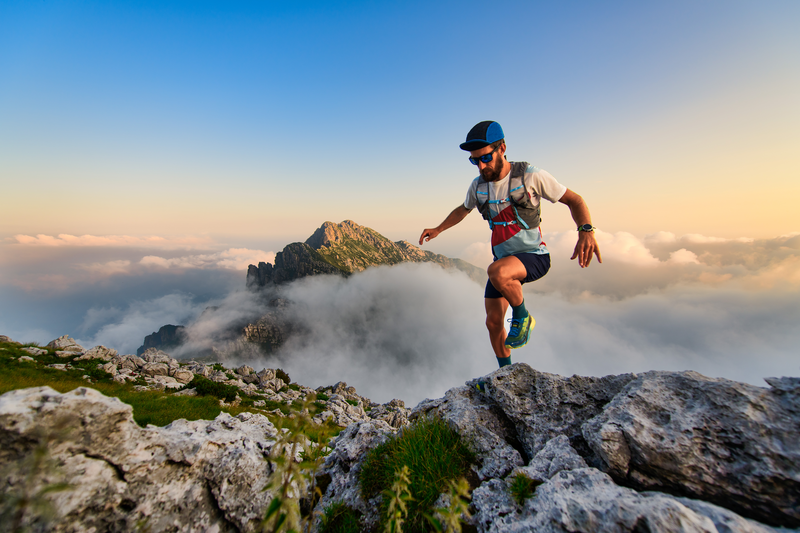
94% of researchers rate our articles as excellent or good
Learn more about the work of our research integrity team to safeguard the quality of each article we publish.
Find out more
PERSPECTIVE article
Front. Physiol. , 19 June 2017
Sec. Invertebrate Physiology
Volume 8 - 2017 | https://doi.org/10.3389/fphys.2017.00403
This article is part of the Research Topic The Digestive Tract of Cephalopods: at the Interface Between Physiology and Ecology View all 19 articles
Ensuring the health and welfare of animals in research is paramount, and the normal functioning of the digestive tract is essential for both. Here we critically assess non- or minimally-invasive techniques which may be used to assess a cephalopod's digestive tract functionality to inform health monitoring. We focus on: (i) predatory response as an indication of appetitive drive; (ii) body weight assessment and interpretation of deviations (e.g., digestive gland weight loss is disproportionate to body weight loss in starvation); (iii) oro-anal transit time requiring novel, standardized techniques to facilitate comparative studies of species and diets; (iv) defecation frequency and analysis of fecal color (diet dependent) and composition (parasites, biomarkers, and cytology); (v) digestive tract endoscopy, but passage of the esophagus through the brain is a technical challenge; (vi) high resolution ultrasound that offers the possibility of imaging the morphology of the digestive tract (e.g., food distribution, indigestible residues, obstruction) and recording contractile activity; (vii) needle biopsy (with ultrasound guidance) as a technique for investigating digestive gland biochemistry and pathology without the death of the animal. These techniques will inform the development of physiologically based assessments of health and the impact of experimental procedures. Although intended for use in the laboratory they are equally applicable to cephalopods in public display and aquaculture.
Interest in cephalopods and their welfare is stimulated by significant policy changes initiated at European Union (EU) level, that are recognized to have an impact beyond Europe (Di Cristina et al., 2015). Such European Policies and Directives have implications for cephalopods in scientific research, aquaculture or public display, and relevance for fisheries and ecology, i.e., Directive 2010/63/EU, EU Common Fisheries Policy, Marine Strategy Framework Directive, the revision of IUCN Red List (review in: ICES, 2014; Di Cristina et al., 2015; Xavier et al., 2015).
Cephalopods are a special case in a “regulation” context since: (i) it is the first time that research on an entire class of invertebrate has been regulated in the European Union (Smith et al., 2013), and (ii) the species are very diverse in terms of ecological, biological, physiological, and behavioral adaptations (e.g., Packard, 1972; Borrelli and Fiorito, 2008; Grasso and Basil, 2009; Kröger et al., 2011; Albertin et al., 2015).
Daily assessment of animal well-being is required under Directive 2010/63/EU (Fiorito et al., 2015), but few indicators of digestive system physiology are available. Fiorito and co-workers list five (out of 27) signs potentially linked to the digestive system that may indicate an alteration in normal behavior or physiology (see Table 5 in Fiorito et al., 2015).
How can the functionality of the digestive tract be assessed routinely at the “tank–side”?
Here we review and suggest a series of non-invasive and/or minimally invasive approaches (Table 1).
Table 1. Summary of parameters that could be used to monitor cephalopod digestive tract functioning by non-invasive or minimally invasive techniques to provide either a direct or indirect insight into the physiology of the digestive tract. Examples are taken mainly from studies on S. officinalis or Nautilus pompilius and/or octopus (mostly O. vulgaris), but all techniques appear equally applicable to squid.
The vast majority of cephalopod species are active predators, and prey attack is considered to be a good indicator of overall health (Fiorito et al., 2014). Latency to attack either a live- or an artificial crab is used as an indication of health in Octopus vulgaris (Amodio et al., 2014), and a prompt feeding response to a live fish is proposed for cuttlefish and squid as an indication of full recovery after transport (Oestmann et al., 1997). Latency to attack may vary between individuals (Lee et al., 1991) which probably denotes different temperaments (e.g., Sinn et al., 2001; Sinn and Moltschaniwskyj, 2005; Borrelli, 2007; Carere et al., 2015), and these differences should be taken into account (see discussion in Borrelli and Fiorito, 2008). In addition, it may be influenced by housing, as shown by laboratory reared juvenile cuttlefish that have a shorter attack latency (three times faster) if housed together (Warnke, 1994), and if there is more available space (Boal et al., 1999).
Ingestion of a normal amount of food (assuming “normal” can be defined for species, age, sex, and temperature) at a regular frequency is probably the most useful indicator of health. However, monitoring food intake requires knowledge of the amount of food provided and of possible remains. Therefore, tanks should be inspected for empty carapaces, shells, and other residues as well as uneaten food. For prepared diets whenever accepted, allowance needs to be made for portions of the food pellet lost, due to leaching and disaggregation, and not ingested.
If animals are fed live prey, uneaten specimens must be removed to avoid possible welfare issues; the prey may become the “predator,” particularly if the size difference between the two individuals is not large (e.g., a small cuttlefish and a shrimp). In addition, leaving uneaten prey may lead to inaccurate estimation of intake over a particular time period.
An additional issue is to ensure that every animal has sufficient food. This can be challenging for species which may be housed in groups such as S. officinalis, Loligo vulgaris (or other squid) or O. vulgaris that are kept under culture conditions. This may be assisted by observation, but feeding hierarchies should be taken into consideration when animals (e.g., cultured cuttlefish) are housed together (see discussion in Warnke, 1994). To circumvent these issues, and again in an aquaculture context animals are fed ad libitum, but accurate monitoring of food remains is required.
Food intake may decrease with increasing size in cephalopods, and depends on food availability, its quality and size, the duration of digestion, maturation, and temperature (Mangold, 1983).
Feeding behavior and food intake may be affected by experimental procedures; resumption of the normal status should be assessed at individual animal level. A few examples from the literature are provided below.
Octopus tetricus attacked and ate crabs within 15 min after brief anesthesia used to facilitate handling for weighing (Joll, 1977). Similarly, S. officinalis resumed feeding on grass shrimp 7 min after recovery from anesthesia used for handling (Gonçalves et al., 2012). Cuttlefish resumed feeding 48 h post-surgery after kainic acid lesion of the vertical lobe (Graindorge et al., 2008). O. vulgaris performed a normal predatory response 1 h after anesthesia and arm surgery (Shaw et al., 2016). However, recovery of the attack response following cold water “anaesthesia” in the same species is prolonged with respect to circumstances when magnesium chloride is used as agent (Agnisola et al., 1996).
Cephalopod paralarvae or other early stages provide further challenges. Ingestion of food and estimation of food intake may be assessed by direct observation or through the use of microfluorospheres (around 10 μm diameter) included in the food (see Villanueva and Norman, 2008, and below), since at early stages most species are transparent.
Loss of body weight or growth below particular pre-set limits are frequently used as humane end points (see discussion in: Smith et al., 2013; Fiorito et al., 2015) in regulated procedures. However, setting limits requires knowledge of the normal variations in body weight for the species studied and the housing conditions (particularly water temperature). Total body length is not considered a valid index of growth in cephalopods because of variables including the elasticity of tentacles and arms, and differences in relative growth linked to sex and season (e.g., Bello, 1991; Cortez et al., 1999; Pierce et al., 1999; Sivashanthini et al., 2009).
Gross growth efficiency is dependent on food quality, its ingestion and water temperature (Mangold, 1983). Growth curves for several cephalopod species in captivity show a reduction in growth rate over Winter, due to a temperature decrease in open aquaria (e.g., Nixon, 1966; Joll, 1977; Boyle and Knobloch, 1982; Domingues et al., 2002). However, in constant temperature aquaria, a reduction in growth rate is most likely due to reduced food intake.
Additionally, the available bottom area of the tank and the stocking density may also influence growth rates (see for example: Forsythe et al., 2002; Correia et al., 2005; Delgado et al., 2010; Domingues and Márquez, 2010; Domingues et al., 2010).
The contribution of total body water (intra- and extracellular) to body weight should not be overlooked. Cephalopods ingest and absorb water from the sea and from the diet via the digestive tract, and prevention of fluid absorption from the digestive tract leads to ~10% loss in body weight in 24 h in O. vulgaris (Wells and Wells, 1989, 1993).
Body weight loss should always be investigated, and if a reduction in food intake is identified as the primary cause then this also needs investigation.
Body weight and food intake decrease in several species with maturation (e.g., Mangold and Boletzky, 1973; Lee, 1995) and around the time of egg laying, and since this may affect females, their reproductive status should be checked in cases of evident reduction of body weight/food intake. In females of many, but not all species, reproductive status is accompanied by loss of body weight due to reduced or absent food intake (e.g., Wodinsky, 1977; but see Sykes et al., 2013b), and reduction of food intake may also occur in senescent mature males (Anderson et al., 2002).
Individual tissues may be affected to a greater degree than is apparent from body weight. For example, the proportionate weight loss of the digestive gland in food deprived O. vulgaris is greater than might be suspected from the change in body weight (see Supplementary Figure 1).
If an animal is losing weight, but food intake is within normal limits then the cause is likely to be the functioning of the digestive tract. However, as the pathophysiology of the cephalopod digestive tract has not been studied in any detail, we can only hypothesize about the causes by analogy with vertebrates (see Table 1).
Body weight is probably the best overall indicator of adequate nutrition, but it may not be the most practical parameter to use for routine health assessment. Repeated measures of body weight show variability (Nixon, 1971) and may induce effects linked to handling (e.g., Locatello et al., 2013). Indeed, the effects of repeated measures of body weight on animal welfare have not been properly investigated, to the best of our knowledge. Repeated handling of the animal may be stressful (for example see: Malham et al., 1998; Grimaldi et al., 2013). According to Nixon (1966) frequent handling of O. vulgaris may reduce growth rate, but this does not appear to be the case in cuttlefish (Sykes et al., 2003, 2013a,b).
Weighing usually requires removal of the animal from its tank for measurement in air, although animals can be weighed in water (Aronson, 1982). Body weight may not be a sufficiently sensitive welfare indicator for daily assessment, although it may be useful over longer intervals. However, for species housed in groups reliable identification of individuals is required, and several methods are described for cephalopods (e.g., Huffard et al., 2008; Ikeda et al., 2009; Zeeh and Wood, 2009; Byrne et al., 2010; Barry et al., 2011; Estefanell et al., 2011; Sykes et al., 2017).
This is the time from food ingestion until exit as feces, and provides an overall measure of the key digestive tract functions of motility, secretion, and absorption. The most common method is to mark the food with a chemical marker (e.g., carmine in Bidder, 1957) or to incorporate an indigestible marker into the food (e.g., glass beads encapsulated in Artemia nauplii, Villanueva and Norman, 2008). However, if particular dietary constituents are associated with a fecal color change then by diet switching it would be possible to estimate transit time for that food.
A study in Nautilus pompilius applied X-ray imaging of food labeled with contrast medium (barium sulfate) to monitor the time course of digestion (about 12 h at 18–19°C Westermann et al., 2002). This method has been also explored in S. officinalis (Figure 1H). However, this requires brief but repeated anesthesia which, together with restraint and handling, is likely to affect the oro-anal transit time of the animal.
Figure 1. Sonographic scanning of the digestive tract of Octopus vulgaris (A–G) and X-ray imaging in juvenile S. officinalis (H). (A–G) The digestive tract of O. vulgaris as it appears during ultrasound examination (VEVO 2100, VisualSonics). (A–C) The anterior part of the digestive system (note the crop full of food) and its relationship to other parts within the mantle. (A) Ultrasound examination in the longitudinal plane with supra- (SEM) and sub-oesophageal masses (SUB, sagittal view) and the esophagus (Oes) and the crop (CR, on the right). The posterior salivary glands (PSG) are also clearly identifiable. (B) Sonographic scanning using a transverse plane reveals a distended crop (CR) full of food, the esophagus (Oes), and the cephalic aorta (CA) lying on its dorsal surface between the posterior salivary glands (PSG). (C) Sonographic examination (longitudinal plane) showing one posterior salivary gland (PSG) with its typical leaf-shaped appearance, the distended crop (CR), and the hepatopancreas (HP), ventrally. (D) A sequence of frames from the sonographic examination (transversal plane) of the octopus digestive tract octopus reveals the crop of an animal fed 6 h before the ultrasound scan (about 30 s); the peristaltic motility of the crop is evident through the sequence of snapshots (from t = 0 to 33 s) with contractions and relaxations moving the crop contents. (E) The sequence of frames taken from the same animal during ultrasound examination in the longitudinal plane identifies contraction and relaxation of the crop dividing the bolus. (F) The caecum with its characteristic spiral organization as it appears during sonographic scanning. (G) High resolution (48 MHz) ultrasound scanning of the caecum showing the “villi-like” structures. (H) X-ray imaging of food labeled with contrast medium (barium sulfate) to monitor the course of digestion in juvenile S. officinalis. Scanning performed with a Kodak DXS-4000 Pro system on anesthetized individual. CA, cephalic aorta; Cae, caecum; CR, crop; Oes, esophagus; HP, hepatopancreas; PSG, posterior salivary gland; SEM, supra-oesophageal mass; SUB, sub-oesophageal mass. Images provided here resulted from examinations carried out in compliance with local regulations, and for veterinary purposes. Scale bar, A–F: 5 mm; G: 1 mm.
The relatively few studies undertaken measured oro-anal transit times (see Supplementary Table 1) ranging from 2–10 h in squid (temperatures 16–22°C) to 8–24 h in S. officinalis (14–23°C), and 8–30 h in octopus (10–30°C). Overall, transit is faster in animals living at higher water temperatures; the slowest time we were able to find was for Benthoctopus levis (>30 h at 6°C: Mangold and Lu, unpublished, cited in Mangold and Bidder, 1989).
High resolution ultrasound may provide a method for monitoring movement of digestive tract contents (see below), provided that the animal could be adapted to remain relatively quiescent during repeated imaging sessions lasting a few minutes. This approach is tractable in cuttlefish and octopus, but may be problematic with squid because of their preference to moving in the water column rather than remaining quiet on the bottom of a tank. It is important that measurements are taken in non-sedated and unrestrained animals as this will modify the results.
Fecal analysis provides a non-invasive method for monitoring digestive tract functionality. Defecation and fecal composition are considered separately.
Based upon transit times (see above) and tank inspection it is usually assumed that defaecation takes place at least once daily in S. officinalis and O. vulgaris, but this has yet to be confirmed by a direct study.
Defecation is highly likely to be under central nervous system control as “reflex” expulsion of feces, in the presence of a predator when an animal is attempting to hide, would be disadvantageous. Central control of defecation is likely to be via the visceral nerve originating in the palliovisceral lobe (part of the posterior sub-oesophageal mass) which in O. vulgaris is described as supplying specific branches with relatively large axons (~5 μm) to the terminal rectum and anal flaps (Young, 1967). Injection of 5-hydroxytryptamine (5-HT) into the brain blood supply (via an implanted cannula in the dorsal aorta) evoked defecation in O. vulgaris. This was not induced by nicotine, gamma amino butyric acid, and L-glutamate injections (Andrews et al., 1983). Defecation was not evoked by 5-HT following removal of the supra-oesophageal lobes, suggesting that it may be under “higher” motor control.
Descriptions of defecation in cephalopods are rare; in both cuttlefish and O. vulgaris feces are expelled from the anus in a “rope” and are ejected from the mantle cavity via the siphon by mantle contraction referred, for octopus, as a “cough” (Wells, 1978).
Studies of defecatory behavior are needed to establish normal patterns for each laboratory housed species in relation to the diet. In this way criteria can be set for when a change (increase or decrease) requires investigation or intervention.
Descriptions of the appearance of cephalopod feces are scant. In N. pompilius fed on shrimp feces are described as “red-brown threads, 2 cm in length and with no solid components” (Westermann et al., 2002, p. 1620). These appear “long filiform, but quite variable in size and in color” in O. vulgaris (Taki, 1941), and grayish brown in color when the animals are fed bivalves. An orange brown color is more characteristic of the feces in O. vulgaris fed on crabs, and an orange/red color is characteristic of feces of S. officinalis fed on grass shrimp or crabs most likely due to carotenes. Feces after feeding animals with fish or prepared diets not rich in crustaceans will lack any obvious pigmentation and may be white. The “fecal ropes” have an obvious mucus coating and presumably contain excretory products of metabolism from the digestive gland and any undigested or unabsorbed food. Feces may contain “chips of cuticle” and fish scales (Wells, 1978) and dead cells (a possible source of cells for genotyping) sloughed from the digestive tract epithelium or the digestive gland, digestive tract flora, and shed parasites and cysts.
Steroid hormones have been detected in the feces of Enteroctopus dofleini (Larson and Anderson, 2010), further illustrating the potential of feces as source of biomarkers thus serving as indicators of animals' health and welfare. Overall, the feces are an overlooked potential source of information about the physiology of the digestive tract and their utility as a non-invasive monitor of animal health should be investigated.
Endoscopy is a technique widely used for human and veterinary clinical investigation of the digestive tract (for example see Fritscher-Ravens et al., 2014; Sladakovic et al., 2017) to examine the mucosa for abnormalities (e.g., polyps, parasites) or to perform a biopsy for subsequent analysis and for some surgical procedures. The technique has also been used to investigate finfish (Moccia et al., 1984) and crab digestive tract (Heinzel et al., 1993). Endoscopy requires sedation or general anesthesia so the potential stress of this must also be taken into account when considering welfare implications.
The size of endoscopes is a limiting factor in the application to investigate the cephalopod digestive tract with the restriction placed on the esophagus by the supra- and sub-oesophageal lobes and their connecting circum-oesophageal structures being a particular issue. The lower digestive tract is, in theory, accessible to endoscopic inspection via the anal sphincter, but the size of the endoscope will again be a limiting factor. In addition, as far as can be ascertained in all cephalopods, the intestine exits the gastro-caecal junction running caudally and dorsal, but during its course turns rostrally and ventral to exit the mantle near the siphon. Therefore, inspection of the proximal intestine would require a very flexible endoscope.
Ultrasound is utilized for non-invasive imaging of the mantle, vasculature, brain and arms of cephalopods. Ultrasonographic examination can be undertaken without sedation or anesthesia as carried out in S. officinalis (King et al., 2005; King and Adamo, 2006) or in O. vulgaris (Grimaldi et al., 2007). However, in other circumstances light anesthesia is required to ensure stable images for quantitative analysis of arm or brain morphology (Grimaldi et al., 2007; Margheri et al., 2011).
If an animal stops eating for no apparent reason, ultrasound may help to investigate the digestive tract and search for an obstruction; it should also be possible to view contractile activity of the crop, stomach, caecum or intestine as illustrated in Figure 1. Since the digestive gland decreases in weight, but has an increased % water with increasing duration of food deprivation (see Supplementary Figure 1) it may be possible to use ultrasound measurements of size and density as an index of the metabolic status of the animal, contributing to overall welfare assessment.
As cephalopods experiencing severe food deprivation mobilize lipids from the digestive gland and proteins from muscle and the gills (Lamarre et al., 2012, 2016; Speers-Roesch et al., 2016), ultrasound examination of these structures may provide insights into the overall health status of the animal, particularly if the same structures were imaged on arrival in the laboratory.
The assessment of cephalopod digestive tract function through non-invasive methods needs to be developed further but the above overview highlights key areas (Table 1). For example, a specific analysis of the oro-anal transit times will facilitate species comparisons, investigation of the effects of environmental change, assessment of the impact of pathogens, investigation of neural and hormonal control, and provide standardized methods for comparison of experimental diets for use in aquaculture. Deviation of body weight from normality is considered a key welfare indicator, and the impact of prolonged food deprivation on welfare should be taken into account. Analysis of fecal composition will also give insights into absorption and secretion in the digestive tract especially if combined with measurements of metabolites in the haemolymph and/or digestive gland using minimally invasive techniques (Lamarre et al., 2012, 2016; Speers-Roesch et al., 2016). Simple methods for assessment of digestive tract function will also facilitate comparative studies of a wider range of species including those found more frequently in public aquaria.
Although we focused our attention on a series of possible markers of digestive tract function to be monitored through routine assessment at the “tank-side,” daily assessment of health and welfare largely relies on observation of the animal. Understanding the external manifestations, including behavioral changes, of underlying digestive tract pathophysiology will be essential to improve welfare assessment tools for cephalopods.
All authors contributed to the manuscript and agreed on the final version.
GP has been supported through RITMARE Flagship Project (Italian Ministry of Education, University and Research—MIUR, and Stazione Zoologica Anton Dohrn—SZN). EA work described here was supported by the OCTOWELF project (AGL 2013-49101-C2-1-R, Spanish Government). AS is supported through Fundação para a Ciência e a Tecnologia (IF/00576/2014 contract and Plurennial funding to CCMAR - UID/Multi/04326/2013).
The authors declare that the research was conducted in the absence of any commercial or financial relationships that could be construed as a potential conflict of interest.
We are indebted to the Stazione Zoologica Anton Dohrn (SZN, Italy) and the Centre for Marine Sciences (CCMAR, Portugal) that provided support for most of the approaches illustrated in this work. We thank Dr. Dieter Fuchs for contribution, Visualsonics and the Association for Cephalopod Research-CephRes for further support and advice. PA wishes to acknowledge that this work is part of the activities included in the tenure of a Research Fellowship at Stazione Zoologica Anton Dohrn Naples (Italy), and would like to thank Dr. G. Fiorito (Head of the Department of Biology and Evolution of Marine Organisms, SZN) and the SZN President Professor Roberto Danovaro. This work benefited from networking activities carried out under the COST ACTION FA1301, and is considered a contribution to the COST (European COoperation on Science and Technology) Action FA1301 “A network for improvement of cephalopod welfare and husbandry in research, aquaculture and fisheries” (http://www.cost.eu/COST_Actions/fa/FA1301).
The Supplementary Material for this article can be found online at: http://journal.frontiersin.org/article/10.3389/fphys.2017.00403/full#supplementary-material
Agnisola, C., Castaldo, P., and Fiorito, G. (1996). Octopus vulgaris (Mollusca, Cephalopoda) as a model in behavioral pharmacology: a test of handling effects. Physiol. Behav. 59, 729–733. doi: 10.1016/0031-9384(95)02153-1
Aguila, J., Cuzon, G., Pascual, C., Domingues, P. M., Gaxiola, G., Sánchez, A., et al. (2007). The effects of fish hydrolysate (CPSP) level on Octopus maya (Voss and Solis) diet: digestive enzyme activity, blood metabolites, and energy balance. Aquaculture 273, 641–655. doi: 10.1016/j.aquaculture.2007.07.010
Albertin, C. B., Simakov, O., Mitros, T., Wang, Z. Y., Pungor, J. R., Edsinger-Gonzales, E., et al. (2015). The octopus genome and the evolution of cephalopod neural and morphological novelties. Nature 524, 220–224. doi: 10.1038/nature14668
Amodio, P., Andrews, P., Salemme, M., Ponte, G., and Fiorito, G. (2014). The use of artificial crabs for testing predatory behavior and health in the octopus. ALTEX 31, 494–499. doi: 10.14573/altex.1401282
Anderson, R. C., Wood, J. B., and Byrne, R. A. (2002). Octopus senescence: the beginning of the end. J. Appl. Anim. Welfare Sci. 5, 275–283. doi: 10.1207/S15327604JAWS0504_02
Andrews, P. L., Darmaillacq, A.-S., Dennison, N., Gleadall, I. G., Hawkins, P., Messenger, J. B., et al. (2013). The identification and management of pain, suffering and distress in cephalopods, including anaesthesia, analgesia and humane killing. J. Exp. Mar. Biol. Ecol. 447, 46–64. doi: 10.1016/j.jembe.2013.02.010
Andrews, P., Messenger, J., and Tansey, E. (1983). The chromatic and motor effects of neurotransmitter injection in intact and brain-lesioned octopus. J. Mar. Biol. Assoc. U. K. 63, 355–370. doi: 10.1017/S0025315400070739
Barry, P. D., Tamone, S. L., and Tallmon, D. A. (2011). A comparison of tagging methodology for North Pacific giant octopus Enteroctopus dofleini. Fish. Res. 109, 370–372. doi: 10.1016/j.fishres.2011.02.011
Bello, G. (1991). “Growth by weight versus growth by length in cephalopods,” in Squid age Determination using Statoliths. Proceeding of an International Workshop, eds P. Jereb, S. Ragonese, and S. V. Boletzky (Mazara del Vallo: NTR-ITPP Spec. Publ.), 35–37.
Bidder, A. (1957). Evidence for an absorptive function in the liver of Octopus vulgaris Lam. Pubblicazioni Stazione Zoologica Napoli 29, 139–150.
Boal, J. G., Hylton, R. A., Gonzalez, S. A., and Hanlon, R. T. (1999). Effects of crowding on the social behavior of cuttlefish (Sepia officinalis). J. Am. Assoc. Lab. Anim. Sci. 38, 49–55.
Borrelli, L. (2007). Testing the Contribution of Relative Brain Size and Learning Capabilities on the Evolution of Octopus vulgaris and Other Cephalopods. Ph.D. Thesis, Stazione Zoologica Anton Dohrn; Open University.
Borrelli, L., and Fiorito, G. (2008). “Behavioral Analysis of learning and memory in cephalopods,” in Learning and Memory: A Comprehensive Reference, ed J. J. Byrne (Oxford: Academic Press), 605–627.
Boucher-Rodoni, R., and Mangold, K. (1985). Ammonia excretion during feeding and starvation in Octopus vulgaris. Mar. Biol. 86, 193–197. doi: 10.1007/BF00399026
Boucher-Rodoni, R., and Mangold, K. (1988). Comparative aspects of ammonia excretion in cephalopods. Malacologia 29, 145–151.
Boyle, P., and Knobloch, D. (1982). On growth of the octopus Eledone cirrhosa. J. Mar. Biol. Assoc. U. K. 62, 277–296. doi: 10.1017/S0025315400057283
Byrne, R. A., Wood, J. B., Anderson, R. C., Griebel, U., and Mather, J. A. (2010). Non-invasive methods of identifying and tracking wild squid. Ferrantia 59, 22–31.
Capaz, J. C., Tunnah, L., Maccormack, T. J., Lamarre, S. G., Sykes, A. V., and Driedzic, W. R. (2017). Hypoxic induced decrease in oxygen consumption in cuttlefish (Sepia officinalis) is associated with minor increases in mantle octopine but no changes in markers of protein turnover. Front. Physiol. 8:344. doi: 10.3389/fphys.2017.00344
Carere, C., Grignani, G., Bonanni, R., Della Gala, M., Carlini, A., Angeletti, D., et al. (2015). Consistent individual differences in the behavioural responsiveness of adult male cuttlefish (Sepia officinalis). Appl. Anim. Behav. Sci. 167, 89–95. doi: 10.1016/j.applanim.2015.03.005
Castellanos-Martínez, S., Arteta, D., Catarino, S., and Gestal, C. (2014a). De novo transcriptome sequencing of the Octopus vulgaris hemocytes using Illumina RNA-Seq technology: response to the infection by the gastrointestinal parasite Aggregata octopiana. PLoS ONE 9:e107873. doi: 10.1371/journal.pone.0107873
Castellanos-Martínez, S., Diz, A. P., Álvarez-Chaver, P., and Gestal, C. (2014b). Proteomic characterization of the hemolymph of Octopus vulgaris infected by the protozoan parasite Aggregata octopiana. J. Proteomics 105, 151–163. doi: 10.1016/j.jprot.2013.12.008
Correia, M., Domingues, P. M., Sykes, A., and Andrade, J. P. (2005). Effects of culture density on growth and broodstock management of the cuttlefish, Sepia officinalis (Linnaeus, 1758). Aquaculture 245, 163–173. doi: 10.1016/j.aquaculture.2004.12.017
Cortez, T., González, A. F., and Guerra, A. (1999). Growth of Octopus mimus (Cephalopoda, Octopodidae) in wild populations. Fish. Res. 42, 31–39. doi: 10.1016/S0165-7836(99)00040-5
Delgado, M., Gairín, J. I., Carbó, R., and Aguilera, C. (2010). Growth of Octopus vulgaris (Cuvier, 1797) in tanks in the Ebro Delta (NE Spain): effects of temperature, salinity and culture density. Sci. Mar. 75, 53–59. doi: 10.3989/scimar.2011.75n1053
Di Cristina, G., Andrews, P., Ponte, G., Galligioni, V., and Fiorito, G. (2015). The impact of Directive 2010/63/EU on cephalopod research. Invert. Neurosci. 15:8. doi: 10.1007/s10158-015-0183-y
Domingues, P. M., Sykes, A., and Andrade, J. P. (2002). The effects of temperature in the life cycle of two consecutive generations of the cuttlefish Sepia officinalis (Linnaeus, 1758), cultured in the Algarve (South Portugal). Aquacult. Int. 10, 207–220. doi: 10.1023/A:1022148802078
Domingues, P., and Márquez, L. (2010). Effects of culture density and bottom area on growth and survival of the cuttlefish Sepia officinalis (Linnaeus, 1758). Aquacult. Int. 18, 361–369. doi: 10.1007/s10499-009-9249-3
Domingues, P., Garcia, S., and Garrido, D. (2010). Effects of three culture densities on growth and survival of Octopus vulgaris (Cuvier, 1797). Aquacult. Int. 18, 165–174. doi: 10.1007/s10499-008-9233-3
Estefanell, J., Socorro, J., Afonso, J., Roo, J., Fernández-Palacios, H., and Izquierdo, M. (2011). Evaluation of two anaesthetic agents and the passive integrated transponder tagging system in Octopus vulgaris (Cuvier 1797). Aquac. Res. 42, 399–406. doi: 10.1111/j.1365-2109.2010.02634.x
Fiorito, G., Affuso, A., Anderson, D. B., Basil, J., Bonnaud, L., Botta, G., et al. (2014). Cephalopods in neuroscience: regulations, Research and the 3Rs. Invert. Neurosci. 14, 13–36. doi: 10.1007/s10158-013-0165-x
Fiorito, G., Affuso, A., Basil, J., Cole, A., De Girolamo, P., D'angelo, L., et al. (2015). Guidelines for the care and welfare of cephalopods in research–A consensus based on an initiative by CephRes, FELASA and the Boyd Group. Lab. Anim. 49, 1–90. doi: 10.1177/0023677215580006
Forsythe, J., Lee, P., Walsh, L., and Clark, T. (2002). The effects of crowding on growth of the European cuttlefish, Sepia officinalis Linnaeus, 1758 reared at two temperatures. J. Exp. Mar. Biol. Ecol. 269, 173–185. doi: 10.1016/S0022-0981(02)00006-0
Fritscher-Ravens, A., Schuppan, D., Ellrichmann, M., Schoch, S., Röcken, C., Brasch, J., et al. (2014). Confocal endomicroscopy shows food-associated changes in the intestinal mucosa of patients with irritable Bowel syndrome. Gastroenterology 147, 1012.e1014–1020.e1014. doi: 10.1053/j.gastro.2014.07.046
García, S., Domíngues, P., Navarro, J. C., Hachero, I., Garrido, D., and Rosas, C. (2011). Growth, partial energy balance, mantle and digestive gland lipid composition of Octopus vulgaris (Cuvier, 1797) fed with two artificial diets. Aquacult. Nutr. 17, e174–e187. doi: 10.1111/j.1365-2095.2009.00746.x
García-Garrido, S., Hachero-Cruzado, I., Garrido, D., Rosas, C., and Domingues, P. (2010). Lipid composition of the mantle and digestive gland of Octopus vulgaris juveniles (Cuvier, 1797) exposed to prolonged starvation. Aquacult. Int. 18, 1223–1241. doi: 10.1007/s10499-010-9335-6
Gestal, C., and Castellanos-Martínez, S. (2015). Understanding the cephalopod immune system based on functional and molecular evidence. Fish Shellfish Immunol. 46, 120–130. doi: 10.1016/j.fsi.2015.05.005
Gonçalves, R. A., Aragão, C., Frias, P. A., and Sykes, A. V. (2012). The use of different anaesthetics as welfare promoters during short-term human manipulation of European cuttlefish (Sepia officinalis) juveniles. Aquaculture 370, 130–135. doi: 10.1016/j.aquaculture.2012.10.014
Graindorge, N., Jozet-Alves, C., Chichery, R., Dickel, L., and Bellanger, C. (2008). Does kainic acid induce partial brain lesion in an invertebrate model: Sepia officinalis? Comparison with electrolytic lesion. Brain Res. 1238, 44–52. doi: 10.1016/j.brainres.2008.08.002
Grasso, F. W., and Basil, J. A. (2009). The evolution of flexible behavioral repertoires in cephalopod molluscs. Brain Behav. Evol. 74, 231–245. doi: 10.1159/000258669
Grimaldi, A. M., Agnisola, C., and Fiorito, G. (2007). Using ultrasound to estimate brain size in the cephalopod Octopus vulgaris Cuvier in vivo. Brain Res. 1183, 66–73. doi: 10.1016/j.brainres.2007.09.032
Grimaldi, A. M., Belcari, P., Pagano, E., Cacialli, F., and Locatello, L. (2013). Immune responses of Octopus vulgaris (Mollusca: Cephalopoda) exposed to titanium dioxide nanoparticles. J. Exp. Mar. Biol. Ecol. 447, 123–127. doi: 10.1016/j.jembe.2013.02.018
Grisley, M., Boyle, P., Pierce, G., and Key, L. (1999). Factors affecting prey handling in lesser octopus (Eledone cirrhosa) feeding on crabs (Carcinus maenas). J. Mar. Biol. Assoc. U. K. 79, 1085–1090. doi: 10.1017/S0025315499001332
Guerra, A., and Nixon, M. (1987). Crab and mollusc shell drilling by Octopus vulgaris (Mollusca: Cephalopoda) in the Ria de Vigo (north-west Spain). J. Zool. 211, 515–523. doi: 10.1111/j.1469-7998.1987.tb01549.x
Heinzel, H. G., Weimann, J. M., and Marder, E. (1993). The behavioral repertoire of the gastric mill in the crab, Cancer pagurus: an in situ endoscopic and electrophysiological examination. J. Neurosci. 13, 1793–1803.
Huffard, C. L., Caldwell, R. L., Deloach, N., Gentry, D. W., Humann, P., Macdonald, B., et al. (2008). Individually unique body color patterns in octopus (Wunderpus photogenicus) allow for photoidentification. PLoS ONE 3:e3732. doi: 10.1371/journal.pone.0003732
ICES (2014). Report of the Working Group on Cephalopod Fisheries and Life History (WGCEPH). 16–19 June 2014, Lisbon, Portugal. ICES International Council for the Exploration of the Sea, Copenhagen.
Ikeda, Y., Sugimoto, C., Yonamine, H., and Oshima, Y. (2009). Method of ethanol anaesthesia and individual marking for oval squid (Sepioteuthis lessoniana Férussac, 1831 in Lesson 1830–1831). Aquac. Res. 41, 157–160. doi: 10.1111/j.1365-2109.2009.02305.x
Joll, L. (1977). Growth and food intake of Octopus tetricus (Mollusca: Cephalopoda) in aquaria. Mar. Freshw. Res. 28, 45–56. doi: 10.1071/MF9770045
Katsanevakis, S., Stephanopoulou, S., Miliou, H., Moraitou-Apostolopoulou, M., and Verriopoulos, G. (2005). Oxygen consumption and ammonia excretion of Octopus vulgaris (Cephalopoda) in relation to body mass and temperature. Mar. Biol. 146, 725–732. doi: 10.1007/s00227-004-1473-9
Kim, J. W., and Shin, S. S. (2017). Ultrasound-guided percutaneous core needle biopsy of abdominal viscera: tips to ensure safe and effective biopsy. Korean J. Radiol. 18, 309–322. doi: 10.3348/kjr.2017.18.2.309
King, A. J., and Adamo, S. A. (2006). The ventilatory, cardiac and behavioural responses of resting cuttlefish (Sepia officinalis L.) to sudden visual stimuli. J. Exp. Biol. 209, 1101–1111. doi: 10.1242/jeb.02116
King, A. J., Henderson, S. M., Schmidt, M. H., Cole, A. G., and Adamo, S. A. (2005). Using ultrasound to understand vascular and mantle contributions to venous return in the cephalopod Sepia officinalis L. J. Exp. Biol. 208, 2071–2082. doi: 10.1242/jeb.01575
Kröger, B., Vinther, J., and Fuchs, D. (2011). Cephalopod origin and evolution: a congruent picture emerging from fossils, development and molecules. Bioessays 33, 602–613. doi: 10.1002/bies.201100001
Lamarre, S. G., Ditlecadet, D., Mckenzie, D. J., Bonnaud, L., and Driedzic, W. R. (2012). Mechanisms of protein degradation in mantle muscle and proposed gill remodeling in starved Sepia officinalis. Am. J. Physiol. Regul. Integr. Comp. Physiol. 303, R427–R437. doi: 10.1152/ajpregu.00077.2012
Lamarre, S. G., Maccormack, T. J., Sykes, A. V., Hall, J. R., Speers-Roesch, B., Callaghan, N. I., et al. (2016). Metabolic rate and rates of protein turnover in food-deprived cuttlefish, Sepia officinalis (Linnaeus 1758). Am. J. Physiol. Regul. Integr. Comp. Physiol. 310, R1160–R1168. doi: 10.1152/ajpregu.00459.2015
Larson, S. E., and Anderson, R. C. (2010). Fecal hormones measured within giant pacific octopuses Enteroctopus dofleini. J. Aquat. Anim. Health 22, 152–157. doi: 10.1577/H09-027.1
Lee, P. (1995). Nutrition of cephalopods: fueling the system. Mar. Freshw. Behav. Physiol. 25, 35–51. doi: 10.1080/10236249409378906
Lee, P. G., Forsythe, J. W., Dimarco, P. F., Derusha, R. H., and Hanlon, R. T. (1991). Initial palatability and growth trials on pelleted diets for cephalopods. Bull. Mar. Sci. 49, 362–372.
Linares, M., Caamal-Monsreal, C., Olivares, A., Sánchez, A., Rodríguez, S., Zúñiga, O., et al. (2015). Timing of digestion, absorption and assimilation in octopus species from tropical (Octopus maya) and subtropical-temperate (O. mimus) ecosystems. Aquat. Biol. 24, 127–140. doi: 10.3354/ab00642
Locatello, L., Fiorito, G., Finos, L., and Rasotto, M. B. (2013). Behavioural and immunological responses to an immune challenge in Octopus vulgaris. Physiol. Behav. 122, 93–99. doi: 10.1016/j.physbeh.2013.08.029
Lopes, V. M., Baptista, M., Repolho, T., Rosa, R., and Costa, P. R. (2014). Uptake, transfer and elimination kinetics of paralytic shellfish toxins in common octopus (Octopus vulgaris). Aquat. Toxicol. 146, 205–211. doi: 10.1016/j.aquatox.2013.11.011
Malham, S., Coulson, C., and Runham, N. (1998). Effects of repeated sampling on the haemocytes and haemolymph of Eledone cirrhosa (Lam.). Comp. Biochem. Physiol. A Mol. Integr. Physiol. 121, 431–440. doi: 10.1016/S1095-6433(98)10154-X
Mangold, K. (1983). Food, feeding and growth in cephalopods. Memoirs Natl. Museum Victoria 44, 81–93.
Mangold, K., and Bidder, A. (1989). L'appareil digestif et la digestion. Traité Zool. Anat. Syst. Biol. Céphalopodes 5, 321–373.
Mangold, K., and Boletzky, S. V. (1973). New data on reproductive biology and growth of Octopus vulgaris. Mar. Biol. 19, 7–12. doi: 10.1007/BF00355414
Margheri, L., Ponte, G., Mazzolai, B., Laschi, C., and Fiorito, G. (2011). Non-invasive study of Octopus vulgaris arm morphology using ultrasound. J. Exp. Biol. 214, 3727–3731. doi: 10.1242/jeb.057323
Mayo-Hernández, E., Barcala, E., Berriatua, E., García-Ayala, A., and Munoz, P. (2013). Aggregata (Protozoa: Apicomplexa) infection in the common octopus Octopus vulgaris from the West Mediterranean Sea: the infection rates and possible effect of faunistic, environmental and ecological factors. J. Sea Res. 83, 195–201. doi: 10.1016/j.seares.2013.04.001
Moccia, R., Wilkie, E., Munkittrick, K., and Thompson, W. (1984). The use of fine needle fibre endoscopy in fish for in vivo examination of visceral organs, with special reference to ovarian evaluation. Aquaculture 40, 255–259. doi: 10.1016/0044-8486(84)90193-5
Nixon, M. (1966). Changes in body weight and intake of food by Octopus vulgaris. J. Zool. 150, 1–9. doi: 10.1111/j.1469-7998.1966.tb02995.x
Nixon, M. (1971). Some parameters of growth in Octopus vulgaris. J. Zool. 163, 277–284. doi: 10.1111/j.1469-7998.1971.tb04535.x
Oestmann, D. J., Scimeca, J. M., Forsythe, J., Hanlon, R., and Lee, P. (1997). Special considerations for keeping cephalopods in laboratory facilities. J. Am. Assoc. Lab. Anim. Sci. 36, 89–93.
Packard, A. (1972). Cephalopods and fish: the limits of convergence. Biol. Rev. 47, 241–307. doi: 10.1111/j.1469-185X.1972.tb00975.x
Penicaud, V., Lacoue-Labarthe, T., and Bustamante, P. (2017). Metal bioaccumulation and detoxification processes in cephalopods: a review. Environ. Res. 155, 123–133. doi: 10.1016/j.envres.2017.02.003
Pierce, G., Key, L., Boyle, P., Siegert, K., Goncalves, J., Porteiro, F., et al. (1999). RNA concentration and the RNA to protein ratio in cephalopod tissues: sources of variation and relationship with growth rate. J. Exp. Mar. Biol. Ecol. 237, 185–201. doi: 10.1016/S0022-0981(99)00008-8
Segawa, S., and Hanlon, R. T. (1988). Oxygen consumption and ammonia excretion rates in Octopus maya, Loligo forbesi and Lolliguncula brevis (Mollusca: Cephalopoda). Mar. Freshw. Behav. Phyisol. 13, 389–400. doi: 10.1080/10236248809378687
Semmens, J., Pecl, G., Villanueva, R., Jouffre, D., Sobrino, I., Wood, J., et al. (2004). Understanding octopus growth: patterns, variability and physiology. Mar. Freshw. Res. 55, 367–377. doi: 10.1071/MF03155
Shaw, T. J., Osborne, M., Ponte, G., Fiorito, G., and Andrews, P. L. (2016). Mechanisms of wound closure following acute arm injury in Octopus vulgaris. Zool. Lett. 2:8. doi: 10.1186/s40851-016-0044-5
Sinn, D. L., and Moltschaniwskyj, N. A. (2005). Personality traits in Dumpling squid (Euprymna tasmanica): context-specific traits and their correlation with biological characteristics. J. Comp. Psychol. 119, 99–110. doi: 10.1037/0735-7036.119.1.99
Sinn, D. L., Perrin, N. A., Mather, J. A., and Anderson, R. C. (2001). Early temperamental traits in an octopus (Octopus bimaculoides). J. Comp. Psychol. 115, 351–364. doi: 10.1037/0735-7036.115.4.351
Sivashanthini, K., Charles, G., and Thulasitha, W. (2009). Length-weight relationship and growth pattern of Sepioteuthis lessoniana Lesson 1830 (Cephalopoda: Teuthida) from the Jaffna Lagoon, Sri Lanka. J. Biol. Sci. 9, 357–361. doi: 10.3923/jbs.2009.357.361
Sladakovic, I., Ellis, A. E., and Divers, S. J. (2017). Evaluation of gastroscopy and biopsy of the proventriculus and ventriculus in pigeons (Columba livia). Am. J. Vet. Res. 78, 42–49. doi: 10.2460/ajvr.78.1.42
Smith, J. A., Andrews, P. L. R., Hawkins, P., Louhimies, S., Ponte, G., and Dickel, L. (2013). Cephalopod research and EU Directive 2010/63/EU: requirements, impacts and ethical review. J. Exp. Mar. Biol. Ecol. 447, 31–45. doi: 10.1016/j.jembe.2013.02.009
Speers-Roesch, B., Callaghan, N. I., Maccormack, T. J., Lamarre, S. G., Sykes, A. V., and Driedzic, W. R. (2016). Enzymatic capacities of metabolic fuel use in cuttlefish (Sepia officinalis). J. Comp. Physiol. B 186, 711–725. doi: 10.1007/s00360-016-0991-3
Sykes, A. V., Domingues, P. M., Loyd, M., Sommerfield, A., and Andrade, J. P. (2003). The influence of culture density and enriched environments on the first stage culture of young cuttlefish, Sepia officinalis (Linnaeus, 1758). Aquacult. Int. 11, 531–544. doi: 10.1023/B:AQUI.0000013262.15437.e4
Sykes, A. V., Domingues, P., and Andrade, J. P. (2014). “Sepia officinalis,” in Cephalopod Culture, eds J. Iglesias, L. Fuentes, and R. Villanueva (Dordrecht: Springer), 175–204.
Sykes, A. V., Gonçalves, R. A., and Andrade, J. P. (2013a). Early weaning of cuttlefish (Sepia officinalis, L.) with frozen grass shrimp (Palaemonetes varians) from the first day after hatching. Aquac. Res. 44, 1815–1823. doi: 10.1111/j.1365-2109.2012.03186.x
Sykes, A. V., Pereira, D., Rodríguez, C., Lorenzo, A., and Andrade, J. P. (2013b). Effects of increased tank bottom areas on cuttlefish (Sepia officinalis, L.) reproduction performance. Aquac. Res. 44, 1017–1028. doi: 10.1111/j.1365-2109.2012.03106.x
Sykes, A., Alves, A., Capaza, J. C., Madeira, C., Couto, A. T., Gonçalves, R. A., et al. (2017). Refining tools for studying cuttlefish (Sepia officinalis) reproduction in captivity: in vivo sexual determination, tagging and DNA collection. Aquaculture 479, 13–16. doi: 10.1016/j.aquaculture.2017.05.021
Taki, I. (1941). On keeping octopods in an aquarium for physiological experiments, with remarks on some operative techniques. Venus Jpn. J. Malacol. 10, 139–156.
Valverde, J. C., and García, B. G. (2005). Suitable dissolved oxygen levels for common octopus (Octopus vulgaris Cuvier, 1797) at different weights and temperatures: analysis of respiratory behaviour. Aquaculture 244, 303–314. doi: 10.1016/j.aquaculture.2004.09.036
Villanueva, R., and Norman, M. D. (2008). “Biology of the planktonic stages of benthic octopuses,” in Oceanography and Marine Biology: An Annual Review, eds R. Gibson, R. Atkinson, and J. Gordon (Boca Raton, FL: Taylor & Francis), 105–202.
Warnke, K. (1994). Some aspects of social interaction during feeding in Sepia officinalis (Mollusca: Cephalopoda) hatched and reared in the laboratory. Vie Milieu 44, 125–131.
Wells, M. J. (1978). Octopus: Physiology and Behaviour of an Advanced Invertebrate. London: Chapman and Hall.
Wells, M., and Wells, J. (1989). Water uptake in a cephalopod and the function of the so-called “pancreas.” J. Exp. Biol. 145, 215–226.
Wells, M., and Wells, J. (1993). Fluid uptake and the maintenance of blood volume in Octopus. J. Exp. Biol. 175, 211–218.
Westermann, B., Ruth, P., Litzlbauer, H. D., Beck, I., Beuerlein, K., Schmidtberg, H., et al. (2002). The digestive tract of Nautilus pompilius (Cephalopoda, Tetrabranchiata): an X-ray analytical and computational tomography study on the living animal. J. Exp. Biol. 205, 1617–1624.
Wodinsky, J. (1977). Hormonal inhibition of feeding and death in octopus: control by optic gland secretion. Science 198, 948–951. doi: 10.1126/science.198.4320.948
Xavier, J. C., Allcock, A. L., Cherel, Y., Lipinski, M. R., Pierce, G. J., Rodhouse, P. G., et al. (2015). Future challenges in cephalopod research. J. Mar. Biol. Assoc. U.K. 95, 999–1015. doi: 10.1017/S0025315414000782
Young, J. Z. (1967). The visceral nerves of Octopus. Philos. Trans. R. Soc. Lond. B Biol. Sci. 253, 1–22. doi: 10.1098/rstb.1967.0032
Zatylny-Gaudin, C., Cornet, V., Leduc, A., Zanuttini, B., Corre, E., Le Corguillé, G., et al. (2016). Neuropeptidome of the cephalopod Sepia officinalis: Identification, tissue mapping, and expression pattern of neuropeptides and neurohormones during egg laying. J. Proteome Res. 15, 48–67. doi: 10.1021/acs.jproteome.5b00463
Keywords: cephalopods, digestive tract, Directive 2010/63/EU, feces, food intake, nutrition, ultrasound, welfare assessment
Citation: Ponte G, Sykes AV, Cooke GM, Almansa E and Andrews PLR (2017) The Digestive Tract of Cephalopods: Toward Non-invasive In vivo Monitoring of Its Physiology. Front. Physiol. 8:403. doi: 10.3389/fphys.2017.00403
Received: 14 March 2017; Accepted: 29 May 2017;
Published: 19 June 2017.
Edited by:
Fernando Ariel Genta, Oswaldo Cruz Foundation, BrazilReviewed by:
Matthieu Dacher, Université Pierre et Marie Curie, FranceCopyright © 2017 Ponte, Sykes, Cooke, Almansa and Andrews. This is an open-access article distributed under the terms of the Creative Commons Attribution License (CC BY). The use, distribution or reproduction in other forums is permitted, provided the original author(s) or licensor are credited and that the original publication in this journal is cited, in accordance with accepted academic practice. No use, distribution or reproduction is permitted which does not comply with these terms.
*Correspondence: Giovanna Ponte, Z2lvdi5wb250ZUBnbWFpbC5jb20=
†These authors have contributed equally to this work.
Disclaimer: All claims expressed in this article are solely those of the authors and do not necessarily represent those of their affiliated organizations, or those of the publisher, the editors and the reviewers. Any product that may be evaluated in this article or claim that may be made by its manufacturer is not guaranteed or endorsed by the publisher.
Research integrity at Frontiers
Learn more about the work of our research integrity team to safeguard the quality of each article we publish.