- 1Division of Oral Biology, Leeds School of Dentistry, St. James University Hospital, University of Leeds, Leeds, UK
- 2Department Oral Biology, Faculty of Dentistry, King AbdulAziz University, Jeddah, Saudi Arabia
- 3Department of Oral Pathology, Faculty of Dentistry, Suez Canal University, Ismailia, Egypt
We have isolated dental pulp cells (DPCs) from three healthy (hDPCs) and three carious (cDPCs) donors and shown that compared to hDPCs cells isolated from superficial carious lesions show higher clonogenic potential; show an equivalent proportion of cells with putative stem cell surface markers; show enhanced matrix mineralization capability; have enhanced angiogenic marker expression and retain the inflammatory phenotype in vitro characteristic of superficial caries lesions in vivo. Our findings suggest that cDPCs may be used for further investigation of the cross talk between inflammatory, angiogenic and mineralization pathways in repair of carious pulp. In addition cells derived from carious pulps (almost always discarded) may have potential for future applications in mineralized tissue repair and regeneration.
Introduction
Dental pulp cells (DPCs) contain a subset of stem cells DPSCs (Gronthos et al., 2000; Shi et al., 2001) which under appropriate conditions can differentiate into multiple lineages in vitro (Dominici et al., 2006; Huang et al., 2009; Martens et al., 2012). Although, evidence of differentiated functional tissue derived from transplanted DPSCs is limited some studies show that such cells can interact with biologically compatible scaffolds as part of successful tissue engineering strategies (El-Gendy et al., 2015). As an example of this transplantation of DPSCs into SCID mice was shown to result in formation of dentin pulp-like tissue structures (Gronthos et al., 2002). In situ DPCs are involved in dentin repair following damage by noxious stimuli (Gronthos et al., 2000, 2002; El-Gendy et al., 2015) and ease of access along with routine tooth banking make dental pulp an attractive potential source of multipotent cells for autologous regenerative therapies (Gronthos et al., 2000, 2002; Krebsbach and Robey, 2002; Gafni et al., 2004; Nakashima et al., 2004; Papaccio et al., 2006; Zhang et al., 2006; d'Aquino et al., 2008; Lin et al., 2008). In fact such cells have been used in maxillofacial reconstruction and periodontal ligament regeneration protocols (Huang et al., 2009; Lei et al., 2014; Ledesma-Martínez et al., 2015).
Inflammation in dental pulp induces mineralized tissue regeneration which is important for healing. This process is regulated locally by growth factors and other cytokines (Simon et al., 2011; Smith et al., 2012). In response to appropriate stimuli DPSCs differentiate into odontoblast-like cells and form reparative dentin. This mineralization process is accompanied by neo- vascularization (Freeman et al., 2015) and dental pulp is therefore considered an ideal source of mesenchymal stem cells (MSCs) for regeneration of vascularized hard tissue (El-Gendy et al., 2015). Although, there is a substantial literature on DPCs derived from healthy pulp much less information is available on DPCs derived from caries affected teeth especially with respect to stem cell characterization (Yamada et al., 2010) and whether such cells retain the same regenerative abilities as hDPCs (Gronthos et al., 2000, 2002; Huang et al., 2009; Suchanek et al., 2009; Yalvac et al., 2010; Rodríguez-Lozano et al., 2011; Eslaminejad et al., 2015). This study compares cDPCs isolated from teeth with superficial caries (Yamada et al., 2010) and hDPCs with respect to clonogenic potential, putative stem cell marker expression, mineralization capacity, expression of angiogenic genes (PECAM-1 and VEGFR2), and genes and proteins associated with the inflammatory process (TLR-2/4 and IL-6/8).
Materials and Methods
Cell Isolation and Culture
Freshly extracted healthy and carious third molars were collected from adult patients. Teeth were obtained through Leeds Dental and Skeletal tissue bank (LDI Research Tissue Bank; 130111/AH/75), with informed patient consent. Carious lesions were chosen based on depth of decay in the dentin layer and assessment of this was made during sectioning of teeth both visually and with a WHO periodontal probe. Teeth with >2 mm of sound dentin measured from the edge of the carious lesion to the pulp tissue were included in this study and categorized as shallow caries (McLachlan et al., 2004; Bjørndal, 2008; Kim et al., 2010). DPCs were isolated by enzymatic digestion of pulp tissue as previously described (Alkharobi et al., 2016). hDPCs and cDPCs at passage 4 were seeded in 6-well plates at 105 cells per well under basal conditions (α-MEM 20% FBS, 200 mM L-glutamine, and 100 U/mL Pen Strep). At approximately 80% confluency cells were cultured in triplicate under basal or matrix mineralization conditions (basal medium + 10 nM dexamethasone, 100 μM L-ascorbic acid). Cultures were routinely terminated at 1 and 3 wk. for qRT-PCR analysis cytokine assay and histochemical staining [Alkaline Phosphatase (ALP) and Alizarin red stains]. Experiments were performed with cells derived from three healthy and three carious donors with technical triplicates at each time point and for each culture condition.
Colony Forming Efficiency Assay
Freshly isolated cells from both hDPCs and cDPCs (n = 3 in both instances) were seeded at 106 cells/dish in 10 cm Petri dishes and cultured under basal conditions for 14 days, washed with PBS and fixed with absolute ethanol for 20 min. Cells were stained with 10% (v/v) Trypan Blue for 5 min and washed gently with distilled water. Aggregates of >50 cells were defined as a colony and were counted under the light microscope.
Flow Cytometry
Appropriate antibody dilutions were determined by titration and isotype controls were used in conjunction which each test antibody (see Table 1). Staining was performed essentially according to manufacturer protocols. Briefly 106 cells were incubated with 0.1% Fixable Viability Stain (FVS) in 1 ml PBS at 4°C for 30–60 min in the dark. Cells were washed 2 × 2 ml with staining buffer and Fc receptors were blocked (0.5% blocking solution 10 min RT). Cells were then incubated with CD146/PE-Cy7, CD90/PerCP-Cy5.5, CD105/BV421, CD45/APC-Cy7, and CD31/FITC in 100 μL of staining buffer and incubated at 4°C for 30–60 min. Brilliant stain buffer was added (50 μl) to reduce non-specific interaction between polymer based brilliant violet dyes and to improve the staining quality when multiple dyes are used in the same experiment. Following antibody incubation cells were washed and re-suspended gently in 500 μl staining buffer. Analysis was performed on an LSRII FACS analyser (BD Biosciences) using 405, 488, and 640 nm laser excitation. Data was collected and analyzed using both FACS DivA software (BD Biosciences) and FlowJoV10 (Tree Star). Single color stained CompBeads were used for purpose of compensation. Unstained cells, cells labeled with mouse IgG1 Isotype and fluorescence minus one (FMO) were also used as controls as described elsewhere (Roederer, 2001).
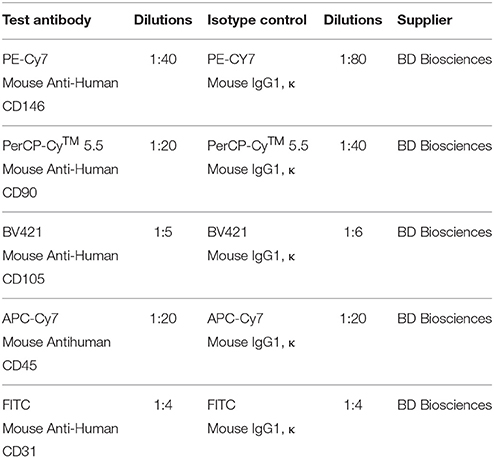
Table 1. Dilutions of test and isotype control antibodies used in FACS analysis of hDPC and cDPC cell surface marker expression.
qRT-PCR
Mineralization (ALP, RUNX-2, OC), angiogenic (VEGFR2 & PECAM1) and inflammatory (TLR-2, TLR-4) marker expression was assessed using TaqMan based qRT-PCR as described previously (Alkharobi et al., 2016). GAPDH was used as housekeeping gene. Basal expression of markers is expressed as 2−ΔCt relative to GAPDH and changes in gene expression under differentiating v basal conditions were determined using the 2−ΔΔCt method also as described previously (Alkharobi et al., 2016) Data are presented as mean ± SD (n = 3) and represent triplicate technical repeats from each of 3 healthy and 3 caries derived DPC cultures.
Cytokine Assay
BD™ CBA Human Inflammatory cytokine kit was used to quantify Interleukin-8 (IL-8), and interleukin-6 (IL-6) protein levels in media conditioned by hDPCs and cDPCs grown under basal and mineralizing conditions for 1 and 3 wk. Assays were performed exactly according to the manufacturer's protocol. Data acquisition and analysis was by flow cytometer LSR II 4 Laser using flow cytometry analysis program (FCAP) array software.
Statistics
Differences between hDPCs and cDPCs were analyzed using 1-way analysis of variance (ANOVA) followed by Tukey's multiple comparisons test (Prism 6.0). Significance was reported at p < 0.05.
Results
Colony Forming Unit (CFU) Efficiency
Colonies were counted in primary basal cultures of hDPCs (n = 3) and cDPCs (n = 3) isolated from third molars. Colonies were identified as clusters of >50 cells. Both hDPCs (60 ±10) and cDPCs (100 ± 7.6) formed CFUs. A significant increase in CFUs was seen in cDPCs compared to hDPCs (p = 0.0053) suggesting an increased colony forming efficiency in cDPCs (Figure 1).
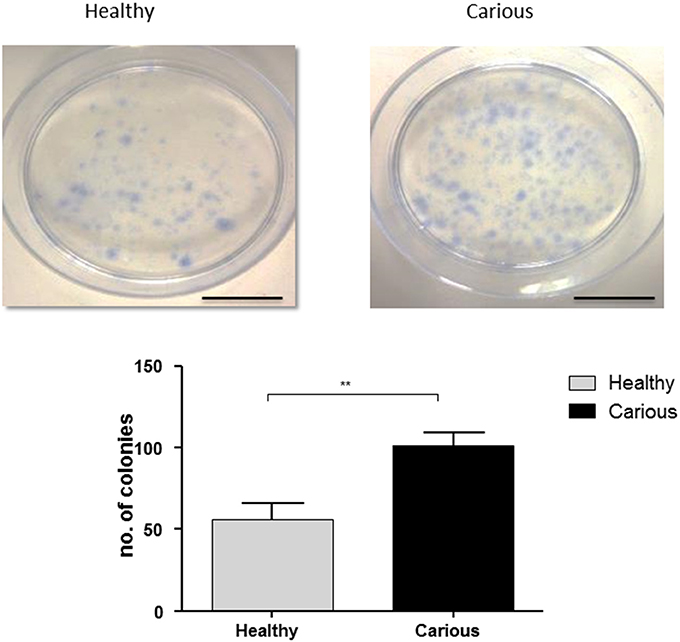
Figure 1. Colony formation in hDPC and cDPC cultures. Cells were cultured for 14 days under basal conditions and stained with Toluidine blue as described in Methods. Scale bar = 5 cm. Cultures from 3 donors for each of hDPC and cDPC were performed and a representative image is shown. Data from all cultures is shown in the lower panel and reported as mean ± SD (n = 3). P < 0.01 (Student's unpaired t-test).
Cell Surface Markers
Flow cytometric analysis was used to characterize surface marker expression of cDPCs vs. hDPCs using a gating strategy to isolate the CD90+/CD105+/CD146+/CD45-/CD31- cells as a putative stem cell population (Figure 2). The data suggested that the cDPCs expressed a higher percentage stem cell population (34 ± 16.6%) compared with hDPCs (18.5 ± 19.3%) although there was a large amount of variation in the data mainly due to variation in the expression of CD146 (Table 2) such that no significant difference was apparent (see also Supplementary Figure 1).
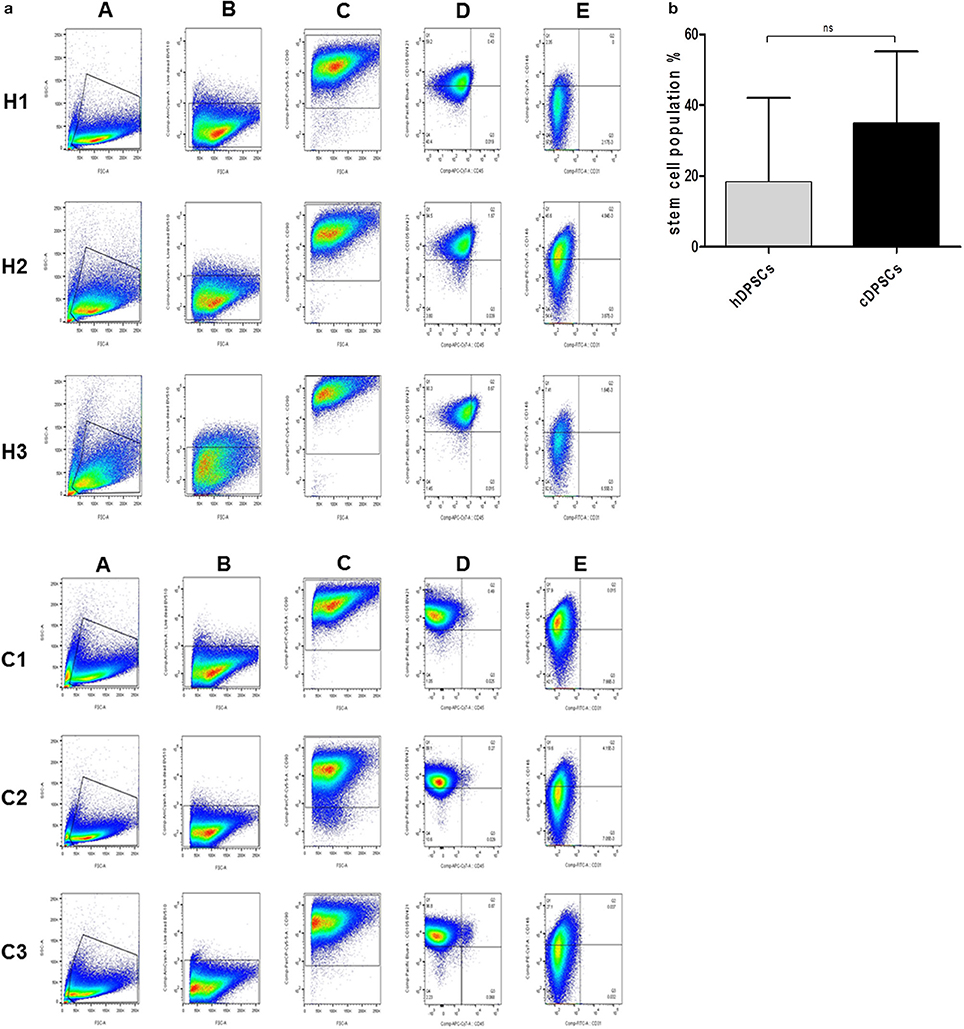
Figure 2. (a) Gating strategy identifies CD90+/CD105+/CD146+/CD31−/CD45− population in DPCs derived from three healthy (H1, H2, and H3) and three carious (C1, C2, and C3) donors. (A) Representative dot plots of intact cellular bodies gating in DPCs. (B) Representative dot plots of living cells gating in DPCs using fixable viability dye. (C) Representative dot plots of CD90+ cells gating in DPCs. (D) Representative of dot plots of CD105 (Y axis) against CD45 (X axis) surface markers from selected CD90+ subpopulation. (E) Representative dot plots of CD146 (Y axis) against CD31 (X axis) surface markers from selected CD105+/CD45− subpopulation in the previous plot. (b) Percentage CD90+/CD105+/CD146+/CD31−/CD45− in hDPCs and cDPCs. Data are presented as mean ± SD (n = 3); p = 0.414.

Table 2. Percentage positive cell staining for cell surface markers in h and cDPCs (see also Supplementary Figure 1).
Histochemical Staining
Positive ALP staining was seen in cultures from all donors in both hDPCs and cDPCs groups under basal and mineralizing conditions at 1 and 3 wk. (Figure 3) However, the results clearly indicated more intense staining under mineralizing conditions at both 1 and 3 wk. There was evidence that cDPCs showed increased ALP staining under mineralizing conditions at 1 and 3 wk. compared with hDPCs. One donor from each group is presented in Figure 3 and similar results were obtained using DPCs from other donors. Matrix mineralization (as determined by Alizarin Red staining) was enhanced under differentiation conditions at both 1 and 3 wk. time points for both hDPCs and cDPCs cultures. As for ALP staining there was evidence for increased Alizarin Red staining in cDPCs compared to hDPCs (Figure 3).
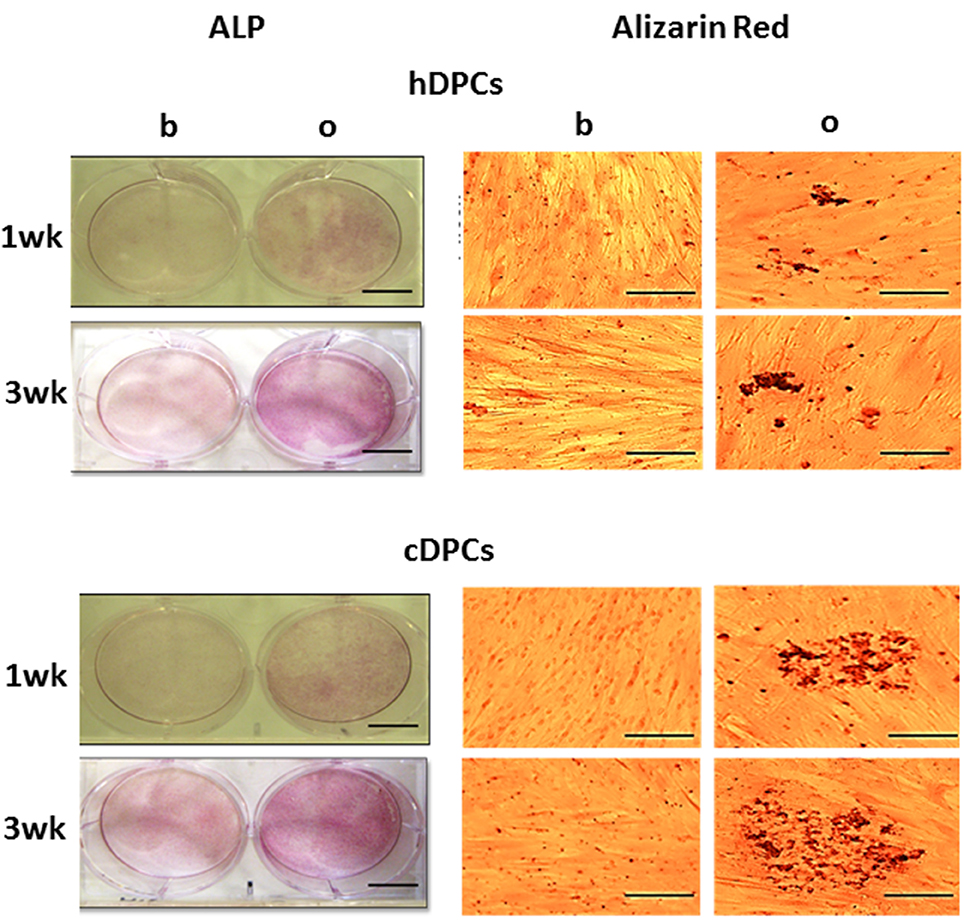
Figure 3. ALP (left panels, scale bar= 5 cm) and Alizarin red (right panels, scale bar = 100 μm) staining of hDPCs (upper panels) and cDPCs (lower panels) cultured under basal (b) or mineralizing (o) conditions for 1 and 3 wk.
Gene Expression: Basal Conditions
The expression of mineralization (ALPL, OC, and RUNX-2), angiogenic (VEGFR-2, PECAM-1) and inflammatory (TLR-2, TLR-4) markers, was investigated in primary cultures of hDPCs and cDPCs grown under basal conditions (Figure 4A). ALP expression was significantly increased in hDPCs and cDPCs at wk.3 compared with wk.1 under basal conditions. OC and RunX2 were expressed at approximately the same level as ALP although there was no time dependent change in expression of these genes. The angiogenic markers VEGFR-2 and PECAM1 were expressed at lower levels than mineralization markers (compare y-axis scales) approximately 1,000-fold for VEGFR2 and 10-fold for PECAM-1. At the 3wk time point there was a trend for higher expression of both VEGFR2 and PECAM-1 in cDPCs and although this reached statistical significance for VEGFR2, data was characterized by high SD values. The inflammatory markers TLR-2 and TLR-4 were expressed at 10-fold lower levels than mineralization markers in both hDPCs and cDPCs. However the most striking feature was that both inflammatory markers were upregulated in cDPCs when compared with hDPCs (approximately 4- to 5-fold) at both 1 and 3wk time points.
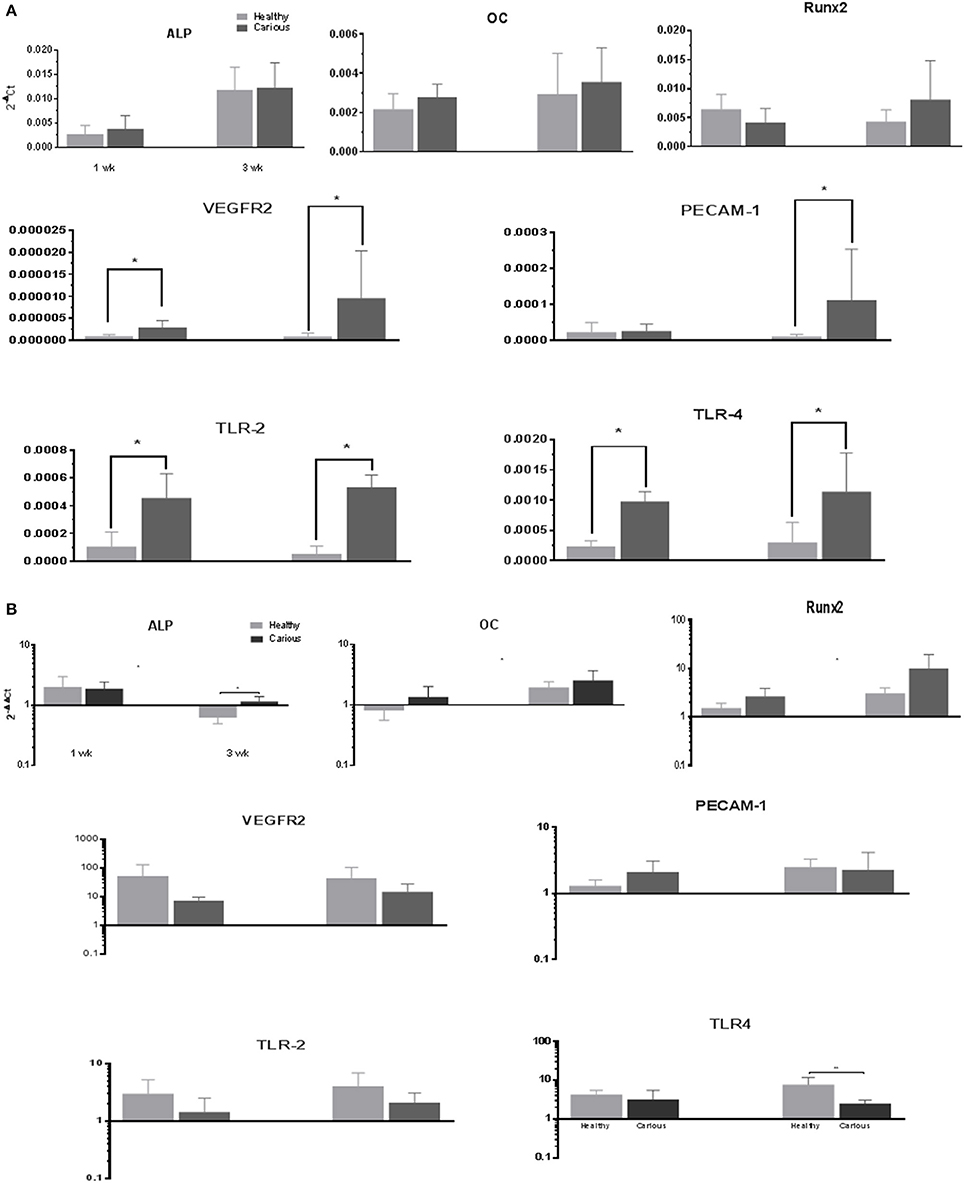
Figure 4. (A) qRT-PCR analysis of basal expression of mineralization, angiogenic and inflammatory markers in hDPCs and cDPCs. Data is presented for both 1 and 3 wk time points expressed relative to GAPDH (2−ΔCt) and represents triplicate technical replicates for each of 3 healthy and 3 carious donors; pooled data are expressed as mean ± SD (n = 3) *p < 0.05. (B) Changes in marker gene expression under mineralizing conditions. Data is presented for both 1 and 3 wk time points expressed as fold change (2−ΔΔCt) mineralizing v basal and represent triplicate technical replicates for each of 3 healthy and 3 carious donors; pooled data are expressed mean ± SD (n = 3) *p < 0.05
Gene Expression: Mineralizing Conditions
Changes in the gene expression in primary cultures of hDPCs and cDPCs grown under mineralizing conditions were investigated (Figure 4B). ALP expression was upregulated in both cell types at wk1 and downregulated in hDPCs at wk3. OC expression was upregulated at wk3 in both hDPCs and cDPCs. RUNX-2 expression was increased at both 1 and 3 wk in both hDPCs and cDPCs although there was a trend for greater upregulation at wk 3 compared to wk 1. The angiogenic markers VEGFR-2 and PECAM-1 were also consistently upregulated under mineralizing conditions in both cell types compared to basal controls. VEGFR-2 expression was lower in cDPCs compared to hDPCs under mineralizing conditions at 1 and 3 wk. No statistical difference in the level of upregulation for hDPC v cDPC was apparent TLR-2 expression was upregulated under mineralizing conditions in both hDPCs and cDPCs although this effect was more apparent at the 3 wk time point. TLR-4 expression was also upregulated under mineralizing conditions in both cell types and at both time points compared to basal controls. At wk 3 cDPCs showed significantly lower levels of TLR-4 compared to hDPCs.
Interleukin Expression
IL-6 and IL-8 proteins were secreted by primary cultures of hDPCs and cDPCs (Figure 5). IL-6 levels were significantly lower in medium conditioned by cells grown in mineralization medium for both hDPCs and cDPCs. In addition, under basal conditions IL-6 levels were significantly higher in carious compared to healthy DPC cultures. In contrast IL-8 levels were higher in medium conditioned by cells grown under mineralizing conditions compared to cells grown under basal conditions although this was only statistically significant at 3 wk. Similarly to IL-6, under basal conditions IL-8 concentrations were higher in cDPCs compared to hDPCs at both 1 and 3 wk time points (Figure 5).
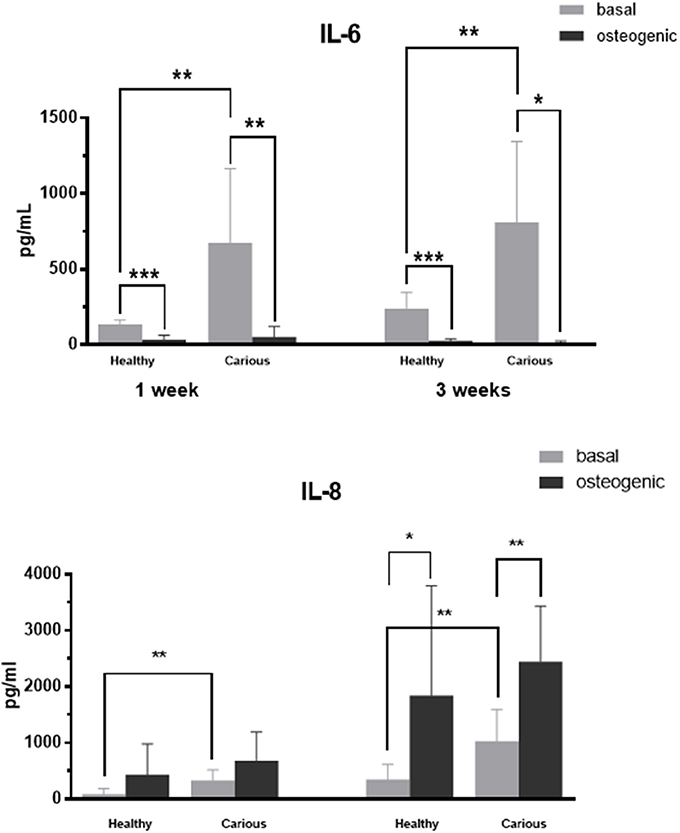
Figure 5. IL-6 (upper panels) and IL-8 (lower panels) concentration in media conditioned by hDPCs and cDPCs cultured under basal and mineralizing conditions for 1 and 3 wk. Data are shown as pg/ml and represent triplicate technical replicates for each of 3 healthy and 3 carious donors; means ± SD (n = 3). *P < 0.05; **P < 0.01; ***P < 0.0001.
Discussion
There is very little data on the characterization of DPCs isolated from teeth with shallow caries (cDPCs). In this report we have characterized several properties of these cells in comparison with cells isolated from healthy dental pulp tissue (hDPCs). We reasoned that some understanding of the inflammation-regeneration processes might help to determine whether the cDPCs can be used for mineralized tissue regeneration, either in situ for dentin/pulp complex formation or as a source of autologous stem cells for bone regeneration. We found that colonies of cDPSCs occurred at higher frequency in comparison to hDPSCs (Figure 1) in agreement with previous observations showing a higher clonogenic potential of cDPCs isolated from third molars with deep caries (Ma et al., 2012). This increased clonogenic potential of cDPCs may reflect the retention of a phenotype associated with early dentinal repair processes in response to pathological stimuli.
We confirmed that both hDPCs and cDPCs demonstrated the classical MSC surface marker profile CD146+/CD105+/CD90+/CD45−/CD31− (Dominici et al., 2006; Huang et al., 2009; Martens et al., 2012; see Figure 2A). The levels of expression of CD90 and CD105 reported in the current study are largely in agreement with other studies, which reported high expression (>95%) of both CD90 and CD105 in dental pulp tissues isolated from normal healthy teeth (Lindroos et al., 2008; Eslaminejad et al., 2015). We found that CD90 and CD105 were comparably expressed in hDPCs and cDPCs. Although, previous analysis of cDPCs showed increased expression of CD90 and CD146 compared to hDPCs these studies were performed on cDPCs isolated from teeth with irreversible pulpitis or from deep carious lesions (Alongi et al., 2010; Ma et al., 2012) and this may explain the differences between these studies and our current findings. In addition previous studies typically isolated DPCs from pulp chambers only, while in the present study cells were isolated from the pulp chamber and upper portion of the root canal and this may also account for some of the observed differences. We found that CD146 was expressed in around 23% of the hDPCs. Higher levels of expression (>80%) were reported in a previous study although this may be age related as young donors (aged 16–18 year.) were used in that study (Bakopoulou et al., 2011). In addition high vascularity is needed to complete root formation at the eruption stage and CD146 is expressed in perivascular MSCs (Baksh et al., 2007). In our study hDPCs and cDPCs showed low reactivity toward the hematopoietic stem cell marker CD45 (leukocyte common antigen) and this agrees with previous data which indicated that DPCs were either negative for CD45 or expressed this marker at very low concentrations <1–2% of the cell population (Lindroos et al., 2008; Huang et al., 2009; Bansal and Jain, 2015; Isobe et al., 2015) It was reported that leukocytes represent <1% of dental pulp cell population after enzymatic digestion and harvesting (Gaudin et al., 2015) although early passages of heterogeneous stromal cell populations may still contain traces of a CD45 positive population. hDPCs and cDPCs did not express the endothelial cell marker CD31 and this also agrees broadly with other reports (Lindroos et al., 2008; Vishwanath et al., 2013).
Previous studies have shown that hDPCs can differentiate to a matrix mineralization phenotype (Gronthos et al., 2000, 2002; d'Aquino et al., 2007; El-Gendy, 2010), while controversial data have been published regarding cDPCs isolated from permanent (Alongi et al., 2010; Wang et al., 2010; Pereira et al., 2012; Ma et al., 2014; Yazid et al., 2014) or deciduous (Yu et al., 2014; Werle et al., 2015) teeth affected by deep caries. Our data clearly show that cDPCs isolated from shallow carious lesions can differentiate to this phenotype and form a mineralized matrix (Figure 3). In fact cDPCs appeared to exhibit a higher differentiation potential compared with hDPCs as assessed by mineralization assay and marker gene expression (Figure 3) although there was no statistically significant difference in mineralization marker gene expression between hDPCs and cDPCs under either basal or mineralizing conditions (Figures 4A,B respectively). Nonetheless differentiation of cDPCs may be affected by the inflammatory micro-environment and this may argue for the retention of this phenotype in cultured cDPCs. Although, this requires further experimental verification such observations are consistent with earlier studies which showed that pro-inflammatory cytokines promoted the mineralization of DPCs (Yang et al., 2012).
Under basal conditions we report the up regulation of VEGFR2 and PECAM-1 in DPCs derived from carious dental pulp compared to cells derived from healthy pulp (Figure 4A). This agrees with previous findings in inflamed dental pulp and may occur in response to the inflammatory cytokines that are released into the pulp interstitial fluid during inflammation (Cohen et al., 1996; Chu et al., 2004). We also found up regulation of the inflammatory markers TLR-2 and TLR-4 under basal conditions in cDPCs v hDPCs and some evidence suggests cross talk between the TLR and VEGF axis to co-ordinate neo-angiogenic and inflammatory responses during tissue repair at site of injury (Akira et al., 2006; Bachmann et al., 2006; Pazgier et al., 2006).
The role of inflammatory cytokines during mineralized tissue repair is also controversial. We found that IL-6 and IL-8 were secreted into media conditioned by DPCs and there was a trend for higher concentrations of IL-6 and IL-8 in media conditioned by cDPCs compared with hDPCs under basal conditions (Figure 5). These findings are supported by earlier studies, which found that IL-6 and IL-8 expression was higher in carious than in heathy pulp (Zehnder et al., 2003; McLachlan et al., 2004; Silva et al., 2009) and argues that in vitro cDPCs retain an inflammatory phenotype. IL-6 was down-regulated in both hDPCs and cDPCs under mineralizing conditions. However treatment with the synthetic glucocorticoid dexamethasone inhibits IL-6 expression in different cell/tissue culture systems (Malaval et al., 1998; Liu et al., 2002; Cooper et al., 2014) and dexamethasone-free induction medium is required to examine whether IL-6 expression remains down-regulated. In contrast, IL-8 secretion into conditioned medium increased under mineralizing conditions. This agrees with previous reports showing increased IL-8 expression under mineralizing conditions in human MSCs (Pereira et al., 2009) and up-regulation of IL-8 in bone marrow MSCs during BMP induced differentiation (Zachos et al., 2006).
In conclusion we have shown that, compared to healthy DPCs, cells derived from pulp with superficial caries involvement (cDPCs)—show higher clonogenic potential; have an equivalent proportion of putative stem cell populations; show enhanced matrix mineralization capability; have enhanced angiogenic marker expression; retain the inflammatory phenotype in vitro characteristic of superficial caries lesions in vivo. These findings suggest that cDPCs may be used for further investigation of the cross talk between inflammatory, angiogenic, and mineralization pathways in repair of carious pulp. In addition cells derived from carious pulps (almost always discarded) may have potential for future applications in mineralized tissue repair and regeneration.
Author Contributions
HA conducted experiments, collected and analyzed data, and contributed to writing the manuscript. RE conducted experiments, collected and analyzed data, designed experiments and contributed to writing the manuscript. JM and DD analyzed data and contributed to writing the paper. JB designed experiments and prepared the final version of the manuscript.
Conflict of Interest Statement
The authors declare that the research was conducted in the absence of any commercial or financial relationships that could be construed as a potential conflict of interest.
Acknowledgments
HA acknowledges the Royal Embassy of Saudi Arabia–Cultural Bureau (UK) for financial support. RE acknowledges WELMEC a Centre of Excellence in Medical Engineering funded by Wellcome Trust and EPSRC under grant number WT088908/Z/09/Z for financial support.
Supplementary Material
The Supplementary Material for this article can be found online at: http://journal.frontiersin.org/article/10.3389/fphys.2017.00244/full#supplementary-material
Supplementary Figure 1. Single parameter histograms showing the expression of cell surface markers in hDPSCs (H1, H2, H3) and cDPSCs (C1, C2, C3) cultures under basal conditions. Data are shown for isotype controls (gray) and positively stained cells – blue (hDPCs) and red (cDPCs). (a) CD146 (PE-Cy7); (b) CD90 (PerCP-5.5); (c) CD105 (BV421); (d) CD45 (APC-Cy7); (e) CD31 (FITC). Percentage positively stained cells are indicated for each marker.
References
Akira, S., Uematsu, S., and Takeuchi, O. (2006). Pathogen recognition and innate immunity. Cell 124, 783–801. doi: 10.1016/j.cell.2006.02.015
Alkharobi, H., Alhodhodi, A., Hawsawi, Y., Alkafaji, H., Devine, D., El-Gendy, R., et al. (2016). IGFBP-2 and -3 co-ordinately regulate IGF1 induced matrix mineralisation of differentiating human dental pulp cells. Stem Cell Res. 17, 517–522. doi: 10.1016/j.scr.2016.09.026
Alongi, D. J., Yamaza, T., Song, Y., Fouad, A. F., Romberg, E. E., Shi, S., et al. (2010). Stem/progenitor cells from inflamed human dental pulp retain tissue regeneration potential. Regen. Med. 5, 617–631. doi: 10.2217/rme.10.30
Bachmann, M. F., Kopf, M., and Marsland, B. J. (2006). Chemokines: more than just road signs. Nature Rev. Immunol. 6, 159–164. doi: 10.1038/nri1776
Bakopoulou, A., Leyhausen, G., Volk, J., Tsiftsoglou, A., Garefis, P., Koidis, P., et al. (2011). Comparative analysis of in vitro osteo/odontogenic differentiation potential of human dental pulp stem cells (DPSCs) and stem cells from the apical papilla (SCAP). Arch. Oral Biol. 56, 709–721. doi: 10.1016/j.archoralbio.2010.12.008
Baksh, D., Yao, R., and Tuan, R. S. (2007). Comparison of proliferative and multilineage differentiation potential of human mesenchymal stem cells derived from umbilical cord and bone marrow. Stem Cells 25, 1384–1392. doi: 10.1634/stemcells.2006-0709
Bansal, R., and Jain, A. (2015). Current overview on dental stem cells applications in regenerative dentistry. J. Nat. Sci. Biol. Med. 6:29. doi: 10.4103/0976-9668.149074
Bjørndal, L. (2008). The caries process and its effect on the pulp: the science is changing and so is our understanding. Pediatr. Dent. 30, 192–196. doi: 10.1016/j.joen.2008.02.037
Chu, S. C., Tsai, C. H. S., Yang, F. F., Huang, M. Y., Su, F. Y., Hsieh, Y. S., et al. (2004). Induction of vascular endothelial growth factor gene expression by proinflammatory cytokines in human pulp and gingival fibroblasts. J. Endod. 30, 704–707. doi: 10.1097/01.DON.0000129962.65752.C6
Cohen, T., Nahari, D., Cerem, L. W., Neufeld, G., and Levi, Z. B. (1996). Interleukin 6 induces the expression of vascular endothelial growth factor. J. Biol. Chem. 271, 736–741. doi: 10.1074/jbc.271.2.736
Cooper, P. R., Holder, M. J., and Smith, A. J. (2014). Inflammation and regeneration in the dentin-pulp complex: a double-edged sword. J. Endod. 40, S46–S51. doi: 10.1016/j.joen.2014.01.021
d'Aquino, R., Graziano, A., Sampaolesi, M., Laino, G., Pirozzi, G., De Rosa, A., et al. (2007). Human postnatal dental pulp cells co-differentiate into osteoblasts and endotheliocytes: a pivotal synergy leading to adult bone tissue formation. Cell Death Differ. 14, 1162–1171. doi: 10.1038/sj.cdd.4402121
d'Aquino, R., Papaccio, G., Laino, G., and Graziano, A. (2008). Dental pulp stem cells: a promising tool for bone regeneration. Stem Cell Rev. 4, 21–26. doi: 10.1007/s12015-008-9013-5
Dominici, M., Le Blanc, K., Mueller, I., Slaper-Cortenbach, I., Marini, F., Krause, D., et al. (2006). Minimal criteria for defining multipotent mesenchymal stromal cells. International society for cellular therapy position statement. Cytotherapy 8, 315–317. doi: 10.1080/14653240600855905
El-Gendy, R. O. O. M. (2010). Bone Tissue Engineering Using Dental Pulp Stem Cells. Leeds: University of Leeds.
El-Gendy, R., Kirkham, J., Newby, P. J., Mohanram, Y., Boccaccini, A. R., and Yang, X. B. (2015). Investigating the vascularization of tissue-engineered bone constructs using dental pulp cells and 45S5 Bioglass(R) Scaffolds. Tissue Eng. Part A 21, 2034–2043. doi: 10.1089/ten.tea.2014.0485
Eslaminejad, M. B., Nazarian, H., Shariati, M., Vahabi, S., and Falahi, F. (2015). Isolation and in vitro characterization of mesenchymal stem cells derived from the pulp tissue of human third molar tooth. Iran. J. Med. Sci. 35, 216–225. doi: 10.1007/s00418-009-0646-5
Freeman, F. E., Haugh, M. G., and McNamara, L. M. (2015). An in vitro bone tissue regeneration strategy combining chondrogenic and vascular priming enhances the mineralization potential of mesenchymal stem cells in vitro while also allowing for vessel formation. Tissue Eng. Part A 21, 1320–1332. doi: 10.1089/ten.tea.2014.0249
Gafni, Y., Turgeman, G., Liebergal, M., Pelled, G., Gazit, Z., and Gazit, D. (2004). Stem cells as vehicles for orthopedic gene therapy. Gene Ther. 11, 417–426. doi: 10.1038/sj.gt.3302197
Gaudin, A., Renard, E., Hill, M., Bouchet-Delbos, L., Bienvenu-Louvet, G., Farges, J. C., et al. (2015). Phenotypic analysis of immunocompetent cells in healthy human dental pulp. J. Endod. 41, 621–627. doi: 10.1016/j.joen.2015.01.005
Gronthos, S., Brahim, J., Li, W., Fisher, L., Cherman, N., Boyde, A., et al. (2002). Stem cell properties of human dental pulp stem cells. J. Dent. Res. 81, 531–535. doi: 10.1177/154405910208100806
Gronthos, S., Mankani, M., Brahim, J., Robey, P. G., and Shi, S. (2000). Postnatal human dental pulp stem cells (DPSCs) in vitro and in vivo. Proc. Natl. Acad. Sci. U.S.A. 97, 13625–13630. doi: 10.1073/pnas.240309797
Huang, G.-J., Gronthos, S., and Shi, S. (2009). Mesenchymal stem cells derived from dental tissues vs. those from other sources: their biology and role in regenerative medicine. J. Dent. Res. 88, 792–806. doi: 10.1177/0022034509340867
Isobe, Y., Koyama, N., Nakao, K., Osawa, K., Ikeno, M., Yamanaka, S., et al. (2015). Comparison of human mesenchymal stem cells derived from bone marrow, synovial fluid, adult dental pulp, and exfoliated deciduous tooth pulp. Int. J. Oral Maxillofac. Surg. 45, 124–31. doi: 10.1016/j.ijom.2015.06.022
Kim, Y. S., Min, K. S., Jeong, D. H., Jang, J. H., Kim, H. W., and Kim, E. C. (2010). Effects of fibroblast growth factor-2 on the expression and regulation of chemokines in human dental pulp cells. J. Endod. 36, 1824–1830. doi: 10.1016/j.joen.2010.08.020
Krebsbach, P. H., and Robey, P. G. (2002). Dental and skeletal stem cells: potential cellular therapeutics for craniofacial regeneration. J. Dent. Educ. 66, 766–773
Ledesma-Martínez, E., Mendoza-Núñez, V. M., and Santiago-Osorio, E. (2015). Mesenchymal stem cells derived from dental pulp: a review. Stem Cells Int. 2016:4709572. doi: 10.1155/2016/4709572
Lei, M., Li, K., Li, B. L., Gao, N. F., Chen, M., and Jin, Y. (2014). Mesenchymal stem cell characteristics of dental pulp and periodontal ligament stem cells after in vivo transplantation. Biomaterials 35, 6332–6343. doi: 10.1016/j.biomaterials.2014.04.071
Lin, N. H., Gronthos, S., and Bartold, P. (2008). Stem cells and periodontal regeneration. Aust. Dent. J. 53, 108–121. doi: 10.1111/j.1834-7819.2008.00019.x
Lindroos, B., Mäenpää, K., Ylikomi, T., Oja, H., Suuronen, R., and Miettinen, S. (2008). Characterisation of human dental stem cells and buccal mucosa fibroblasts. Biochem. Biophys. Res. Commun. 368, 329–335. doi: 10.1016/j.bbrc.2008.01.081
Liu, F., Aubin, J., and Malaval, L. (2002). Expression of leukemia inhibitory factor (LIF)/interleukin-6 family cytokines and receptors during in vitro osteogenesis: differential regulation by dexamethasone and LIF. Bone 31, 212–219. doi: 10.1016/s8756-3282(02)00806-2
Ma, D., Cui, L., Gao, J., Yan, W., Liu, Y., Xu, S., et al. (2014). Proteomic analysis of mesenchymal stem cells from normal and deep carious dental pulp. PLos ONE 9:e97026. doi: 10.1371/journal.pone.0097026
Ma, D., Gao, J., Yue, J., Yan, W., Fang, F., and Wu, B. (2012). Changes in proliferation and osteogenic differentiation of stem cells from deep caries in vitro. J. Endod. 38, 796–802. doi: 10.1016/j.joen.2012.02.014
Malaval, L., Gupta, A. K., Liu, F., Delmas, P. D., and Aubin, J. E. (1998). LIF, but Not IL-6, regulates osteoprogenitor differentiation in rat calvaria cell cultures: modulation by dexamethasone. J. Bone Miner. Res. 13, 175–184. doi: 10.1359/jbmr.1998.13.2.175
Martens, W., Wolfs, E., Struys, T., Politis, C., Bronckaers, A., and Lambrichts, I. (2012). Expression pattern of basal markers in human dental pulp stem cells and tissue. Cells Tissues Organs 196, 490–500. doi: 10.1159/000338654
McLachlan, J. L., Sloan, A. J., Smith, A. J., Landini, G., and Cooper, P. R. (2004). S100 and cytokine expression in caries. Infect. Immun. 72, 4102–4108. doi: 10.1128/IAI.72.7.4102-4108.2004
Nakashima, M., Iohara, K., Ishikawa, M., Ito, M., Tomokiyo, A., Tanaka, T., et al. (2004). Stimulation of reparative dentin formation by ex vivo gene therapy using dental pulp stem cells electrotransfected with growth/differentiation factor 11 (Gdf11). Hum. Gene Ther. 15, 1045–1053. doi: 10.1089/1043034042431164
Papaccio, G., Graziano, A., d'Aquino, R., Graziano, M. F., Pirozzi, G., Menditti, D., et al. (2006). Long-term cryopreservation of dental pulp stem cells (SBP-DPSCs) and their differentiated osteoblasts: a cell source, for tissue repair. J. Cell. Physiol. 208, 319–325. doi: 10.1002/jcp.20667
Pazgier, M., Hoover, D., Yang, D., Lu, W., and Lubkowski, J. (2006). Human β-defensins. Cell. Mol. Life Sci. 63, 1294–1313. doi: 10.1007/s00018-005-5540-2
Pereira, C. T., Huang, W., Jarrahy, R., Rudkin, G., Yamaguchi, D. T., and Miller, T. A. (2009). Human and mouse osteoprogenitor cells exhibit distinct patterns of osteogenesis in three-dimensional tissue engineering scaffolds. Plast. Reconstr. Surg. 124, 1869–1879. doi: 10.1097/PRS.0b013e3181bf81ab
Pereira, L., Rubini, M., Silva, J., Oliveira, D., Silva, I. M., Poças-Fonseca, M. J., et al. (2012). Comparison of stem cell properties of cells isolated from normal and inflamed dental pulps. Int. Endod. J. 45, 1080–1090. doi: 10.1111/j.1365-2591.2012.02068.x
Rodríguez-Lozano, F. J., Bueno, C., Insausti, C. L., Meseguer, L., Ramirez, M., Blanquer, M., et al. (2011). Mesenchymal stem cells derived from dental tissues. Int. Endod. J. 44, 800–806. doi: 10.1111/j.1365-2591.2011.01877.x
Roederer, M. (2001). Spectral compensation for flow cytometry: visualization artifacts, limitations, and caveats. Cytometry 45, 194–205. doi: 10.1002/1097-0320(20011101)45:3<194::AID-CYTO1163>3.0.CO;2-C
Shi, S., Robey, P. G., and Gronthos, S. (2001). Comparison of human dental pulp and bone marrow stromal stem cells by cDNA microarray analysis. Bone 29, 532–539. doi: 10.1016/S8756-3282(01)00612-3
Silva, A. C. O., Faria, M. R., Fontes, A., Campos, M. S., and Cavalcanti, B. N. (2009). Interleukin-1 beta and interleukin-8 in healthy and inflamed dental pulps. J. Appl. Oral Sci. 17, 527–532. doi: 10.1590/S1678-77572009000500031
Simon, S., Berdal, A., Cooper, P., Lumley, P., Tomson, P., and Smith, A. (2011). Dentin-pulp complex regeneration from lab to clinic. Adv. Dent. Res. 23, 340–345. doi: 10.1177/0022034511405327
Smith, A., Scheven, B., Takahashi, Y., Ferracane, J., Shelton, R., and Cooper, P. (2012). Dentine as a bioactive extracellular matrix. Arch. Oral Biol. 57, 109–121. doi: 10.1016/j.archoralbio.2011.07.008
Suchanek, J., Soukup, T., Visek, B., Ivancakova, R., Kucerova, L., and Mokry, J. (2009). Dental pulp stem cells and their characterization. Biomed. Pap. 153, 31–35. doi: 10.5507/bp.0.2009.005
Vishwanath, V. R., Nadig, R. R., Nadig, R., Prasanna, J. S., Karthik, J., and Pai, V. S. (2013). Differentiation of isolated and characterized human dental pulp stem cells and stem cells from human exfoliated deciduous teeth: an in vitro study. J. Conserv. Dent. 16:423. doi: 10.4103/0972-0707.117509
Wang, Z., Pan, J., Wright, J. T., Bencharit, S., Zhang, S., Everett, E. T., et al. (2010). Putative stem cells in human dental pulp with irreversible pulpitis: an exploratory study. J. Endod. 36, 820–825. doi: 10.1016/j.joen.2010.02.003
Werle, S. B., Lindemann, D., Steffens, D., Demarco, F. F., de Araujo, F. B., Pranke, P., et al. (2015). Carious deciduous teeth are a potential source for dental pulp stem cells. Clin. Oral Investig. 20, 75–81. doi: 10.1007/s00784-015-1477-5
Yalvac, M., Ramazanoglu, M., Rizvanov, A., Sahin, F., Bayrak, O., Salli, U., et al. (2010). Isolation and characterization of stem cells derived from human third molar tooth germs of young adults: implications in neo-vascularization, osteo-, adipo-and neurogenesis. Pharmacogenomics J. 10, 105–113. doi: 10.1038/tpj.2009.40
Yamada, Y., Nakamura, S., Ito, K., Sugito, T., Yoshimi, R., Nagasaka, T., et al. (2010). A feasibility of useful cell-based therapy by bone regeneration with deciduous tooth stem cells, dental pulp stem cells, or bone marrow-derived mesenchymal stem cells for clinical study using tissue engineering technology. Tissue Eng. Part A 16, 1891–1900. doi: 10.1089/ten.tea.2009.0732
Yang, X., Zhang, S., Pang, X., and Fan, M. (2012). Retracted: pro-inflammatory cytokines induce odontogenic differentiation of dental pulp-derived stem cells. J. Cell. Biochem. 113, 669–677. doi: 10.1002/jcb.0.23396
Yazid, F. B., Gnanasegaran, N., Kunasekaran, W., Govindasamy, V., and Musa, S. (2014). Comparison of immunodulatory properties of dental pulp stem cells derived from healthy and inflamed teeth. Clin. Oral Investig. 18, 2103–2112. doi: 10.1007/s00784-014-1207-4
Yu, S., Diao, S., Wang, J., Ding, G., Yang, D., and Fan, Z. (2014). Comparative analysis of proliferation and differentiation potentials of stem cells from inflamed pulp of deciduous teeth and stem cells from exfoliated deciduous teeth. Biomed. Res. Int. 2014:930907. doi: 10.1155/2014/930907
Zachos, T. A., Shields, K. M., and Bertone, A. L. (2006). Gene-mediated osteogenic differentiation of stem cells by bone morphogenetic proteins-2 or-6. J. Orthop. Res. 24, 1279–1291. doi: 10.1002/jor.20068
Zehnder, M., Delaleu, N., Du, Y., and Bickel, M. (2003). Cytokine gene expression part of host defence in pulpitis. Cytokine 22, 84–88. doi: 10.1016/S1043-4666(03)00116-9
Keywords: dental pulp cells (DPCs), osteogenesis, odontogenesis, inflammation, regeneration
Citation: Alkharobi H, Beattie J, Meade J, Devine D and El-Gendy R (2017) Dental Pulp Cells Isolated from Teeth with Superficial Caries Retain an Inflammatory Phenotype and Display an Enhanced Matrix Mineralization Potential. Front. Physiol. 8:244. doi: 10.3389/fphys.2017.00244
Received: 08 February 2017; Accepted: 07 April 2017;
Published: 28 April 2017.
Edited by:
Catherine Chaussain, Université Paris Descartes, FranceReviewed by:
Mina Mina, University of Connecticut Health Center, USAIvo Lambrichts, University of Hasselt, Belgium
Jean-Christophe Farges, Claude Bernard University Lyon 1, France
Copyright © 2017 Alkharobi, Beattie, Meade, Devine and El-Gendy. This is an open-access article distributed under the terms of the Creative Commons Attribution License (CC BY). The use, distribution or reproduction in other forums is permitted, provided the original author(s) or licensor are credited and that the original publication in this journal is cited, in accordance with accepted academic practice. No use, distribution or reproduction is permitted which does not comply with these terms.
*Correspondence: Reem El-Gendy, ci5lbC1nZW5keUBsZWVkcy5hYy51aw==