunoreactivity of GHR was recognized in the GI tract and accessory organs of several species (Hayashida et al., 2002; Zhao and Sakai, 2008; Kasacka et al., 2014). GHR producing cells were found in the proventriculus, gizzard, and intestine of avians (Wada et al., 2003; Shao et al., 2010) and in stomach and intestinal epithelia of teleosts (Feng et al., 2013), as well as in molluscs (Ngernsoungnern and Ngernsoungnern, 2016). The GHR gene has been characterized in Carassius auratus (Unniappan et al., 2002), Oreochromis mossambicus (Kaiya et al., 2003), Danio rerio (Amole and Unniappan, 2009), and Thunnus orientalis (Suda et al., 2012). GHR mRNA is mainly expressed in fish stomach and intestine, but it has also been detected in the hypothalamus, spleen, and gill (Unniappan et al., 2002; Amole and Unniappan, 2009). Zebrafish (Danio rerio) is a research model also for studies on fish nutrition. The zebrafish gastrointestinal anatomy is similar to mammalian small intestine. In zebrafish wild habitats, its diet comprises zooplankton, insect larvae and algae. To maintain zooplankton and insect larvae in the laboratory is not easy, moreover, energy is lost when dried. For these reasons, over the last few decades there has been an increasing interest in the commercial production of food-grade macro- and micro-algae to support growth and increase performance of omnivorous fish (Stanley and Jones, 1976; Wallace et al., 2005; Hardy, 2007; Lawrence, 2007; Spence et al., 2007; Bertuccio, 2013; Marino et al., 2016; Rizzo et al., 2017). Spirulina (Arthrospira platensis), (Spirulinaceae, Cyanobacteria), one of the most commonly used microalgae in aquafeeds, appears to be a good candidate for feeding adult fish. Its use in cichlid farming, in a closed ecological recirculating aquaculture system (CERAS), has been suggested (Lu and Takeuchi, 2004; Guroy et al., 2011, 2012). Furthermore, there is strong scientific interest in Spirulina and its potential therapeutic health benefit as a commercial food supplement and source of potential pharmaceuticals. Spirulina show a unique nutrient blend that no single source can offer, containing several nutrients: amino acids, particularly leucine, valine, isoleucine; essential fatty acids, such as linoleic acid (LA) and γ-linolenic acid (GLA); phycobiliproteins, as phycocyanin and allophycocyanin; β-carotene; E and B-complex vitamins; minerals; and a number of unexplored bioactive compounds. Spirulina lacks cellulose cell walls and therefore is easy to digest (Nakagawa and Montgomery, 2007). The effects of this alga on fish have been investigated in several fish species, including tilapia, Oreochromis niloticus (Mustafa et al., 1997; Nandeesha et al., 1998; Takeuchi et al., 2002) white sturgeon, Acipenser transmontanus (Palmegiano et al., 2005) and rainbow trout, Oncorhynchus mykiss (Matty and Smith, 1978).
This study aims to evaluate the short-term effect of food deprivation and refeeding with a Spirulina diet, on gastrointestinal morphology, as well as on the immunohistochemical expression of PepT1 and hunger hormone GHR, in adult zebrafish (Danio rerio) to highlight a potential mechanism driving compensatory growth.
Materials and Methods
A total of 84 adult (10–12 g) zebrafish (Danio rerio), obtained from the Centre for Experimental Fish Pathology of Sicily (CISS), Department of Veterinary Sciences (in accordance with EU Dir 63/2010), were used in this study. To acclimatize to experimental conditions, specimens were stocked for 15 days in 0.4 m3 aquarium, filled with dechlorinated freshwater at 25–26°C, pH 7.8 and equipped with a circular water system. These water parameters were used in all trials. Photoperiodic regime was 12L:12D. Zebrafish were fed daily, each morning, with a commercial flake diet (SERA Vipagran, centesimal composition: crude protein 40.4%, crude fat 8.7%, crude fiber 3.4%, crude ash 4.0%, humidity 6.1%; additives per kg: Vit. A 37000 IU, Vit. B1 35 mg, Vit. B2 90 mg, Vit. C 550 mg, Vit. D3 1800 IU, Vit. E 120 mg).
This study used live fish for experimental purposes and was performed under authorization from the Italian Ministry of Health (Prot. CISS 51/2013, DGSAF0022072) according to EU 63/2010 Directive. The in vivo study was carried out at CISS, Establishment for Users recognized by the Italian Ministry of Health for experimental activity on aquatic organisms (according to IT D.L. 26/2014), at the Department of Veterinary Sciences. Fish were only fed with commercial pellet supplemented with algae. Water parameters and health status were checked daily. No deaths or pain were caused to fish. At the end of the experimental trial the fish were euthanatized using anesthetics tricaine methanesulfonate (MS-222).
For the experimental trials, fish were divided into 7 groups, each of 12 individuals (Table 1). The controls (CF) were fed with a commercial flake diet. Two groups were sacrificed after 2 days (F2) or 5 days (F5) of food deprivation; the remaining groups were refed following the same period of food deprivation as follows: two groups were refed ad libitum, twice daily, respectively, for 2 days (F2R2 and F5R2) and further two groups for 5 days (F2R5 and F5R5) with commercial Sera Spirulina tabs, in which Spirulina is the major constituent (centesimal composition of Sera micron manufacture declaration: protein 50.2%, fat 8.1%, moisture 5%, fiber 9.2%, ash 11.9%, and particle size 62 ± 20 μm).
The experimental protocol was in accordance with the guide for the care and use of laboratory animals and with the Helsinki declaration.
Digestive tracts were dissected under a binocular microscope for future evaluations. The gut was exported from each animal and was fixed in 10% paraformaldehyde in phosphate buffered saline (PBS) 0.1 mol/l (pH 7.4) for 24 h, dehydrated in graded ethanol, cleared in xylene, embedded in Paraplast (McCormick Scientific, St. Louis, MO, United States) and cut into 5 μm triple serial sections. The sections were stained with haematoxylin and eosin (H&E), alcian blue-periodic acid Schiff (AB-PAS; pH 2.5) and May-Grünwald-Giemsa (MGG). Sections were examined under a Zeiss Axioskop 2 plus microscope equipped with a Sony Digital Camera DSC-85.
To better describe cell morphology both immunoperoxidase and immunofluorescence methods were used.
Immunohistochemical investigations were carried out using indirect method of peroxidase with a primary antibody specific for peptide transporter 1 (anti-PepT1 H-235, Santa Cruz Biotechnology, Inc.) and ghrelin (Anti-Ghrelin IN, Z-FishTM, AnaSpec). Serial sections were incubated with 0.3% H2O2 in PBS pH = 7.4 for 30 min to eliminate endogenous peroxidase activity. They were then rinsed and normal goat antiserum (1:20; Sigma, St. Louis, MO, United States) was added for 60 min. Sections were rinsed and incubated with a primary antibody anti-PepT1 (1:100) and anti-Ghrelin (1:10.000), in a moist chamber at 4°C for 12 h. Following this, the sections were rinsed and incubated for 2 h at room temperature in goat and anti-rabbit immunoglobulins (IgG) peroxidase (HRP) conjugated (1:50; Sigma, St. Louis, MO, United States). The sections were incubated for 1–5 min at room temperature in a 0.02% diaminobenzidine solution (DAB) and 0.015% hydrogen peroxide to visualized peroxidase activity. After rinsing in PBS, sections were dehydrated, mounted, and examined under an Axiophot Zeiss microscope (Carl Zeiss MicroImaging GmbH, Germany) equipped with a Sony Digital Camera DSC-85.
For the immunofluorescence method, serial sections were deparaffinised and rehydrated, rinsed several times in PBS and blocked in 10% normal goat serum for 1 h. Primary antibody anti-PepT1 and anti-Ghrelin were diluted in a permeabilizing solution (PBS, 0.2% Triton X-100, and 0.1% sodium azide) 1:100 and 1:10,000, respectively, placed on slides and incubated overnight in a moist chamber for 12 h at 4°C. Sections were then treated with fluorescent labeled secondary antibody diluted in PBS goat-anti-rabbit conjugated with the Rhodamine Red, Alexa 568 Fluor Dye (Molecular Probes, Invitrogen, Eugene, OR, United States; 1:100) for PepT1 and goat anti-rabbit conjugated with Fluorescent Isothiocyanate (FITC) (Cedarlane Laboratories, Burlington, ON, Canada; 1:50) for ghrelin, and left at room temperature for 2 h in the dark. After rinsing, the sections were mounted with Vectashield (Vector Labs, Burlingame, CA, United States) to prevent photo bleaching, and coverslipped. Control experiments were performed excluding primary antibody.
Results
Fish fed with Sera Spirulina tabs displayed a high survival rate (98%). This diet provided all the necessary components for promoting zebrafish wellness: color, size, quality, as well as attractiveness and availability.
The zebrafish has an anterior intestine, called the intestinal bulb, the lumen of which is larger than the lumen of the posterior section, which, similar to a stomach, may function as a reservoir. Intestinal crypts lack a gut epithelial layer; however, folds are present and decreased in size from the anterior to the posterior portion. Absorptive enterocytes (differentiated epithelial cells) (anterior and mid-intestine), mucin-producing goblet cells (entire intestine), and enteroendocrine cells (anterior intestine) were found. Cross-sections (6 μm) of the intestinal segments of control samples, observed with Galgano triple-staining, AB-PAS (Figure 1), revealed a simple architecture of the digestive tract. The smooth muscle layer and blood capillaries were directly linked to the mucosa, as the submucosa appeared thinned. Zebrafish enteric nerve cell bodies were present between the smooth muscle layers.
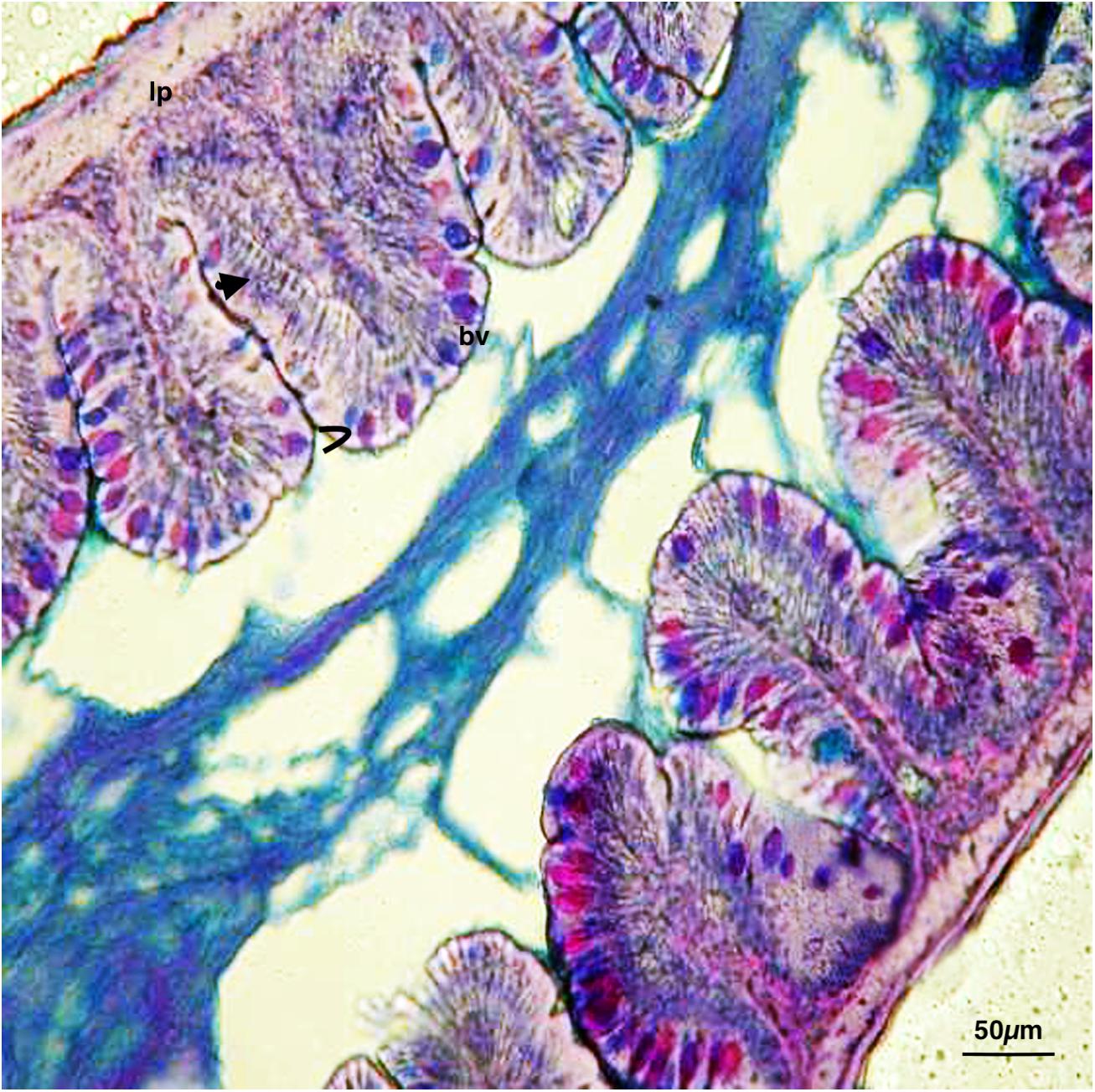
FIGURE 1. Digestive tract of zebrafish control: Continuous feeding, AB-PAS. , enterocytes; >, goblet cells; bv, blood vessel; lp, lamina propria. Scale bars: 50 μm.
After the 2- and 5-day fasting periods, folds of intestinal mucosa became smaller or fewer and in some sections, linked by anastomosis, obliterated the intestinal lumen. Mucous-secreting goblet cells were decreased in size, number, and intensity. Observations on cross sections of the intestine of specimens refeeding after 2 and 5 days, allowed to reveal the gradual recovery of the fold morphostructure and of the intestinal lumen, respectively. Marked alcianophilia and PAS positivity in the cells of the intestinal mucosa of the samples after 2 days and even more after 5 days of fasting were revealed.
Immunohistochemical investigation in peroxidase (HRP) and rhodamine-fluorescence, performed with the use of antibody for PepT1 (Figure 2), revealed weak immunopositivity in enteroendocrine cells of control samples. After 2 days fasting, immunopositivity decreased compared to controls, increasing after 2 days and even more after 5 days of refeeding with Spirulina. Similar results were observed after fasting for 5 days, and a marked immunopositivity was detected after 5 days of refeeding.
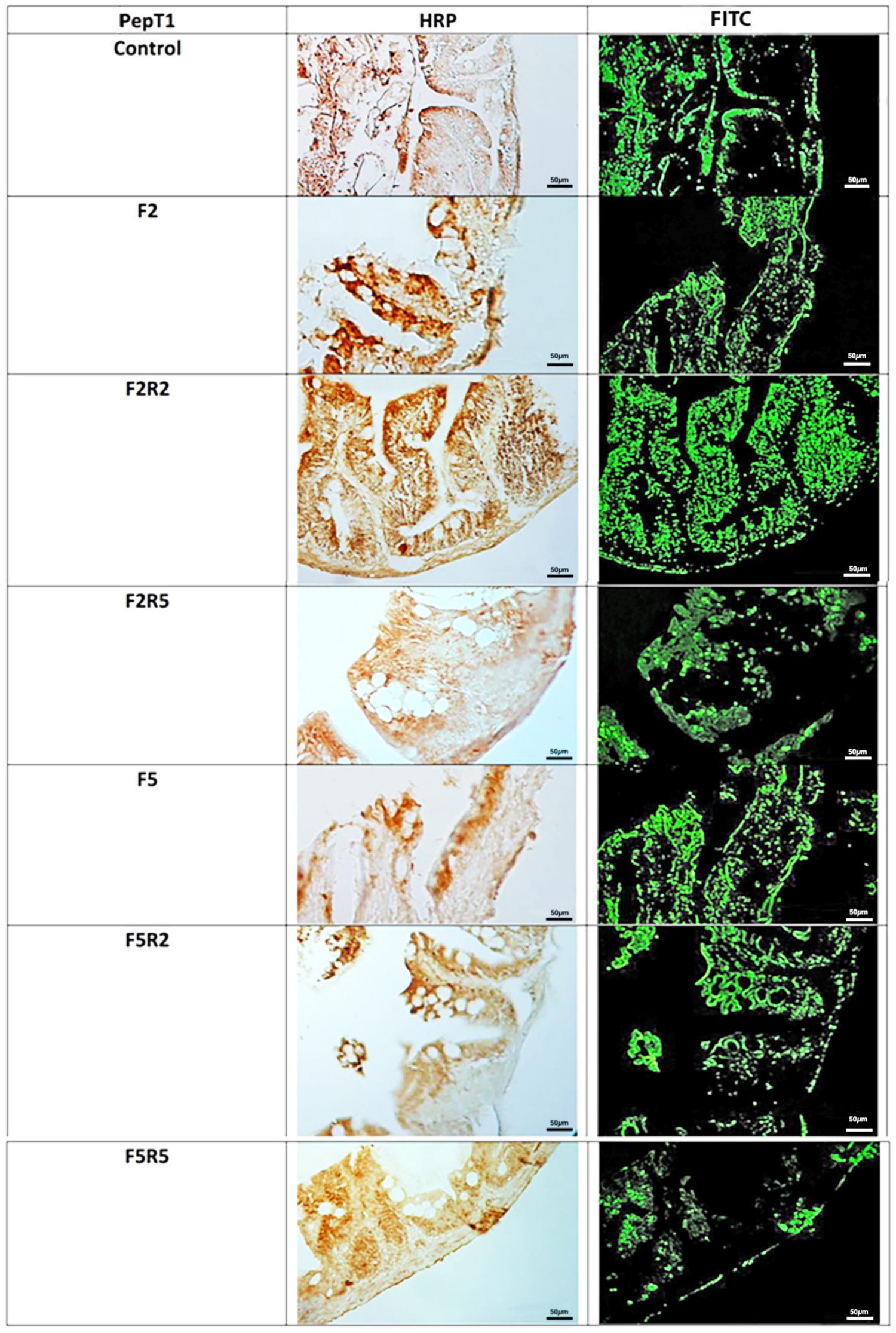
FIGURE 2. Immunohistochemical detection in HRP and Rhodamine of PepT1 in the digestive tract of zebrafish. Control: Continuous feeding; F2: 2 fasting days; F2R2: 2 fasting days followed by 2 refeeding days; F2R5: 2 fasting days followed by 5 refeeding days; F5: 5 fasting days; F5R2: 5 fasting days followed by 2 refeeding days; F5R5: 5 fasting days followed by 5 refeeding days. Scale bars: 10 μm (F5 and F5R5); 20 μm (F2, F2R2, and F5R2); 50 μm (Control and F2R5).
Immunohistochemical investigation in HRP and Fluorescein Isothiocyanate (FITC) performed with the use of antibody for GHR (Figure 3) revealed weak immunopositivity in enteroendocrine cells of control samples. After 2 days of fasting, immunopositivity increased. Refeeding for two and 5 days caused a decrease of immunopositivity, which was particularly marked after 5 days.
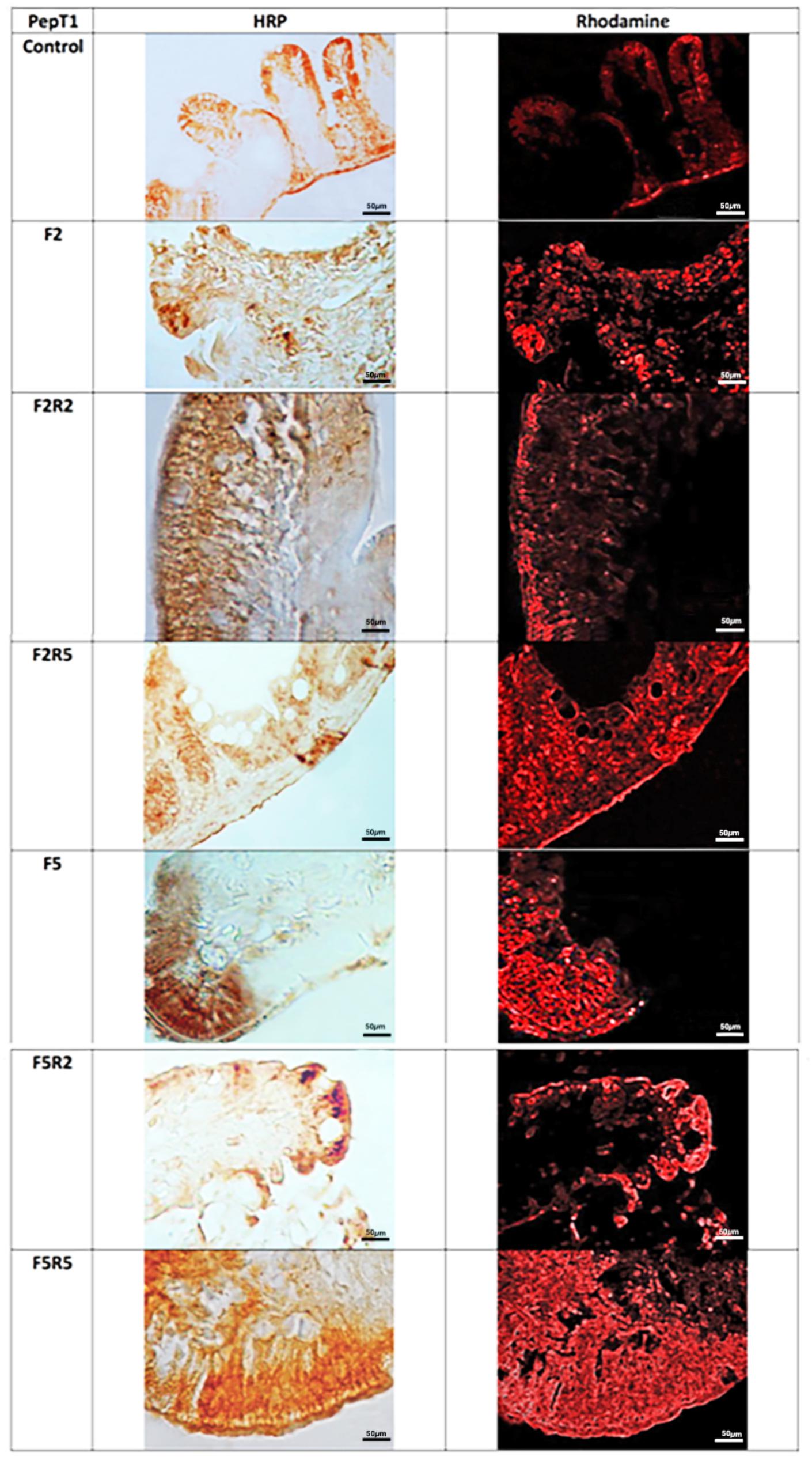
FIGURE 3. Immunohistochemical detection in HRP and FITC of Ghrelin in the digestive tract of zebrafish. Control: Continuous feeding; F2: 2 fasting days; F2R2: 2 fasting days followed by 2 refeeding days; F2R5: 2 fasting days followed by 5 refeeding days; F5: 5 fasting days; F5R2: 5 fasting days followed by 2 refeeding days; F5R5: 5 fasting days followed by 5 refeeding days. Scale bars: 50 μm.
Discussion
In this study, the regulation of PepT1 and GHR after vegetable protein sources were used as an alternative diet of zebrafish, was investigated. In fish subjected to a protocol of fasting of 2 and 5 days followed by refeeding ad libitum for 2 and 5 days, using a diet of Spirulina tabs, immunohistochemical analysis showed the levels of PepT1 and GHR compared to the control group. PepT1 levels progressively decreased during food deprivation and increased fourfold after refeeding; instead, GHR levels increased after 2 days of fasting, decreased gradually after refeeding by twice as much as fish fed prior to fasting. PepT1 affinity and its stereoselective capacity varies for different types of peptides, suggesting that its activity may be modulated through diet, particularly using different protein sources (Ostaszewska et al., 2010a,b; Bertuccio, 2013). High rates of several terrestrial plant meals have been successfully included in the feed without influencing fish growth and production quality (Carter and Hauler, 2000; Aslaksen et al., 2007; Sánchez-Lozano et al., 2011). The high level of PepT1 conservation through evolution is not only consistent with its important role in growth and metabolism, but also suggests that its biological action may be well conserved. Therefore, numerous aspects of digestion and absorption in fish and mammals are similar, demonstrating high conservation of these mechanisms during evolution, in humans (Ford et al., 2003) and fish species such as Atlantic cod, sea bass (Rønnestad et al., 2007; Terova et al., 2009), weather loach (Gonçalves et al., 2007), zebrafish (Verri et al., 2003), and sea bream (Lauriano et al., 2012). mRNA transcription and activity of Pep T1 have been shown to increase to compensate absorptive capability in patients with short-bowel syndrome chronic ulcerative colitis and with Crohn’s disease (Merlin et al., 2001; Ziegler et al., 2002). A similar strategy may be active in fish that exhibit compensatory growth following a period of food deprivation (Navarro et al., 1997; Belanger et al., 2002). In support of this, it has been shown that in sea bass (Dicentrarchus labrax) Pep T1 was involved in compensatory growth during refeeding after a period of fasting (Verri et al., 2011). These mechanisms also influence gastrointestinal hormone levels such as cholecystokinin, gastrin-releasing peptide, GHR (Darcel et al., 2005; Liou et al., 2011). GHR is involved in the regulation of appetite and feeding in vertebrates. There is evidence that GHR functions in energy homeostasis control and food intake increase (Volkoff and Peter, 2006). GHR presence increases in the hypothalamus and gut of goldfish and in the stomach of sea bass with food deprivation (Terova et al., 2008). In this study, GHR was mainly found in the intestinal tract of zebrafish after 2, and much more after 5, days of fasting. Similar to studies of other fish species without a stomach, such as goldfish and common carp, GHR was predominantly found in the gut (Unniappan et al., 2002). Elevated GHR levels in the intestine of blunt snout bream may be related to its biological regulation of appetite. For fish species with stomachs, such as rainbow trout (Kaiya et al., 2003), sea bass (Terova et al., 2008), and Atlantic cod (Xu and Volkoff, 2009), GHR was firstly detected in the stomach. In conclusion, fasting and refeeding experiments confirmed that GHR has the opposite function to PepT1 and CCK, involved in the regulation of feeding in zebrafish. These findings give a further contribution to understanding the role of GHR, in the regulation of zebrafish appetite and could be useful for providing basic information for a responsible aquaculture of teleosts.
The results confirm that the role of PepT1 and GHR is of major nutritional significance for intraluminal products of protein digestion. Although there are many differences between teleosts and mammalian intestines, this paper confirms high conservation of these physiological mechanisms during evolution.
Author Contributions
PL and CC: protocol design and manuscript writing. CB: experimental challenge and immunohistochemistry. CI: animal care and histology. FM: manuscript editing and animal welfare. MD: data analysis.
Conflict of Interest Statement
The authors declare that the research was conducted in the absence of any commercial or financial relationships that could be construed as a potential conflict of interest.
References
Abolfathi, M., Hajimoradloo, A., Ghorbani, R., and Zamani, A. (2012). Effect of starvation and refeeding on digestive enzyme activities in juvenile roach, Rutilus rutilus caspicus. Comp. Biochem. Physiol. A Mol. Integr. Physiol. 161, 166–173. doi: 10.1016/j.cbpa.2011.10.020
Amole, N., and Unniappan, S. (2009). Fasting induces preproghrelin mRNA expression in the brain and gut of zebrafish, Danio rerio. Gen. Comp. Endocrinol. 161, 133–137. doi: 10.1016/j.ygcen.2008.11.002
Aslaksen, M. A., Kraugerud, O. F., Penn, M., Svihus, B., Denstadli, V., Jørgensen, H. Y., et al. (2007). Screening of nutrient digestibilities and intestinal pathologies in Atlantic salmon, Salmo salar, fed diets with legumes, oilseeds, or cereals. Aquaculture 272, 541–555. doi: 10.1016/j.aquaculture.2007.07.222
Belanger, F., Blier, P. U., and Dutil, J. D. (2002). Digestive capacity and compensatory growth in Atlantic cod (Gadus morhua). J. Fish Biol. 26, 121–128.
Bertuccio, C. (2013). Macroalgae. A Guide to the Observation of the Macroalgal Flora in Ganzirri Brackish Pond Cape Peloro Lagoon (Messina, Sicily) Unicum of Biodiversity and Culture. Rome: Aracne, 1–236.
Burger, K. S., and Berner, L. A. (2014). A functional neuroimaging review of obesity, appetitive hormones and ingestive behavior. Physiol. Behav. 136, 121–127. doi: 10.1016/j.physbeh.2014.04.025
Carter, C. G., and Hauler, R. C. (2000). Fishmeal replacement by plant meals in extruded feeds for Atlantic salmon, Salmo salar L. Aquaculture 185, 299–311. doi: 10.1016/S0044-8486(99)00353-1
Caruso, G., Denaro, M. G., Caruso, R., De Pasquale, F., Genovese, L., and Maricchiolo, G. (2014). Changes in digestive enzyme activities of red porgy Pagrus pagrus during a fasting-refeeding experiment. Fish Physiol. Biochem. 40, 1373–1382. doi: 10.1007/s10695-014-9931-x
Caruso, G., Denaro, M. G., Caruso, R., Genovese, L., Mancari, F., and Maricchiolo, G. (2012). Short fasting and refeeding in red porgy (Pagrus pagrus, Linnaeus 1758): response of some haematological, biochemical and non-specific immune parameters. Mar. Environ. Res. 81, 18–25. doi: 10.1016/j.marenvres.2012.07.003
Caruso, G., Denaro, M. G., Caruso, R., Mancari, F., Genovese, L., and Maricchiolo, G. (2011). Response to short term starvation of growth, haematological, biochemical and non-specific immune parameters in European sea bass (Dicentrarchus labrax) and blackspot sea bream (Pagellus bogaraveo). Mar. Environ. Res. 72, 46–52. doi: 10.1016/j.marenvres.2011.04.005
Darcel, N. P., Liou, A. P., Tomé, D., and Raybould, H. E. (2005). Activation of vagal afferents in the rat duodenum by protein digests requires PepT1. J. Nutr. 135, 1491–1495. doi: 10.1093/jn/135.6.1491
Denaro, M. G., Caruso, G., and Genovese, L. (2006). Gastro-protective effect of capsaicin in Anguilla anguilla (Linneus, 1758): evidence from an experimental study on gastric bags. Ittiopatologia 3, 263–271.
Dickson, S. L., Egecioglu, E., Landgren, S., Skibicka, K. P., Engel, J. A., and Jerlhag, E. (2011). The role of the central ghrelin system in reward from food and chemical drugs. Mol. Cell. Endocrinol. 340, 80–87. doi: 10.1016/j.mce.2011.02.017
Feng, K., Zhang, G. R., Wei, K. J., and Xiong, B. X. (2013). Molecular cloning, tissue distribution, and ontogenetic expression of ghrelin and regulation of expression by fasting and refeeding in the grass carp (Ctenopharyngodon idellus). J. Exp. Zool. A Ecol. Genet. Physiol. 319, 202–212. doi: 10.1002/jez.1784
Ford, D., Howard, A., and Hirst, B. H. (2003). Expression of the peptide transporter hPepT1 in human colon: a potential route for colonic protein nitrogen and drug absorption. Histochem. Cell Biol. 119, 37–43.
Gonçalves, A. F., Castro, L. F., Pereira-Wilson, C., Coimbra, J., and Wilson, J. M. (2007). Is there a compromise between nutrient uptake and gas exchange in the gut of Misgurnus anguillicaudatus, an intestinal air-breathing fish? Comp. Biochem. Physiol. D 2, 345–355. doi: 10.1016/j.cbd.2007.08.002
Guroy, B., Sahin, I., Mantoglu, S., and Kayali, S. (2012). Spirulina as a natural carotenoid source on growth, pigmentation and reproductive performance of yellow tail cichlid Pseudotropheus acei. Aquacult. Int. 20, 869–878. doi: 10.1007/s10499-012-9512-x
Guroy, D., Guroy, B., Merrifield, D. L., Ergun, S., Tekinay, A. A., and Yigit, M. (2011). Effect of dietary Ulva and Spirulina on weight loss and body composition of rainbow trout, Oncorhynchus mykiss (Walbaum), during a starvation period. J. Anim. Physiol. Anim. Nutr. 95, 320–327. doi: 10.1111/j.1439-0396.2010.01057.x
Hayashida, T., Nakahara, K., Mondal, M. S., Date, Y., Nakazato, M., Kojima, M., et al. (2002). Ghrelin in neonatal rats: distribution in stomach and its possible role. J. Endocrinol. 173, 239–245. doi: 10.1677/joe.0.1730239
Jonsson, E., Forsman, A., Einarsdottir, I. E., Egner, B., Ruohonen, K., and Bjornsson, B. T. (2006). Circulating levels of cholecystokinin and gastrin-releasing peptide in rainbow trout fed different diets. Gen. Comp. Endocrinol. 148, 187–194. doi: 10.1016/j.ygcen.2006.02.016
Kaiya, H., Koizumi, Y., Konno, N., Yamamoto, K., Uchiyama, M., Kangawa, K., et al. (2011). Ghrelin receptor in two species of anuran amphibian, bullfrog (Rana catesbeiana), and Japanese Tree Frog (Hyla japonica). Front. Endocrinol. 26:31. doi: 10.3389/fendo.2011.00031
Kaiya, H., Kojima, M., Hosoda, H., Riley, L. G., Hirano, T., Grau, E. G., et al. (2003). Identification of tilapia ghrelin and its effects on growth hormone and prolactin release in the tilapia, Oreochromis mossambicus. Comp. Biochem. Physiol. B Biochem. Mol. Biol. 135, 421–429. doi: 10.1016/S1096-4959(03)00109-X
Kasacka, I., Arciszewski, M., and Łebkowski, W. (2014). Extraordinary level of hormone and number of ghrelin cells in the stomach and duodenum of an obese woman. Acta Histochem. 116, 230–234. doi: 10.1016/j.acthis.2013.05.007
Kojima, M., Hosoda, H., Date, Y., Nakazato, M., Matsuo, H., and Kangawa, K. (1999). Ghrelin is a growth-hormone-releasing acylated peptide from stomach. Nature 9, 656–660. doi: 10.1038/45230
Koven, W., and Schulte, P. (2012). The effect of fasting and refeeding on mRNA expression of PepT1 and gastrointestinal hormones regulating digestion and food intake in zebrafish (Danio rerio). Fish Physiol. Biochem. 38, 1565–1575. doi: 10.1007/s10695-012-9649-6
Krogdahl, A., and Bakke-McKellep, A. M. (2005). Fasting and refeeding cause rapid changes in intestinal tissue mass and digestive enzyme capacities of Atlantic salmon (Salmo salar L.). Comp. Biochem. Physiol. A Mol. Integr. Physiol. 141, 450–460. doi: 10.1016/j.cbpb.2005.06.002
Lauriano, E. R., Calò, M., Silvestri, G., Zaccone, D., Pergolizzi, S., and Lo Cascio, P. (2012). Mast cells in the intestine and gills of the sea bream, Sparus aurata, exposed to a polychlorinated biphenyl, PCB 126. Acta Histochem. 114, 166–171. doi: 10.1016/j.acthis.2011.04.004
Lawrence, C. (2007). The husbandry of zebrafish (Danio rerio): a review. Aquaculture 269, 1–20. doi: 10.1016/j.aquaculture.2007.04.077
Liou, A. P., Chavez, D. I., Espero, E., Hao, S., Wank, S. A., and Raybould, H. E. (2011). Protein hydrolysate-induced cholecystokinin secretion from enteroendocrine cells is indirectly mediated by the intestinal oligopeptide transporter PepT1. Am. J. Physiol. Gastrointest. Liver Physiol. 300, G895–G902. doi: 10.1152/ajpgi.00521.2010
Lo Cascio, P., Calabrò, C., Bertuccio, C., Paterniti, I., Palombieri, D., Calò, M., et al. (2017). Effects of fasting and refeeding on the digestive tract of zebrafish (Danio rerio) fed with Spirulina (Arthrospira platensis), a high protein feed source. Nat. Prod. Res. 1478–1485. doi: 10.1080/14786419.2016.1274893
Lu, J., and Takeuchi, T. (2004). Spawning and egg quality of the tilapia Oreochromis niloticus fed solely on raw Spirulina throughout three generations. Aquaculture 234, 625–640. doi: 10.1016/j.aquaculture.2003.12.027
Marino, F., Di Caro, G., Gugliandolo, C., Spanò, A., Faggio, C., Genovese, G., et al. (2016). Preliminary study on the in vitro and in vivo effects of Asparagopsis taxiformis bioactive phycoderivates on teleosts. Front. Physiol. 7:459. doi: 10.3389/fphys.2016.00459
Matty, A., and Smith, P. (1978). Evaluation of a yeast, a bacterium and an alga as a protein source for rainbow trout: I. Effect of protein level on growth, gross conversion efficiency and protein conversion efficiency. Aquaculture 14, 235–246. doi: 10.1016/0044-8486(78)90097-2
Merlin, D., Si-Tahar, M., Sitaraman, S. V., Eastburn, K., Williams, I., Liu, X., et al. (2001). Colonic epithelial hPepT1 expression occurs in inflammatory bowel disease: transport of bacterial peptides influences expression of MHC class 1 molecules. Gastroenterology 120, 1666–1679. doi: 10.1053/gast.2001.24845
Mustafa, M. G., Umino, T., and Nakagawa, H. (1997). Limited synergistic effect of dietary Spirulina on vitamin C nutrition of red sea bream Pagrus major. J. Mar. Biotechnol. 5, 129–132.
Nakagawa, H., and Montgomery, W. L. (2007). “Algae,” in Dietary Supplements for the Health and Quality of Cultured Fish, eds H. Nakagawa, M. Sato, and D. M. Gatlin III (Cambridge: CABI International), 133–167. doi: 10.1079/9781845931995.0133
Nakazato, M., Murakami, N., Date, Y., Kojima, M., Matsuo, H., Kangawa, K., et al. (2001). A role for ghrelin in the central regulation of feeding. Nature 11, 194–198. doi: 10.1038/35051587
Nandeesha, M. C., Gangadhar, B., Varghese, T. J., and Keshavanath, P. (1998). Effect of feeding Spirulina platensis on the growth, proximate composition and organoleptic quality of common carp, Cyprinus carpio L. Aquac. Res. 29, 305–312. doi: 10.1111/j.1365-2109.1998.tb01135.x
Navarro, I., Blasco, J., Banos, N., and Gutierez, J. (1997). Effects of fasting and feeding on plasma amino acid levels in brown trout. Fish Physiol. Biochem. 16, 303–309. doi: 10.1023/A:1007759316656
Ngernsoungnern, A., and Ngernsoungnern, P. (2016). Localization of ghrelin-like peptide in the gastrointestinal tract of the golden apple snail (Pomacea canaliculata) and changing of its concentration during fasting. Acta Histochem. 118, 244–251. doi: 10.1016/j.acthis.2016.01.005
Olsson, C., Holbrook, J. D., Bompadre, G., Jonsson, E., Hoyle, C. H., Sanger, G. J., et al. (2008). Identification of genes for the ghrelin and motilin receptors and a novel related gene in fish, and stimulation of intestinal motility in zebrafish (Danio rerio) by ghrelin and motilin. Gen. Comp. Endocrinol. 155, 217–226. doi: 10.1016/j.ygcen.2007.05.016
Ostaszewska, T., Dabrowski, K., Kamaszewski, M., Grochowski, P., Verri, T., Rzepkowska, M., et al. (2010a). The effect of plant protein-based diet supplemented with dipeptide or free amino acids on digestive tract morphology and PepT1 and PepT2 expressions in common carp (Cyprinus carpio L.). Comp. Biochem. Physiol. A Mol. Integr. Physiol. 157, 158–169. doi: 10.1016/j.cbpa.2010.06.162
Ostaszewska, T., Kamaszewski, M., Grochowski, P., Dabrowski, K., Verri, T., Aksakal, E., et al. (2010b). The effect of peptide absorption on PepT1 gene expression and digestive system hormones in rainbow trout (Oncorhynchus mykiss). Comp. Biochem. Physiol. A Mol. Integr. Physiol. 155, 107–114. doi: 10.1016/j.cbpa.2009.10.017
Palmegiano, G. B., Agradi, E., Forneris, G., Gai, F., Gasco, L., Rigamonti, E., et al. (2005). Spirulina as a nutrient source in diets for growing sturgeon (Acipenser baeri). Aquacult. Res. 36, 188–195. doi: 10.1111/j.1365-2109.2005.01209.x
Rizzo, C., Genovese, G., Morabito, M., Faggio, C., Pagano, M., Spanò, A., et al. (2017). Potential antibacterial activity of marine macroalgae against pathogens relevant for aquaculture and human health. JPAM 11, 1695–1706. doi: 10.22207/JPAM.11.4.07
Rønnestad, I., Gavaia, P. J., Viegas, C. S., Verri, T., Romano, A., Nilsen, T. O., et al. (2007). Cloning, tissue expression and comparative aspects of the Atlantic cod (Gadus morhua L.) oligopeptide transporter PepT1. J. Exp. Biol. 210, 3883–3896. doi: 10.1242/jeb.007898
Sakata, I., and Sakai, T. (2010). Ghrelin cells in the gastrointestinal tract. Int. J. Pept. 2010:945056. doi: 10.1155/2010/945056
Sánchez-Lozano, N. B., Martínez-Llorens, S., Tomás-Vidal, A., and Cerdà, M. J. (2011). Amino acid retention of gilthead sea bream (Sparus aurata, L.) fed a pea protein concentrate. Aquacult. Nutr. 17, 604–614. doi: 10.1111/j.1365-2095.2010.00803.x
Shao, Y., Liu, S., Tang, X., Gao, J., Wu, G., and Li, Z. (2010). Ontogeny of ghrelin mRNA expression and identification of ghrelin-immunopositive cells in the gastrointestinal tract of the Peking duck, Anas platyrhynchos. Gen. Comp. Endocrinol. 166, 12–18. doi: 10.1016/j.ygcen.2009.11.002
Shousha, S., Nakahara, K., Kojima, M., Miyazato, M., Hosoda, H., Kangawa, K., et al. (2005). Different effects of peripheral and central ghrelin on regulation of food intake in the Japanese quail. Gen. Comp. Endocrinol. 141, 178–183. doi: 10.1016/j.ygcen.2004.12.021
Spence, R., Fatema, M. K., Ellis, S., Ahmed, Z. F., and Smith, C. (2007). Diet, growth and recruitment of wild zebrafish in Bangladesh. J. Fish Biol. 71, 304–309. doi: 10.1111/j.1095-8649.2007.01492.x
Stanley, J. G., and Jones, J. B. (1976). Feeding algae to fish. Aquaculture 7, 2192–2223. doi: 10.1016/0044-8486(76)90140-X
Suda, A., Kaiya, H., Nikaido, H., Shiozawa, S., Mishiro, K., and Ando, H. (2012). Identification and gene expression analyses of ghrelin in the stomach of Pacific bluefin tuna (Thunnus orientalis). Gen. Comp. Endocrinol. 178, 89–97. doi: 10.1016/j.ygcen.2012.04.026
Takeuchi, T., Lu, J., Yoshizaki, G., and Satoh, S. (2002). Effect on the growth and body composition of juvenile tilapia Oreochromis niloticus fed raw Spirulina. Fish. Sci. 68, 34–40. doi: 10.1046/j.1444-2906.2002.00386.x
Terova, G., Corà, S., Verri, T., Rimoldi, S., Bernardini, G., and Saroglia, M. (2009). Impact of feed availability on PepT1 mRNA expression levels in sea bass (Dicentrarchus labrax). Aquaculture 294, 288–299. doi: 10.1016/j.aquaculture.2009.06.014
Terova, G., Rimoldi, S., Bernardini, G., Gornati, R., and Saroglia, M. (2008). Sea bass ghrelin: molecular cloning and mRNA quantification during fasting and refeeding. Gen. Comp. Endocrinol. 155, 341–351. doi: 10.1016/j.ygcen.2007.05.028
Unniappan, S., Lin, X., Cervini, L., Rivier, J., Kaiya, H., Kangawa, K., et al. (2002). Goldfish ghrelin: molecular characterization of the complementary deoxyribonucleic acid, partial gene structure and evidence for its stimulatory role in food intake. Endocrinology 143, 4143–4146. doi: 10.1210/en.2002-220644
Verri, T., Kottra, G., Romano, A., Tiso, N., Peric, M., Maffia, M., et al. (2003). Molecular and functional characterization of the zebrafish (Danio rerio) PepT1-type peptide transporter. FEBS Lett. 549, 115–122. doi: 10.1016/S0014-5793(03)00759-2
Verri, T., Terova, G., Dabrowski, K., and Saroglia, M. (2011). Peptide transport and animal growth: the fish paradigm. Biol. Lett. 74, 597–600. doi: 10.1098/rsbl.2010.1164
Volkoff, H., and Peter, R. E. (2006). Feeding behavior of fish and its control. Zebrafish 3, 131-140. doi: 10.1089/zeb.2006.3.131
Wada, R., Sakata, I., Kaiya, H., Nakamura, K., Hayashi, Y., Kangawa, K., et al. (2003). Existence of ghrelin-immunopositive and -expressing cells in the proventriculus of the hatching and adult chicken. Regul. Pept. 28, 123–128. doi: 10.1016/S0167-0115(02)00265-3
Wallace, K. N., Akhter, S., Smith, E. M., Lorent, K., and Pack, M. (2005). Intestinal growth and differentiation in zebrafish. Mech. Dev. 122, 157–173. doi: 10.1016/j.mod.2004.10.009
Xu, M., and Volkoff, H. (2009). Cloning, tissue distribution and effects of food deprivation on pituitary adenylate cyclase activating polypeptide (PACAP)/PACAP-related peptide (PRP) and preprosomatostatin 1 (PPSS 1) in Atlantic cod (Gadus morhua). Peptides 30, 766–776. doi: 10.1016/j.peptides.2008.12.010
Zhao, Z., and Sakai, T. (2008). Characteristic features of ghrelin cells in the gastrointestinal tract and the regulation of stomach ghrelin expression and production. World J. Gastroenterol. 14, 6306–6311. doi: 10.3748/wjg.14.6306
Ziegler, T. R., Fernández-Estívariz, C., Gu, L. H., Bazargan, N., Umeakunne, K., Wallace, T. M., et al. (2002). Distribution of the H+/peptide transporter PepT1 in human intestine: up-regulated expression in the colonic mucosa of patients with short-bowel syndrome. Am. J. Clin. Nutr. 75, 922–930. doi: 10.1093/ajcn/75.5.922
Keywords: immunohistochemistry, PepT1, GHR, zebrafish, digestive tract, Spirulina sp.
Citation: Lo Cascio P, Calabrò C, Bertuccio C, Iaria C, Marino F and Denaro MG (2018) Immunohistochemical Characterization of PepT1 and Ghrelin in Gastrointestinal Tract of Zebrafish: Effects of Spirulina Vegetarian Diet on the Neuroendocrine System Cells After Alimentary Stress. Front. Physiol. 9:614. doi: 10.3389/fphys.2018.00614
Received: 20 February 2018; Accepted: 07 May 2018;
Published: 24 May 2018.
Edited by:
Rubina Sirri, Università degli Studi di Bologna, ItalyReviewed by:
Tiziano Verri, University of Salento, ItalyMaurizio Manera, Università degli Studi di Teramo, Italy
Copyright © 2018 Lo Cascio, Calabrò, Bertuccio, Iaria, Marino and Denaro. This is an open-access article distributed under the terms of the Creative Commons Attribution License (CC BY). The use, distribution or reproduction in other forums is permitted, provided the original author(s) and the copyright owner are credited and that the original publication in this journal is cited, in accordance with accepted academic practice. No use, distribution or reproduction is permitted which does not comply with these terms.
*Correspondence: Fabio Marino, bWFyaW5vZkB1bmltZS5pdA==