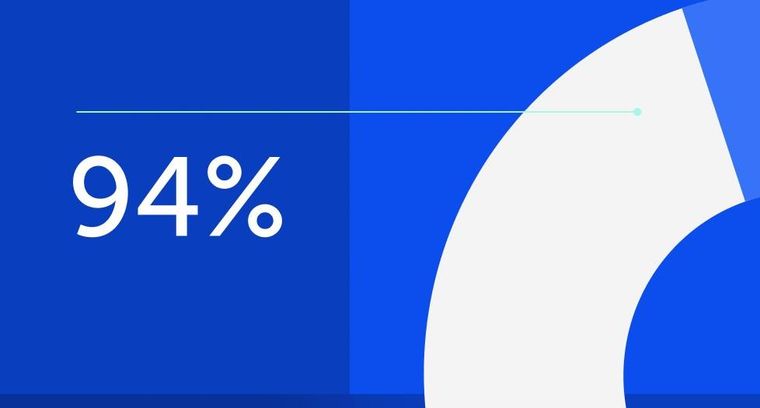
94% of researchers rate our articles as excellent or good
Learn more about the work of our research integrity team to safeguard the quality of each article we publish.
Find out more
REVIEW article
Front. Physiol., 09 June 2017
Sec. Membrane Physiology and Membrane Biophysics
Volume 8 - 2017 | https://doi.org/10.3389/fphys.2017.00392
This article is part of the Research TopicInvolvements of TRP Channels and Oxidative Stress in PainView all 11 articles
According to the International Association for the Study of Pain (IASP) pain is characterized as an “unpleasant sensory and emotional experience associated with actual or potential tissue damage”. The TRP super-family, compressing up to 28 isoforms in mammals, mediates a myriad of physiological and pathophysiological processes, pain among them. TRP channel might be constituted by similar or different TRP subunits, which will result in the formation of homomeric or heteromeric channels with distinct properties and functions. In this review we will discuss about the function of TRPs in pain, focusing on TRP channles that participate in the transduction of noxious sensation, especially TRPV1 and TRPA1, their expression in nociceptors and their sensitivity to a large number of physical and chemical stimuli.
Cytosolic free Ca2+ concentration ([Ca2+]c) is a key factor for the regulation of a large variety of cellular functions, ranging from short-term processes, such as muscle contraction, exocytosis, or platelet aggregation, to long-term events, including cell proliferation or apoptosis (Berridge et al., 2000). Physiological agonists modulate [Ca2+]c through the regulation of a number of Ca2+ transport mechanisms, based on the activation of more or less Ca2+ selective channels and transporters. Agonist-induced Ca2+ mobilization consist, among others, of (1) the release of Ca2+ from agonist-sensitive Ca2+ stores, mostly the endoplasmic reticulum (ER) and acidic organelles (Lopez et al., 2005; Galione, 2006; Aulestia et al., 2011), (2) extracellular Ca2+ entry through plasma membrane permeable channels (Salido et al., 2009a), (3) cytosolic Ca2+ clearance either by Ca2+ uptake into intracellular stores (Lipskaia et al., 2014) or Ca2+ extrusion across the plasma membrane (Redondo et al., 2005), and (4) Ca2+ buffering with the participation of the mitochondria (Montero et al., 2001). While Ca2+ release from the finite intracellular Ca2+ compartments has been reported to regulate different cellular events, Ca2+ entry from the extracellular medium is required for the replenishment of the internal stores and also for full activation of different cellular functions. Ca2+ entry might occur through a variety of mechanisms, which might be grouped in voltage-operated and receptor-operated Ca2+ influx processes. In turn, according to the activation route, receptor-operated Ca2+ entry might be classified into receptor-mediated, second messenger-operated and store-operated Ca2+ entry mechanisms. The simplest mechanism is receptor-mediated Ca2+ influx, which occurs through channels allosterically regulated by agonist binding. Second messenger-operated Ca2+ entry requires the generation of a second messenger that directly gates the channel. On the other hand, store-operated Ca2+ entry (SOCE) is regulated by the filling state of the intracellular Ca2+ stores, mainly the ER (Putney, 1986), but also acidic organelles (Zbidi et al., 2011). According to this, a reduction in the intraluminal Ca2+ concentration results in the opening of channels in the plasma membrane (Putney, 1986).
Among the wide variety of Ca2+-permeable channels identified, TRP channels play a relevant functional role in mammalian cells. TRP channels were identified in a Drosophila mutant with visual defects, where a mutation in a channel permeable to Na+ and Ca2+ leads to transient, rather than sustained, receptor potential in the photoreceptors (Minke, 1977). Since the identification of the mammalian homologs of the Drosophila TRPs in 1995 (Wes et al., 1995; Zhu et al., 1995) these channels have been proposed as candidates to conduct both second messenger- as well as store-operated Ca2+ entry.
TRP channels are a group of ion channels located in the plasma membrane as well as in the membrane of a number of intracellular organelles, where they participate in the homeostasis of intracellular Ca2+, as well as other ions, such as Mg2+ (Fleig and Penner, 2004; Ambudkar et al., 2007; Salido et al., 2009b). Since TRP proteins were first described a number of isoforms have been identified, which are grouped into seven subfamilies: TRPC, TRPV, TRPM, TRPP, TRPML, TRPA, and TRPN (the latter only expressed in fish, flies, and worms) and each subfamily includes one or more members (Montell et al., 2002; Li et al., 2011).
The structure of TRP channels comprises six membrane-spanning helices with a pore-forming loop between the last two transmembrane segments. The N- and C-terminal segments are located in the cytosol and vary in the number of amino acids and the functional motifs among the different subfamilies. Thus, the N termini of TRPC, TRPA, TRPV, and TRPN subfamilies contain between 4 and 30 tandem copies of ankyrin repeat domains, involved in protein-protein interaction (Latorre et al., 2009). Furthermore, the cytoplasmic N and/or C-termini of TRPC, TRPM, TRPP, and TRPV channels have been reported to contain coiled coil domains, which play an important role in the assembly of homomeric and heteromeric complexes (Lepage and Boulay, 2007; Schindl and Romanin, 2007) as well as in the interaction with the ER Ca2+ sensor STIM1 (Lee et al., 2014). TRPC, TRPV, and TRPM subfamilies also contain a conserved TRP box, a short hydrophobic region located just C-terminal of the putative last transmembrane segment (Nilius et al., 2006). Certain TRP members are regulated by cytosolic Ca2+ through the interaction with C-terminal located EF-hand motifs, including TRPA1, TRPML1, and TRPP2 (Tsiokas, 2009), or calmodulin and IP3 receptor-binding regions, this is the case of TRPC, TRPM, and TRPV members (Tang et al., 2001; Dionisio et al., 2011). Finally, other more restricted motifs have been reported in different TRP members, including the tubulin-binding domain reported in TRPV1 (Sardar et al., 2012), the kinase domain of TRPM6 and TRPM7 (Schlingmann and Gudermann, 2005), the conserved proline-rich region, downstream of the EWKFAR motif, responsible for the interaction with Homer proteins and immunophilins (Yuan et al., 2003; Sinkins et al., 2004; Jardin et al., 2013; Lopez et al., 2013; Dionisio et al., 2015) or the voltage sensing domain reported in TRPV1, TRPV3, TRPM8, and TRPM4 (Nilius et al., 2003, 2005), among others.
TRP channels are activated and modulated by a wide variety of chemical and physical stimuli including receptor occupation via activation of phospholipase C, which, in turn, leads to the hydrolysis of phosphatidylinositol 4,5-bisphospate (PIP2) and the generation of lipid messengers, biosynthesis of IP3, and subsequent Ca2+ release from the intracellular stores, the activation of serine/threonine or tyrosine kinases or ligand binding, including exogenous ligands, such as capsaicin or allyl isothiocyanate, and endogenous molecules, including eicosanoids, diacylglycerol, phosphoinositides, purine nucleotides, or inorganic ions, such as Ca2+ and Mg2+ (Harteneck et al., 2011; Vetter and Lewis, 2011).
The sensitivity of TRP channels to a number of physical and chemical stimuli allows these channels to be essential components of different sensory processes, such as vision, hearing, taste, tactile and thermal sensation, redox status, or pain (Voets et al., 2005; Woodard et al., 2007; Wetsel, 2011; Feng, 2014; Ogawa et al., 2016).
Pain is a subjective unpleasant sensory experience that might be associated to real or potential damage. Noxious stimuli are detected by pain receptors or nociceptors, nerve endings that specifically respond to damaging stimuli and transmit the information to the spinal cord, through which the message is transmitted to higher nerve centers, including the brain stem reticular formation, thalamus, somatosensory cortex, and limbic system (Osterweis et al., 1987). Nociception, therefore, is the process of transmission of noxious signals by nociceptors in the primary afferent nerve fibers (Dai, 2016). Noxious stimuli are classified into chemical, mechanical and thermal. The transduction of nociception includes several chemical compounds that might be released by damaged tissue, such as K+, histamine and serotonin, or generated by enzymes activated by tissue damage, including prostaglandins, leukotrienes, or bradikinin (Schaible et al., 2011; Viguier et al., 2013).
A major function of the nociceptors is to detect potentially damaging stimuli with a threshold that allows perform activities without pain but sensitive enough to warn of the risk of damage (Patapoutian et al., 2009). The detection of noxious stimuli by nociceptors involves the expression of nociceptive ion channels, which basically define the functional properties of nociceptors. The largest group of nociceptive ion channels is the TRP channel family (Clapham, 2003; Patapoutian et al., 2009), especially TRPV1 and TRPA1 members. Activation of nociceptive TRP ion channels in sensitive (i.e., dorsal root ganglion, DRG) neurons leads to the influx of Na+ and Ca2+ across the plasma membrane resulting in membrane depolarization that, in turn, might trigger voltage-gated ion channel-dependent action potentials (Gees et al., 2010) that transmit the information to the spinal cord and the higher nerve centers as described above.
TRPV1 is one of the six members of the TRPV subfamily and is involved in the detection of noxious sensation (Caterina and Julius, 2001). TRPV1 has been found to be highly expressed in the plasma membrane of nociceptive DRG neurons (Caterina and Julius, 2001). Furthermore, functional expression of this channel has also been reported to be expressed in the ER of DRG neurons, where it is involved in Ca2+ efflux from the ER upon stimulation with vanilloids; although its sensitivity to agonists is smaller when located in the ER membrane probably due to a mechanism mediated by calmodulin, which might be important for neuronal biology (Gallego-Sandin et al., 2009). The structure of TRPV1 follows the pattern of the TRP channels, with six transmembrane spanning domains, six ankyrin repeats in the N-terminus and a large C-terminal region (Cao et al., 2013; Figure 1). Three splice variants of TRPV1 have been described: VR.5'sv, TRPV1b, and TRPV1var. VR.5'sv (vanilloid receptor 5' splice variant) shows a shorter N-terminal region due to both an alternative initiation of translation and the lack of transcription of an exon resulting in loss of 60 amino acids in the N-terminus (Schumacher et al., 2000) and do not respond to capsaicin (Eilers et al., 2007). TRPV1b shows a modification in the N-terminal region encoded by exon 7 that leads to loss of 10 amino acids (Wang et al., 2004) and, as well as the VR.5'sv variant, has been propose to function as a dominant-negative channel subunit (Pecze et al., 2008; Schumacher and Eilers, 2010). TRPV1var is generated by a failure to splice out intron 5, thus leading to translation of a portion of the N-terminal region that lacks the transmembrane spanning domains and the C-terminal intracellular region (Tian et al., 2006). It has been reported that TRPV1var, when coexpressed with the full-length TRPV1 subunits, might modulate its responses, for instance, it has been shown to increase the response of TRPV1 to resiniferatoxin (Tian et al., 2006).
Figure 1. Molecular structure of TRPA1 and TRPV1. (A) Scheme of TRPV1 and TRPA1 channels depicting individual domains. Numbers correspond to amino acids positions of human TRPV1 and TRPA1, respectively. (B) Cartoon representing TRPV1 and TRPA1 monomers morphology within a bilayer membrane. The channel spans the membrane up to six times with the pore located between transmembrane domains (TM) 5 and 6, and both N-terminal and C-terminal domains situated in the cytosol. (C,D) Cartoons depicting the tetrameric assembly of TRPV1 subunits based on the X-ray crystal structure of Rattus norvegicus as described in Cao et al. (2013). As mentioned above, the ion permeation pathway is formed by TM5 and TM6, while the remaining TM domains 1–4, surround the pore. (C) Represents a frontal view of the channel while (D) sketches an upside-down perspective.
TRPV1 is a polymodal channel sensitive to different physical and chemical stimuli, including heat (see above), pH under 5.9 (Tominaga et al., 1998), and mechanical stimuli (Walker et al., 2003). In addition, TRPV1 is activated by a variety of ligands (Table 1) including vanilloids, such as capsaicinoids (the most representative is capsaicin, the major pungent constituent of Capsicum fruit; Caterina et al., 1997) and resiniferanoids (Szallasi and Blumberg, 1989), α, β-unsaturated dialdehydes isolated from a variety of plants, fungi, algae, sponges, arthropods, and molluscs (Jonassohn et al., 1995), cannabinoids from Cannabis sativa (Bisogno et al., 2001), ginsenosides found in the ginseng Panax ginseng (Jung et al., 2001), a number of animal-derived toxins, such as VaTx1, VaTx2, and VaTx3 found in the venom of the tarantula Psalmopoeus cambridgei activates TRPV1 channels (Siemens et al., 2006) while other toxins, including agatoxin 489 and agatoxin 505, from the spider Agelenopsis aperta, and the analgesic polypeptide HC1, from the see anemona Heteractis crispa, elicits TRPV1 inhibition (Kitaguchi and Swartz, 2005; Andreev et al., 2008). Furthermore, a number of endogenous molecules, known as endovanilloids, including leukotriene B4 and 12-S-HPETE and anandamide (a cannabinoid receptor agonist) have been found to be potent activators of TRPV1 channels (Di Marzo et al., 2002; Hermann et al., 2003). On the other hand, alkaloids, such as nicotine (from Nicotiana tabacum) or yohimbine (from the bark of the tree Pausinystalia yohimbe), phenols like grifolin, neogrifolin, and albaconol (present in the mushroom Albatrellus confluens) or acetylsalicylic acid have been found to inhibit the activity of TRPV1 channels, for a review see Vetter and Lewis (2011). Endogenous modulators of TRPV1 activity include noradrenaline, which is able to attenuate capsaicin-activated response by ~60%, a mechanism mediated by activation of α2 adrenergic receptors that has been reported to underlie the inhibition of the incoming noxious stimuli at the dorsal horn of the spinal cord (Chakraborty et al., 2017).
TRPA1, also known as P120 and ANKTM1, is the sole member of the TRPA subfamily. It was first described in human fibroblasts where its expression is lost after oncogenic transformation (Jaquemar et al., 1999). TRPA1 has been found to be expressed in peptidergic nociceptors, as well as in a number of non-neuronal cells, including keratinocytes (Atoyan et al., 2009), megakaryocytes (Albarran et al., 2013) or enterochromaffin cells (Nozawa et al., 2009), and tissues (for a review see Benemei et al., 2014).
In addition to TRPV1, TRPA1 is specialized in the transduction of noxious stimuli in mammals. In fact, a certain degree of interaction between both channels has been reported. TRPV1 is expressed in most TRPA1-expressing neurons and about 30% of TRPV1-expressing sensory neurons also exhibit TRPA1 expression (Story et al., 2003). Furthermore, TRPV1 has been reported to influence several features of the TRPA1 channel, such as voltage–current relationships and open probability (Staruschenko et al., 2010). Further pieces of evidence for the functional interaction between both channels comes from studies reporting that the biophysical properties of TRPA1 are different when TRPA1 is expressed alone or coexpressed with TRPV1 and that the TRPV1 and TRPA1 agonists, capsaicin and mustard oil, are able to induce heterologously desensitization of TRPA1 and TRPV1 via calcineurin-dependent and independent pathways, respectively (Ruparel et al., 2008).
The structure of TRPA1 shows the features of the TRP family and consists of six membrane-spanning domains and a presumed pore-forming region between the fifth and sixth transmembrane domains. Its N- and C-terminal segments are predicted to be located in the cytoplasm (Figure 1). In addition, an unusual and characteristic feature of TRPA1 is the presence of a very long N-terminus, which contains at least 16 predicted ankyrin repeat domains (Story et al., 2003; Paulsen et al., 2015). It is the only mammalian TRP channel with such high number of ankyrin repeats, which might provide the protein a certain degree of elasticity, as well as, the ability to interact with other proteins, especially those of the cytoskeleton (Corey et al., 2004; Sotomayor et al., 2005).
TRPA1 is a polymodal ion channel that can be activated by a number of physical and chemical stimuli. Among the physical stimuli, TRPA1 is sensitive to temperature. The 10 thermo-TRP channels identified to date, including TRPV1-4, TRPM2, TRPM4, TRPM5, TRPM8, TRPC5, and TRPA1, are activated by different temperature ranges. The mammalian TRPs activated by heat are TRPV2 (activated at temperatures over 52°C), TRPV1 (sensitive to temperatures over 42°C), TRPV4 (activated by temperatures between 27 and 42°C), TRPV3 (by temperature over 33°C), TRPM2 (sensitive to temperatures between 35 and 42°C), TRPM4 and TRPM5 (sensitive to temperatures between 15 and 35°C). On the other hand, TRPC5 activity is potentiated at temperatures below 30°C, TRPM8 is sensitive to temperatures below 25°C and TRPA1 is activated at temperatures below 17°C (Caterina et al., 1999; Dhaka et al., 2006; Vriens et al., 2014). As TRPV1 has been associated to painful heat, TRPA1 has been reported to be associated to noxious cold sensation (Patapoutian et al., 2003). The thermal sensitivity of TRPA1 is conserved throughout evolution, although the range of temperatures that activates the channel differs among the distinct vertebrates, thus, in reptiles and amphibians TRPA1 is sensitive to heat and, in certain snakes, TRPA1 provides sufficient thermal sensitivity for infrared detection (Poletini et al., 2015; Kang, 2016).
In addition, TRPA1 can be activated by a number of chemical stimuli (Table 1), including exogenous compounds, such as isothiocyanates, cinnamaldehyde, and methyl salicylate (the pungent compounds associated to burning sensation present in mustard oil, wasabi, horseradish, cinnamon and wintergreen oil; Bandell et al., 2004), allicin, ajoene, and diallyl sulfides (organosulfur compounds present in garlic; Bautista et al., 2006), acrolein, and crotonaldehyde (present in cigarette smoke; Andre et al., 2008), cannabinoids, such as Δ9 tetra-hydrocannabinoland cannabinol (Jordt et al., 2004), alkylamides, including hydroxy-α-sanshool (one of the compounds of the Szechuan pepper; Riera et al., 2009; Vetter and Lewis, 2011), or endogenous compounds such as hydrogen peroxide (Trevisan et al., 2014), nitro-oleic acid, a byproduct of nitric oxide (Sculptoreanu et al., 2010), 4-hydroxy-2-nonenal (Trevisani et al., 2007), the cyclopentenone prostaglandin D2 metabolite 15-deoxy- Δ12, 14-prostaglandin J2 (Cruz-Orengo et al., 2008), and different epoxyeicosatrienoic acids (EET), including 5,6-EET (Sisignano et al., 2012) and 8,9-EET (Brenneis et al., 2011). By contrast, a number of TRPA1 antagonists have been identified, including the synthetic HC-030031, its derivative chembridge-5861528 or A-967079, among others (Table 1). Most exogenous compounds activate TRPA1 channels by covalent modification of cysteines and lysines in the N-terminus (Hinman et al., 2006; Macpherson et al., 2007; Nilius et al., 2011), although it remains to be determined the mechanism of activation of the channel by certain endogenous compounds.
In addition to TRPV1 and TRPA1, other TRP members have been associated to noxious sensation, including TRPM3, expressed in a number of small-diameter sensory neurons from dorsal root and trigeminal ganglia where it is involved in the nocifensive response to heat (Vriens et al., 2011), TRPV4, presented as an osmo-transducer in primary afferent nociceptive nerve fibers (Alessandri-Haber et al., 2003), TRPC1 and TRPC6, which cooperate with TRPV4 in the mediation of hyperalgesia to mechanical and hypotonic stimuli induced by inflammatory mediators (Alessandri-Haber et al., 2009), TRPV3, a channel sensitive to farnesyl pyrophosphate that is involved in the sensitivity to noxious heat (Bang et al., 2010) TRPM8, involved in cold hyperalgesia and tactile allodynia (Salat and Filipek, 2015), TRPC3, associated to the mediation of store- and receptor-operated Ca2+ entry in DRG neurons (Alkhani et al., 2014), TRPC4, which is required for the detection or transmission of colonic visceral pain sensation, and TRPC5, which, together with TRPC4, is relevant for pain hypersensitivity and neuropathic pain (Westlund et al., 2014; Wei et al., 2015); however, the involvement of these channels in pain detection or transmission has been less characterized than that of TRPV1 or TRPA1.
Nociceptive TRP channels have been found to be involved in a number of pain modalities, including inflammatory pain, neuropathic pain, visceral pain, and pain associated to certain pathological conditions, including cancer or migraine (Mickle et al., 2016).
The involvement of TRPV1 in inflammatory pain is the most prominent among the TRP channels. TRPV1 antagonists have been shown to be efficient attenuating thermal hyperalgesia induced under inflammatory conditions and increasing the noxious heat threshold (Tekus et al., 2010) and similar results have been obtained in TRPV1 lacking mice models (Davis et al., 2000). Further evidence supporting the role of TRPV1 in inflammatory pain comes from studies reporting that TRPV1 is essential for the analgesia induced by electroacupuncture in a mouse model of inflammatory pain (Liao et al., 2017). In addition to TRPV1, TRPA1 has been presented as a candidate to mediate inflammatory mechanical hyperalgesia as well as cold hyperalgesia under inflammatory conditions (Eid et al., 2008; Da Costa et al., 2010). Furthermore, TRPA1 has been reported to modulate inflammation and pruritogen responses in allergic contact dermatitis. TRPA1 is involved in skin edema, leukocyte infiltration and antihistamine-resistant scratching in mice treated with oxazolone (Liu et al., 2013).
Neuropathic pain occurs as a result of nerve injury. The role of TRPV1 has been demonstrated in neuropathic pain associated to diabetes or the administration of chemotherapeutics (Bourinet et al., 2014). A more recent study has revealed a high coexpression between TRPV1 and different sensitizing agents, such as PKCε, during the development of neuropathic pain (Malek et al., 2015) and blockade of this mechanism by quercetin has been found to attenuate paclitaxel-induced neuropathic pain (Gao et al., 2016). TRPA1 has also been proposed to mediate mechanical hyperalgesia and allodynia during neuropathic pain in diabetic patients or derived from the administration of chemotherapeutics, probably mediated by the synthesis of reactive oxygen and nitrogen species (Kim and Hwang, 2013; Huang et al., 2017), which are well-known TRPA1 activators (Trevisan et al., 2016). TRPA1 and TRPV1 have also been involved in the development of migraine, which can be activated by a number of TRPA1 agonists (Benemei et al., 2014) and might be attenuated by repeated desensitizing administration of capsaicin to the nasal mucosa (Fusco et al., 2003). Furthermore, ethanol, a well-known trigger of migraine, has been reported to induce TRPV1 activation (Nicoletti et al., 2008). Activation of TRP channels, such as TRPV1 and TRPA1, has been reported to induce the trigeminal calcitonin gene-related peptide pathway, which mediate neurogenic inflammation, thus leading to the migraine attacks (Benemei et al., 2013).
Visceral pain occurs in internal organs and its transduction involves different TRP family members, including TRPV1, TRPA1, and TRPM8. Silencing the expression of TRPV1 by RNAi has been reported to attenuate visceral pain in vivo (Christoph et al., 2006). Consistent with this, a more recent study has revealed that decreased expression of miR-199 in irritable bowel syndrome, which results in enhanced expression of TRPV1, leads to increased visceral hypersensitivity (Zhou et al., 2016). On the other hand, the luminal gasotransmitter hydrogen disulphide has been reported to induce colonic pain and hyperalgesia via activation of Cav3.2 and TRPA1 channels (Tsubota-Matsunami et al., 2012). Furthermore, the TRPA1 agonist ASP7663 has been reported to prevent constipation (a gastrointestinal motility disorder) when administered orally, and induce analgesic abdominal effects when it is intravenously administered (Kojima et al., 2014). Finally, TRPM8 has been found to play a relevant role in overactive bladder and painful bladder syndrome and it has been reported that administration of the TRPM8 channel blocker AMTB is able attenuate this syndrome in rats (Lashinger et al., 2008).
Chronic pain is also a multidimensional complication of cancer or its treatment. The role of TRPV1 in bone cancer pain has been widely investigated. TRPV1 has been found to be associated to bone cancer pain, as demonstrated by pharmacological inactivation of TRPV1 as well as disruption of the TRPV1 gene (Ghilardi et al., 2005). Furthermore, the TRPV1 antagonist SB366791 has been reported to potentiate the analgesic effect of intraperitoneal administration of morphine in a mouse model of bone cancer pain. The expression of TRPV1, as well as the TRPV1-dependent currents, have been found to be enhanced upon the development of bone cancer in DRG neurons. In these cells, capsaicin-mediated currents were potentiated by administration of lysophosphatidic acid through a mechanism dependent on PKCε but independent on PKA and the small GTPase Rho (Pan et al., 2010). Two more recent studes have revealed that the up-regulated expression and function of TRPV1 in bone cancer pain might be attributed to the the presence of tumor tissue-derived endogenous formaldehyde, which enhances TRPV1 expression via mitogen-activated protein kinase and PI3K, but independently on PKC (Han et al., 2012), as well as the regulatory effects of insulin-like growth factor-1 (Li et al., 2014). Finally, JAK/PI3K-dependent TRPV1 up-regulation has been reported to be involved in peripheral sensitization and bone cancer-induced pain evoked by interleukin-6 (Fang et al., 2015). TRPV1 and TRPA1 have also been found to be involved in neuropathic pain due to the administration of chemotherapeutics, including oxaliplatin (Park et al., 2015), 5-fluorouracil (Yamaguchi et al., 2016), or docetaxel (Huang et al., 2017).
Currently, there is a body of studies and clinical trials identifying new antagonists of the nociceptive TRPs and characterizing their effects in the in situ attenuation of pain transduction at the nociceptors.
JR, IJ, and JL drafted the manuscript. IJ performed the figure. RD, JS, CC, LA, and TS performed the bibliographic revision. GW, GS, and PR revised the manuscript and performed the final check.
The authors declare that the research was conducted in the absence of any commercial or financial relationships that could be construed as a potential conflict of interest.
This work was supported by MINECO (Grants BFU2013-45564-C2-1-P, BFU2013-45564-C2-2-P, BFU2016-74932-C2-1-P, and BFU2016-74932-C2-2-P) and Junta de Extremadura-FEDER (GR15029), JL is supported by Juan de la Cierva Program (JCI-2012-12934).
Albarran, L., Lopez, J. J., Dionisio, N., Smani, T., Salido, G. M., and Rosado, J. A. (2013). Transient receptor potential ankyrin-1 (TRPA1) modulates store-operated Ca2+ entry by regulation of STIM1-Orai1 association. Biochim. Biophys. Acta 1833, 3025–3034. doi: 10.1016/j.bbamcr.2013.08.014
Alessandri-Haber, N., Dina, O. A., Chen, X., and Levine, J. D. (2009). TRPC1 and TRPC6 channels cooperate with TRPV4 to mediate mechanical hyperalgesia and nociceptor sensitization. J. Neurosci. 29, 6217–6228. doi: 10.1523/JNEUROSCI.0893-09.2009
Alessandri-Haber, N., Yeh, J. J., Boyd, A. E., Parada, C. A., Chen, X., Reichling, D. B., et al. (2003). Hypotonicity induces TRPV4-mediated nociception in rat. Neuron 39, 497–511. doi: 10.1016/S0896-6273(03)00462-8
Alkhani, H., Ase, A. R., Grant, R., O'donnell, D., Groschner, K., and Seguela, P. (2014). Contribution of TRPC3 to store-operated calcium entry and inflammatory transductions in primary nociceptors. Mol. Pain 10:43. doi: 10.1186/1744-8069-10-43
Ambudkar, I. S., Ong, H. L., Liu, X., Bandyopadhyay, B. C., and Cheng, K. T. (2007). TRPC1: the link between functionally distinct store-operated calcium channels. Cell Calcium 42, 213–223. doi: 10.1016/j.ceca.2007.01.013
Andre, E., Campi, B., Materazzi, S., Trevisani, M., Amadesi, S., Massi, D., et al. (2008). Cigarette smoke-induced neurogenic inflammation is mediated by alpha, beta-unsaturated aldehydes and the TRPA1 receptor in rodents. J. Clin. Invest. 118, 2574–2582. doi: 10.1172/JCI34886
Andreev, Y. A., Kozlov, S. A., Koshelev, S. G., Ivanova, E. A., Monastyrnaya, M. M., Kozlovskaya, E. P., et al. (2008). Analgesic compound from sea anemone Heteractis crispa is the first polypeptide inhibitor of vanilloid receptor 1 (TRPV1). J. Biol. Chem. 283, 23914–23921. doi: 10.1074/jbc.M800776200
Atoyan, R., Shander, D., and Botchkareva, N. V. (2009). Non-neuronal expression of transient receptor potential type A1 (TRPA1) in human skin. J. Invest. Dermatol. 129, 2312–2315. doi: 10.1038/jid.2009.58
Aulestia, F. J., Redondo, P. C., Rodriguez-Garcia, A., Rosado, J. A., Salido, G. M., Alonso, M. T., et al. (2011). Two distinct calcium pools in the endoplasmic reticulum of HEK293T cells. Biochem. J. 435, 227–235. doi: 10.1042/BJ20101427
Bandell, M., Story, G. M., Hwang, S. W., Viswanath, V., Eid, S. R., Petrus, M. J., et al. (2004). Noxious cold ion channel TRPA1 is activated by pungent compounds and bradykinin. Neuron 41, 849–857. doi: 10.1016/S0896-6273(04)00150-3
Bang, S., Yoo, S., Yang, T. J., Cho, H., and Hwang, S. W. (2010). Farnesyl pyrophosphate is a novel pain-producing molecule via specific activation of TRPV3. J. Biol. Chem. 285, 19362–19371. doi: 10.1074/jbc.M109.087742
Bautista, D. M., Jordt, S. E., Nikai, T., Tsuruda, P. R., Read, A. J., Poblete, J., et al. (2006). TRPA1 mediates the inflammatory actions of environmental irritants and proalgesic agents. Cell 124, 1269–1282. doi: 10.1016/j.cell.2006.02.023
Behrendt, H. J., Germann, T., Gillen, C., Hatt, H., and Jostock, R. (2004). Characterization of the mouse cold-menthol receptor TRPM8 and vanilloid receptor type-1 VR1 using a fluorometric imaging plate reader (FLIPR) assay. Br. J. Pharmacol. 141, 737–745. doi: 10.1038/sj.bjp.0705652
Benemei, S., De Cesaris, F., Fusi, C., Rossi, E., Lupi, C., and Geppetti, P. (2013). TRPA1 and other TRP channels in migraine. J. Headache Pain 14:71. doi: 10.1186/1129-2377-14-71
Benemei, S., Fusi, C., Trevisan, G., and Geppetti, P. (2014). The TRPA1 channel in migraine mechanism and treatment. Br. J. Pharmacol. 171, 2552–2567. doi: 10.1111/bph.12512
Berridge, M. J., Lipp, P., and Bootman, M. D. (2000). The versatility and universality of calcium signalling. Nat. Rev. Mol. Cell Biol. 1, 11–21. doi: 10.1038/35036035
Bisogno, T., Hanus, L., De Petrocellis, L., Tchilibon, S., Ponde, D. E., Brandi, I., et al. (2001). Molecular targets for cannabidiol and its synthetic analogues: effect on vanilloid VR1 receptors and on the cellular uptake and enzymatic hydrolysis of anandamide. Br. J. Pharmacol. 134, 845–852. doi: 10.1038/sj.bjp.0704327
Bourinet, E., Altier, C., Hildebrand, M. E., Trang, T., Salter, M. W., and Zamponi, G. W. (2014). Calcium-permeable ion channels in pain signaling. Physiol. Rev. 94, 81–140. doi: 10.1152/physrev.00023.2013
Brenneis, C., Sisignano, M., Coste, O., Altenrath, K., Fischer, M. J., Angioni, C., et al. (2011). Soluble epoxide hydrolase limits mechanical hyperalgesia during inflammation. Mol. Pain 7:78. doi: 10.1186/1744-8069-7-78
Cao, E., Liao, M., Cheng, Y., and Julius, D. (2013). TRPV1 structures in distinct conformations reveal activation mechanisms. Nature 504, 113–118. doi: 10.1038/nature12823
Caterina, M. J., and Julius, D. (2001). The vanilloid receptor: a molecular gateway to the pain pathway. Annu. Rev. Neurosci. 24, 487–517. doi: 10.1146/annurev.neuro.24.1.487
Caterina, M. J., Rosen, T. A., Tominaga, M., Brake, A. J., and Julius, D. (1999). A capsaicin-receptor homologue with a high threshold for noxious heat. Nature 398, 436–441. doi: 10.1038/18906
Caterina, M. J., Schumacher, M. A., Tominaga, M., Rosen, T. A., Levine, J. D., and Julius, D. (1997). The capsaicin receptor: a heat-activated ion channel in the pain pathway. Nature 389, 816–824. doi: 10.1038/39807
Chakraborty, S., Elvezio, V., Kaczocha, M., Rebecchi, M., and Puopolo, M. (2017). Presynaptic inhibition of transient receptor potential vanilloid type 1 (TRPV1) receptors by noradrenaline in nociceptive neurons. J. Physiol. 595, 2639–2660. doi: 10.1113/JP273455
Christoph, T., Grunweller, A., Mika, J., Schafer, M. K., Wade, E. J., Weihe, E., et al. (2006). Silencing of vanilloid receptor TRPV1 by RNAi reduces neuropathic and visceral pain in vivo. Biochem. Biophys. Res. Commun. 350, 238–243. doi: 10.1016/j.bbrc.2006.09.037
Clapham, D. E. (2003). TRP channels as cellular sensors. Nature 426, 517–524. doi: 10.1038/nature02196
Corey, D. P., Garcia-Anoveros, J., Holt, J. R., Kwan, K. Y., Lin, S. Y., Vollrath, M. A., et al. (2004). TRPA1 is a candidate for the mechanosensitive transduction channel of vertebrate hair cells. Nature 432, 723–730. doi: 10.1038/nature03066
Cruz-Orengo, L., Dhaka, A., Heuermann, R. J., Young, T. J., Montana, M. C., Cavanaugh, E. J., et al. (2008). Cutaneous nociception evoked by 15-delta PGJ2 via activation of ion channel TRPA1. Mol. Pain 4:30. doi: 10.1186/1744-8069-4-30
Da Costa, D. S., Meotti, F. C., Andrade, E. L., Leal, P. C., Motta, E. M., and Calixto, J. B. (2010). The involvement of the transient receptor potential A1 (TRPA1) in the maintenance of mechanical and cold hyperalgesia in persistent inflammation. Pain 148, 431–437. doi: 10.1016/j.pain.2009.12.002
Davis, J. B., Gray, J., Gunthorpe, M. J., Hatcher, J. P., Davey, P. T., Overend, P., et al. (2000). Vanilloid receptor-1 is essential for inflammatory thermal hyperalgesia. Nature 405, 183–187. doi: 10.1038/35012076
Dhaka, A., Viswanath, V., and Patapoutian, A. (2006). Trp ion channels and temperature sensation. Annu. Rev. Neurosci. 29, 135–161. doi: 10.1146/annurev.neuro.29.051605.112958
Di Marzo, V., Blumberg, P. M., and Szallasi, A. (2002). Endovanilloid signaling in pain. Curr. Opin. Neurobiol. 12, 372–379. doi: 10.1016/S0959-4388(02)00340-9
Dionisio, N., Albarran, L., Berna-Erro, A., Hernandez-Cruz, J. M., Salido, G. M., and Rosado, J. A. (2011). Functional role of the calmodulin- and inositol 1,4,5-trisphosphate receptor-binding (CIRB) site of TRPC6 in human platelet activation. Cell. Signal. 23, 1850–1856. doi: 10.1016/j.cellsig.2011.06.022
Dionisio, N., Smani, T., Woodard, G. E., Castellano, A., Salido, G. M., and Rosado, J. A. (2015). Homer proteins mediate the interaction between STIM1 and Cav1.2 channels. Biochim. Biophys. Acta 1853, 1145–1153. doi: 10.1016/j.bbamcr.2015.02.014
Eid, S. R., Crown, E. D., Moore, E. L., Liang, H. A., Choong, K. C., Dima, S., et al. (2008). HC-030031, a TRPA1 selective antagonist, attenuates inflammatory- and neuropathy-induced mechanical hypersensitivity. Mol. Pain 4:48. doi: 10.1186/1744-8069-4-48
Eilers, H., Lee, S. Y., Hau, C. W., Logvinova, A., and Schumacher, M. A. (2007). The rat vanilloid receptor splice variant VR.5'sv blocks TRPV1 activation. Neuroreport 18, 969–973. doi: 10.1097/WNR.0b013e328165d1a2
Fang, D., Kong, L. Y., Cai, J., Li, S., Liu, X. D., Han, J. S., et al. (2015). Interleukin-6-mediated functional upregulation of TRPV1 receptors in dorsal root ganglion neurons through the activation of JAK/PI3K signaling pathway: roles in the development of bone cancer pain in a rat model. Pain 156, 1124–1144. doi: 10.1097/j.pain.0000000000000158
Feng, Q. (2014). Temperature sensing by thermal TRP channels: thermodynamic basis and molecular insights. Curr. Top. Membr. 74, 19–50. doi: 10.1016/B978-0-12-800181-3.00002-6
Fleig, A., and Penner, R. (2004). The TRPM ion channel subfamily: molecular, biophysical and functional features. Trends Pharmacol. Sci. 25, 633–639. doi: 10.1016/j.tips.2004.10.004
Fusco, B. M., Barzoi, G., and Agro, F. (2003). Repeated intranasal capsaicin applications to treat chronic migraine. Br. J. Anaesth. 90:812. doi: 10.1093/bja/aeg572
Galione, A. (2006). NAADP, a new intracellular messenger that mobilizes Ca2+ from acidic stores. Biochem. Soc. Trans. 34, 922–926. doi: 10.1042/BST0340922
Gallego-Sandin, S., Rodriguez-Garcia, A., Alonso, M. T., and Garcia-Sancho, J. (2009). The endoplasmic reticulum of dorsal root ganglion neurons contains functional TRPV1 channels. J. Biol. Chem. 284, 32591–32601. doi: 10.1074/jbc.M109.019687
Gao, W., Zan, Y., Wang, Z. J., Hu, X. Y., and Huang, F. (2016). Quercetin ameliorates paclitaxel-induced neuropathic pain by stabilizing mast cells, and subsequently blocking PKCepsilon-dependent activation of TRPV1. Acta Pharmacol. Sin. 37, 1166–1177. doi: 10.1038/aps.2016.58
Gees, M., Colsoul, B., and Nilius, B. (2010). The role of transient receptor potential cation channels in Ca2+ signaling. Cold Spring Harb. Perspect. Biol. 2:a003962. doi: 10.1101/cshperspect.a003962
Ghilardi, J. R., Rohrich, H., Lindsay, T. H., Sevcik, M. A., Schwei, M. J., Kubota, K., et al. (2005). Selective blockade of the capsaicin receptor TRPV1 attenuates bone cancer pain. J. Neurosci. 25, 3126–3131. doi: 10.1523/JNEUROSCI.3815-04.2005
Han, Y., Li, Y., Xiao, X., Liu, J., Meng, X. L., Liu, F. Y., et al. (2012). Formaldehyde up-regulates TRPV1 through MAPK and PI3K signaling pathways in a rat model of bone cancer pain. Neurosci. Bull. 28, 165–172. doi: 10.1007/s12264-012-1211-0
Harteneck, C., Klose, C., and Krautwurst, D. (2011). Synthetic modulators of TRP channel activity. Adv. Exp. Med. Biol. 704, 87–106. doi: 10.1007/978-94-007-0265-3_4
Hermann, H., De Petrocellis, L., Bisogno, T., Schiano Moriello, A., Lutz, B., and Di Marzo, V. (2003). Dual effect of cannabinoid CB1 receptor stimulation on a vanilloid VR1 receptor-mediated response. Cell. Mol. Life Sci. 60, 607–616. doi: 10.1007/s000180300052
Hinman, A., Chuang, H. H., Bautista, D. M., and Julius, D. (2006). TRP channel activation by reversible covalent modification. Proc. Natl. Acad. Sci. U.S.A. 103, 19564–19568. doi: 10.1073/pnas.0609598103
Huang, K., Bian, D., Jiang, B., Zhai, Q., Gao, N., and Wang, R. (2017). TRPA1 contributed to the neuropathic pain induced by docetaxel treatment. Cell Biochem. Funct. 35, 141–143. doi: 10.1002/cbf.3258
Jaquemar, D., Schenker, T., and Trueb, B. (1999). An ankyrin-like protein with transmembrane domains is specifically lost after oncogenic transformation of human fibroblasts. J. Biol. Chem. 274, 7325–7333. doi: 10.1074/jbc.274.11.7325
Jardin, I., Lopez, J. J., Berna-Erro, A., Salido, G. M., and Rosado, J. A. (2013). Homer proteins in Ca2+ entry. IUBMB Life 65, 497–504. doi: 10.1002/iub.1162
Jonassohn, M., Anke, H., Morales, P., and Sterner, O. (1995). Structure-activity relationships for unsaturated dialdehydes. 10. The generation of bioactive products by autoxidation of isovelleral and merulidial. Acta Chem. Scand. 49, 530–535. doi: 10.3891/acta.chem.scand.49-0530
Jordt, S. E., Bautista, D. M., Chuang, H. H., McKemy, D. D., Zygmunt, P. M., Hogestatt, E. D., et al. (2004). Mustard oils and cannabinoids excite sensory nerve fibres through the TRP channel ANKTM1. Nature 427, 260–265. doi: 10.1038/nature02282
Jung, S. Y., Choi, S., Ko, Y. S., Park, C. S., Oh, S., Koh, S. R., et al. (2001). Effects of ginsenosides on vanilloid receptor (VR1) channels expressed in Xenopus oocytes. Mol. Cells 12, 342–346.
Kang, K. (2016). Exceptionally high thermal sensitivity of rattlesnake TRPA1 correlates with peak current amplitude. Biochim. Biophys. Acta 1858, 318–325. doi: 10.1016/j.bbamem.2015.12.011
Karashima, Y., Damann, N., Prenen, J., Talavera, K., Segal, A., Voets, T., et al. (2007). Bimodal action of menthol on the transient receptor potential channel TRPA1. J. Neurosci. 27, 9874–9884. doi: 10.1523/JNEUROSCI.2221-07.2007
Kim, S., and Hwang, S. W. (2013). Emerging roles of TRPA1 in sensation of oxidative stress and its implications in defense and danger. Arch. Pharm. Res. 36, 783–791. doi: 10.1007/s12272-013-0098-2
Kitaguchi, T., and Swartz, K. J. (2005). An inhibitor of TRPV1 channels isolated from funnel Web spider venom. Biochemistry 44, 15544–15549. doi: 10.1021/bi051494l
Kojima, R., Nozawa, K., Doihara, H., Keto, Y., Kaku, H., Yokoyama, T., et al. (2014). Effects of novel TRPA1 receptor agonist ASP7663 in models of drug-induced constipation and visceral pain. Eur. J. Pharmacol. 723, 288–293. doi: 10.1016/j.ejphar.2013.11.020
Lashinger, E. S., Steiginga, M. S., Hieble, J. P., Leon, L. A., Gardner, S. D., Nagilla, R., et al. (2008). AMTB, a TRPM8 channel blocker: evidence in rats for activity in overactive bladder and painful bladder syndrome. Am. J. Physiol. Renal Physiol. 295, F803–F810. doi: 10.1152/ajprenal.90269.2008
Latorre, R., Zaelzer, C., and Brauchi, S. (2009). Structure-functional intimacies of transient receptor potential channels. Q. Rev. Biophys. 42, 201–246. doi: 10.1017/S0033583509990072
Lee, K. P., Choi, S., Hong, J. H., Ahuja, M., Graham, S., Ma, R., et al. (2014). Molecular determinants mediating gating of Transient Receptor Potential Canonical (TRPC) channels by stromal interaction molecule 1 (STIM1). J. Biol. Chem. 289, 6372–6382. doi: 10.1074/jbc.M113.546556
Lepage, P. K., and Boulay, G. (2007). Molecular determinants of TRP channel assembly. Biochem. Soc. Trans. 35, 81–83. doi: 10.1042/BST0350081
Li, M., Yu, Y., and Yang, J. (2011). Structural biology of TRP channels. Adv. Exp. Med. Biol. 704, 1–23. doi: 10.1007/978-94-007-0265-3_1
Li, Y., Cai, J., Han, Y., Xiao, X., Meng, X. L., Su, L., et al. (2014). Enhanced function of TRPV1 via up-regulation by insulin-like growth factor-1 in a rat model of bone cancer pain. Eur. J. Pain 18, 774–784. doi: 10.1002/j.1532-2149.2013.00420.x
Liao, H. Y., Hsieh, C. L., Huang, C. P., and Lin, Y. W. (2017). Electroacupuncture attenuates CFA-induced inflammatory pain by suppressing Nav1.8 through S100B, TRPV1, opioid, and adenosine pathways in Mice. Sci. Rep. 7:42531. doi: 10.1038/srep42531
Lipskaia, L., Keuylian, Z., Blirando, K., Mougenot, N., Jacquet, A., Rouxel, C., et al. (2014). Expression of sarco (endo) plasmic reticulum calcium ATPase (SERCA) system in normal mouse cardiovascular tissues, heart failure and atherosclerosis. Biochim. Biophys. Acta 1843, 2705–2718. doi: 10.1016/j.bbamcr.2014.08.002
Liu, B., Escalera, J., Balakrishna, S., Fan, L., Caceres, A. I., Robinson, E., et al. (2013). TRPA1 controls inflammation and pruritogen responses in allergic contact dermatitis. FASEB J. 27, 3549–3563. doi: 10.1096/fj.13-229948
Lopez, E., Berna-Erro, A., Salido, G. M., Rosado, J. A., and Redondo, P. C. (2013). FKBP52 is involved in the regulation of SOCE channels in the human platelets and MEG 01 cells. Biochim. Biophys. Acta 1833, 652–662. doi: 10.1016/j.bbamcr.2012.11.029
Lopez, J. J., Camello-Almaraz, C., Pariente, J. A., Salido, G. M., and Rosado, J. A. (2005). Ca2+ accumulation into acidic organelles mediated by Ca2+- and vacuolar H+-ATPases in human platelets. Biochem. J. 390, 243–252. doi: 10.1042/BJ20050168
Macpherson, L. J., Dubin, A. E., Evans, M. J., Marr, F., Schultz, P. G., Cravatt, B. F., et al. (2007). Noxious compounds activate TRPA1 ion channels through covalent modification of cysteines. Nature 445, 541–545. doi: 10.1038/nature05544
Malek, N., Pajak, A., Kolosowska, N., Kucharczyk, M., and Starowicz, K. (2015). The importance of TRPV1-sensitisation factors for the development of neuropathic pain. Mol. Cell. Neurosci. 65, 1–10. doi: 10.1016/j.mcn.2015.02.001
McGaraughty, S., Chu, K. L., Perner, R. J., Didomenico, S., Kort, M. E., and Kym, P. R. (2010). TRPA1 modulation of spontaneous and mechanically evoked firing of spinal neurons in uninjured, osteoarthritic, and inflamed rats. Mol. Pain 6:14. doi: 10.1186/1744-8069-6-14
Mickle, A. D., Shepherd, A. J., and Mohapatra, D. P. (2016). Nociceptive TRP channels: sensory detectors and transducers in multiple pain pathologies. Pharmaceuticals 9:E72. doi: 10.3390/ph9040072
Minke, B. (1977). Drosophila mutant with a transducer defect. Biophys. Struct. Mech. 3, 59–64. doi: 10.1007/BF00536455
Montell, C., Birnbaumer, L., and Flockerzi, V. (2002). The TRP channels, a remarkably functional family. Cell 108, 595–598. doi: 10.1016/S0092-8674(02)00670-0
Montero, M., Alonso, M. T., Albillos, A., Cuchillo-Ibanez, I., Olivares, R., García, A. G., et al. (2001). Control of secretion by mitochondria depends on the size of the local [Ca2+] after chromaffin cell stimulation. Eur. J. Neurosci. 13, 2247–2254. doi: 10.1046/j.0953-816x.2001.01602.x
Nicoletti, P., Trevisani, M., Manconi, M., Gatti, R., De Siena, G., Zagli, G., et al. (2008). Ethanol causes neurogenic vasodilation by TRPV1 activation and CGRP release in the trigeminovascular system of the guinea pig. Cephalalgia 28, 9–17. doi: 10.1111/j.1468-2982.2007.01448.x
Nilius, B., Mahieu, F., Prenen, J., Janssens, A., Owsianik, G., Vennekens, R., et al. (2006). The Ca2+-activated cation channel TRPM4 is regulated by phosphatidylinositol 4,5-biphosphate. EMBO J. 25, 467–478. doi: 10.1038/sj.emboj.7600963
Nilius, B., Prenen, J., and Owsianik, G. (2011). Irritating channels: the case of TRPA1. J. Physiol. 589, 1543–1549. doi: 10.1113/jphysiol.2010.200717
Nilius, B., Prenen, J., Droogmans, G., Voets, T., Vennekens, R., Freichel, M., et al. (2003). Voltage dependence of the Ca2+-activated cation channel TRPM4. J. Biol. Chem. 278, 30813–30820. doi: 10.1074/jbc.M305127200
Nilius, B., Talavera, K., Owsianik, G., Prenen, J., Droogmans, G., and Voets, T. (2005). Gating of TRP channels: a voltage connection? J. Physiol. 567, 35–44. doi: 10.1113/jphysiol.2005.088377
Nozawa, K., Kawabata-Shoda, E., Doihara, H., Kojima, R., Okada, H., Mochizuki, S., et al. (2009). TRPA1 regulates gastrointestinal motility through serotonin release from enterochromaffin cells. Proc. Natl. Acad. Sci. U.S.A. 106, 3408–3413. doi: 10.1073/pnas.0805323106
Ogawa, N., Kurokawa, T., and Mori, Y. (2016). Sensing of redox status by TRP channels. Cell Calcium 60, 115–122. doi: 10.1016/j.ceca.2016.02.009
Osterweis, M., Kleinman, A., and Mechanic, D. (1987). Pain and Disability: Clinical, Behavioral, and Public Policy Perspectives. Washington, DC: National Academies Press.
Pan, H. L., Zhang, Y. Q., and Zhao, Z. Q. (2010). Involvement of lysophosphatidic acid in bone cancer pain by potentiation of TRPV1 via PKCepsilon pathway in dorsal root ganglion neurons. Mol. Pain 6:85. doi: 10.1186/1744-8069-6-85
Park, J. H., Chae, J., Roh, K., Kil, E. J., Lee, M., Auh, C. K., et al. (2015). Oxaliplatin-induced peripheral neuropathy via trpa1 stimulation in mice dorsal root ganglion is correlated with aluminum accumulation. PLoS ONE 10:e0124875. doi: 10.1371/journal.pone.0124875
Patapoutian, A., Peier, A. M., Story, G. M., and Viswanath, V. (2003). ThermoTRP channels and beyond: mechanisms of temperature sensation. Nat. Rev. Neurosci. 4, 529–539. doi: 10.1038/nrn1141
Patapoutian, A., Tate, S., and Woolf, C. J. (2009). Transient receptor potential channels: targeting pain at the source. Nat. Rev. Drug Discov. 8, 55–68. doi: 10.1038/nrd2757
Paulsen, C. E., Armache, J. P., Gao, Y., Cheng, Y., and Julius, D. (2015). Structure of the TRPA1 ion channel suggests regulatory mechanisms. Nature 520, 511–517. doi: 10.1038/nature14367
Pecze, L., Szabo, K., Szell, M., Josvay, K., Kaszas, K., Kusz, E., et al. (2008). Human keratinocytes are vanilloid resistant. PLoS ONE 3:e3419. doi: 10.1371/journal.pone.0003419
Planells-Cases, R., Valente, P., Ferrer-Montiel, A., Qin, F., and Szallasi, A. (2011). Complex regulation of TRPV1 and related thermo-TRPs: implications for therapeutic intervention. Adv. Exp. Med. Biol. 704, 491–515. doi: 10.1007/978-94-007-0265-3_27
Poletini, M. O., Moraes, M. N., Ramos, B. C., Jeronimo, R., and Castrucci, A. M. (2015). TRP channels: a missing bond in the entrainment mechanism of peripheral clocks throughout evolution. Temperature 2, 522–534. doi: 10.1080/23328940.2015.1115803
Putney, J. W. Jr. (1986). A model for receptor-regulated calcium entry. Cell Calcium 7, 1–12. doi: 10.1016/0143-4160(86)90026-6
Rami, H. K., Thompson, M., Stemp, G., Fell, S., Jerman, J. C., Stevens, A. J., et al. (2006). Discovery of SB-705498: a potent, selective and orally bioavailable TRPV1 antagonist suitable for clinical development. Bioorg. Med. Chem. Lett. 16, 3287–3291. doi: 10.1016/j.bmcl.2006.03.030
Redondo, P. C., Rosado, J. A., Pariente, J. A., and Salido, G. M. (2005). Collaborative effect of SERCA and PMCA in cytosolic calcium homeostasis in human platelets. J. Physiol. Biochem. 61, 507–516. doi: 10.1007/BF03168376
Riera, C. E., Menozzi-Smarrito, C., Affolter, M., Michlig, S., Munari, C., Robert, F., et al. (2009). Compounds from Sichuan and Melegueta peppers activate, covalently and non-covalently, TRPA1 and TRPV1 channels. Br. J. Pharmacol. 157, 1398–1409. doi: 10.1111/j.1476-5381.2009.00307.x
Ruparel, N. B., Patwardhan, A. M., Akopian, A. N., and Hargreaves, K. M. (2008). Homologous and heterologous desensitization of capsaicin and mustard oil responses utilize different cellular pathways in nociceptors. Pain 135, 271–279. doi: 10.1016/j.pain.2007.06.005
Salat, K., and Filipek, B. (2015). Antinociceptive activity of transient receptor potential channel TRPV1, TRPA1, and TRPM8 antagonists in neurogenic and neuropathic pain models in mice. J. Zhejiang Univ. Sci. B 16, 167–178. doi: 10.1631/jzus.B1400189
Salido, G. M., Sage, S. O., and Rosado, J. A. (2009a). Biochemical and functional properties of the store-operated Ca2+ channels. Cell. Signal. 21, 457–461. doi: 10.1016/j.cellsig.2008.11.005
Salido, G. M., Sage, S. O., and Rosado, J. A. (2009b). TRPC channels and store-operated Ca2+ entry. Biochim. Biophys. Acta 1793, 223–230. doi: 10.1016/j.bbamcr.2008.11.001
Sardar, P., Kumar, A., Bhandari, A., and Goswami, C. (2012). Conservation of tubulin-binding sequences in TRPV1 throughout evolution. PLoS ONE 7:e31448. doi: 10.1371/journal.pone.0031448
Schaible, H. G., Ebersberger, A., and Natura, G. (2011). Update on peripheral mechanisms of pain: beyond prostaglandins and cytokines. Arthritis Res. Ther. 13:210. doi: 10.1186/ar3305
Schindl, R., and Romanin, C. (2007). Assembly domains in TRP channels. Biochem. Soc. Trans. 35, 84–85. doi: 10.1042/BST0350084
Schlingmann, K. P., and Gudermann, T. (2005). A critical role of TRPM channel-kinase for human magnesium transport. J. Physiol. 566, 301–308. doi: 10.1113/jphysiol.2004.080200
Schumacher, M. A., and Eilers, H. (2010). TRPV1 splice variants: structure and function. Front. Biosci. 15, 872–882. doi: 10.2741/3651
Schumacher, M. A., Moff, I., Sudanagunta, S. P., and Levine, J. D. (2000). Molecular cloning of an N-terminal splice variant of the capsaicin receptor. Loss of N-terminal domain suggests functional divergence among capsaicin receptor subtypes. J. Biol. Chem. 275, 2756–2762. doi: 10.1074/jbc.275.4.2756
Sculptoreanu, A., Kullmann, F. A., Artim, D. E., Bazley, F. A., Schopfer, F., Woodcock, S., et al. (2010). Nitro-oleic acid inhibits firing and activates TRPV1- and TRPA1-mediated inward currents in dorsal root ganglion neurons from adult male rats. J. Pharmacol. Exp. Ther. 333, 883–895. doi: 10.1124/jpet.109.163154
Siemens, J., Zhou, S., Piskorowski, R., Nikai, T., Lumpkin, E. A., Basbaum, A. I., et al. (2006). Spider toxins activate the capsaicin receptor to produce inflammatory pain. Nature 444, 208–212. doi: 10.1038/nature05285
Sinkins, W. G., Goel, M., Estacion, M., and Schilling, W. P. (2004). Association of immunophilins with mammalian TRPC channels. J. Biol. Chem. 279, 34521–34529. doi: 10.1074/jbc.M401156200
Sisignano, M., Park, C. K., Angioni, C., Zhang, D. D., Von Hehn, C., Cobos, E. J., et al. (2012). 5,6-EET is released upon neuronal activity and induces mechanical pain hypersensitivity via TRPA1 on central afferent terminals. J. Neurosci. 32, 6364–6372. doi: 10.1523/JNEUROSCI.5793-11.2012
Sotomayor, M., Corey, D. P., and Schulten, K. (2005). In search of the hair-cell gating spring elastic properties of ankyrin and cadherin repeats. Structure 13, 669–682. doi: 10.1016/j.str.2005.03.001
Staruschenko, A., Jeske, N. A., and Akopian, A. N. (2010). Contribution of TRPV1-TRPA1 interaction to the single channel properties of the TRPA1 channel. J. Biol. Chem. 285, 15167–15177. doi: 10.1074/jbc.M110.106153
Story, G. M., Peier, A. M., Reeve, A. J., Eid, S. R., Mosbacher, J., Hricik, T. R., et al. (2003). ANKTM1, a TRP-like channel expressed in nociceptive neurons, is activated by cold temperatures. Cell 112, 819–829. doi: 10.1016/S0092-8674(03)00158-2
Szallasi, A., and Blumberg, P. M. (1989). Resiniferatoxin, a phorbol-related diterpene, acts as an ultrapotent analog of capsaicin, the irritant constituent in red pepper. Neuroscience 30, 515–520. doi: 10.1016/0306-4522(89)90269-8
Tang, J., Lin, Y., Zhang, Z., Tikunova, S., Birnbaumer, L., and Zhu, M. X. (2001). Identification of common binding sites for calmodulin and inositol 1,4,5-trisphosphate receptors on the carboxyl termini of trp channels. J. Biol. Chem. 276, 21303–21310. doi: 10.1074/jbc.M102316200
Tekus, V., Bolcskei, K., Kis-Varga, A., Dezsi, L., Szentirmay, E., Visegrady, A., et al. (2010). Effect of transient receptor potential vanilloid 1 (TRPV1) receptor antagonist compounds SB705498, BCTC and AMG9810 in rat models of thermal hyperalgesia measured with an increasing-temperature water bath. Eur. J. Pharmacol. 641, 135–141. doi: 10.1016/j.ejphar.2010.05.052
Tian, W., Fu, Y., Wang, D. H., and Cohen, D. M. (2006). Regulation of TRPV1 by a novel renally expressed rat TRPV1 splice variant. Am. J. Physiol. Renal Physiol. 290, F117–F126. doi: 10.1152/ajprenal.00143.2005
Tominaga, M., Caterina, M. J., Malmberg, A. B., Rosen, T. A., Gilbert, H., Skinner, K., et al. (1998). The cloned capsaicin receptor integrates multiple pain-producing stimuli. Neuron 21, 531–543. doi: 10.1016/S0896-6273(00)80564-4
Trevisan, G., Benemei, S., Materazzi, S., De Logu, F., De Siena, G., Fusi, C., et al. (2016). TRPA1 mediates trigeminal neuropathic pain in mice downstream of monocytes/macrophages and oxidative stress. Brain 139, 1361–1377. doi: 10.1093/brain/aww038
Trevisan, G., Hoffmeister, C., Rossato, M. F., Oliveira, S. M., Silva, M. A., Silva, C. R., et al. (2014). TRPA1 receptor stimulation by hydrogen peroxide is critical to trigger hyperalgesia and inflammation in a model of acute gout. Free Radic. Biol. Med. 72, 200–209. doi: 10.1016/j.freeradbiomed.2014.04.021
Trevisani, M., Siemens, J., Materazzi, S., Bautista, D. M., Nassini, R., Campi, B., et al. (2007). 4-Hydroxynonenal, an endogenous aldehyde, causes pain and neurogenic inflammation through activation of the irritant receptor TRPA1. Proc. Natl. Acad. Sci. U.S.A. 104, 13519–13524. doi: 10.1073/pnas.0705923104
Tsiokas, L. (2009). Function and regulation of TRPP2 at the plasma membrane. Am. J. Physiol. Renal Physiol. 297, F1–F9. doi: 10.1152/ajprenal.90277.2008
Tsubota-Matsunami, M., Noguchi, Y., Okawa, Y., Sekiguchi, F., and Kawabata, A. (2012). Colonic hydrogen sulfide-induced visceral pain and referred hyperalgesia involve activation of both Ca(v)3.2 and TRPA1 channels in mice. J. Pharmacol. Sci. 119, 293–296. doi: 10.1254/jphs.12086SC
Varga, A., Nemeth, J., Szabo, A., McDougall, J. J., Zhang, C., Elekes, K., et al. (2005). Effects of the novel TRPV1 receptor antagonist SB366791 in vitro and in vivo in the rat. Neurosci. Lett. 385, 137–142. doi: 10.1016/j.neulet.2005.05.015
Vetter, I., and Lewis, R. J. (2011). Natural product ligands of TRP channels. Adv. Exp. Med. Biol. 704, 41–85. doi: 10.1007/978-94-007-0265-3_3
Viguier, F., Michot, B., Hamon, M., and Bourgoin, S. (2013). Multiple roles of serotonin in pain control mechanisms–implications of 5-HT(7) and other 5-HT receptor types. Eur. J. Pharmacol. 716, 8–16. doi: 10.1016/j.ejphar.2013.01.074
Voets, T., Talavera, K., Owsianik, G., and Nilius, B. (2005). Sensing with TRP channels. Nat. Chem. Biol. 1, 85–92. doi: 10.1038/nchembio0705-85
Vriens, J., Nilius, B., and Voets, T. (2014). Peripheral thermosensation in mammals. Nat. Rev. Neurosci. 15, 573–589. doi: 10.1038/nrn3784
Vriens, J., Owsianik, G., Hofmann, T., Philipp, S. E., Stab, J., Chen, X., et al. (2011). TRPM3 is a nociceptor channel involved in the detection of noxious heat. Neuron 70, 482–494. doi: 10.1016/j.neuron.2011.02.051
Walker, K. M., Urban, L., Medhurst, S. J., Patel, S., Panesar, M., Fox, A. J., et al. (2003). The VR1 antagonist capsazepine reverses mechanical hyperalgesia in models of inflammatory and neuropathic pain. J. Pharmacol. Exp. Ther. 304, 56–62. doi: 10.1124/jpet.102.042010
Wang, C., Hu, H. Z., Colton, C. K., Wood, J. D., and Zhu, M. X. (2004). An alternative splicing product of the murine trpv1 gene dominant negatively modulates the activity of TRPV1 channels. J. Biol. Chem. 279, 37423–37430. doi: 10.1074/jbc.M407205200
Wei, H., Hamalainen, M. M., Saarnilehto, M., Koivisto, A., and Pertovaara, A. (2009). Attenuation of mechanical hypersensitivity by an antagonist of the TRPA1 ion channel in diabetic animals. Anesthesiology 111, 147–154. doi: 10.1097/ALN.0b013e3181a1642b
Wei, H., Sagalajev, B., Yuzer, M. A., Koivisto, A., and Pertovaara, A. (2015). Regulation of neuropathic pain behavior by amygdaloid TRPC4/C5 channels. Neurosci. Lett. 608, 12–17. doi: 10.1016/j.neulet.2015.09.033
Wes, P. D., Chevesich, J., Jeromin, A., Rosenberg, C., Stetten, G., and Montell, C. (1995). TRPC1, a human homolog of a Drosophila store-operated channel. Proc. Natl. Acad. Sci. U.S.A. 92, 9652–9656. doi: 10.1073/pnas.92.21.9652
Westlund, K. N., Zhang, L. P., Ma, F., Nesemeier, R., Ruiz, J. C., Ostertag, E. M., et al. (2014). A rat knockout model implicates TRPC4 in visceral pain sensation. Neuroscience 262, 165–175. doi: 10.1016/j.neuroscience.2013.12.043
Wetsel, W. C. (2011). Sensing hot and cold with TRP channels. Int. J. Hyperthermia 27, 388–398. doi: 10.3109/02656736.2011.554337
Woodard, G. E., Sage, S. O., and Rosado, J. A. (2007). Transient receptor potential channels and intracellular signaling. Int. Rev. Cytol. 256, 35–67. doi: 10.1016/S0074-7696(07)56002-X
Xia, R., Dekermendjian, K., Lullau, E., and Dekker, N. (2011). TRPV1: a therapy target that attracts the pharmaceutical interests. Adv. Exp. Med. Biol. 704, 637–665. doi: 10.1007/978-94-007-0265-3_34
Yamaguchi, K., Ono, K., Hitomi, S., Ito, M., Nodai, T., Goto, T., et al. (2016). Distinct TRPV1- and TRPA1-based mechanisms underlying enhancement of oral ulcerative mucositis-induced pain by 5-fluorouracil. Pain 157, 1004–1020. doi: 10.1097/j.pain.0000000000000498
Yuan, J. P., Kiselyov, K., Shin, D. M., Chen, J., Shcheynikov, N., Kang, S. H., et al. (2003). Homer binds TRPC family channels and is required for gating of TRPC1 by IP3 receptors. Cell 114, 777–789. doi: 10.1016/S0092-8674(03)00716-5
Zbidi, H., Jardin, I., Woodard, G. E., Lopez, J. J., Berna-Erro, A., Salido, G. M., et al. (2011). STIM1 and STIM2 are located in the acidic Ca2+ stores and associates with orai1 upon depletion of the acidic stores in human platelets. J. Biol. Chem. 286, 12257–12270. doi: 10.1074/jbc.M110.190694
Zhou, Q., Yang, L., Larson, S., Basra, S., Merwat, S., Tan, A., et al. (2016). Decreased miR-199 augments visceral pain in patients with IBS through translational upregulation of TRPV1. Gut 65, 797–805. doi: 10.1136/gutjnl-2013-306464
Keywords: calcium entry, TRPs, TRPA1, TRPV1, noxious sensation
Citation: Jardín I, López JJ, Diez R, Sánchez-Collado J, Cantonero C, Albarrán L, Woodard GE, Redondo PC, Salido GM, Smani T and Rosado JA (2017) TRPs in Pain Sensation. Front. Physiol. 8:392. doi: 10.3389/fphys.2017.00392
Received: 25 April 2017; Accepted: 26 May 2017;
Published: 09 June 2017.
Edited by:
Laszlo Pecze, University of Fribourg, SwitzerlandReviewed by:
Carlos Villalobos, Consejo Superior de Investigaciones Científicas (CSIC), SpainCopyright © 2017 Jardín, López, Diez, Sánchez-Collado, Cantonero, Albarrán, Woodard, Redondo, Salido, Smani and Rosado. This is an open-access article distributed under the terms of the Creative Commons Attribution License (CC BY). The use, distribution or reproduction in other forums is permitted, provided the original author(s) or licensor are credited and that the original publication in this journal is cited, in accordance with accepted academic practice. No use, distribution or reproduction is permitted which does not comply with these terms.
*Correspondence: José J. López, ampsb3BlekB1bmV4LmVz
Disclaimer: All claims expressed in this article are solely those of the authors and do not necessarily represent those of their affiliated organizations, or those of the publisher, the editors and the reviewers. Any product that may be evaluated in this article or claim that may be made by its manufacturer is not guaranteed or endorsed by the publisher.
Research integrity at Frontiers
Learn more about the work of our research integrity team to safeguard the quality of each article we publish.