- 1Department of Biomaterials, Institute of Clinical Dentistry, University of Oslo, Oslo, Norway
- 2Department of Bioinformatics, Institute of Organic Chemistry and Biochemistry, Czech Academy of Sciences, Prague, Czechia
During amelogenesis the extracellular enamel matrix protein AMBN is quickly processed into 17 kDa (N-terminus) and 23 kDa (C-terminus) fragments. In particular, alternatively spliced regions derived by exon 5/6 within the N-terminus region are known to be critical in biomineralization. Human mesenchymal stem cells (hMSC) also express and secrete AMBN, but it is unclear if this expression has effects on the hMSC themselves. If, as suggested from previous findings, AMBN act as a signaling molecule, such effects could influence hMSC growth and differentiation, as well as promoting the secretion of other signaling proteins like cytokines and chemokines. If AMBN is found to modulate stem cell behavior and fate, it will impact our understanding on how extracellular matrix molecules can have multiple roles during development ontogenesis, mineralization and healing of mesenchymal tissues. Here we show that synthetic peptides representing exon 5 promote hMSC proliferation. Interestingly, this effect is inhibited by the application of a 15 aa peptide representing the alternatively spliced start of exon 6. Both peptides also influence gene expression of RUNX2 and osteocalcin, and promote calcium deposition in cultures, indicating a positive influence on the osteogenic capacity of hMSC. We also show that the full-length AMBN-WT and N-terminus region enhance the secretion of RANTES, IP-10, and IL-8. In contrast, the AMBN C-terminus fragment and the exon 5 deleted AMBN (DelEx5) have no detectable effects on any of the parameters investigated. These findings suggest the signaling effect of AMBN is conveyed by processed products, whereas the effect on proliferation is differentially modulated through alternative splicing during gene expression.
Introduction
Ameloblastin (AMBN) is an extracellular matrix protein expressed in mesenchymal and epithelial cells (Fong et al., 1998). Epithelial-mesenchymal interactions initiate tooth development and has been shown to induce the expression of AMBN (Takahashi et al., 2012). AMBN is also involved in biomineralization in other tissues than teeth, and is expressed and secreted from cultured human mesenchymal stem cells (hMSC) and osteoblasts (Tamburstuen et al., 2011). Expression of AMBN (Spahr et al., 2006; Tamburstuen et al., 2010) in cells bordering bone defects suggest a role for AMBN in the recruitment, growth and differentiation of hMSC, and is a potential target for clinical interventions for bone healing.
AMBN is known to modulate proliferation and differentiation of ameloblasts, periodontal ligament cells (PDL), pulp cells (Nakamura et al., 2006) and hMSC; (Fukumoto et al., 2004; Sonoda et al., 2009; Tamburstuen et al., 2010; Zhang et al., 2011). AMBN splice variants are widely distributed in time and location, and have several roles during dental biomineralization. During early tooth development in mice, the predominant splice variant is 15 aa shorter than the splice variant expressed in later stages. These 15 aa (Q9NP70) derive from the start of exon 6 (Cerný et al., 1996; Fong et al., 1996; Hu et al., 1997; Lee et al., 2003; Ravindranath et al., 2007). However, spatial distribution and post-translational modification of the splice variants present in mesenchymal tissues like bone still needs to be investigated.
Extracellular full-length AMBN has not been identified in vivo. This is most probably due to rapid degradation by co-secreted specific matrix metalloproteases like MMP-20 (Uchida et al., 1997). Thus, it is important to understand the dynamics of AMBN processing and the biological role of the processed products. It is interesting that recombinant full-length AMBN has been found to enhance the secretion of cytokines and chemokines involved in inflammation and recruitment of progenitor cells (Tamburstuen et al., 2010). This effect is evident in the healing of critical-size defects in the jawbone of rats (Spahr et al., 2006; Tamburstuen et al., 2010) and in pulpal wound healing in pigs (Nakamura et al., 2006). These findings suggest that the full-length product has a biological (or at least pharmacological) effect on its own or that the full-length molecule act as a founding source for shorter, active, peptides.
Unprocessed ameloblastin (AMBN-WT) is a two-domain protein where the amino- and carboxyl ends are differently organized with opposing chemical properties (Vymetal et al., 2008). The N-terminus is defined by the first 10 exons encoding 222 aa (human). Both the unprocessed ameloblastin and the N-terminus may form fibrils through self-assembly supported by the exon 5 derived region (Wald et al., 2013). The C-terminus is defined by the last three exons encoding 225 aa (Toyosawa et al., 2000). It has been suggested that parts of the porcine N-terminus (17 kDa fragment) is active in mineralization and regeneration (Fukae et al., 2006; Stout et al., 2014), whereas the C-terminus product may have a role in cell surface attachment (Sonoda et al., 2009).
In silico modeling of AMBN-WT folding, suggests that some discrete peptide-sequences are exposed on the surface of the folded protein structure (Vymetal et al., 2008). It is reasonable to assume these domains have biological functions that are presently unknown. Whether these domains act in concert or have individual activities also need assessment. Based on in silico modeling, we have designed synthetic peptides from exons 2–13 (without signal peptide) representing these exposed domains. In an attempt to look for biological effects of AMBN and the processing products as suggested by Tamburstuen et al. and others (Nakamura et al., 2006; Spahr et al., 2006; Tamburstuen et al., 2011), these peptides and various other AMBN fragments were added to cultures of hMSC, and the cells were monitored for changes in growth and differentiation as well as effects on levels of selected cytokine and chemokine secretion.
Materials and Methods
Experimental Design
Human mesenchymal stem cells (hMSC; Cat.no: PT-2501, Lonza Walkersville, MD, USA) were maintained in growth medium [GM; Cat.no: PT-3238 supplemented with MSCGM SingleQuots, Cat.no: PT-4105, Lonza (http://www.lonza.com/products-services/bio-research/stem-cells/adult-stem-cells-and-media/human-mesenchymal-stem-cells-media.aspx)] and changed every 3rd day. These hMSC cells are isolated from normal (non-diabetic) adult human bone marrow withdrawn from bilateral punctures of the posterior iliac crests of healthy volunteers.
The recombinant AMBN protein, fragments, and peptides, presented in Figure 1, were produced and purified as described by Wald et al. (2013).
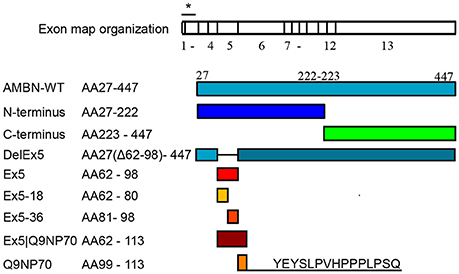
Figure 1. Overview of recombinant ameloblastin (AMBN) proteins and derivatives of the N-terminus region. Signal sequence of the 26 amino acids is indicated with asterisk in the exon map that is scaled according to the stretch of residue length in each exon.
Human MSC were incubated with 0.1 μM and 0.2 μM of AMBN-WT, AMBN-WT without Exon 5 (DelEx5), N-terminus of AMBN, C-terminus of AMBN, or 0.2 μM of exon 5 related peptides [Ex5 (AA62-98), Ex5-18 (AA62-80), Ex5-36 (AA81-98), Ex5|Q9NP70 (AA62-113), and Q9NP70 (AA99-113)]. Untreated hMSC were used as control at each time point tested. Cells and culture medium were harvested after 1, 3, 7, 14, 21, and 28 days of incubation.
Proliferation Assay
hMSC (6000 cells/well) were seeded in 48 well plates and incubated with the various test fragments or controls for 24 h. New DNA produced in the cells was labeled with 0.1 μCi [3H]-thymidine in a 12 h pulse prior to harvesting. The cells were then washed twice in PBS and then twice in 5% TCA to remove excess thymidine, and the remaining pellet dissolved in 1 M NaOH. Optifluor Scintillation liquid (Lumagel LSC GE BV, Groningen, Netherlands; 4 ml) was added, and radioactivity was measured in a Packard 1500 TRI-CARB liquid scintillation counter (Perkin Elmer, Shelton, CT, USA).
Measurements of Secreted Biomarkers
Harvested cell-culture-medium samples were concentrated 5-fold in spin columns with a 3 kD cut-off (Pall Life Science, Ann Arbor, MI, USA).
The concentrations of Eotaxin, granulocyte-colony stimulating factor (G-CSF), interferon (IFN) α2, IFNγ, interleukin (IL)-1α, IL-1β, IL-1 Receptor Antagonist (RA), IL-2, IL-4, IL-5, IL-6, IL-7, IL-8, IL-10, IL-12, IL-13, IL-15, IL-17, interferon gamma-inducible protein (IP-10), monocyte chemoattractant protein-1 (MCP-1), macrophage inflammatory protein-1 alpha (MIP-1α), macrophage inflammatory protein-1 beta (MIP-1β), regulated upon activation normal T-cell expressed and secreted (RANTES), tumor necrosis factor alpha (TNFα), vascular endothelial growth factor (VEGF), as well as adreno-corticotrophic hormone (ACTH), Dickkopf-1 (DKK1), Insulin, Leptin, osteoprotegerin (OPG), osteocalcin (OCN), osteopontin (OPN), sclerostin (SOST), fibroblast growth factor-23 (FGF-23), respectively, were measured using HCYTOMAG-60K and HBNMAG-51K assays (MILLIPORE corporation, Billerica, MA, USA), respectively, and analyzed with the Luminex xPONENT version 3.1.871 or MILLIPLEX™ Analyst version 5.1 software in the Luminex-200 system (Luminex Corp., Austin, TX, USA). Only the cytokines and chemokines that showed significant change are discussed here.
mRNA Isolation
hMSC were washed in PBS and lysed, and the mRNA was isolated using magnetic beads according to manufacturer's instructions (Dynabeads Oligo (dT)25, Life Technologies, Gaithersburg, MD, USA). The mRNA was separated from the beads by heat treatment (80°C for 2 min), and quantified using a nano-drop spectrophotometer (ND-1000, Thermo Scientific, Wilmington, DE, USA, with software version 3.3.1.).
Real Time PCR
cDNA was generated from mRNA using Revertaid First Strand cDNA synthesis kit (Fermentas, Burlington, Ontario, Canada) according to the producer's instructions. Real time PCR was performed using Ssoadvanced SYBRGreen Supermix (Bio-rad, Hercules, CA, USA) in a reaction mix of 20 μl (1 ng cDNA) in 96 well plates using the CFX Connect™-system. Gene expression was normalized to reference housekeeping genes β-actin and glyceraldehyde phosphate (GADPH) using the ΔΔCT method with Bio-Rad CFX Manager software version 2.1. The primer sequences used are listed in Table 1.
Mineralization
Cells were cultured to confluence in 12-well plates and then treated with AMBN or its fragments in either GM or osteogenic differentiation media (DM; Cat.no: PT-3924, supplemented with hMSC osteogenic SinglequotsTM) for up to 28 days. Medium was changed every 3rd day. Upon harvest, the cells were washed three times with PBS, fixed in 95% ethanol for 30 min, and then stained with 1% alizarin red for 5 min as described elsewhere (Dahl, 1952). To quantify mineralization, the alizarin red deposition was extracted with cetyl pyridinium chloride (Sigma-Aldrich, St. Louis, MO, USA) at room temperature, and measured at 562 nm in (EL × 800 Absorbance Reader, BioTek instruments, Winooski, VT, USA).
Statistics
Student t-test was used to evaluate the effect of AMBN and its fragments compared to untreated controls at each individual time point. The Mann-Whitney U- Test was used if the results were not normally distributed. The significance level was set to P ≤ 0.05.
Result
Proliferation of hMSC is Influenced by Peptides Derived by exon 5 and Q9NP70
AMBN-WT (0.1 μM) enhanced cell proliferation of hMSC to 1.8-fold (P = 0.002) of the control. DelEx5 was found to enhance proliferation to 1.5-fold (P = 0.004), whereas no effects were observed from the C-terminus or the N-terminus fragments alone at the time points tested (Figure 2A). Among the tested peptides, Ex5 enhanced the proliferation to 2.6-fold (P = 0.004) while Q9NP70 and Ex5|Q9NP70 both inhibited proliferation to 0.5-fold of control (P = 0.015 and P = 0.003, respectively; Figure 2B).
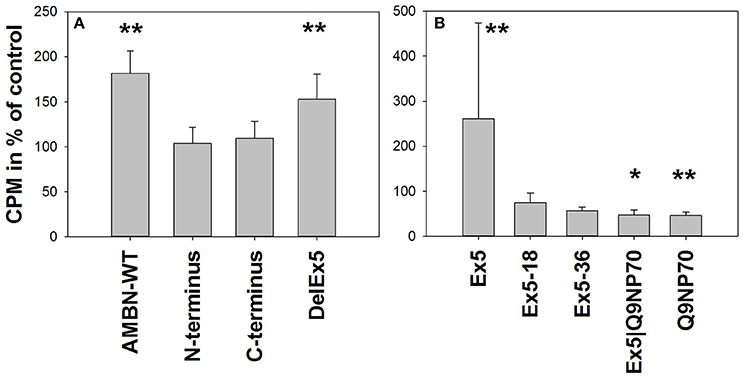
Figure 2. Proliferation measured as [3H]-thymidine incorporation in hMSC exposed to (A) 0.1 μM AMBN-WT, N-terminus, C-terminus or DelEx5 (n = 6). (B) 0.2 μM Ex5, Ex5-18, Ex5-36, Ex5|Q9NP70, or Q9NP70 (n = 12). 3H-thymidine incorporation (CPM) was calculated relative to untreated control cells (%), and presented as mean ± SD. *Indicate P < 0.05, **Indicate P < 0.01.
Cytokine and Chemokine Secretion is Enhanced by AMBN-WT and N-Terminus
AMBN-WT (0.2 μM) enhanced the secretion of RANTES 19 and 21-fold at day 7 and 14 respectively (P = 0.015 and P = 0.019). The secretion of IP-10 was enhanced 22-fold and 24-fold at day 1 and day 14, respectively (P = 0.029 and P = 0.047). Finally AMBN-WT enhanced the secretion of MIP-1α 8-fold at day 1 (P = 0.043) and 12-fold at day 14 (P = 0.001; Table 2). Lower concentration (0.1 μM) of AMBN-WT produced only a slight increase in secretion of MCP-1 and IL-6 at day 1 (values not shown).
N-terminus (0.2 μM) enhanced the secretion of RANTES 2.5, 4.5, and 3-fold at days 1, 7, and 14 respectively (P = 0.036, P = < 0.001, P = 0.003). The secretion of IP-10 was enhanced 5.5 and 2.7-fold at day 1 and 14, respectively (P = 0.003 and P = 0.037). N-terminus enhanced the secretion of MIP-1α 2.6-fold at day 14 (P = 0.004). The N-terminus also significantly enhanced secretion of IL-8 2.5-fold at day 3 (P = 0.038; Table 2).
The C-terminus and DelEx5 did not have any significant effects on the secretion of cytokines or chemokines, nor did they influence any differentiation markers tested.
Differentiation of hMSC are Stimulated by Peptides Derived by exon 5 and Q9NP70
The N-terminus and the Ex5, Ex5-18, and Ex5|Q9NP70 peptides all stimulated the mRNA expression of RUNX2 (3-fold (P = 0.008), 1.9 -fold (P = 0.016), 2.1-fold (P = 0.004), and 1.5-fold (P = 0.019)), respectively (Figure 3A). Ex5 stimulated the mRNA expression of OCN 2.5-fold (P = 0.009; Figure 3B), however no significant effect was observed on the secretion of OCN to the cell culture medium (results not shown). AMBN-WT stimulated the mRNA expression of RANKL; however neither AMBN nor its fragments had any significant effect on mRNA expression of OPG at the time-point analyzed (Figures 3C,D, respectively).
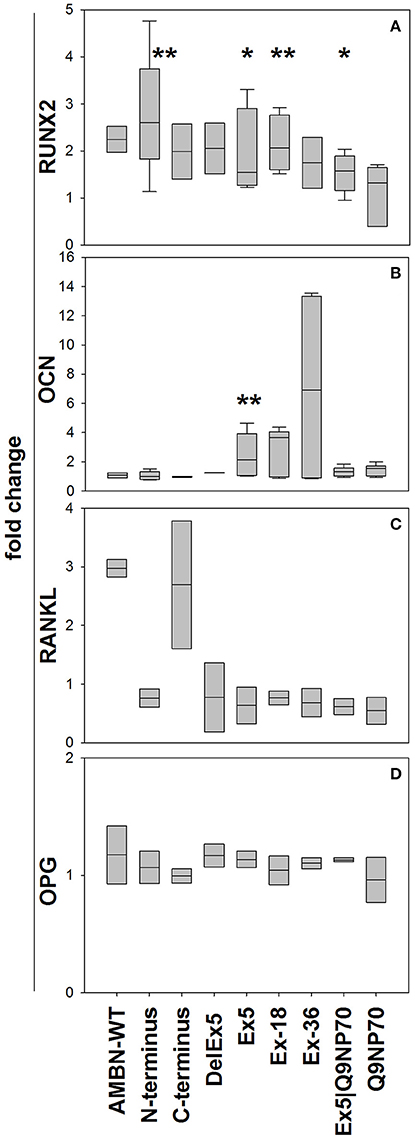
Figure 3. Gene expression of RUNX2 (A), OCN (B), RANKL (C), and OPG (D) in human hMSCs at 72 h. Expression was normalized against the reference genes Glyceraldehyde-3-Phosphate Dehydrogenase (GADPH) and Beta Actin using the ΔΔCT method. Data are calculated relative to untreated control as fold-changes and presented as mean ± SD (n = 5) (%). *Indicate P < 0.05, **Indicate P < 0.01.
Mineralization of hMSC is Mostly Influenced by exon 5 Derived Peptides
In initial tests with hMSC growing in regular medium for 21 days, none of the larger fragments AMBN-WT, N-terminus, C-terminus, or DelEx5, promoted in vitro mineralization. However, exon 5 and Q9NP70 derived peptides had a visible but not statistically significant, effect on the formation of mineralized nodules in hMSC cell cultures (results not shown). Only when a combination of Ex5 peptide and osteogenic medium (DM) was used did the mineralization increase significantly to 1.7-fold over the DM-only control (P = 0.029; Figure 4).
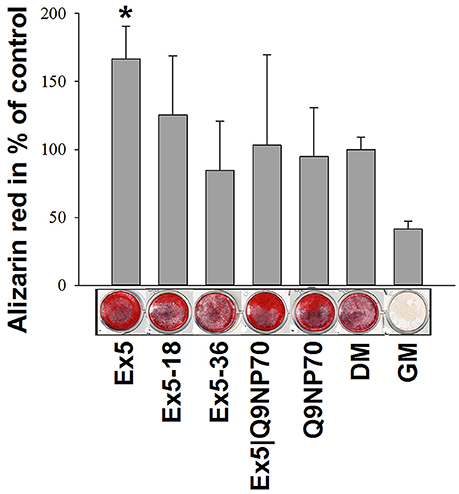
Figure 4. Extracted alizarin red from mineralized surfaces of hMSC after 28 days incubation with Ex5, Ex5-18, Ex5-36, Ex5|Q9NP70, or Q9NP70 (0.2 μM). Data are presented as mean ± SD (n = 5) (%). *Indicate P < 0.05.
Discussion
AMBN is first and foremost an extra cellular matrix protein, and may as such constitute a slow-release depot for biological signals. AMBN has not been identified as an intact soluble protein in vivo (Brookes et al., 2001; Iwata et al., 2007), and no complete information is available on the processing of AMBN in tissues other than in teeth. In other tissues, fragments are probably released into solution from the self-assembled AMBN complex, but little is known about the nature and the function of these fragments. Here we have shown that some selected fragments have discrete and significant effects on cultured hMSC.
To be able to compare different peptides and fragments we performed the experiments with equimolar concentrations. The recombinant proteins and peptides were administered to hMSC in a dosage (0.2 μM) to reflect the levels previously shown to be secreted from cultured hMSC (Tamburstuen et al., 2011) and that has been shown to have effects in other experiments with hMSC (Tamburstuen et al., 2010). We also included a lower concentration of AMBN to test the responsiveness to concentration levels similar as found in mature osteoblasts (0.1 μM; Tamburstuen et al., 2011). Both concentrations induced effects on proliferation and secretion of chemokines, suggesting that the AMBN secreted from progenitor cells and osteoblasts (Tamburstuen et al., 2011) indeed can have an effect as a signaling molecule in mesenchymal tissues.
Proliferation of hMSC Were Modulated by AMBN-WT and Regions Derived by exon 5 and Q9NP70
Proliferation of stem cells is a key feature in healing and tissue homeostasis. Here we have shown that AMBN-WT and peptide Ex5 stimulate hMSC proliferation. In fact Ex5 alone is more efficient as a signal for proliferation for these cells than the whole WT molecule, most probably due to superior bioavailability and/or a more favorable structural conformation. Interestingly, when exon 5 was deleted out of the full length AMBN there was little effect on proliferation, suggesting that Ex5 is a key element for this process. This was further demonstrated by the fact that additional processing of Ex5 into smaller fragments minimizes the effect on proliferation. The other fragments tested here did not significantly alter proliferation of hMSC. This is also supported by other studies, where a 15 aa peptide derived by exon 2 and exon 3 was shown not to influence proliferation (Kitagawa et al., 2011, 2016). Moreover, overexpression of AMBN lacking exon 5 and exon 6 in mice resulted in reduced bone growth, femur length, and higher fracture rate (Lu et al., 2016a,b) indirectly suggesting the importance of exon 5 in cell proliferation.
The regions derived by exon 5 and exon 6 have been shown to be vital for proper development of enamel (Smith et al., 2009; Wazen et al., 2009). Interestingly, the upstream part of exon 6 encodes a peptide (Q9NP70) that was found to inhibit proliferation of hMSC. This inhibition is probably stronger than the positive signal from Ex5 since the combination of the two (Ex5|Q9NP70) has a net inhibitory effect. However, this may also be due to steric inhibition or interfering pathways. The exon 6 derived Q9NP70 has been found in vivo during post-natal tooth development (Lee et al., 2003; Ravindranath et al., 2007) and thus may contribute by inhibiting cell proliferation at a stage where differentiation probably is more developmentally desired than proliferation.
The C-terminus fragment of AMBN showed no effect on hMSC proliferation. This fragment has however, been found to inhibit the proliferation of PDL and dental follicle cells (Zhang et al., 2011). This suggests that the effect of the various processed AMBN fragments might be tissue specific in real life situations.
Cytokines/Chemokines Secretion Enhanced by AMBN Fragments
Several reports suggest that AMBN can play a role in regeneration of bone (Nakamura et al., 2006; Spahr et al., 2006; Tamburstuen et al., 2010; Lu et al., 2016a,b). Overexpression of AMBN has been found to increase osteoclastogenesis (Lu et al., 2013). Osteoclastogenesis and inflammation are both critical processes influencing the early stages in regeneration of bone (Mountziaris and Mikos, 2008). Here we have demonstrated that AMBN-WT significantly enhanced the secretion of MCP-1, IL-6, RANTES, MIP-1α, and IP-10. All these factors have been associated with osteoclastogenesis (Kotake et al., 1996; Watanabe et al., 2004; Kim et al., 2005, 2006) in addition to inflammatory processes (Schall et al., 1990; Dufour et al., 2002). Interestingly, again, this effect is only observed from peptides derived from the N-terminus part of the AMBN molecule. Moreover, when the N-terminus fragment was tested alone it also enhanced the secretion of IL-8.
None of the other fragments, DelEx5, C-terminus, Ex5, Ex5-18, Ex5-36, Ex5|Q9NP70, or Q9NP70, had any effect on the markers analyzed here. Relevant for bone formation is the fact that IL-8 so far is the only chemokine known to enhance secretion of MMPs (Li et al., 2003).
Both the N-terminus and AMBN-WT self-assemble into fibrils (Wald et al., 2013). It is a surprising but striking feature that only these fibril forming regions affected the secretion of cytokines / chemokines from hMSC. Receptor oligomerization (George et al., 2002) has been shown to enhance secretion of chemokines (Martinez-Martin et al., 2015). Fibril formation of AMBN may be a way to expose multiple signaling motifs in close proximity, which in turn could bind several motifs at once and thus initiating chemokine associated receptor oligomerization.
The AMBN-WT includes the C-terminus that has been suggested to provide cell anchoring (Fukumoto et al., 2004) through DGEA binding motifs (Cerný et al., 1996). AMBN has also been shown to bind integrin β1 (Iizuka et al., 2011; Lu et al., 2013), and Integrin β1 ligands have been shown to enhance secretion of RANTES (Peng et al., 2005). This may explain the observation that AMBN-WT is more potent in enhancing secretion of cytokines and chemokines than its processed products.
Expressed Markers for Differentiation and Mineralization
The expression of RUNX2, a transcriptional factor for extracellular matrix proteins like collagen and OCN (Ducy et al., 1997; Kern et al., 2001), has been found to be enhanced by AMBN and synthetic peptides derived from its 17 kDa fragment in PDL (Kitagawa et al., 2011, 2016). Enhanced hMSC expression of RUNX2 and OCN by the N-terminus, exon 5, and Q9NP70 peptides presented here, support the idea that AMBN has effect on bone differentiation and mineralization.
This is further underlined by AMBN knockdown experiments that show reduction in alizarin red deposition during mineralized nodule formation (Iizuka et al., 2011). In the initial experiments on hMSC grown in GM we here found effects on mineralization only with the exon 5 and the Q9NP70 derivedpeptides. Exon 5 and exon 6 (including Q9NP70) have previously been shown to be important in enamel mineralization (Smith et al., 2009; Wazen et al., 2009). Accordingly, we confirmed that the Ex5 peptide stimulates calcium deposition under mineralizing conditions (DM) as visualized by alizarin staining.
Conclusion
AMBN-WT enhances the proliferation of hMSCs and secretion of cytokines/chemokines. Moreover, the two main AMBN processing fragments and derived peptides have markedly diverse effects. The N-terminus portion seems to enhance secretion of cytokines/chemokines, whereas peptides derived by exon 5 and Q9NP70 modulate proliferation, enhance secretion of markers for hMSC differentiation and extracellular mineralization. In contrast the C-terminus fragment shows no discernible effect on hMSC differentiation or proliferation and is probably only involved in cell attachment.
Author Contributions
ØS contributed in experimental design, performed and analyzed experiments, drafted and wrote the manuscript, SL contributed in experimental design, drafting and finalizing the manuscript. JV provided essential materials and contributed in experimental design. JG contributed in experimental design and in drafting of the manuscript, JR contributed in experimental design, drafting and finalizing the manuscript.
Conflict of Interest Statement
The authors declare that the research was conducted in the absence of any commercial or financial relationships that could be construed as a potential conflict of interest.
Acknowledgments
This work was supported by grants from EU (QLK3-CT-2001-00090) and the Research Council of Norway (231530) The authors are grateful for the skillful technical assistance of Aina-Mari Lian, Oral Research Laboratory and Rune Hartvig, Department of Biomaterials. The authors declare no conflict of interest.
References
Brookes, S. J., Kirkham, J., Shore, R. C., Wood, S. R., Slaby, I., and Robinson, C. (2001). Amelin extracellular processing and aggregation during rat incisor amelogenesis. Arch. Oral Biol. 46, 201–208. doi: 10.1016/S0003-9969(00)00121-7
Cerný, R., Slaby, I., Hammarstrom, L., and Wurtz, T. (1996). A novel gene expressed in rat ameloblasts codes for proteins with cell binding domains. J. Bone Miner. Res. 11, 883–891. doi: 10.1002/jbmr.5650110703
Dahl, L. K. (1952). A simple and sensitive histochemical method for calcium. Proc. Soc. Exp. Biol. Med. 80, 474–479. doi: 10.3181/00379727-80-19661
Ducy, P., Zhang, R., Geoffroy, V., Ridall, A. L., and Karsenty, G. (1997). Osf2/Cbfa1: a transcriptional activator of osteoblast differentiation. Cell 89, 747–754. doi: 10.1016/S0092-8674(00)80257-3
Dufour, J. H., Dziejman, M., Liu, M. T., Leung, J. H., Lane, T. E., and Luster, A. D. (2002). IFN-gamma-inducible protein 10 (IP-10; CXCL10)-deficient mice reveal a role for IP-10 in effector T cell generation and trafficking. J. Immunol. 168, 3195–3204. doi: 10.4049/jimmunol.168.7.3195
Fong, C. D., Cerny, R., Hammarstrom, L., and Slaby, I. (1998). Sequential expression of an amelin gene in mesenchymal and epithelial cells during odontogenesis in rats. Eur. J. Oral Sci. 106(Suppl. 1), 324–330. doi: 10.1111/j.1600-0722.1998.tb02193.x
Fong, C. D., Slaby, I., and Hammarstrom, L. (1996). Amelin: an enamel-related protein, transcribed in the cells of epithelial root sheath. J. Bone Miner. Res. 11, 892–898. doi: 10.1002/jbmr.5650110704
Fukae, M., Kanazashi, M., Nagano, T., Tanabe, T., Oida, S., and Gomi, K. (2006). Porcine sheath proteins show periodontal ligament regeneration activity. Eur. J. Oral Sci. 114(Suppl. 1), 212–218. discussion: 254–216, 381–212. doi: 10.1111/j.1600-0722.2006.00309.x
Fukumoto, S., Kiba, T., Hall, B., Iehara, N., Nakamura, T., Longenecker, G., et al. (2004). Ameloblastin is a cell adhesion molecule required for maintaining the differentiation state of ameloblasts. J. Cell Biol. 167, 973–983. doi: 10.1083/jcb.200409077
George, S. R., O'Dowd, B. F., and Lee, S. P. (2002). G-Protein-coupled receptor oligomerization and its potential for drug discovery. Nat. Rev. Drug Discov. 1, 808–820. doi: 10.1038/nrd913
Hu, C. C., Fukae, M., Uchida, T., Qian, Q., Zhang, C. H., Ryu, O. H., et al. (1997). Sheathlin: cloning, cDNA/polypeptide sequences, and immunolocalization of porcine enamel sheath proteins. J. Dent. Res. 76, 648–657. doi: 10.1177/00220345970760020501
Iizuka, S., Kudo, Y., Yoshida, M., Tsunematsu, T., Yoshiko, Y., Uchida, T., et al. (2011). Ameloblastin regulates osteogenic differentiation by inhibiting Src kinase via cross talk between integrin beta1 and CD63. Mol. Cell. Biol. 31, 783–792. doi: 10.1128/MCB.00912-10
Iwata, T., Yamakoshi, Y., Hu, J. C., Ishikawa, I., Bartlett, J. D., Krebsbach, P. H., et al. (2007). Processing of ameloblastin by MMP-20. J. Dent. Res. 86, 153–157. doi: 10.1177/154405910708600209
Kern, B., Shen, J., Starbuck, M., and Karsenty, G. (2001). Cbfa1 contributes to the osteoblast-specific expression of type I collagen genes. J. Biol. Chem. 276, 7101–7107. doi: 10.1074/jbc.M006215200
Kim, M. S., Day, C. J., and Morrison, N. A. (2005). MCP-1 is induced by receptor activator of nuclear factor-{kappa}B ligand, promotes human osteoclast fusion, and rescues granulocyte macrophage colony-stimulating factor suppression of osteoclast formation. J. Biol. Chem. 280, 16163–16169. doi: 10.1074/jbc.M412713200
Kim, M. S., Magno, C. L., Day, C. J., and Morrison, N. A. (2006). Induction of chemokines and chemokine receptors CCR2b and CCR4 in authentic human osteoclasts differentiated with RANKL and osteoclast like cells differentiated by MCP-1 and RANTES. J. Cell. Biochem. 97, 512–518. doi: 10.1002/jcb.20649
Kitagawa, M., Ando, T., Subarnbhesaj, A., Uchida, T., Miyauchi, M., and Takata, T. (2016). N-terminal region of human ameloblastin synthetic peptide promotes bone formation. Odontology 105, 116–121. doi: 10.1007/s10266-016-0243-8
Kitagawa, M., Kitagawa, S., Nagasaki, A., Miyauchi, M., Uchida, T., and Takata, T. (2011). Synthetic ameloblastin peptide stimulates differentiation of human periodontal ligament cells. Arch. Oral Biol. 56, 374–379. doi: 10.1016/j.archoralbio.2010.10.012
Kotake, S., Sato, K., Kim, K. J., Takahashi, N., Udagawa, N., Nakamura, I., et al. (1996). Interleukin-6 and soluble interleukin-6 receptors in the synovial fluids from rheumatoid arthritis patients are responsible for osteoclast-like cell formation. J. Bone Miner. Res. 11, 88–95. doi: 10.1002/jbmr.5650110113
Lee, S. K., Kim, S. M., Lee, Y. J., Yamada, K. M., Yamada, Y., and Chi, J. G. (2003). The structure of the rat ameloblastin gene and its expression in amelogenesis. Mol. Cells 15, 216–225.
Li, A., Dubey, S., Varney, M. L., Dave, B. J., and Singh, R. K. (2003). IL-8 directly enhanced endothelial cell survival, proliferation, and matrix metalloproteinases production and regulated angiogenesis. J. Immunol. 170, 3369–3376. doi: 10.4049/jimmunol.170.6.3369
Lu, X., Fukumoto, S., Yamada, Y., Evans, C. A., Diekwisch, T. G., and Luan, X. (2016a). Ameloblastin, an extracellular matrix protein, affects long bone growth and mineralization. J. Bone Miner. Res. 31, 1235–1246. doi: 10.1002/jbmr.2788
Lu, X., Ito, Y., Atsawasuwan, P., Dangaria, S., Yan, X., Wu, T., et al. (2013). Ameloblastin modulates osteoclastogenesis through the integrin/ERK pathway. Bone 54, 157–168. doi: 10.1016/j.bone.2013.01.041
Lu, X., Li, W., Fukumoto, S., Yamada, Y., Evans, C. A., Diekwisch, T., et al. (2016b). The ameloblastin extracellular matrix molecule enhances bone fracture resistance and promotes rapid bone fracture healing. Matrix Biol. 52–54, 113–126. doi: 10.1016/j.matbio.2016.02.007
Martinez-Martin, N., Viejo-Borbolla, A., Martin, R., Blanco, S., Benovic, J. L., Thelen, M., et al. (2015). Herpes simplex virus enhances chemokine function through modulation of receptor trafficking and oligomerization. Nat. Commun. 6, 6163. doi: 10.1038/ncomms7163
Mountziaris, P. M., and Mikos, A. G. (2008). Modulation of the inflammatory response for enhanced bone tissue regeneration. Tissue Eng. Part B Rev. 14, 179–186. doi: 10.1089/ten.teb.2008.0038
Nakamura, Y., Slaby, I., Spahr, A., Pezeshki, G., Matsumoto, K., and Lyngstadaas, S. P. (2006). Ameloblastin fusion protein enhances pulpal healing and dentin formation in porcine teeth. Calcif. Tissue Int. 78, 278–284. doi: 10.1007/s00223-005-0144-2
Peng, Q., Lai, D., Nguyen, T. T., Chan, V., Matsuda, T., and Hirst, S. J. (2005). Multiple beta 1 integrins mediate enhancement of human airway smooth muscle cytokine secretion by fibronectin and type I collagen. J. Immunol. 174, 2258–2264. doi: 10.4049/jimmunol.174.4.2258
Ravindranath, R. M., Devarajan, A., and Uchida, T. (2007). Spatiotemporal expression of ameloblastin isoforms during murine tooth development. J. Biol. Chem. 282, 36370–36376. doi: 10.1074/jbc.M704731200
Schall, T. J., Bacon, K., Toy, K. J., and Goeddel, D. V. (1990). Selective attraction of monocytes and T lymphocytes of the memory phenotype by cytokine RANTES. Nature 347, 669–671.
Smith, C. E., Wazen, R., Hu, Y., Zalzal, S. F., Nanci, A., Simmer, J. P., et al. (2009). Consequences for enamel development and mineralization resulting from loss of function of ameloblastin or enamelin. Eur. J. Oral Sci. 117, 485–497. doi: 10.1111/j.1600-0722.2009.00666.x
Sonoda, A., Iwamoto, T., Nakamura, T., Fukumoto, E., Yoshizaki, K., Yamada, A., et al. (2009). Critical role of heparin binding domains of ameloblastin for dental epithelium cell adhesion and ameloblastoma proliferation. J. Biol. Chem. 284, 27176–27184. doi: 10.1074/jbc.M109.033464
Spahr, A., Lyngstadaas, S. P., Slaby, I., and Pezeshki, G. (2006). Ameloblastin expression during craniofacial bone formation in rats. Eur. J. Oral Sci. 114, 504–511. doi: 10.1111/j.1600-0722.2006.00403.x
Stout, B. M., Alent, B. J., Pedalino, P., Holbrook, R., Gluhak-Heinrich, J., Cui, Y., et al. (2014). Enamel matrix derivative: protein components and osteoinductive properties. J. Periodontol. 85, e9–e17. doi: 10.1902/jop.2013.130264
Takahashi, K., Shimonishi, M., Wang, R., Watanabe, H., and Kikuchi, M. (2012). Epithelial-mesenchymal interactions induce enamel matrix proteins and proteases in the epithelial cells of the rests of Malassez in vitro. Eur. J. Oral Sci. 120, 475–483. doi: 10.1111/j.1600-0722.2012.01002.x
Tamburstuen, M. V., Reppe, S., Spahr, A., Sabetrasekh, R., Kvalheim, G., Slaby, I., et al. (2010). Ameloblastin promotes bone growth by enhancing proliferation of progenitor cells and by stimulating immunoregulators. Eur. J. Oral Sci. 118, 451–459. doi: 10.1111/j.1600-0722.2010.00760.x
Tamburstuen, M. V., Reseland, J. E., Spahr, A., Brookes, S. J., Kvalheim, G., Slaby, I., et al. (2011). Ameloblastin expression and putative autoregulation in mesenchymal cells suggest a role in early bone formation and repair. Bone 48, 406–413. doi: 10.1016/j.bone.2010.09.007
Toyosawa, S., Fujiwara, T., Ooshima, T., Shintani, S., Sato, A., Ogawa, Y., et al. (2000). Cloning and characterization of the human ameloblastin gene. Gene 256, 1–11. doi: 10.1016/S0378-1119(00)00379-6
Uchida, T., Murakami, C., Dohi, N., Wakida, K., Satoda, T., and Takahashi, O. (1997). Synthesis, secretion, degradation, and fate of ameloblastin during the matrix formation stage of the rat incisor as shown by immunocytochemistry and immunochemistry using region-specific antibodies. J. Histochem. Cytochem. 45, 1329–1340. doi: 10.1177/002215549704501002
Vymetal, J., Slaby, I., Spahr, A., Vondrasek, J., and Lyngstadaas, S. P. (2008). Bioinformatic analysis and molecular modelling of human ameloblastin suggest a two-domain intrinsically unstructured calcium-binding protein. Eur. J. Oral Sci. 116, 124–134. doi: 10.1111/j.1600-0722.2008.00526.x
Wald, T., Osickova, A., Sulc, M., Benada, O., Semeradtova, A., Rezabkova, L., et al. (2013). Intrinsically disordered enamel matrix protein ameloblastin forms ribbon-like supramolecular structures via an N-terminal segment encoded by exon 5. J. Biol. Chem. 288, 22333–22345. doi: 10.1074/jbc.M113.456012
Watanabe, T., Kukita, T., Kukita, A., Wada, N., Toh, K., Nagata, K., et al. (2004). Direct stimulation of osteoclastogenesis by MIP-1alpha: evidence obtained from studies using RAW264 cell clone highly responsive to RANKL. J. Endocrinol. 180, 193–201. doi: 10.1677/joe.0.1800193
Wazen, R. M., Moffatt, P., Zalzal, S. F., Yamada, Y., and Nanci, A. (2009). A mouse model expressing a truncated form of ameloblastin exhibits dental and junctional epithelium defects. Matrix Biol. 28, 292–303. doi: 10.1016/j.matbio.2009.04.004
Keywords: ameloblastin, biomineralization, bone growth, exon 5, human mesenchymal stem cells, osteogenesis, proliferation
Citation: Stakkestad Ø, Lyngstadaas SP, Vondrasek J, Gordeladze JO and Reseland JE (2017) Ameloblastin Peptides Modulates the Osteogenic Capacity of Human Mesenchymal Stem Cells. Front. Physiol. 8:58. doi: 10.3389/fphys.2017.00058
Received: 09 November 2016; Accepted: 23 January 2017;
Published: 07 February 2017.
Edited by:
Ariane Berdal, Inserm and University Paris-Diderot, FranceReviewed by:
Anne Poliard, Paris Descartes University, FranceAna Angelova Volponi, King's College London, UK
Copyright © 2017 Stakkestad, Lyngstadaas, Vondrasek, Gordeladze and Reseland. This is an open-access article distributed under the terms of the Creative Commons Attribution License (CC BY). The use, distribution or reproduction in other forums is permitted, provided the original author(s) or licensor are credited and that the original publication in this journal is cited, in accordance with accepted academic practice. No use, distribution or reproduction is permitted which does not comply with these terms.
*Correspondence: Janne Elin Reseland, ai5lLnJlc2VsYW5kQG9kb250LnVpby5ubw==