- 1Center for Human Movement Sciences, University Medical Center, Groningen, Netherlands
- 2Human Performance Lab, Schulthess Clinic, Zurich, Switzerland
- 3Institut NeuroMyoGène, Université Claude Bernard Lyon 1, INSERM U1217, CNRS UMR 5310, Villeurbanne, France
- 4Institute of Sport Sciences, University of Lausanne, Lausanne, Switzerland
- 5Department of Physiology, Faculty of Biology and Medicine, University of Lausanne, Lausanne, Switzerland
Introduction
Various electrical stimulation modalities are used as adjuvants to conventional training and rehabilitation programs to increase bodily function or to reduce symptoms, such as pain. One of these modalities, neuromuscular electrical stimulation (NMES), commonly refers to the transcutaneous application of electrical currents to a target muscle group with the objective to depolarize motor neurons and consequently elicit skeletal muscle contractions of substantial intensity (usually ranging from 10 to 60% of the maximal voluntary contraction). Because NMES can generate considerable muscle tension, it is frequently used as a strength training technique for healthy adults and athletes, but also as a rehabilitation tool to increase or preserve muscle function and mass in individuals with muscle weakness or patients who cannot perform voluntary contractions [e.g., patients suffering from chronic heart failure (CHF), chronic obstructive pulmonary disease (COPD), or critical illness; for reviews, see Roig and Reid, 2009; Sillen et al., 2009; Sbruzzi et al., 2010; Maddocks et al., 2011; Maffiuletti et al., 2011; Smart et al., 2013; Burke et al., 2016]. Under certain conditions, NMES training may also improve muscle oxidative capacity and result in a fast-to-slow muscle fiber type transition (Pérez et al., 2002; Gondin et al., 2011a), which could potentially enhance endurance performance. However, the relevance of such adaptations in skeletal muscle tissue for the translation to functional performance that is particularly important for sport and daily activities is not always self-evident, mainly because of the heterogeneity in study populations, NMES parameters, and outcome measures. Unfortunately, the bodies of literature that either focus on mechanistic (i.e., muscle endurance) or clinical (i.e., functional endurance) outcomes are often too disconnected. In this opinion paper, we aim to bring these bodies of literature together and discuss the impact of high- vs. low-frequency NMES training on muscle vs. functional endurance in healthy vs. clinical populations. As such, we focus on human studies that chronically applied NMES for at least 3 weeks in healthy persons and patients, and distinguish between the effectiveness of non-tetanic low-frequency NMES (that is usually administered continuously at frequencies close to 10 Hz) and tetanic high-frequency NMES (that is usually administered intermittently at frequencies close to 50 Hz) on muscle endurance and functional endurance. For clarity purposes, we refer to muscle endurance as the exercise-induced decline in voluntary or electrically-evoked force (Duchateau and Hainaut, 1988; Gondin et al., 2006) or the endurance time of a sustained single-joint contraction (Gondin et al., 2006). In contrast, we refer to functional endurance as the maximal oxygen consumption or workload (Pérez et al., 2002; Porcelli et al., 2012), the distance covered in a given time (e.g., 6-minute walk test) or the endurance time (Kim et al., 1995) for whole-body exercises such as walking and cycling. In the last section, we will provide some recommendations for better clinical use of NMES, and suggest potential directions for future research.
Effects of NMES Training on Endurance Performance in Healthy Subjects
High-frequency NMES training enhances muscle strength (for a review, see Gondin et al., 2011b), but may not affect (Duchateau and Hainaut, 1988) or even decrease muscle endurance, as demonstrated for example by a reduced ability to sustain a submaximal contraction (Gondin et al., 2006). High-frequency NMES training also appears to have a negligible influence on functional endurance, as illustrated for example by maximal oxygen consumption results (Figure 1A) (Pérez et al., 2002; Porcelli et al., 2012). These limited effects of high-frequency NMES training on endurance performance are somewhat surprising for different reasons. First, a single session of high-frequency NMES induces an exaggerated metabolic and cardiovascular stress when compared to torque-matched voluntary contractions (McNeil et al., 2006; Theurel et al., 2007) and high levels of muscle fatigue, mainly due to the motor unit recruitment pattern of NMES that is considerably different from voluntary contractions (for a review, see Bickel et al., 2011). Second, high-frequency NMES results in a fast-to-slow shift in fiber type distribution together with increased oxidative capacity and capillarization of the stimulated muscles (Pérez et al., 2002; Gondin et al., 2011a), i.e., adaptations that are characteristic of endurance training. However, methodological limitations pertaining to the definition and assessment of muscle or functional endurance complicate the interpretation of the data. Also, the evaluation of endurance for commonly-used muscles such as the quadriceps in normally functioning people may not be optimal as it likely suffers from ceiling effects. This suggestion is confirmed by the observation that high-frequency NMES training of abdominal muscles in healthy individuals resulted in substantial increases in abdominal strength and endurance time (Alon et al., 1987).
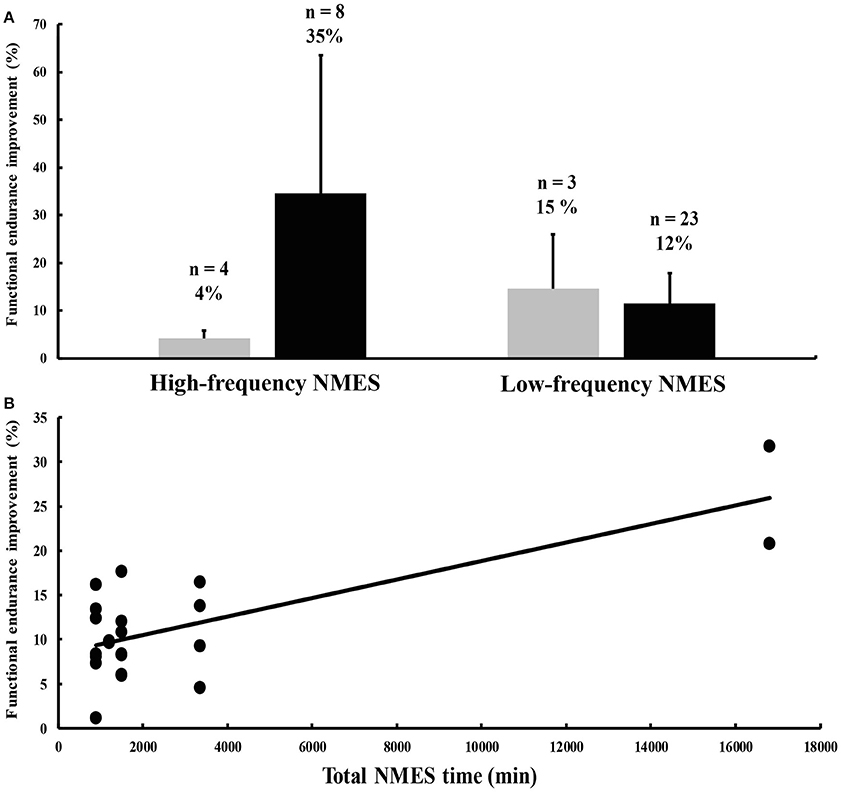
Figure 1. (A) Percent improvements in functional endurance after high- and low-frequency neuromuscular electrical stimulation (NMES) interventions in healthy subjects (gray histograms) and patient populations (black histograms). The numbers above each histogram represent the number of outcome measures the mean change was computed from, and the mean percent change of these outcome measures. Most of the data, which are not stratified by condition and type of outcome measure, were extracted from Sillen et al. (2009). Vertical bars denote one standard deviation. (B) Relationship between the total duration of NMES treatment on the x-axis and NMES training-induced percent improvements in functional endurance in patients suffering from chronic heart failure (CHF) on the y-axis (anecdotally, the R2 is 0.54). Data were obtained from eight different studies published between 2003 and 2006 (Harris et al., 2003; Nuhr et al., 2003; Eicher et al., 2004; Banerjee et al., 2005; Deley et al., 2005; Dobsák et al., 2006; Karavidas et al., 2006; LeMaitre et al., 2006).
Data on the effects of low-frequency NMES training on muscle and functional endurance in healthy participants are relatively scarce. Three studies show improvements in functional endurance following low-frequency NMES training (e.g., improved work capacity and oxygen consumption at the anaerobic threshold), possibly mediated by adaptations in aerobic-oxidative metabolism and increased capillarization (Thériault et al., 1996; Nuhr et al., 2003; Miyamoto et al., 2016). Notwithstanding the limited data, the effects of low-frequency NMES training on functional endurance appear in general superior to those induced by high-frequency NMES training, despite the apparently similar fast-to-slow transition in fiber type distribution induced by the two NMES modalities (Thériault et al., 1996; Nuhr et al., 2003). Such divergent effectiveness of high- vs. low-frequency NMES training on endurance performance is likely caused by methodological differences between the two modalities. High-frequency NMES is usually applied intermittently and with higher current intensities in comparison with low-frequency NMES. Because of better current tolerance, low-frequency NMES sessions are generally considerably longer and can reach up to 240 min of continuous stimulation per day (e.g., Nuhr et al., 2003), vs. 20–30 min of intermittent high-frequency NMES per day (Gondin et al., 2011a). Such differences may have contributed to the greater increases in functional endurance observed after low- vs. high-frequency NMES training and strongly suggest that the long duration of low-frequency NMES sessions may be the most important parameter for increasing functional endurance.
In summary, little is known about the impact of NMES training on muscle and functional endurance in a healthy population. High-frequency NMES training has no influence or may even have a negative impact on muscle endurance while for functional endurance, low-frequency NMES training appears favorable, possibly because of the very long treatment sessions. Studies in healthy individuals that often targeted the quadriceps muscle may have suffered from ceiling effects. We speculate that NMES interventions on less-used muscle groups and with stronger study designs (large sample size, homogeneous subject characteristics, sham condition) will provide more insights into the real effectiveness of NMES training.
Effects of NMES Training on Endurance Performance in Patient Populations
The suggestion that less-used muscle groups are more prone to improvement is confirmed by findings obtained in patients with pathologies affecting muscle, pulmonary, and cardiovascular function. In general, NMES training protocols are more effective in patients compared to healthy individuals, at least for functional endurance (Figure 1A). In various patient groups such as COPD and CHF, NMES training improved muscle strength and respiratory function, and consequently, functional endurance as reflected by increased oxygen uptake and workload, longer endurance times and farther movement distances, often indexed by the 6-minute walk test (for reviews, see Roig and Reid, 2009; Sillen et al., 2009; Sbruzzi et al., 2010; Maddocks et al., 2011; Smart et al., 2013; Burke et al., 2016). In contrast, there are few data on adaptations in muscle endurance after high- or low-frequency NMES training in patient populations. To the best of our knowledge, only three studies reported improvements in muscle endurance in different patient groups following NMES interventions (Quittan et al., 2001; Doucet and Griffin, 2013; Erickson et al., 2016). Clinical trials that applied high- and low-frequency NMES training focused mostly on functional endurance because of its clinical relevance (Vaquero et al., 1998; Bourjeily-Habr et al., 2002; Neder et al., 2002; Harris et al., 2003; Nuhr et al., 2003; Eicher et al., 2004; Banerjee et al., 2005; Deley et al., 2005; Dobsák et al., 2006; Karavidas et al., 2006; LeMaitre et al., 2006; Vivodtzev et al., 2006, 2012; Dal Corso et al., 2007).
Figure 1A, which contains data from the 14 aforementioned clinical trials, shows more favorable effects of high-frequency over low-frequency NMES training on functional endurance. This non-comprehensive analysis is confounded by the disparate definitions of functional endurance, the absence of stratification for condition, and the heterogeneity in NMES parameters. For example, for some unknown reasons, five studies in patients suffering from COPD used exclusively high-frequency NMES and showed greater increases in functional endurance (43%) compared to nine studies conducted in CHF patients that were administered almost exclusively low-frequency NMES (12%). Nevertheless, the rate of improvement may also depend on the severity of the disease. For example, patients with severe CHF improved more (26%; Nuhr et al., 2003) compared to stable patients (10%; e.g., Deley et al., 2005) following a low-frequency NMES training program. More importantly, the total duration of the NMES treatment (i.e., the training volume) from eight different studies conducted on CHF patients seems to be positively related to the magnitude of the improvement in endurance performance (Figure 1B).
In summary, both high- and low-frequency NMES training can increase functional endurance in patient populations, while their influence on muscle endurance is largely unknown (probably because of the poor clinical relevance of this latter variable). The impact of NMES training protocols on endurance performance appears highly dependent on the type of disease, its severity, and the total exposure to the treatment.
Conclusions
High- and low-frequency NMES training can increase functional endurance, with the magnitude of the effects being dependent on the initial condition. That is, inactive patients with advanced disease are more likely to benefit from NMES training than more active patients with stable symptoms and healthy individuals. The most likely explanation for this observation is that the quadriceps muscle, mostly targeted by NMES, is highly involved in several activities of daily living and is therefore less sensitive to improvements in active individuals. We therefore argue that NMES training is particularly useful for patients that are unable or unwilling to participate in daily activities or in regular physical exercise. Despite the heterogeneity of the studies in terms of study populations, NMES parameters, and outcome measures, we provide recommendations for clinical use and present ideas to increase treatment effectiveness. Although recent comparison studies did not show differences in the acute responses between high- and low-frequency NMES in both CHF (Sbruzzi et al., 2010) and COPD patients (Sillen et al., 2011), low-frequency NMES training seems to be particularly effective for patients with CHF, with longer treatment durations causing larger increases in functional endurance (Figure 1B), while for COPD patients, it is difficult to provide specific recommendations concerning high- vs. low-frequency NMES due to limited data.
Although the literature has provided us with many mechanistic and clinical insights into NMES training-induced effects on muscle and functional endurance, the trials conducted so far have some methodological limitations and need to be improved. First, there is a clear distinction between the trials in healthy participants and those in patient populations. While studies in healthy individuals focus on mechanistic parameters such as fiber type composition and capillarization and have few outcome measures that are clinically relevant, studies in patient populations merely focus on functional/clinical outcomes. While such distinctions are understandable from a patient-burden point of view, the mechanisms underlying NMES-induced adaptations may differ between diseased and healthy individuals. Therefore, randomized controlled trials are needed that compare high- and low-frequency NMES training programs for both lower and upper extremity muscle groups in patient populations vs. age- and gender-matched healthy controls. In addition, because the clinical applicability also depends on whether NMES-induced effects can still be observed after several months, follow-up measures should be included in future trials.
In conclusion, we propose here that both high- and low-frequency NMES training (and probably a combination of the two, depending on clinicians' needs) are potentially relevant to improve endurance performance, and that although their physiological effects are relatively well understood in healthy subjects, more evidence-based research is required to optimize NMES treatment protocols for various patient populations.
Author Contributions
All authors listed, have made substantial, direct and intellectual contribution to the work, and approved it for publication.
Conflict of Interest Statement
The authors declare that the research was conducted in the absence of any commercial or financial relationships that could be construed as a potential conflict of interest.
References
Alon, G., McCombe, S. A., Koutsantonis, S., Stumphauzer, L. J., Burgwin, K. C., Parent, M. M., et al. (1987). Comparison of the effects of electrical stimulation and exercise on abdominal musculature. J. Orthop. Sport. Phys. Ther. 8, 567–573. doi: 10.2519/jospt.1987.8.12.567
Banerjee, P., Caulfield, B., Crowe, L., and Clark, A. (2005). Prolonged electrical muscle stimulation exercise improves strength and aerobic capacity in healthy sedentary adults. J. Appl. Physiol. 99, 2307–2311. doi: 10.1152/japplphysiol.00891.2004
Bickel, C. S., Gregory, C. M., and Dean, J. C. (2011). Motor unit recruitment during neuromuscular electrical stimulation: a critical appraisal. Eur. J. Appl. Physiol. 111, 2399–2407. doi: 10.1007/s00421-011-2128-4
Bourjeily-Habr, G., Rochester, C. L., Palermo, F., Snyder, P., and Mohsenin, V. (2002). Randomised controlled trial of transcutaneous electrical muscle stimulation of the lower extremities in patients with chronic obstructive pulmonary disease. Thorax 57, 1045–1049. doi: 10.1136/thorax.57.12.1045
Burke, D., Gorman, E., Stokes, D., and Lennon, O. (2016). An evaluation of neuromuscular electrical stimulation in critical care using the ICF framework: a systematic review and meta-analysis. Clin. Respir. J. 10, 407–420. doi: 10.1111/crj.12234
Dal Corso, S., Nápolis, L., Malaguti, C., Gimenes, A. C., Albuquerque, A., Nogueira, C. R., et al. (2007). Skeletal muscle structure and function in response to electrical stimulation in moderately impaired COPD patients. Respir. Med. 101, 1236–1243. doi: 10.1016/j.rmed.2006.10.023
Deley, G., Kervio, G., Verges, B., Hannequin, A., Petitdant, M.-F., Salmi-Belmihoub, S., et al. (2005). Comparison of low-frequency electrical myostimulation and conventional aerobic exercise training in patients with chronic heart failure. Eur. J. Cardiovasc. Prev. Rehabil. 12, 226–233. doi: 10.1097/00149831-200506000-00007
Dobsák, P., Nováková, M., Fiser, B., Siegelová, J., Balcárková, P., Spinarová, L., et al. (2006). Electrical stimulation of skeletal muscles: an alternative to aerobic exercise training in patients with chronic heart failure? Int. Heart J. 47, 441–453. doi: 10.1536/ihj.47.441
Doucet, B. M., and Griffin, L. (2013). High-versus low-frequency stimulation effects on fine motor control in chronic hemiplegia: a Pilot Study. Top. Stroke Rehabil. 20, 299–307. doi: 10.1310/tsr2004-299
Duchateau, J., and Hainaut, K. (1988). Training effects of sub-maximal electrostimulation in a human muscle. Med. Sci. Sport. Exerc. 20, 99–104. doi: 10.1249/00005768-198802000-00015
Eicher, J. C., Dobsak, P., Berteau, O., Walker, P., Verges, B., Maillefert, J. F., et al. (2004). Rehabilitation in chronic congestive heart failure: comparison of bicycle training and muscle electrical stimulation. Scr. Medica Fac. Medicae Univ. Brun. Masaryk. 77, 261–270. Available online at: http://www.med.muni.cz/biomedjournal/pdf/2004/05/261-270.pdf
Erickson, M. L., Ryan, T. E., Backus, D., and McCully, K. K. (2016). Endurance neuromuscular electrical stimulation training improves skeletal muscle oxidative capacity in individuals with motor-complete spinal cord injury. Muscle Nerve. doi: 10.1002/mus.25393. [Epub ahead of print].
Gondin, J., Brocca, L., Bellinzona, E., D'Antona, G., Maffiuletti, N. A., Miotti, D., et al. (2011a). Neuromuscular electrical stimulation training induces atypical adaptations of the human skeletal muscle phenotype: a functional and proteomic analysis. J. Appl. Physiol. 110, 433–450. doi: 10.1152/japplphysiol.00914.2010
Gondin, J., Cozzone, P. J., and Bendahan, D. (2011b). Is high-frequency neuromuscular electrical stimulation a suitable tool for muscle performance improvement in both healthy humans and athletes? Eur. J. Appl. Physiol. 111, 2473–2487. doi: 10.1007/s00421-011-2101-2
Gondin, J., Guette, M., Jubeau, M., Ballay, Y., and Martin, A. (2006). Central and peripheral contributions to fatigue after electrostimulation training. Med. Sci. Sports Exerc. 38, 1147–1156. doi: 10.1249/01.mss.0000222843.04510.ca
Harris, S., LeMaitre, J. P., Mackenzie, G., Fox, K. A. A., and Denvir, M. A. (2003). A randomised study of home-based electrical stimulation of the legs and conventional bicycle exercise training for patients with chronic heart failure. Eur. Heart J. 24, 871–878. doi: 10.1016/S0195-668X(02)00822-9
Karavidas, A. I., Raisakis, K. G., Parissis, J. T., Tsekoura, D. K., Adamopoulos, S., Korres, D. A., et al. (2006). Functional electrical stimulation improves endothelial function and reduces peripheral immune responses in patients with chronic heart failure. Eur. J. Cardiovasc. Prev. Rehabil. 13, 592–597. doi: 10.1097/01.hjr.0000219111.02544.ff
Kim, C. K., Takala, T. E. S., Seger, J., and Karpakka, J. (1995). Training effects of electrically induced dynamic contractions in human quadriceps muscle. Aviat. Sp. Environ. Med. 66, 251–255.
LeMaitre, J. P., Harris, S., Hannan, J., Fox, K. A. A., and Denvir, M. A. (2006). Maximum oxygen uptake corrected for skeletal muscle mass accurately predicts functional improvements following exercise training in chronic heart failure. Eur. J. Heart Fail. 8, 243–248. doi: 10.1016/j.ejheart.2005.07.011
Maddocks, M., Gao, W., Higginson, I. J., and Wilcock, A. (2011). Neuromuscular electrical stimulation for muscle weakness in adults with advanced disease. Cochrane Database Syst. Rev. 31:CD009419. doi: 10.1002/14651858.CD009419
Maffiuletti, N. A., Minetto, M. A., Farina, D., and Bottinelli, R. (2011). Electrical stimulation for neuromuscular testing and training: state-of-the art and unresolved issues. Eur. J. Appl. Physiol. 111, 2391–2397. doi: 10.1007/s00421-011-2133-7
McNeil, C. J., Murray, B. J., and Rice, C. L. (2006). Differential changes in muscle oxygenation between voluntary and stimulated isometric fatigue of human dorsiflexors. J. Appl. Physiol. 100, 890–895. doi: 10.1152/japplphysiol.00921.2005
Miyamoto, T., Kamada, H., Tamaki, A., and Moritani, T. (2016). Low-intensity electrical muscle stimulation induces significant increases in muscle strength and cardiorespiratory fitness. Eur. J. Sport Sci. 16, 1104–1110. doi: 10.1080/17461391.2016.1151944
Neder, J. A., Sword, D., Ward, S. A., Mackay, E., Cochrane, L. M., and Clark, C. J. (2002). Home based neuromuscular electrical stimulation as a new rehabilitative strategy for severely disabled patients with chronic obstructive pulmonary disease (COPD). Thorax 57, 333–337. doi: 10.1136/thorax.57.4.333
Nuhr, M., Crevenna, R., Gohlsch, B., Bittner, C., Pleiner, J., Wiesinger, G., et al. (2003). Functional and biochemical properties of chronically stimulated human skeletal muscle. Eur. J. Appl. Physiol. 89, 202–208. doi: 10.1007/s00421-003-0792-8
Pérez, M., Lucia, A., Rivero, J. L. L., Serrano, A. L., Calbet, J. A. L., Delgado, M. A., et al. (2002). Effects of transcutaneous short-term electrical stimulation on M. vastus lateralis characteristics of healthy young men. Pflugers Arch. Eur. J. Physiol. 443, 866–874. doi: 10.1007/s00424-001-0769-6
Porcelli, S., Marzorati, M., Pugliese, L., Adamo, S., Gondin, J., Bottinelli, R., et al. (2012). Lack of functional effects of neuromuscular electrical stimulation on skeletal muscle oxidative metabolism in healthy humans. J. Appl. Physiol. 113, 1101–1109. doi: 10.1152/japplphysiol.01627.2011
Quittan, M., Wiesinger, G. F., Sturm, B., Puig, S., Mayr, W., Sochor, A., et al. (2001). Improvement of thigh muscles by neuromuscular electrical stimulation in patients with refractory heart failure: a single-blind, randomized, controlled trial. Am. J. Phys. Med. Rehabil. 80, 206–214. quiz: 215–216, 224. doi: 10.1097/00002060-200103000-00011
Roig, M., and Reid, W. D. (2009). Electrical stimulation and peripheral muscle function in COPD: a systematic review. Respir. Med. 103, 485–495. doi: 10.1016/j.rmed.2008.11.008
Sbruzzi, G., Ribeiro, R. A., Schaan, B. D., Signori, L. U., Silva, A. M. V., Irigoyen, M. C., et al. (2010). Functional electrical stimulation in the treatment of patients with chronic heart failure: a meta-analysis of randomized controlled trials. Eur. J. Cardiovasc. Prev. Rehabil. 17, 254–260. doi: 10.1097/hjr.0b013e328339b5a2
Sillen, M. J. H., Speksnijder, C. M., Eterman, R. M. A., Janssen, P. P., Wagers, S. S., Wouters, E. F. M., et al. (2009). Effects of neuromuscular electrical stimulation of muscles of ambulation in patients with chronic heart failure or COPD: a systematic review of the english-language literature. Chest 136, 44–61. doi: 10.1378/chest.08-2481
Sillen, M. J. H., Wouters, E. F. M., Franssen, F. M. E., Meijer, K., Stakenborg, K. H. P., and Spruit, M. A. (2011). Oxygen uptake, ventilation, and symptoms during low-frequency versus high-frequency NMES in COPD: a pilot study. Lung 189, 21–26. doi: 10.1007/s00408-010-9265-0
Smart, N. A., Dieberg, G., and Giallauria, F. (2013). Functional electrical stimulation for chronic heart failure: a meta-analysis. Int. J. Cardiol. 167, 80–86. doi: 10.1016/j.ijcard.2011.12.019
Thériault R., Boulay M. R., Thériault G., and Simoneau J. A. (1996). Electrical stimulation-induced changes in performance and fiber type proportion of human knee extensor muscles. Eur. J. Appl. Physiol. Occup. Physiol. 74, 311–317. doi: 10.1007/BF02226926
Theurel, J., Lepers, R., Pardon, L., and Maffiuletti, N. A. (2007). Differences in cardiorespiratory and neuromuscular responses between voluntary and stimulated contractions of the quadriceps femoris muscle. Respir. Physiol. Neurobiol. 157, 341–347. doi: 10.1016/j.resp.2006.12.002
Vaquero, A. F., Chicharro, J. L., Gil, L., Ruiz, M. P., Sánchez, V., Lucia, A., et al. (1998). Effects of muscle electrical stimulation on peak VO2 in cardiac transplant patients. Int. J. Sports Med. 19, 317–322. doi: 10.1055/s-2007-971924
Vivodtzev, I., Debigaré, R., Gagnon, P., Mainguy, V., Saey, D., Dubé, A., et al. (2012). Functional and muscular effects of neuromuscular electrical stimulation in patients with severe COPD: a randomized clinical trial. Chest 141, 716–725. doi: 10.1378/chest.11-0839
Keywords: neuromuscular electrical stimulation, skeletal muscle, muscle endurance, functional endurance, rehabilitation
Citation: Veldman MP, Gondin J, Place N and Maffiuletti NA (2016) Effects of Neuromuscular Electrical Stimulation Training on Endurance Performance. Front. Physiol. 7:544. doi: 10.3389/fphys.2016.00544
Received: 14 October 2016; Accepted: 28 October 2016;
Published: 16 November 2016.
Edited by:
Benjamin Pageaux, Université de Bourgogne, FranceReviewed by:
Roger Enoka, University of Colorado, USACopyright © 2016 Veldman, Gondin, Place and Maffiuletti. This is an open-access article distributed under the terms of the Creative Commons Attribution License (CC BY). The use, distribution or reproduction in other forums is permitted, provided the original author(s) or licensor are credited and that the original publication in this journal is cited, in accordance with accepted academic practice. No use, distribution or reproduction is permitted which does not comply with these terms.
*Correspondence: Nicola A. Maffiuletti, bmljb2xhLm1hZmZpdWxldHRpQGt3cy5jaA==