- 1Department of Biology, City College of New York, New York, NY, USA
- 2Department of Clinical Oncology, Queen Elizabeth Hospital, Kowloon, Hong Kong
The liver is the center for drug and xenobiotic metabolism, which is influenced most with medication/xenobiotic-mediated toxic activity. Drug-induced hepatotoxicity is common and its actual frequency is hard to determine due to underreporting, difficulties in detection or diagnosis, and incomplete observation of exposure. The death rate is high, up to about 10% for drug-induced liver damage. Endorsed medications represented >50% of instances of intense liver failure in a study from the Acute Liver Failure Study Group of the patients admitted in 17 US healing facilities. Albeit different studies are accessible uncovering the mechanistic aspects of medication prompted hepatotoxicity, we are in the dilemma about the virtual story. The expanding prevalence and effectiveness of Ayurveda and natural products in the treatment of various disorders led the investigators to look into their potential in countering drug-induced liver toxicity. Several natural products have been reported to date to mitigate the drug-induced toxicity. The dietary nature and less adverse reactions of the natural products provide them an extra edge over other candidates of supplementary medication. In this paper, we have discussed the mechanism involved in drug-induced liver toxicity and the potential of herbal antioxidants as supplementary medication.
Introduction
The leading cause of drug non-approval and drug withdrawal by the Food and Drug Administration (FDA) in the US is drug-induced hepatotoxicity (Ostapowicz et al., 2002; Pandit et al., 2012). More than a thousand medicines and chemicals have been reported to cause liver injury (Larrey, 2000; Biour et al., 2004; Upadhyay et al., 2010b; Porceddu et al., 2012). Drug-induced liver injury may account for approximately 10% of all cases of acute hepatitis, 5% of all hospital admissions, and 50% of all acute liver failures (Pandit et al., 2012). It is remarkable that more than 75% of cases of idiosyncratic drug reactions result in liver transplantation or death (Ostapowicz et al., 2002; Pandit et al., 2012). Drug-induced liver injury is a relatively common cause of acute liver disease and carries a mortality of around 10% (Lewis and Zimmerman, 1989; Shapiro and Lewis, 2007; Chalasani et al., 2008; Bell and Chalasani, 2009; Holt and Ju, 2010; Upadhyay et al., 2010b; Pandit et al., 2012; Björnsson et al., 2013). Inefficient drug metabolism, as is observed in the renal transplant (RT) recipients with the chronic liver disease, make them more prone to drug-induced hepatotoxicity (Contreras et al., 2007). Extensive research on drug-induced hepatic cell damage worldwide has been done in the past and is also an area of major concern at present since hepatotoxicity due to these drugs, when it becomes terminal, results in malnutrition, organ dysfunction, and death (Björnsson, 2009; Porceddu et al., 2012).
The liver plays a major role in the metabolism and removal of drugs (Pandit et al., 2012). Detoxification of drugs and xenobiotics in the liver by drug metabolizing enzymes (DMEs) is an important phenomenon in the acquisition of homeostasis (Upadhyay et al., 2007, 2008). Alteration in homeostatic status leads to a shift in the dynamic equilibrium of metabolism toward the ROS generation thereby oxidative stress leading to organ malfunction. Liver insufficiency and damage resulted from exposure to environmental toxicants, particular combinations or dosages of pharmaceuticals, and microbial metabolites are major causes of disease and death worldwide (Upadhyay et al., 2007, 2008). Intake of drugs, their metabolism and removal make a condition of dynamic equilibrium to maintain homeostasis. Phase I and phase II enzymes play a crucial role in the metabolism and detoxification of various drugs and xenobiotics. For the acquisition of dynamic homeostasis, highly tuned metabolic control of drugs or xenobiotics by xenobiotic metabolizing enzymes is needed. Any imbalance in the activity of these enzymes ultimately leads to the shifting of equilibrium toward free radical generation that could finally bind to macromolecules such as DNA to cause mutation, lipid to cause membrane damage, or proteins to alter their activities (Upadhyay et al., 2007, 2008, 2010a,b). The generation of reactive intermediates is a common event in liver damage resulting from a variety of hepatotoxic drugs and solvents (Coleman et al., 2007). The hepatotoxic reactions caused by drugs may be summarized as acute reactions (which consist of hepatocellular necrosis), cholestasis (with or without inflammation), and miscellaneous reactions; however, some drugs can cause chronic damage and may even lead to tumor growth (Table 1). In this article, we have discussed the drug-induced hepatotoxicity, factors that may add to its toxicity, mechanism, and prevention.
Factors Affecting Drug-Induced Hepatotoxicity
There are various factors that enhance a person's susceptibility to a potentially hepatotoxic drug (Figure 1). Advancing age, gender, lifestyle factors, obesity, nutritional status, genetic background, dose, and duration of drugs may affect the risk of drug-mediated hepatotoxic reactions. Persons suffering from other diseases, such as, human immunodeficiency virus, hepatitis C, rheumatoid arthritis, and systemic lupus erythematosus, are more prone to toxic drug reactions. Drug composition and drug-drug interaction may also be a reason for increased risk of drug-induced hepatotoxicity. For example, certain drugs containing a nitro-aromatic moiety or drugs interacting with nuclear receptors such as phenobarbital may cause organ-selective toxicity or may potentiate the toxicity of other drugs (Yamazaki et al., 2005; Boelsterli et al., 2006).
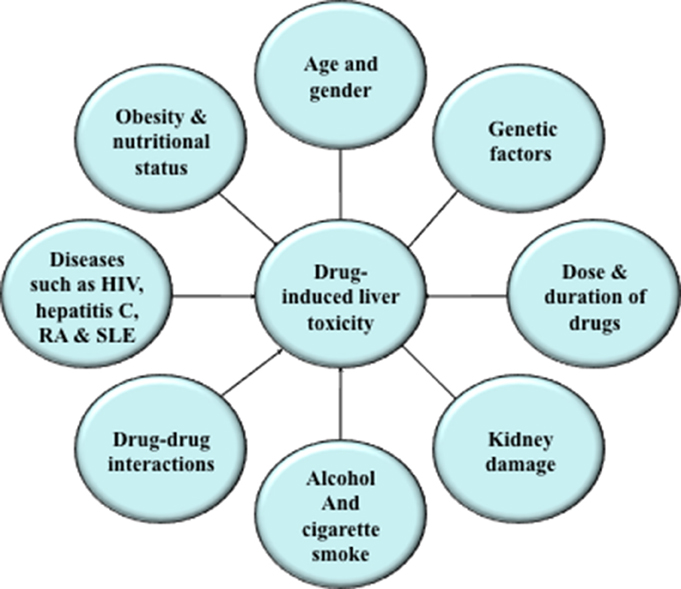
Figure 1. Factors affecting drug-induced hepatic toxicity. Various factors, such as, advancing age, gender, lifestyle factors, obesity, nutritional status, genetic background, dose, and duration of drugs may increase the risk of drug-mediated hepatotoxic reactions.
Drug-Induced Damage
A rising number of cases of acute liver failures are reported every year in the United States, and drugs contribute majority of them, e.g., acetaminophen and idiosyncratic reactions due to other medications (Pandit et al., 2012). Two to five percent of jaundice cases and over 10% cases of acute hepatitis are also contributed by drug-mediated side reactions (Pandit et al., 2012). Several drugs and chemicals have been withdrawn from the market worldwide due to their hepatotoxic reactions (Bakke et al., 1995; Shah, 1999; Lee, 2003; Mohapatra et al., 2005; Upadhyay et al., 2010a,b; Pandit et al., 2012), yet epidemiological studies are alarming and suggest that the drug trial process should be more rigorous. Some drugs have been extensively studied in humans and animals to elucidate the biochemical and molecular mechanism of drug-induced hepatotoxicity (Upadhyay et al., 2007, 2008, 2010a,b). Most of the drugs have a signature effect, and exhibit a pattern of liver injury. Nevertheless, certain drugs like rifampicin may produce all kind of symptoms associated with liver injury, ranging from cholestasis, hepatocellular injury, to even isolated hyper-bilirubinemia. Therefore, the knowledge of the most commonly used drugs/agents, such as, anti-tubercular drugs, acetaminophen, diclophenac, pyrogallol, statins and so forth, and a high index of suspicion are essential in diagnosis.
Rifampicin
Hepatitis has been reported to occur 0.46% of individuals undergoing anti-tubercular therapy and receiving anti-tubercular drugs. The rate of hepatotoxic reaction was much higher in Indian patients as compared to that reported from developed countries (Ramachandran, 1980; Alexander et al., 1982; Mindie and Gabriel, 2002). It is readily absorbed from the gastrointestinal tract (90%) and most of it is bound to plasma proteins in circulation. The involvement of oxidative stress in rifampicin-induced hepatotoxicity has been demonstrated previously in experimental rats (Sodhi et al., 1998). Rifampicin is a potent inducer of cytochrome P450 action and enhances the covalent binding of reactive metabolites of acetyl hydrazine to the macromolecules of hepatocytes leading to hepatic cell damage (Powell-Jackson et al., 1982; Sinha, 1987). Additionally, desacetylrifampicin, another reactive metabolite of rifampicin, also contributes to some of its adverse effects. It has been reported to modulate the membrane permeability and cause membrane damage (Rana et al., 2006). Its prolonged exposure significantly decreases glucose-6-phosphatase activity, which could be a reason for the increased level of lipid peroxidation (Koster and Slee, 1980; Saraswathy and Shyamala Devi, 2001). Anti-tubercular drugs increase intracellular calcium concentration leading to the induction of phospholipase A2 which degrade membrane phospholipids (Karthikeyan, 2005; Tasduq et al., 2005). Additionally, CYP2E1 activation and fatty acid accumulation in the liver either due to excessive supply of lipids to the liver or interference with lipid deposition, has been documented in the anti-tubercular drug-induced liver disorders (Anundi et al., 1993; Farombi et al., 1999; Upadhyay et al., 2007). Anti-tubercular drugs also induce hypercholesterolemia that might be due to increased uptake of LDL from the blood, by the tissues (Kissler et al., 2005; Santhosh et al., 2006).
Isoniazid
Isoniazid, an anti-tubercular drug, is used alone or in combination with other drugs to eliminate the active (growing) bacteria. Usually, the therapy with isoniazid is continued for a longer time (6–12 months) since the bacteria may exist in a resting state for a longer period of time. The studies have indicated severe and fatal hepatitis with isoniazid therapy (Huang et al., 2003; Saukkonen et al., 2006). The frequency of hepatotoxic reactions are higher in aged patients over 65 years. Additionally, daily alcohol consumption increases the risk of hepatitis. In the patients receiving isoniazid, the symptoms of hepatic damage appear late, usually after 3 months of treatment.
In the liver, isoniazid is metabolized primarily by N-acetyl transferase 2 (NAT-2) to acetyl-isoniazid, which subsequently is converted to mono-acetyl hydrazine (MAH) and non-toxic diacetyl hydrazine, as well as other minor metabolites (Huang et al., 2002; Saukkonen et al., 2006). Studies have revealed that reactive metabolites of MAH are toxic to tissues due to ROS generation (Mitchell et al., 1975; Saukkonen et al., 2006). Isoniazid inhibits glutathione biosynthesis, activities of antioxidant glutathione peroxidase and catalase activity in rats (Sodhi et al., 1996; Attri et al., 2001; Saukkonen et al., 2006). Furthermore, acetyl-hydrazine, a metabolite of isoniazid, causes damage to hepatic cells by covalently binding to liver macromolecules (Mitchell et al., 1975; Saukkonen et al., 2006). In an epidemiological study, it has been reported that homozygous CYP2E1 c1/c1 host gene polymorphism, which results in enhanced CYP2E1 activity, causes higher risk of hepatotoxicity in patients (Huang et al., 2003; Saukkonen et al., 2006).
Acetaminophen
Acetaminophen (APAP) is a commonly used analgesic and antipyretic, which is relatively safe at recommended therapeutic doses (Blazer and Wu, 2009). However, its associated hepatotoxicity is a major concern and the leading cause of drug-induced liver failure in many countries when used at high doses (Larson et al., 2005; Mitka, 2014; Wang et al., 2015). Acetaminophen has been extensively studied in order to understand the mechanism of drug-induced hepatotoxicity. Its hepatotoxicity pattern is different in various age groups for example the hepatotoxic incidences are less in neonates than in older children and adults. It has been suggested that in neonates, the oxidative enzyme activity is limited therefore the incidences are less common (Jacqz-Aigrain and Anderson, 2006). The acetaminophen-induced liver injury leads to a functional suppression of the immune system as dictated by the hindrance of a deferred hypersensitivity reaction to dinitrochlorobenzene (Masson et al., 2007). Subsequent studies with adrenalectomized mice, recommended a role of corticosterone in the exhaustion of lymphocytes taking after APAP-instigated liver damage (Masson et al., 2007). Acetaminophen is metabolized to N-acetyl-p-benzoquinone imine by CYP enzymes, which is subsequently detoxified by reduced glutathione (GSH) to a threshold concentration, after which GSH depletion occur leading to the covalent binding of the metabolite to the macromolecules (Reid et al., 2005; Wolf et al., 2007; Olaleye and Rocha, 2008; Saito et al., 2010; McGill et al., 2012). APAP overdose has been reported to induce massive necrosis in the liver in animal models. It is remarkable that only those hepatocytes undergo necrosis in which acetaminophen-protein adducts formation occur (Hinson et al., 2004; Saito et al., 2010). It is also suggested that the APAP toxicity propagates through nitric oxide (NO), which scavenges superoxide to produce peroxynitrite, thereby causing protein nitration (3-ntrotyrosine) and tissue injury (Jaeschke et al., 2002; Hinson et al., 2004). Three-Nitrotyrosine is usually detoxified by conjugation with reduced GSH. Thus, acetaminophen toxicity occurs with increased oxygen/nitrogen stress (Hinson et al., 2004).
Pyrogallol
Pyrogallol is an anti-psoriatic drug and has been reported to cause liver damage in mouse and rat models (Gupta et al., 2002; Upadhyay et al., 2007, 2008, 2010a,b). In the presence of metal ions under certain conditions in vitro, pyrogallol causes oxidative damage to macromolecules (Singh et al., 1994). Pro-oxidant action of pyrogallol is suggested to be the major cause of harmful effects such as mutagenesis, carcinogenesis and hepatotoxicity (Akagawa et al., 2003; Upadhyay et al., 2007). It is suggested that the auto-oxidation property of pyrogallol due to the attack by reactive oxygen species such as •OH, , and hydrogen peroxides contribute greatly to its pro-oxidant actions (Cao et al., 1997; Hayakawa et al., 1997; Mochizuki et al., 2002; Akagawa et al., 2003). Studies have now established the involvement of toxicant responsive genes for example CYP1A2, CYP2E1, glutathione-S-transferase, glutathione reductase, and glutathione peroxidase in pyrogallol-induced membrane damage and hepatotoxicity (Upadhyay et al., 2007, 2008, 2010a,b).
Diclofenac
Diclofenac-mediated liver toxicity is a prime example of idiosyncratic liver injury (Mitchell et al., 1973) and the increased hepatotoxicity markers have been noted in about 15% of patients receiving diclofenac regularly. Elderly females are more susceptible to diclofenac-induced liver injury. (Banks et al., 1995; Kaplowitz, 2001; Mitchell and Hilmer, 2010) Diclofenac predominantly exhibits hepatocellular pattern of liver injury; however, cholestatic pattern of liver injury and cases resembling autoimmune hepatitis have also been observed (Aithal, 2004). Metabolism of diclofenac by Cyp2C8/9 or by UDP-glucuronosyltransferase-2B7 results in unstable intermediate compounds that modify proteins covalently and increase the risk of hepatotoxicity. Additionally, covalent binding of reactive metabolites to “self” proteins results in the formation of neoantigens, which could be recognized by helper T cells leading to their activation and an effector-cell response. It is remarkable that hepatocytes express MHC I molecules on their surfaces and may present diclofenac adducts, making them prone for T-cell mediated liver injury. Alternatively, diclofenac adducts on the plasma membrane of hepatocytes may be recognized by B cells resulting in their maturation into plasmacytes, the secretion of antibodies and ultimately immunological destruction of hepatocytes (Aithal, 2011).
Statins/HMG-CoA Reductase Inhibitors
Statins are the widely used drugs in the Western countries including United States. Statins inhibit the process of cholesterol biosynthesis by competitively inhibiting HMG-CoA reductase. Further, they also decrease the low-density lipoprotein levels and increase the atherosclerotic plaques stability (Jacobson, 2006). The use of higher statin doses is shown to exhibit biochemical abnormalities of liver function as indicated by moderate elevations of hepatotoxicity markers in animal models (Horsmans et al., 1990). High doses of derivatives of statins, lovastatin and simvastatin, have been shown to cause significant hepatocellular necrosis in rabbits and guinea pigs respectively (Horsmans et al., 1990). Nevetheless, hepatocellular necrosis by statins is rarely observed in humans (Alonso et al., 1999).
Other Drugs
There are some other commonly used drugs that cause liver damage, for example, valproic acid, an antiepileptic drug, may cause liver damage in approximately 20% patients; antibiotics namely ciprofloxacin, erythromycin, amoxicillin may produce symptoms ranging from jaundice, acute hepatotoxicity to liver malfunction; chlorpromazine is found to produce symptoms of jaundice; amiodarone causes acute hepatotoxicity in both animal and human studies; oral contraceptives may cause jaundice and cholestatic liver injury and so forth.
Understanding the Mechanism of Hepatotoxicity
Mechanism of drug-induced hepatotoxicity is variable and complex (Figure 2). Some drugs are directly toxic and begin to exhibit hepatotoxic reactions, which are dose related, within hours of exposure, whereas others may produce liver injury only in susceptible people and symptoms appear after few days or weeks. These reactions are rarely allergic or more accurately described as idiosyncratic. Drug-drug interaction, though the drugs are not hepatotoxic themselves, may also play a critical role in the propagation of toxicity (Yamazaki et al., 2005). The pathogenesis of drug-induced toxicity mediated by reactive metabolites has been a center of research enthusiasm since spearheading examinations in the 1950s uncovered the connection between these metabolites and chemical carcinogenesis (Park et al., 2005). A major cause of hepatotoxic reactions may be drug-induced intrahepatic cholestasis, which often occurs during the drug discovery and development process. The vital roles of ROS in the cellular damage are widely investigated and it has been suggested that the covalent binding of ROS as well as reactive intermediates to macromolecules could likely contribute to the severe harmful drug reactions (Racknagel et al., 1989; Masubuchi et al., 2007). There are several studies that suggest the generation of reactive metabolites and free radicals from hepatotoxic drugs (Racknagel et al., 1989; Park et al., 2005). Membrane lipid peroxidation is directly related to the depletion of tissue GSH (an intracellular antioxidant) leading to the altered functional integrity of these structures and if the damage is sever, it could be fatal (Ross, 1988; Guven and Gulmez, 2003). Membrane lipid peroxidation may lead to alteration in membrane fluidity and permeability, enhanced rates of protein degradation, and ultimately cell death (García et al., 1997). The assumption is upheld by the way that oxidative damage to erythrocytes causes loss of membrane capacity by enhancing lipid peroxidation (LPO) and modifying the erythrocyte antioxidant framework (Vajdovich et al., 1995). The concentration of intracellular GSH, therefore, is the key determinant of membrane integrity and the extent of toxicant-induced hepatic cell injury (Ross, 1988; Guven and Gulmez, 2003).
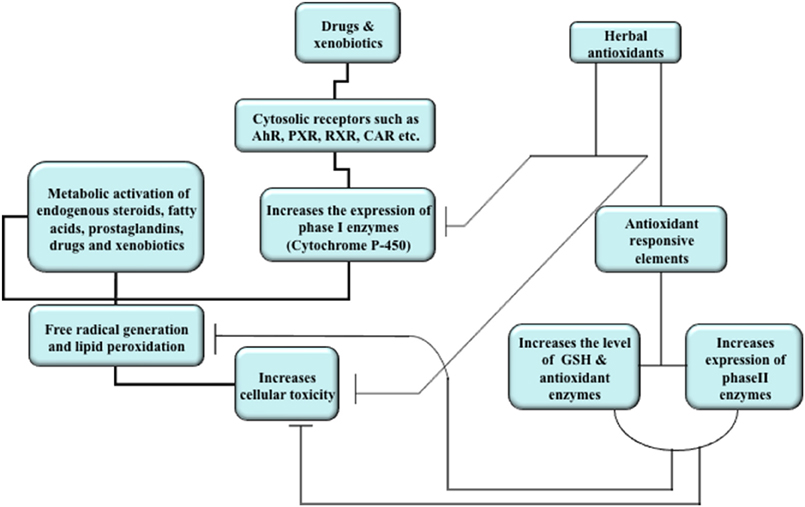
Figure 2. Overview of mechanism of drug and xenobiotic metabolism and effects of herbal antioxidants. Induction of nuclear receptors by drugs and xenobiotics lead to translocation in the nucleus where they increase the expression of cytochrome P450 enzymes. The activity of these enzymes generates reactive metabolites and free radicals which in turn bind to macromolecules, cause membrane lipid peroxidation, and increase cellular toxicity. Natural products increase the expression of phase II enzymes, the level of intracellular antioxidant (GSH), and antioxidant enzymes. The natural products may also inhibit the activities of cytochrome P450 enzymes in order to attain dynamic homeostasis and reduce cellular toxicity.
Drug-Metabolizing Enzymes (DMEs)
DMEs and transporters are key factors that affect the disposition of xenobiotics (Cheng et al., 2005). These DMEs include mix function oxidases and phase II backup machinery that are actively involved in the detoxification process. Recently, the roles of transporters (Phase III enzymes) also have been implicated in the detoxification procedure.
Phase I Enzymes (Mixed Function Oxidases)
Cytochrome P450 (CYP), a super gene family of heme-containing, mixed function oxidase enzymes, are involved in the metabolism of endogenous steroid hormones, vitamins, endobiotics (fatty acid derivatives), various drugs/xenobiotics, and are readily inducible by many exogenous and endogenous substances (Nelson et al., 1996; Ahmed and Pawar, 1997). The CYP-catalyzed oxidation of xenobiotics generate a highly electrophilic intermediates capable of forming covalent adducts with critical cell macromolecules, such as thiol-containing membrane proteins that direct Ca++ homeostasis (Bellomo and Orrenius, 1985; Dahm and Jones, 1996; Vessey, 1996). The activation of increased intracellular calcium may be the regular pathway promoting cell death. CYP-mediated reduction of halogenated hydrocarbons, for example, carbon tetrachloride or halothane, can likewise create free radical intermediates, which can straightforwardly harm cell layers through lipid peroxidation, or can target nucleophilic DNA deposits (Thor and Orrenius, 1980; De Groot and Noll, 1983; Racknagel et al., 1989; Dahm and Jones, 1996).
Phase I reactions, catalyzed by various drugs and xenobiotics, are mediated by various isoforms of CYPs and are regulated through various nuclear receptors. Phase I enzyme impelling by classes of microsomal inducers happens by means of enactment of transcription factors, for example, aryl hydrocarbon receptor (AhR), constitutive androstane receptor (CAR), pregnane X receptor (PXR) and peroxisome proliferator-actuated receptor α (PPARα) (Cheng et al., 2005). Various isoforms of CYP are reported to be involved in the metabolism and therefore bioactivation of drugs. CYP3A, CYP2D, and CYP2C sub-families are involved in the metabolic activation of 50%, 25%, and 20%, respectively, of the currently used clinical drugs (Rendic, 2002; Deng et al., 2008). CYP1A2, 2C7, 2C11, 2D2, 2E1, 2B1/2, and 3A1/2 are the major CYP isoforms involved in the drug metabolism in rats (Kobayashi et al., 2002; Deng et al., 2008). Involvement of CYP2E1 and 1A2 in the acetaminophen and pyrogallol activation has been well established in animal models (Raucy et al., 1989; Snawder et al., 1994; Upadhyay et al., 2007). The activation of paracetamol to N-acetyl-para-benzoquinoneimine (NAPQI) is catalyzed by CYP2E1, 3A4, and 1A2 in humans (Raucy et al., 1989; Snawder et al., 1994). The involvement of CYP2E1 in rifampicin and ethanol-induced liver toxicity has also been reported (Jaeschke et al., 2002; Upadhyay et al., 2007).
Regulation of Phase I Enzymes: Nuclear Receptors
Regulation of phase I enzymes, particularly CYPs is coupled with various transcription factors also called nuclear receptors (NR). These are the largest known family of transcription factors that modulates the tissue gene expression and are associated largely with metabolism, developmental function, and cell differentiation. There are 49 known members of the nuclear receptor superfamily and each share key structural features (Urquhart et al., 2007; Woods et al., 2007). These are the targets of approximately 10–15% pharmaceuticals and explore about $400 billion global pharmaceutical markets (Woods et al., 2007). The majority of the nuclear receptors target on various proteins included in xenobiotic metabolism, in particular, CYP enzymes (Waxman, 1999; Honkakoski and Negishi, 2000; Johnson and Klaassen, 2002). Several receptors require heterodimerization with retinoid X receptor (RXR) (Mangelsdorf and Evans, 1995). Activation of nuclear receptors, for example, aryl hydrocarbon receptors (AhR), RXR, pregane X receptors (PXR), and peroxisome proliferator-initiated receptors (PPAR) are crucial for some clinically imperative drug-drug interactions. Activation of phase I enzymes by agonists can be ascribed for activation of signal transduction pathways. Various microsomal protein inducers display their effects through prompting of diverse orphan nuclear receptors, therefore enhancing target gene expression (Denison and Nagy, 2003; Sonoda et al., 2003). For example, 2,3,7,8-tetrachlorodibenzo-p-dioxin (TCDD) binds to the AhR, which releases the AhR from the cytosolic tethering protein HSP90, permitting AhR translocation to the nucleus, heterodimerization with aryl hydrocarbon receptor nuclear translocator (ARNT), and the binding to xenobiotic response elements on the CYP1A1 promoter. Also, CAR ligands affect CYP2B10, PXR ligands incite CYP3A11, and peroxisome proliferator-initiated receptor α (PPAR α) ligands activate CYP4A14 (Cheng et al., 2005). The pregnane X receptor (PXR, NR1I2), a member of the nuclear receptor superfamily, is activated by a lot of compounds and natural products. It is involved in the regulation of various CYP isoforms such as CYP 3A4, which is involved in the metabolism of about 60% of all prescription drugs (Lehmann et al., 1998; Guengerich, 1999; Kliewer, 2003). PXR sometimes mediates the protective effects also in case of some herbal remedies besides its undesirable effects in patients on combination therapy (Staudinger et al., 2006).
Phase II Enzymes
Detoxification by phase II enzymes eliminates the reactive intermediates from cellular environments and therefore decreases the burden of bio-molecular adducts on cellular homeostasis (Habig et al., 1974; Moon et al., 2006). Phase II enzymes are mainly involved in the conjugation of activated pro-toxicants with endogenous bio-molecules like GSH or glucuronic acid which reduces toxicity and increases water solubility (Habig et al., 1974; Moon et al., 2006). Glutathione-S-transferases (GST), a crucial Phase II enzyme, initiates the detoxification process by catalyzing the conjugation of –SH moiety of glutathione to xenobiotics, thereby neutralizing the electrophilic sites and rendering the products more water-soluble. Glutathione conjugates are thought to be metabolized further by cleavage of the glutamate and glycine residues, followed by acetylation of resultant free amino groups of the cysteinyl residue, to produce the final product, a mercapturic acid (Boyland and Nery, 1969; Wood and Woodcock, 1970).
Regulation of Phase II Enzymes
Phase II enzymes are regulated by regulatory elements called ARE (anti-oxidant response elements), which are situated upstream to promoter regions (Hayes and McMahon, 2001). Regulation is coupled with the activation of ARE through activation of a transcription factor, nuclear erythroid factor 2—related factor 2 (Nrf2; Lee and Surh, 2005). Nrf2 either upregulates or inhibits transcription of phase II enzymes through ARE by the heterodimerization with an array of leucine b-zip family members such as small maf proteins, fos, jun, and so forth (Jaiswal, 2000; Cheng et al., 2005). Nrf2 is tightly coupled with Keap1, which acts as a negative regulator of Nrf2 and as a sensor of xenobiotic and oxidative stresses (Motohashi and Yamamoto, 2004). Nrf2 activation leads to its release from Keap1 followed by translocation to the nucleus, heterodimerization to other leucine zipper proteins, and binding to ARE in order to transcriptionally activate the downstream targets (Motohashi and Yamamoto, 2004). Nrf2 activation is triggered by the ROS modulation in cells via the interaction of a various signaling molecules specified by cell types (Hayes and McMahon, 2001). Therefore, most chemopreventive operators are the modulators of cell ROS and subsequently activate Nrf2 pathway, which thusly incite phase II detoxifying response (Hayes and McMahon, 2001). In addition to the dynamic equilibrium of Phase I and Phase II enzymes, reduced GSH, glutathione peroxidase (GPx) and catalase (CAT) are also critical in acquisition of normal cellular physiology as these are involved in the elimination of lipid peroxides and toxic oxygen radicals formed during the cellular metabolism (Trackshel and Maines, 1988; Tanaka et al., 2004; Pal et al., 2006).
Another adoptive cellular response against drugs/toxicants is the induction of heme oxygenase-1 (HO-1), the rate-limiting enzyme in the breakdown of heme into carbon monoxide (CO), iron and bilirubin (Farombi and Surh, 2006). HO-1 is instigated by oxidative stress stimuli and its enactment is considered an adoptive survival response (Immenschuh and Ramadori, 2000). HO-1 impelling possibly provides security against oxidative damage, for example, in liver ischemia/reperfusion secondary to transplantation or hemorrhage/resuscitation. Induction of HO-1 controls the intracellular levels of “free” heme (a prooxidant), increases the delivery of biliverdin (an antioxidant), enhances nutritive perfusion, and cultivates the amalgamation of the Fe-binding protein ferritin to ensure the protection of cells against oxidative stress (Bauer and Bauer, 2002). CO and biliverdin/bilirubin, which are generated as a product of HO-1 activity, have been accounted for the protective effects in a few organs, including the liver (Farombi and Surh, 2006). Additionally, HO-1 is an intense defensive component for cytokine-and CD95-intervened apoptotic liver damages (Sass et al., 2003). Nevertheless, the heme oxygenase gives a defensive shield against oxidative stress to a certain level of expression and a large amounts of HO-1 may even sensitize the cells to oxidative damage by increasing the level of “free” reactive iron (Bauer and Bauer, 2002).
Role of Transporters
The reduction in expression of uptake transporters, and augmentation in expression of export transporters, as well as detoxification enzymes, may prevent the hepatocytes from accumulating potentially toxic products (Aleksunes et al., 2005). Therefore, the instances and propagation of liver injury appears to be a coordinated phenomenon involving both transport and detoxification genes (Aleksunes et al., 2005). The phenomenon of extraction and subsequent excretion of drugs and their toxic metabolites from portal blood is performed by basolateral and canalicular transporters in hepatocyte plasma membranes (Arrese and Accatino, 2002). Uptake carriers, such as organic anion-transporting polypeptides (Oatps) and the sodium/taurocholate-cotransporting polypeptide (Ntcp), and export transporters such as multidrug resistance proteins (Mdrs), multidrug resistance-associated proteins (Mrps), bile salt export pump (Bsep), and breast cancer resistance protein (Bcrp) are actively involved in the process of transportation of drugs/xenobiotics from absorption till excretion (Arrese and Accatino, 2002; Geier et al., 2002; Song et al., 2003). The transporters perform specialized functions, for example, canalicular transporters (Mrp2, Mdrs, Bcrp, and Bsep), are involved in the excretion of xenobiotics and their metabolites from hepatocytes into bile, and basolateral transporters (Mrp 1, 3–6) mediate the efflux of drugs and chemicals from hepatocytes into blood (Arrese and Accatino, 2002; Geier et al., 2002; Song et al., 2003). Various reports are available online implicating the involvement of these transporters in the xenobiotics metabolism and propagation of cell injury (Arrese and Accatino, 2002; Geier et al., 2002; Song et al., 2003; Ghanem et al., 2004; Heijne et al., 2004).
Herbal Medication
Herbal antioxidants have attracted the researchers due to its potential and efficacy against drug-induced liver toxicity. Natural products used in China and India as traditional medicine for the treatment of liver disorders are of great interest in these days. These are the potential sources for new therapeutic agents that could be used in the prevention of hepatic injuries. Natural products rich in triterpenes, flavonoids or polyphenols, have been now established as powerful hepatoprotective agents in experimental liver-injury cell and animal models (Table 2; Gupta et al., 2002, 2004; King and Cousins, 2006; Upadhyay et al., 2007, 2008, 2010a,b). The basis behind the protection provided by the natural products is hypothesized to be their antioxidant property through which they remove the free radicals from the cellular environment and therefore provide protection against ROS mediated damage to membrane lipids and macromolecules (Figure 2; Gupta et al., 2002, 2004; Upadhyay et al., 2007, 2008, 2010a,b). Additionally, the protective potential of natural products is also contributed by its interaction with various CYP isoforms, its capability to increase GSH biosynthesis, level of Phase II/antioxidant enzymes and to inhibit the entry of toxins to the cells (Figure 2; Gupta et al., 2002, 2004; King and Cousins, 2006; Upadhyay et al., 2007, 2008, 2010a,b; Yarnell and Abascal, 2007). Some of the natural products containing polyphenols are considered as potential chemopreventive and hepatoprotective agents. In the last decade, several studies have been conducted to investigate the mechanism of action of natural products at biochemical, genomic and proteomic levels. The most extensively investigated natural products for hepatoprotection are silymarin, resveratrol, curcumin and gingko due to their high efficacies, low or no toxicity and easy availabilities.
Silymarin
Silymarin, isolated from the seeds of milk thistle (Silybum marianum), is an unique flavonoid-complex containing silybinin, silydianin, and silychristin and have been extensively studied (Flora et al., 1996; Madrigal-Santillán et al., 2013, 2014). Silibinin is major constituent of silymarin. Two main mechanisms of action of silymarin have been proposed based on its cell-regenerating function and cytoprotective effect. Cytoprotection is mediated by its antioxidant properties and direct interaction with cell membrane components (Muriel and Mourelle, 1990; Mira et al., 1994). Inhibition of lipid peroxidation, as reported in various in vitro studies using erythrocytes (Valenzuela et al., 1987), isolated and cultured hepatocytes (Joyeux et al., 1990; Miguez et al., 1994), and human mesangial cells, is accepted as one of silymarins major protective mechanisms. Silymarin has been also reported to have anti-inflammatory (De la Puerta et al., 1996), anti-fibrotic (Fuchs et al., 1997), and anti-proliferative effects (Scambia et al., 1996). Diverse biochemical reactions, such as, the incitement of the synthetic rate of ribosomal RNA (rRNA) species through the induction of polymerase I and rRNA transcription, shielding the cell from free radical-mediated injury and blockage of the uptake of toxins, also contribute to the protective potential of silymarin (Sonnenbichler and Zetl, 1986; Sonnenbichler et al., 1999; Wellington and Jarvis, 2001). Silymarin offers protection against enlarged liver by inhibiting 5-lipoxygenase, production of leukotrienes and generation of free radicals in Kupffer cells. Moreover, silybin, a major component of silymarin, protects against the membrane lipid peroxidation and cellular damage in the mouse hepatocytes (Yin et al., 2011; Bahmani et al., 2015).
Silymarin has been shown to be effective in combating against drug-induced hepatotoxicity in various animal models (Gupta et al., 2002, 2004; Upadhyay et al., 2007). Hepatoprotective potential of silymarin is suggested to be due to its antioxidant, cell regeneration and cytoprotection activity (Sonnenbichler et al., 1999; Gupta et al., 2002, 2004; Upadhyay et al., 2007; Ahmad et al., 2013; Yang et al., 2014b). It is equally efficient in animals as well as in humans. It increases the level of glutathione and glutathione peroxidase in the serum of patients and animals. Although silymarin has low oral absorption, oral dosages of 420 mg/day have shown some therapeutic potential, with good tolerability in alcoholic cirrhosis patients. Moreover, daily dose of silybin (20–48 mg/kg) is supposed to be an antidote for acute mushroom poisoning by Amanita phalloides. Some isoforms of Silybin have been shown to possess the strongest anti-NF-κB and anti-HCV activity (Polyak et al., 2007). It has been shown to offer protection against prostate cancer by the inactivation of erbB1-SHC (Src homology 2 domain containing) signaling pathway and induction of CDKIs, and a resultant G1 arrest (Zi et al., 1998; Zi and Agarwal, 1999). Additionally, it inhibits xanthine oxidase and mRNA expression of TNF-α, which plays critical role in mouse skin tumorigenesis (Zi et al., 1997; Sheu et al., 1998). At lower non-toxic concentrations, it inhibits transformation in cultured rat tracheal epithelial cells treated with benzo[a]pyrene, by which chemopreventive compounds that act at early stages of the carcinogenic process, could be identified (Steele et al., 1990; Kohno et al., 2002). Protective potential of silymarin against rifampicin and pyrogallol-induced hepatotoxicity has been found to be due to its modulatory effect on the augmented level of CYPs and attenuated level of phase II and antioxidant enzymes (Upadhyay et al., 2007). In a clinical trial with over 2000 patients with chronic liver diseases, administration of silymarin extract for 8 weeks resulted in a significant decrease in liver damage index in approximately 88% of the patients (Nasri et al., 2013). It was remarkable that some minor side effects were observed only in less than 1% of patients (Nasri et al., 2013).
Resveratrol
Resveratrol (trans-3,4′,5-trihydroxy-trans-stilbene) is a natural polyphenol present in significant amounts in peanuts, the skin of grapes and red wine. Potential health benefits of resveratrol have been widely investigated in past few years. Its activity in chemoprevention, cardiovascular diseases and neurodegenerative disorders has been reported in preclinical studies (Surh, 1999; Frémont, 2000; Ignatowicz and Baer-Dubowska, 2001; Latruffe et al., 2002; Gescher and Steward, 2003; Srivastava et al., 2013). Mechanism based inactivation of CYP2E1 and CYP1A2 by resveratrol has been reported previously (Chang et al., 2001; Mikstacka et al., 2002; Upadhyay et al., 2008). Further, it has been demonstrated that the metabolic hydroxylation of resveratrol by CYP1B1, results in its conversion to piceatannol, a tyrosine kinase inhibitor and a compound of known anticancer activity (Geahlen and McLaughlin, 1989; Mikstacka et al., 2002; Potter et al., 2002). The substitution of the hydroxy with methoxy groups in resveratrol molecules may increase its lipophilicity and binding to the active sites of CYPs (Chun et al., 2001; Regev-Shoshani et al., 2004).
Recently it has been shown by Wang et al., that resveratrol prevents acetaminophen-mediated liver damage by inhibiting CYP-mediated bioactivation of acetaminophen and regulating SIRT1, p53, cyclin D1, and PCNA to facilitate liver regeneration (Wang et al., 2015). Zhang et al., found that pretreatment of resveratrol effectively reversed As2O3-induced liver toxicity indices and resulted in a significant improvement in hepatic function in cat models. Moreover, resveratrol also improved the glutathione levels, activities of antioxidant enzymes and attenuated As2O3-induced increases in reactive oxygen species and malondialdehyde production in liver tissues (Zhang et al., 2014). Resveratrol has also been shown to offer protection against methotrexate, sodium fluoride and azozymethane- induced hepatotoxicity in animal models (Dalaklioglu et al., 2013; Gurocak et al., 2013; Atmaca et al., 2014).
Curcumin
Curcumin is one of the most widely used herbal formulations to protect drug-induced toxicity. It is the major constituent of the spice turmeric extracted from the root of Curcumalonga Linn. It is metabolized to curcumin glucuronides, sulfates, tetrahydrocurcumin and hexacurcumin in the intestine and liver of human and rats and is reported to exhibit antioxidant, anti-inflammatory, choleretic, anti-carcinogenic, antiviral, and anti-infectious activities (Pan et al., 1999; Joe et al., 2004; Maheshwari et al., 2006). It inhibits the expression of the enzyme cyclooxygenase 2 via interference with activation of the transcription factor NF-κB and is a potential hepatoprotectant (Plummer et al., 1999; Duvoix et al., 2005; Aggarwal and Shishioda, 2006). Curcumin may be used in combination with conventional chemotherapeutic drugs to reverse multi drug resistance (MDR) in cancer cells and is the most effective MDR modulator among curcuminoids (Chearwae et al., 2004). Curcumin attenuates the proliferation of human lymphocytes and the production of several inflammatory mediators such as lipid mediators and cytokines and possess immunosuppressive and anti-rheumatic activity (Fahey et al., 2007; Gautam et al., 2007; Sandur et al., 2007). Curcuminoids have also been reported to inhibit pro-inflammatory induction by enhancing peroxisome proliferator-activated receptor-gamma (PPAR-gamma) activation (Jacob et al., 2007). Soliman et al., showed the curcumin-mediated protection against paracetamol-induced liver damage by restoring antioxidant activity of liver. They further showed that curcumin effectively restored the paracetamol-mediated increase in matrix metalloproteinase-8 (MMP-8), interleukin-1β (IL-1β), IL-8, tumor necrosis factor-α (TNF-α), and acute phase proteins and decrease in the expression of antioxidant genes (Soliman et al., 2014). Curcumin offers hepatoprotection against cisplatin, alcohol and heavy metals-induced liver damage in animals (Lee et al., 2013; García-Niño and Pedraza-Chaverrí, 2014; Waseem et al., 2014; Sankar et al., 2015; Soliman et al., 2015).
Gingko
The Ginkgo biloba extract possess memory-enhancing, cognition-improving, immuno-modulating, apoptosis-inducing, and antiplatelet effects (Oken et al., 1998; Diamond et al., 2000; Wesnes et al., 2000; McKenna et al., 2001; Mahady, 2002; Andrieu et al., 2003; Ponto and Schultz, 2003; Yang et al., 2014a). The therapeutic benefits of this herbal medicine in neurological disorders, chronic refractory schizophrenia, hepatotoxicity, and in sleep disturbance of depressed patients have been reported (Hemmeter et al., 2001; Watanabe et al., 2001; De Smet, 2002; Yang et al., 2011). The major constituents of ginkgo include flavonoids (e.g., kaempferol), terpenoids (e.g., ginkgolides and bilobalaides), and organic acids (e.g., ginkgolic acids and alkylphenols) (Krieglstein et al., 1995; Jaggy and Koch, 1997; Tang et al., 2001; Lichtblau et al., 2002; Van Beek, 2002). As an adjuvant therapeutic drug, ginkgo appears to be promising in diabetics with respect to ischemic myocardium injury (Schneider et al., 2008). Intake of Ginkgo biloba extract may alter the hepatic metabolism by modulating hepatic drug metabolizing enzymes, altering the level of antioxidant enzymes and endogenous antioxidants such as GSH. The altered hepatic metabolism may result in the clearance of co-administered drugs, in particular, that have reduced renal and liver function (Deng et al., 2008).
Other Herbal Medicines
Use of herbs in treatment of various liver disorders is common in China. The potential of these herbs against diseases with few and even no side effects continuously increases its popularity (Table 3). Now these medicines are being gradually accepted worldwide, particularly in Asia, Europe and North America. Nevertheless, the application strategy may differ in East and West due to variety of reasons, such as, philosophical viewpoint, concept of diseases, and treatment approaches.
In a review published in 2007 on Chinese medicine, the authors have summarized 274 species (in 216 genera among 92 families) of herbs possessing protective effect against liver toxicity (Wang et al., 2007). They further suggested a crude classification of these drugs in two groups; firstly, the main ingredients, for example silybin, osthole, cumarin, glycorrhizin, flavonoids and so on; and secondly, the supporting substances like sugars, amino acids, resins, tannins, and volatile oil.
Liver Injury Associated with Herbal Products
The drug-induced liver injuries are debated since decades; the liver toxicity of certain herbal products has only recently been recognized. There are not many studies available on this alarming aspect of herbal products till date; however, the threat cannot be ignored as they may present with the same spectrum of liver pathologies as synthetic products (Stickel and Shouval, 2015). A large number of herbal and dietary products including Shou-Wu Pian (Polygonum multiflorum), Breynia officinalis, Germander (Teucrium chamaedrys), Chaparral (Larrea tridentata), Actractylis gummifera, Impila (Callilepsis laureola), Pennyroyal (Mentha pulegium), Greater celandine (Chelidonium majus), Kava (Piper methysticum), Black cohosh (Cimifuga racemosa), Noni juice (Morinda citrifolia), Gotu Kola (Centella asiatica), etc. are reported to have liver damaging effects (Stickel and Shouval, 2015). These products when consumed may cause symptoms ranging from acute, chronic, cholestatic, fulminant, and acute autoimmune-like hepatitis to acute liver failure, and liver cirrhosis. The mechanism of their toxicities are largely unknown; however, involvement of oxidative stress and apoptosis is also reported (Stickel and Shouval, 2015).
Conclusion
The nature and dose of a particular drug are not the only determining factors of cell injury. Other factors such as an individual's gene expression profile, antioxidant status and the capacity for regeneration are also crucial. Several mechanisms are involved in the initiation of liver cell damage and aggravate ongoing injury processes. Dysfunction of these vital cell organelles results in impairment of dynamic equilibrium in homeostatic condition, thus resulting in intracellular oxidative stress with excessive formation of reactive oxygen species. Major causes of the hepatotoxic reactions by drugs are elevated ROS generation, oxidative stress and suppressed immune responses. Hepatotoxicity remains a major cause of drug withdrawal from the market. Recent examples in the USA and Europe are ximelagatran, nefazodone, nimesulide, ebrotidine, trovafloxacin, troglitazone, bromfenac, and so forth.
Natural products have shown great promise in combating against the toxicity of several commonly used drugs, including acetaminophen and paracetamol. Additionally, some of these natural products, such as resveratrol and curcumin, are now widely accepted chemopreventive agents. Due to easy availability and dietary nature, it is time to promote the natural products as supplementary medication with drugs that also cause toxicity to cells. Although a majority of natural products investigated to date are non-toxic, some studies have shown liver toxicity by certain natural products. Therefore, the proper selection of the natural products is also necessary. It is envisioned that natural products will not only lower the risk of drug-induced liver damage, but also provide an alternative solution to remedy the drug-induced hepatotoxicity.
Author Contributions
DS wrote the manuscript. WC and GU revised the manuscript.
Conflict of Interest Statement
The authors declare that the research was conducted in the absence of any commercial or financial relationships that could be construed as a potential conflict of interest.
References
Aggarwal, B. B., and Shishioda, S. (2006). Molecular targets of dietary agents for prevention and therapy of cancer. Biochem. Pharmacol. 71, 1397–1421. doi: 10.1016/j.bcp.2006.02.009
Ahmad, I., Shukla, S., Kumar, A., Singh, B. K., Kumar, V., Chauhan, A. K., et al. (2013). Biochemical and molecular mechanisms of N-acetyl cysteine and silymarin-mediated protection against maneb- and paraquat-induced hepatotoxicity in rats. Chem. Biol. Interact. 201, 9–18. doi: 10.1016/j.cbi.2012.10.027
Ahmed, R. S., and Pawar, S. S. (1997). Effect of inducers on hepatic microsomal mixed function oxidase system of male rats during food restriction. Indian J. Exp. Biol. 35, 46–49.
Aithal, G. P. (2004). Diclofenac-induced liver injury: a paradigm of idiosyncratic drug toxicity. Expert Opin. Drug Saf. 3, 519–523. doi: 10.1517/14740338.3.6.519
Aithal, G. P. (2011). Hepatotoxicity related to antirheumatic drugs. Nat. Rev. Rheumatol. 7, 139–150. doi: 10.1038/nrrheum.2010.214
Akagawa, M., Shigemitsu, T., and Suyama, K. (2003). Production of hydrogen peroxide by polyphenol and polyphenol-rich beverages under quasi-physiological conditions. Biosci. Biotechnol. Biochem. 67, 2632–2640. doi: 10.1271/bbb.67.2632
Aleksunes, L. M., Slitt, A. M., Cherrington, N. J., Thibodeau, M. S., Klaassen, C. D., and Manautou, J. E. (2005). Differential expression of mouse hepatic transporter genes in response to acetaminophen and carbon tetrachloride. Toxicol. Sci. 83, 44–52. doi: 10.1093/toxsci/kfi013
Alexander, M. R., Louie, S. G., and Guernsey, B. G. (1982). Isoniazid associated hepatitis. Clin. Pharmacol. 1, 148–153.
Allen, S. J., Gan, Q., Matthews, R., and Johnson, P. A. (2003). Comparison of optimised isotherm models for basic dye adsorption by kudzu. Bioresour. Technol. 88, 143–152. doi: 10.1016/S0960-8524(02)00281-X
Alonso, J. J., Osorio, J. M., Cabello, F. G., Osa, A. L., Leon, L., and Garcia, J. D. M. (1999). Atorvastatin induced cholestatic hepatitis in a young woman with systemic lupus erythematosus. Arch. Intern. Med. 159, 1811–1812. doi: 10.1001/archinte.159.15.1811-a
Andrieu, S., Gillette, S., Amouyal, K., Nourhashemi, F., Reynish, E., Ousset, P. J., et al. (2003). Association of Alzheimer's disease onset with ginkgo biloba and other symptomatic cognitive treatments in a population of women aged 75 years and older from the EPIDOS study. J. Gerontol. 58, 372–377. doi: 10.1093/gerona/58.4.m372
Anundi, I., Lahteenmaki, T., Rundgren, M., Moldeus, P., and Lindros, K. O. (1993). Zonation of acetaminophen metabolism and cytochrome P450 2E1-mediated toxicity studies in isolated periportal and perivenous hepatocytes. Biochem. Pharmacol. 45, 1251–1259. doi: 10.1016/0006-2952(93)90277-4
Arrese, M., and Accatino, L. (2002). From blood to bile: recent advances in hepatobiliary transport. Ann. Hepatol. 1, 64–71.
Atmaca, N., Atmaca, H. T., Kanici, A., and Anteplioglu, T. (2014). Protective effect of resveratrol on sodium fluoride-induced oxidative stress, hepatotoxicity and neurotoxicity in rats. Food Chem. Toxicol. 70, 191–197. doi: 10.1016/j.fct.2014.05.011
Attri, S., Rana, S. V., Vaiphie, K., Katyal, R., Sodhi, C. P., Kanwar, S., et al. (2001). Protective effect of N-acetylcysteine in isoniazid induced hepatic injury in growing rats. Indian J. Exp. Biol. 39, 436–440.
Bagchi, D., Bagchi, M., Stohs, S. J., Das, D. K., Ray, S. D., Kuszynski, C. A., et al. (2000). Free radicals and grape seed proanthocyanidin extract: importance in human health and disease prevention. Toxicology 148, 187–197. doi: 10.1016/S0300-483X(00)00210-9
Bahmani, M., Shirzad, H., Rafieian, S., and Rafieian-Kopaei, M. (2015). Silybum marianum: beyond hepatoprotection. J. Evid. Based Complementary Altern. Med. 20, 292–301. doi: 10.1177/2156587215571116
Bakke, O. M., Manocchia, M., de Abajo, F., Kaitin, K. I., and Lasagna, L. (1995). Drug safety discontinuations in the United Kingdom, the United States, and Spain from 1974 through 1993: a regulatory perspective. Clin. Pharmacol. Ther. 58, 108–117. doi: 10.1016/0009-9236(95)90078-0
Banks, A. T., Zimmerman, H. J., Ishak, K. G., and Harter, J. G. (1995). Diclofenac-associated hepatotoxicity: analysis of 180 cases reported to the Food and Drug Administration as adverse reactions. Hepatology 22, 820–827.
Bauer, M., and Bauer, I. (2002). Heme oxygenase-1: redox regulation and role in the hepatic response to oxidative stress. Antioxid. Redox. Signal. 4, 749–758. doi: 10.1089/152308602760598891
Bell, L. N., and Chalasani, N. (2009). Epidemiology of idiosyncratic drug-induced liver injury. Semin. Liver Dis. 29:337. doi: 10.1055/s-0029-1240002
Bellomo, G., and Orrenius, S. (1985). Altered thiol and calcium homeostasis in the oxidative hepatocellular injury. Hepatology 5, 876–882. doi: 10.1002/hep.1840050529
Ben-Amotz, A., Yatziv, S., Sela, M., Greenberg, S., Rachmilevich, B., Shwarzman, M., et al. (1998). Effect of natural beta-carotene supplementation in children exposed to radiation from the Chernobyl accident. Radiat. Environ. Biophys. 37, 87–193. doi: 10.1007/s004110050116
Biour, M., Ben Salem, C., Chazouillères, O., Grangé, J. D., Serfaty, L., and Poupon, R. (2004). Drug-induced liver injury; fourteenth updated edition of the bibliographic database of liver injuries and related drugs. Gastroenterol. Clin. Biol. 28, 720–759. doi: 10.1016/S0399-8320(04)95062-2
Björnsson, E. (2009). The natural history of drug-induced liver injury. Semin. Liver Dis. 29, 357–363. doi: 10.1055/s-0029-1240004
Björnsson, E. S., Bergmann, O. M., Björnsson, H. K., Kvaran, R. B., Olafsson, S., et al. (2013). Incidence, presentation, and outcomes in patients with drug-induced liver injury in the general population of Iceland. Gastroenterology 144:1419. doi: 10.1053/j.gastro.2013.02.006
Blazer, D. G., and Wu, L. T. (2009). Nonprescription use of pain relievers by middle-aged and elderly community-living adults: national survey on drug use and health. J. Am. Geriatr. Soc. 57, 1252–1257. doi: 10.1111/j.1532-5415.2009.02306.x
Boelsterli, U. A., Ho, H. K., Zhou, S., and Leow, K. Y. (2006). Bioactivation and hepatotoxicity of nitroaromatic drugs. Curr. Drug Metab. 7, 715–727. doi: 10.2174/138920006778520606
Bol'shakova, I. V., Lozovskaia, E. L., and Sapezhinskii, I. I. (1997). Antioxidant properties of a series of extracts from medicinal plants. Biofizika 42, 480–483.
Boyland, E., and Nery, R. (1969). Mercapturic acid formation during the metabolism of arecoline and arecaidine in the rat. Biochem. J. 113, 123–130. doi: 10.1042/bj1130123
Cao, G., Sofic, E., and Prior, R. L. (1997). Antioxidant and prooxidant behavior of flavonoids: structure-activity relationships. Free Radic. Biol. Med. 22, 749–760. doi: 10.1016/S0891-5849(96)00351-6
Chalasani, N., Fontana, R. J., Bonkovsky, H. L., Watkins, P. B., Davern, T., Serrano, J., et al. (2008). Causes, clinical features, and outcomes from a prospective study of drug-induced liver injury in the United States. Gastroenterology 135:1924. doi: 10.1053/j.gastro.2008.09.011
Chang, T. K., Chen, J., and Lee, W. B. (2001). Differential inhibition and inactivation of human CYP1 enzymes by trans-resveratrol: evidence for mechanism-based inactivation of CYP1A2. J. Pharmacol. Exp. Ther. 299, 874–882.
Chearwae, W., Anuchapreeda, S., Nandigama, K., Ambudkar, S. V., and Limtrakul, P. (2004). Biochemical mechanism of modulation of human P-glycoprotein (ABCB1) by curcumin I, II, and III purified from Turmeric powder. Biochem. Pharmacol. 68, 2043–2052. doi: 10.1016/j.bcp.2004.07.009
Chen, J., Zhang, W., Lu, T., Li, J., Zheng, Y., and Kong, L. (2006). Morphological and genetic characterization of a cultivated Cordyceps sinensis fungus and its polysaccharide component possessing antioxidant property in H22 tumor-bearing mice. Life Sci. 78, 2742–2748. doi: 10.1016/j.lfs.2005.10.047
Chen, J. W., Chen, Y. H., Lin, F. Y., Chen, Y. L., and Lin, S. J. (2003). Ginkgo biloba extract inhibits tumor necrosis factor-{alpha}-induced reactive oxygen species generation, transcription factor activation, and cell adhesion molecule expression in human aortic endothelial cells. Arterioscler. Thromb. Vasc. Biol. 23, 1559–1566. doi: 10.1161/01.ATV.0000089012.73180.63
Cheng, X., Maher, J., Dieter, M. Z., and Klaassen, C. D. (2005). Regulation of mouse organic anion-transporting polypeptides (oatps) in liver by prototypical microsomal enzyme inducers that activate distinct transcription factor pathways. Drug Metab. Dispos. 33, 1276–1282. doi: 10.1124/dmd.105.003988
Chun, Y. J., Ryu, S. Y., Jeong, T. C., and Kim, M. Y. (2001). Mechanism-based inhibition of human cytochrome P450 1A1 by rhapontigenin. Drug Metab. Dispos. 29, 389–393.
Coleman, J. D., Prabhu, K. S., Thompson, J. T., Reddy, P. S., Peters, J. M., Peterson, B. R., et al. (2007). The oxidative stress mediator 4-hydroxynonenal is an intracellular agonist of the nuclear receptor peroxisome proliferator-activated receptor-beta/delta (PPARbeta/delta). Free Radic. Biol. Med. 42, 1155–1164. doi: 10.1016/j.freeradbiomed.2007.01.003
Contreras, A. M., Monteón, F. J., Flores, M. R., Mendoza-Sánchez, F., and Ruiz, I. (2007). Drug-related hepatotoxicity in a renal transplant recipient with long-term survival and hepatitis C. Ann. Hepatol. 6, 70–73.
Dahm, L. J., and Jones, D. P. (1996). “Mechanisms of chemically induced liver disease,” in Hepatology: A Textbook of Liver Disease, 3rd Edn., eds D. Zakin and T. D. Boyer (Philadelphia: WB Saunders), 875–890.
Dalaklioglu, S., Genc, G. E., Aksoy, N. H., Akcit, F., and Gumuslu, S. (2013). Resveratrol ameliorates methotrexate-induced hepatotoxicity in rats via inhibition of lipid peroxidation. Hum. Exp. Toxicol. 32, 662–671. doi: 10.1177/0960327112468178
DeFeudis, F. V., Papadopoulos, V., and Drieu, K. (2003). Ginkgo biloba extracts and cancer: a research area in its infancy. Fundam. Clin. Pharmacol. 17, 405–417. doi: 10.1046/j.1472-8206.2003.00156.x
De Groot, H., and Noll, T. (1983). Halothane hepatotoxicity: relation between metabolic activation, hypoxia, covalent binding, lipid peroxidation and liver cell damage. Hepatology 3, 601–606. doi: 10.1002/hep.1840030421
De la Puerta, R., Martinez, E., Bravo, L., and Ahumada, M. C. (1996). Effect of silymarin on different acute inflammation models and on leukocyte migration. J. Pharm. Pharmacol. 48, 968–970. doi: 10.1111/j.2042-7158.1996.tb06014.x
Deng, Y., Bi, H. C., Zhao, L. Z., He, F., Liu, Y. Q., Yu, J. J., et al. (2008). Induction of cytochrome P450s by terpene trilactones and flavonoids of the Ginkgo biloba extract EGb 761 in rats. Xenobiotica 38, 465–481. doi: 10.1080/00498250701883233
Denison, M. S., and Nagy, S. R. (2003). Activation of the aryl hydrocarbon receptor by structurally diverse exogenous and endogenous chemicals. Annu. Rev. Pharmacol. Toxicol. 43, 309–334. doi: 10.1146/annurev.pharmtox.43.100901.135828
DerMarderosian, A. (ed.). (2001). The Review of Natural Products, 1st Edn. St. Louis: Facts and Comparisons.
Diamond, B. J., Shiflett, S. C., Feiwel, N., Matheis, R. J., Noskin, O., Richards, J. A., et al. (2000). Ginkgo biloba extract: mechanisms and clinical indications. Arch. Phys. Med. Rehabil. 81, 668–678. doi: 10.1016/s0003-9993(00)90052-2
Duvoix, A., Blasius, R., Delhalle, S., Schenkenburger, M., Morceau, F., Henry, E., et al. (2005). Chemopreventive and therapeutic eVects of curcumin. Cancer Lett. 223, 181–190. doi: 10.1016/j.canlet.2004.09.041
Fahey, A. J., Adrian Robins, R., and Constantinescu, C. S. (2007). Curcumin modulation of IFN-beta and IL-12 signalling and cytokine induction in human T cells. J. Cell Mol. Med. 11, 1129–1137. doi: 10.1111/j.1582-4934.2007.00089.x
Farombi, E. O., Akinloye, O., Akinmoladun, C. O., and Emerole, G. O. (1999). Hepatic drug metabolizing enzyme induction and serum triacylglycerol elevation in rats treated with chlordiazepoxide, griseofulvin, rifampicin and phenytoin. Clin. Chim. Acta 289, 1–10. doi: 10.1016/S0009-8981(99)00143-6
Farombi, E. O., and Surh, Y. J. (2006). Heme oxygenase-1 as a potential therapeutic target for hepatoprotection. J. Biochem. Mol. Biol. 39, 479–491. doi: 10.5483/BMBRep.2006.39.5.479
Flora, K., Hahn, M., Rosen, H., and Brenner, K. (1996). Milk thistle (Silybum marianum) for the therapy of liver disease. Am. J. Gastroenterol. 93, 139–143. doi: 10.1111/j.1572-0241.1998.00139.x
Frémont, L. (2000). Biological effects of resveratrol. Life Sci. 66, 663–673. doi: 10.1016/S0024-3205(99)00410-5
Fuchs, E. C., Weyhenmeyer, R., and Weiner, O. H. (1997). Effects of silibinin and of a synthetic analogue on isolated rat hepatic stellate cells and myofibroblasts. Arzneimittelforschung 47, 1383–1387.
García, J. J., Reiter, R. J., Guerrero, J. M., Escames, G., Yu, B. P., Oh, C. S., et al. (1997). Melatonin prevents changes in microsomal membrane fluidity during induced lipid peroxidation. FEBS Lett. 408, 297–300. doi: 10.1016/S0014-5793(97)00447-X
García-Niño, W. R., and Pedraza-Chaverrí, J. (2014). Protective effect of curcumin against heavy metals-induced liver damage. Food Chem. Toxicol. 69, 182–201. doi: 10.1016/j.fct.2014.04.016
Gautam, S. C., Gao, X., and Dulchavsky, S. (2007). Immunomodulation by curcumin. Adv. Exp. Med. Biol. 595, 321–341. doi: 10.1007/978-0-387-46401-5_14
Geahlen, R. L., and McLaughlin, J. L. (1989). Isolation of piceatannol as the antileukemic principle from the seeds of euphorbia lagascae. Biochem. Biophys. Res. Commun. 165, 241–245. doi: 10.1016/0006-291X(89)91060-7
Geier, A., Kim, S. K., Gerloff, T., Dietrich, C. G., Lammert, F., Karpen, S. J., et al. (2002). Hepatobiliary organic anion transporters are differentially regulated in acute toxic liver injury induced by carbon tetrachloride. J. Hepatol. 37, 198–205. doi: 10.1016/S0168-8278(02)00108-3
Gescher, A. J., and Steward, W. P. (2003). Relationship between mechanisms, bioavailibility, and preclinical chemopreventive efficacy of resveratrol: a conundrum. Cancer Epidemiol. Biomark 12, 953–957.
Ghanem, C. I., Gómez, P. C., Arana, M. C., Perassolo, M., Ruiz, M. L., Villanueva, S. S., et al. (2004). Effect of acetaminophen on expression and activity of rat liver multidrug resistance-associated protein 2 and P-glycoprotein. Biochem. Pharmacol. 68, 791–798. doi: 10.1016/j.bcp.2004.05.014
Gruenwald, J., Brendler, T., and Jaenicke, C. (eds.). (2000). PDR for Herbal Medicine, 2nd Edn. Montvale, NJ: Medical Economics.
Guengerich, F. P. (1999). Cytochrome P-450 3A4: regulation and role in drug metabolism. Annu. Rev. Pharmacol. Toxicol. 39, 1–17. doi: 10.1146/annurev.pharmtox.39.1.1
Guerra, M. C., Speroni, E., Broccoli, M., Cangini, M., Pasini, P., Mingett, A., et al. (2000). Comparison between Chinese medical herb Peuraria lobata crude extract and its main isoflavone puerarin antioxidant properties and effects on rat liver CYP-catalased drug metabolism. Life Sci. 67, 2997–3006. doi: 10.1016/S0024-3205(00)00885-7
Gupta, Y. K., Sharma, M., and Chaudhary, G. (2002). Pyrogallol-induced hepatotoxicity in rats: a model to evaluate antioxidant hepatoprotective agents. Methods Find. Exp. Clin. Pharmacol. 24, 497–500. doi: 10.1358/mf.2002.24.8.705070
Gupta, Y. K., Sharma, M., Chaudhary, G., and Katiyar, C. K. (2004). Hepatoprotective effect of New Livfit, a polyherbal formulation, is mediated through its free radical scavenging activity. Phytother. Res. 18, 362–364. doi: 10.1002/ptr.1272
Gurocak, S., Karabulut, E., Karadag, N., Ozgor, D., Ozkeles, N., and Karabulut, A. B. (2013). Preventive effects of resveratrol against azoxymethane induced damage in rat liver. Asian Pac. J. Cancer Prev. 14, 2367–2370. doi: 10.7314/APJCP.2013.14.4.2367
Guven, A., and Gulmez, M. (2003). The effect of kefir on the activities of GSH-Px, G. S. T., CAT, GSH and LPO levels in carbon tetrachloride-induced mice tissues. J. Vet. Med. B 50, 412–416. doi: 10.1046/j.1439-0450.2003.00693.x
Habig, W. H., Pabst, M. J., and Jakaby, W. B. (1974). Glutathione S-transferase. The first enzymatic step in mercapturic acid formation. J. Biol. Chem. 249, 7130–7139.
Halvorsen, B. L., Holte, K., Myhrstad, M. C., Barikmo, I., Hvattum, E., Remberg, S. F., et al. (2002). A systematic screening of total antioxidants in dietary plants. J. Nutr. 132, 461–471.
Hayakawa, F., Kimura, T., Maeda, T., Fujita, M., Sohmiya, H., Fujii, M., et al. (1997). DNA cleavage reaction and linoleic acid peroxidation induced by tea catechins in the presence of cupric ion. Biochim. Biophys. Acta 1336, 123–131. doi: 10.1016/S0304-4165(97)00019-6
Hayes, J. D., and McMahon, M. (2001). Molecular basis for the contribution of the antioxidant responsive element to cancer chemoprevention. Cancer Lett. 174, 103–113. doi: 10.1016/S0304-3835(01)00695-4
Heijne, W. H., Slitt, A. L., van Bladeren, P. J., Groten, J. P., Klaassen, C. D., Stierum, R. H., et al. (2004). Bromobenzene-induced hepatotoxicity at the transcriptome level. Toxicol. Sci. 79, 411–422. doi: 10.1093/toxsci/kfh128
Hemmeter, U., Annen, B., Bischof, R., Brüderlin, U., Hatzinger, M., Rose, U., et al. (2001). Polysomnographic effects of adjuvant ginkgo biloba therapy in patients with major depression medicated with trimipramine. Pharmacopsychiatry 34, 50–59. doi: 10.1055/s-2001-15182
Hinson, J. A., Reid, A. B., McCullough, S. S., and James, L. P. (2004). Acetaminophen-induced hepatotoxicity: role of metabolic activation, reactive oxygen/nitrogen species, and mitochondrial permeability transition. Drug Metab. Rev. 36, 805–822. doi: 10.1081/DMR-200033494
Holt, M., and Ju, C. (2010). Drug-induced liver injury. Handb. Exp. Pharmacol. 196, 3–27. doi: 10.1007/978-3-642-00663-0_1
Hong, X. Z., Li, L. D., and Wu, L. M. (2007). Effects of fenofibrate and xuezhikang on high-fat diet-induced non-alcoholic fatty liver disease. Clin. Exp. Pharmacol. Physiol. 34, 27–35. doi: 10.1111/j.1440-1681.2007.04547.x
Honkakoski, P., and Negishi, M. (2000). Regulation of cytochrome P450 (CYP) genes by nuclear receptors. Biochem. J. 347(Pt 2), 321–337. doi: 10.1042/bj3470321
Horsmans, Y., Desager, J. P., and Harvengt, C. (1990). Biochemical changes and morphological alterations of the liver in guinea-pigs after administration of simvastatin (HMG CoA reductase-inhibitor). Pharmacol. Toxicol. 67, 336–339. doi: 10.1111/j.1600-0773.1990.tb00840.x
Huang, Y. S., Chern, H. D., Su, W. J., Wu, J. C., Lai, S. L., Yang, S. Y., et al. (2002). Polymorphism of the N-acetyltransferase 2 gene as a susceptibility risk factor for antituberculosis drug-induced hepatitis. Hepatology 35, 883–889. doi: 10.1053/jhep.2002.32102
Huang, Y. S., Chern, H. D., Su, W. J., Wu, J. C., Chang, S. C., Chiang, C. H., et al. (2003). Cytochrome P450 2E1 genotype and the susceptibility to antituberculosis drug-induced hepatitis. Hepatology 37, 924–930. doi: 10.1053/jhep.2003.50144
Hwang, J. M., Wang, C. J., Chou, F. P., Tseng, T. H., Hsieh, Y. S., Hsu, J. D., et al. (2005). Protective effect of baicalin on tert-butyl hydroperoxide-induced rat hepatotoxicity. Arch. Toxicol. 79, 102–109. doi: 10.1007/s00204-004-0588-6
Ignatowicz, E., and Baer-Dubowska, W. (2001). Resveratrol, a natural chemopreventive agent against degenerative diseases. Pol. J. Pharmacol. 53, 557–569.
Immenschuh, S., and Ramadori, G. (2000). Gene regulation of heme oxygenase-1 as a therapeutic target. Biochem. Pharmacol. 60, 1121–1128. doi: 10.1016/S0006-2952(00)00443-3
Jacob, A., Wu, R., Zhou, M., and Wang, P. (2007). Mechanism of the anti-inflammatory effect of curcumin: PPAR-gamma Activation. PPAR Res. 2007:89369. doi: 10.1155/2007/89369
Jacobson, T. A. (2006). The safety of aggressive statin therapy: how much can low-density lipoprotein cholesterol be lowered? Mayo Clin. Proc. 81, 1225–1231. doi: 10.4065/81.9.1225
Jacqz-Aigrain, E., and Anderson, B. J. (2006). Pain control: non-steroidal anti-inflammatory agents. Semin. Fetal Neonatal Med. 11, 251–259. doi: 10.1016/j.siny.2006.02.009
Jaeschke, H., Gores, G. J., Cederbaum, A. I., Hinson, J. A., Pessayre, D., and Lemasters, J. J. (2002). Mechanisms of hepatotoxicity. Toxicol. Sci. 65, 166–176. doi: 10.1093/toxsci/65.2.166
Jaggy, H., and Koch, E. (1997). Chemistry and biology of alkylphenols from Ginkgo biloba L. Pharmazie. 52, 735–738.
Jaiswal, A. K. (2000). Regulation of genes encoding NAD(P)H:quinone oxidoreductases. Free Radic. Biol. Med. 29, 254–262. doi: 10.1016/S0891-5849(00)00306-3
Joe, B., Vijaykumar, M., and Lokesh, B. R. (2004). Biological properties of Curcumin—cellular and molecular mechanisms of action. Crit. Rev. Food Sci. Nutr. 44, 97–111. doi: 10.1080/10408690490424702
Johnson, D. R., and Klaassen, C. D. (2002). Regulation of rat multidrug resistance protein 2 by classes of prototypical microsomal enzyme inducers that activate distinct transcription pathways. Toxico. Sci. 69, 317–321. doi: 10.1093/toxsci/67.2.182
Joyeux, M., Rolland, A., Fleurentin, J., Mortier, F., and Dorfman, P. (1990). Tertbutylhydroperoxide-induced injury in isolated rat hepatocytes: a model for studying antihepatotoxic crude drugs. Planta Med. 56, 171–174. doi: 10.1055/s-2006-960918
Kaplowitz, N. (2001). Drug-induced liver disorders: implications for drug development and regulation. Drug Saf. 24, 483–490. doi: 10.2165/00002018-200124070-00001
Karthikeyan, S. (2005). Isoniazid and rifampicin treatment on phospholipids and their subfractions in liver tissue of rabbits. Drug Chem. Toxicol. 28, 273–280. doi: 10.1081/DCT-200064463
Kim, D. S., Kim, D. S., and Oppel, M. N. (2002). Shogaols from Zingiber officinale protect IMR32 human neuroblastoma and normal human umbilical vein endothelial cells from beta-amyloid (25-35) insult. Planta Med. 68, 375–376. doi: 10.1055/s-2002-26757
King, J. C., and Cousins, R. J. (2006). “Zinc,” in Modern Nutrition in Health and Disease, 10th Edn, eds M. E. Shils, M. Shike, A. C. Ross, B. Caballero, and R. J. Cousins (Philadelphia, PA: Lipponcott Williams and Wilkins), 271–285.
Kissler, H. J., Hauffen, J., Hennig, R., Gepp, H., and Schwille, P. O. (2005). Glucose and lipid metabolism after liver transplantation in inbred rats: consequences of hepatic denervation. Metab. Clin. Exp. 54, 881–890. doi: 10.1016/j.metabol.2005.01.036
Kliewer, S. A. (2003). The nuclear pregnane X receptor regulates xenobiotic detoxification. J. Nutr. 133, 2444S–2447S.
Kobayashi, K., Urashima, K., Shimada, N., and Chiba, K. (2002). Substrate specificity for rat cytochrome P450 (CYP) isoforms: screening with cDNA-expressed systems of the rat. Biochem. Pharmacol. 63, 889–896. doi: 10.1016/S0006-2952(01)00843-7
Kohno, H., Tanaka, T., Kawabata, K., Hirose, Y., Sugie, S., Tsuda, H., and Mori, H. (2002). Silymarin, a naturally occurring polyphenolic antioxidant flavonoid, inhibits azoxymethane-induced colon carcinogenesis in male f344 rats. Int. J. Cancer 101, 461–468. doi: 10.1002/ijc.10625
Koster, J. F., and Slee, R. G. (1980). Lipid peroxidation of rat liver microsomes. Biochim. Biophys. Acta 620, 489–499. doi: 10.1016/0005-2760(80)90141-1
Krieglstein, J., Ausmeier, F., Elabhar, H., Lippert, K., Welsch, M., Rupalla, K., et al. (1995). Neuroprotective effects of Ginkgo biloba constituents. Eur. J. Pharm. Sci. 3, 39–48. doi: 10.1016/0928-0987(94)00073-9
Kvasnicka, F., Bíba, B., Sevcík, R., Voldrich, M., and Krátká, J. (2003). Analysis of the active components of silymarin. J. Chromatogr. A. 990, 239–245. doi: 10.1016/S0021-9673(02)01971-4
Larrey, D. (2000). Drug-induced liver diseases. J. Hepatol. 32(Suppl. 1), 77–88. doi: 10.1016/S0168-8278(00)80417-1
Larson, A. M., Polson, J., Fontana, R. J., Davern, T. J., Lalani, E., Hynan, L. S., et al. (2005). Acute liver failure study group. Acetaminophen-induced acute liver failure: results of a United States multicenter, prospective study. Hepatology 42, 1364–1372. doi: 10.1002/hep.20948
Latruffe, N., Delmas, D., Jannin, B., Cherkaoui-Malki, M., Passilly-Degrace, P., and Berlot, J. P. (2002). Molecular analysis on the chemopreventive properties of resveratrol a plant polyphenol microcomponent. Int. J. Mol. Med. 10, 755–760. doi: 10.3892/ijmm.10.6.755
Lee, H. I., McGregor, R. A., Choi, M. S., Seo, K. I., Jung, U. J., Yeo, J., et al. (2013). Low doses of curcumin protect alcohol-induced liver damage by modulation of the alcohol metabolic pathway, CYP2E1 and AMPK. Life Sci. 93, 693–699. doi: 10.1016/j.lfs.2013.09.014
Lee, J. S., and Surh, Y. J. (2005). Nrf2 as a novel molecular target for chemoprevention. Cancer Lett. 224, 171–184. doi: 10.1016/j.canlet.2004.09.042
Lee, W. M. (2003). Drug-induced hepatotoxicity. N. Engl. J. Med. 349, 474–485. doi: 10.1056/NEJMra021844
Lehmann, J. M., McKee, D. D., Watson, M. A., Willson, T. M., Moore, J. T., and Kliewer, S. A. (1998). The human orphan nuclear receptor PXR is activated by compounds that regulate CYP3A4 gene expression and cause drug interactions. J. Clin. Invest. 102, 1016–1023. doi: 10.1172/JCI3703
Lewis, J. H., and Zimmerman, H. J. (1989). Drug-induced liver disease. Med. Clin. North. Am. 73, 775–792.
Lichtblau, D., Berger, J. M., and Nakanishi, K. (2002). Efficient extraction of ginkgolides and bilobalide from Ginkgo biloba leaves. J. Nat. Prod. 65, 1501–1504. doi: 10.1021/np0201974
Lin, T. H., Ng, L. T., Yen, F. L., and Lin, C. C. (2007). Hepatoprotective effects of Chai-Hu-Ching-Kan-Tang on acetaminophen-induced acute liver injury in rats. Am. J. Chin. Med. 35, 69–79. doi: 10.1142/S0192415X07004631
Liu, C. T., Chuang, P. T., Wu, C. Y., Weng, Y. M., Chen, W., and Tseng, C. Y. (2006). Antioxidative and in vitro hepatoprotective activity of Bupleurum kaoi leaf infusion. Phytother. Res. 20, 1003–1008. doi: 10.1002/ptr.1946
Lu, H., and Liu, G. T. (1992). Anti-oxidant activity of dibenzocyclooctene lignans isolated from Schisandraceae. Planta Med. 58, 311–313. doi: 10.1055/s-2006-961473
Madrigal-Santillán, E., Madrigal-Bujaidar, E., Álvarez-González, I., Sumaya-Martínez, M. T., Gutiérrez-Salinas, J., Bautista, M., et al. (2014). Review of natural products with hepatoprotective effects. World J. Gastroenterol. 20, 14787–14804. doi: 10.3748/wjg.v20.i40.14787
Madrigal-Santillán, E., Madrigal-Bujaidar, E., Cruz-Jaime, S., Valadez-Vega, M. C., Sumaya-Martínez, M. T., Pérez-Ávila, K. G., et al. (2013). “The chemoprevention of chronic degenerative disease through dietary antioxidants: progress, promise and evidences,” in Oxidative Stress and Chronic Degenerative Diseases-a Role for Antioxidants, ed J. A. Morales-González (Rijeka: Croatia InTech), 155–185.
Mahady, G. B. (2002). Ginkgo biloba for the prevention and treatment of cardiovascular disease: a review of the literature. J. Cardiovas. Nurs. 16, 21–32. doi: 10.1097/00005082-200207000-00004
Maheshwari, R. K., Singh, A. K., Gaddipati, J., and Srimal, R. C. (2006). Multiple biological activities of curcumin: a short review. Life Sci. 78, 2081–2087. doi: 10.1016/j.lfs.2005.12.007
Mangelsdorf, D. J., and Evans, R. M. (1995). The RXR heterodimers and orphan receptors. Cell 83, 841–850. doi: 10.1016/0092-8674(95)90200-7
Markham, K. R., and Mitchell, K. A. (2003). The mis-identification of the major antioxidant flavonoids in young barley (Hordeum vulgare) leaves. Z. Naturforsch. C 58, 53–56. doi: 10.1515/znc-2003-1-209
Masson, M. J., Peterson, R. A., Chung, C. J., Graf, M. L., Carpenter, L. D., Ambroso, J. L., et al. (2007). Lymphocyte loss and immunosuppression following acetaminophen-induced hepatotoxicity in mice as a potential mechanism of tolerance. Chem. Res. Toxicol. 20, 20–26. doi: 10.1021/tx060190c
Masubuchi, N., Makino, C., and Murayama, N. (2007). Prediction of in vivo potential for metabolic activation of drugs into chemically reactive intermediate: correlation of in vitro and in vivo generation of reactive intermediates and in vitro glutathione conjugate formation in rats and humans. Chem. Res. Toxicol. 20, 455–464. doi: 10.1021/tx060234h
McGill, M. R., Sharpe, M. R., Williams, C. D., Taha, M., Curry, S. C., and Jaeschke, H. (2012). The mechanism underlying acetaminophen-induced hepatotoxicity in humans and mice involves mitochondrial damage and nuclear DNA fragmentation. J. Clin. Invest. 122, 1574–1583. doi: 10.1172/JCI59755
McKenna, D. J., Jones, K., and Hughes, K. (2001). Efficacy, safety, and use of ginkgo biloba in clinical and preclinical applications. Altern. Ther. Health Med. 7, 70–86.
Miguez, M. P., Anundi, I., Sainz-Pardo, L. A., and Lindros, K. O. (1994). Comparative study of the hepatoprotective effect of silymarin and silybin on isolated rat hepatocytes. Toxicol. In Vitro 8, 581–583. doi: 10.1016/0887-2333(94)90021-3
Mikstacka, R., Gnojkowski, J., and Baer-Dubowska, W. (2002). Effect of natural phenols on the catalytic activity of cytochrome P4502E1. Acta Biochim. Pol. 49, 917–925.
Mindie, H. N., and Gabriel, G. (2002). Does isoniazid cause more serious hepatotoxicity in hepatitis B virus carriors? Am. J. Gastroenterol. 97, 1092–1093. doi: 10.1111/j.1572-0241.2002.05700.x
Mira, L., Silva, M., and Manso, C. F. (1994). Scavenging of reactive oxygen species by silibinin hemisuccinate. Biochem. Pharmacol. 48, 753–759. doi: 10.1016/0006-2952(94)90053-1
Mitchell, J. R., Jollow, D. J., Potter, W. Z., Davis, D. C., Gillette, J. R., and Brodie, B. B. (1973). Acetaminophen-induced hepatic necrosis. I. Role of drug metabolism. J. Pharmacol. Exp. Ther. 187, 185–194.
Mitchell, J. R., Thorgeirsson, U. P., Black, M., Timbrell, J. A., Snodgrass, W. R., Potter, W. Z., et al. (1975). Increased incidence of isoniazid hepatitis in rapid acetylators: possible relation to hydrazine metabolites. Clin. Pharmacol. Ther. 18, 70–79.
Mitchell, S. J., and Hilmer, S. N. (2010). Drug-induced liver injury in older adults. Ther. Adv. Drug Saf. 1, 65–77. doi: 10.1177/2042098610386281
Mitka, M. (2014). FDA asks physicians to stop prescribing high-dose acetaminophen products. JAMA 311, 563. doi: 10.1001/jama.2014.716
Mochizuki, M., Yamazaki, S., Kano, K., and Ikeda, T. (2002). Kinetic analysis and mechanistic aspects of autooxidation of catechins. Biochim. Biophys. Acta 1569, 35–44. doi: 10.1016/S0304-4165(01)00230-6
Mohapatra, R., Tran, M., Gore, J. M., and Spencer, F. A. (2005). A review of the oral direct thrombin inhibitor ximelagatran: not yet the end of the warfarin era. Am. Heart J. 150, 19–26. doi: 10.1016/j.ahj.2005.02.012
Moon, Y. J., Wang, X., and Morris, M. E. (2006). Dietary flavonoids: effects on xenobiotic and carcinogen metabolism. Toxicol. In Vitro 20, 187–210. doi: 10.1016/j.tiv.2005.06.048
Motohashi, H., and Yamamoto, M. (2004). Nrf2–Keap1 defines a physiologically important stress response mechanism. Trends Mol. Med. 10, 549–557. doi: 10.1016/j.molmed.2004.09.003
Muriel, P., and Mourelle, M. (1990). Prevention by silymarin of membrane alterations in acute CCl4 liver damage. J. Appl. Toxicol. 10, 275–279. doi: 10.1002/jat.2550100408
Nasri, H., Sahinfard, N., Rafieian, M., Rafieian, S., Shirzad, M., and Rafieian-Kopaei, M. (2013). Effects of Allium sativum on liver enzymes and atherosclerotic risk factors. J. Herb. Med. Pharmacol. 2, 23–28.
Nelson, D. R., Koymans, L., Kamataki, T., Stegeman, J. J., Feyereisen, R., Waxman, D. J., et al. (1996). P450 superfamily: update on new sequences, gene mapping, accession numbers and nomenclature. Pharmacogenetics 6, 1–42. doi: 10.1097/00008571-199602000-00002
Okada, K., Shoda, J., Kano, M., Suzuki, S., Ohtake, N., Yamamoto, M., et al. (2007). Inchinkoto, a herbal medicine, and its ingredients dually exert Mrp2/MRP2-mediated choleresis and Nrf2-mediated antioxidative action in rat livers. Am. J. Physiol. Gastrointest. Liver Physiol. 292, G1450–G1463. doi: 10.1152/ajpgi.00302.2006
Oken, B. S., Storzbach, D. M., and Kaye, J. A. (1998). The efficacy of Ginkgo biloba on cognitive function in Alzheimer disease. Arch. Neurol. 55, 1409–1415. doi: 10.1001/archneur.55.11.1409
Olaleye, M. T., and Rocha, B. T. (2008). Acetaminophen-induced liver damage in mice: effects of some medicinal plants on the oxidative defense system. Exp. Toxicol. Pathol. 59, 319–327. doi: 10.1016/j.etp.2007.10.003
Ostapowicz, G., Fontana, R. J., Schiødt, F. V., Larson, A., Davern, T. J., Han, S. H., et al. (2002). Results of a prospective study of acute liver failure at 17 tertiary care centers in the United States. Ann. Intern. Med. 137, 947–954. doi: 10.7326/0003-4819-137-12-200212170-00007
Pal, R., Vaiphei, K., Sikander, A., Singh, K., and Rana, S. V. (2006). Effect of garlic on isoniazid and rifampicin-induced hepatic injury in rats. World J. Gastroenterol. 12, 636–639.
Pan, M. H., Huang, T. M., and Lin, J. K. (1999). Biotransformation of curcumin through reduction and glucuronidation in mice. Drug Metabol. Dispos. 27, 486–494.
Pandit, A., Sachdeva, T., and Bafna, P. (2012). Drug-induced hepatotoxicity: a review. J. Appl. Pharm. Sci. 02, 233–243. doi: 10.7324/japs.2012.2541
Park, B. K., Kitteringham, N. R., Maggs, J. L., Pirmohamed, M., and Williams, D. P. (2005). The role of metabolic activation in drug-induced hepatotoxicity. Ann. Rev. Pharmacol. Toxicol. 45, 177–202. doi: 10.1146/annurev.pharmtox.45.120403.100058
Plummer, S. M., Holloway, K. A., Manson, M. M., Munks, R. J. L., Kaptein, A., Farrow, S., et al. (1999). Inhibition of cyclooxygenase 2 expression in colon cells by the chemopreventive agent curcumin involves inhibition of NF- kappa B activation via the NIK/IKK signalling complex. Oncogene 18, 6013–6020. doi: 10.1038/sj.onc.1202980
Polyak, S. J., Morishima, C., Shuhart, M. C., Wang, C. C., Liu, Y., and Lee, D. Y. (2007). Inhibition of T-cell inflammatory cytokines, hepatocyte NF-kappaB signaling, and HCV infection by standardized Silymarin. Gastroenterology 132, 1925–1936. doi: 10.1053/j.gastro.2007.02.038
Ponto, L. L., and Schultz, S. K. (2003). Ginkgo biloba extract: review of CNS effects. Ann. Clin. Psychiatry 15, 109–119. doi: 10.3109/10401230309085676
Porceddu, M., Buron, N., Roussel, C., Labbe, G., Fromenty, B., and Borgne-Sanchez, A. (2012). Prediction of liver injury induced by chemicals in human with a multiparametric assay on isolated mouse liver mitochondria. Toxicol. Sci. 129, 332–345. doi: 10.1093/toxsci/KFS197
Potter, G. A., Patterson, L. H., Wanogho, E., Perry, P. J., Butler, P. C., Ijaz, T., et al. (2002). The cancer preventative agent resveratrol is converted to the anticancer agent piceatannol by the cytochrome P450 enzyme CYP1B1. Br. J. Cancer 86, 774–778. doi: 10.1038/sj.bjc.6600197
Powell-Jackson, P. R., Tredger, J. M., Smith, H. W., and Williams, R. (1982). Effect of isoniazid administration on selected rat and mouse hepatic microsomal mixed-function oxidase and in vitro (C14) acetylhydrazine-derived covalent binding. Biochem. Pharmacol. 31, 4031–4034. doi: 10.1016/0006-2952(82)90651-7
Racknagel, R. O., Glende, E. J. R., Dolak, J. A., and Waller, R. I. (1989). Mechanisms of carbon tetrachloride toxicity. Pharmacol. Ther. 43, 130–154. doi: 10.1016/0163-7258(89)90050-8
Ramachandran, P. C. (1980). Chemotherapy of tubercular meningitis with isoniazid plus rifampicin. Interim findings in a trial in children. Ind. J. Tuberc. 27, 54–56.
Rana, S. V., Attri, S., Vaiphei, K., Pal, R., Attri, A., and Singh, K. (2006). Role of N-acetylcysteine in rifampicin-induced hepatic injury of young rats. World J. Gastroenterol. 12, 287–291. doi: 10.3748/wjg.v12.i2.287
Raucy, J. L., Lasker, J. M., Lieber, C. S., and Black, M. (1989). Acetaminophen ctivation by human liver cytochromes P450IIE1 and P450IA2. Arch. Biochem. Biophys. 271, 270–283. doi: 10.1016/0003-9861(89)90278-6
Regev-Shoshani, G., Shoseyov, O., and Kerem, Z. (2004). Influence of lipophilicity on the interactions of hydroxy stilbenes with cytochrome P450 3A4. Biochem. Biophys. Res. Commun. 323, 668–673. doi: 10.1016/j.bbrc.2004.08.141
Reid, A. B., Kurten, R. C., McCullough, S. S., Brock, R. W., and Hinson, J. A. (2005). Mechanisms of acetaminophen-induced hepatotoxicity: role of oxidative stress and mitochondrial permeability transition in freshly isolated mouse hepatocytes. J. Pharmacol. Exp. Ther. 312, 509–516. doi: 10.1124/jpet.104.075945
Rendic, S. (2002). Summary of information on human CYP enzymes: human P450 metabolism data. Drug Metabol. Rev. 34, 83–448. doi: 10.1081/DMR-120001392
Rezvani, A. H., Overstreet, D. H., Perfumi, M., and Massi, M. (2003). Plant derivatives in the treatment of alcohol dependency. Pharmacol. Biochem. Behav. 75, 593–606. doi: 10.1016/S0091-3057(03)00124-2
Ross, D. (1988). Glutathione, free radicals and chemotherapeutic agents. Pharmacol. Ther. 37, 231–249. doi: 10.1016/0163-7258(88)90027-7
Saito, C., Lemasters, J. J., and Jaeschke, H. (2010). c-Jun N-terminal kinase modulates oxidant stress and peroxynitrite formation independent of inducible nitric oxide synthase in acetaminophen hepatotoxicity. Toxicol. Appl. Pharmacol. 246, 8–17. doi: 10.1016/j.taap.2010.04.015
Sandur, S. K., Pandey, M. K., Sung, B., Ahn, K. S., Murakami, A., Sethi, G., et al. (2007). Curcumin, demethoxycurcumin, bisdemethoxycurcumin, tetrahydrocurcumin and turmerones differentially regulate anti-inflammatory and anti-proliferative responses through a ROS-independent mechanism. Carcinogenesis 28, 1765–1773. doi: 10.1093/carcin/bgm123
Sankar, P., Gopal Telang, A., Kalaivanan, R., Karunakaran, V., Manikam, K., and Sarkar, S. N. (2015). Effects of nanoparticle-encapsulated curcumin on arsenic-induced liver toxicity in rats. Environ. Toxicol. 30, 628–637. doi: 10.1002/tox.21940
Santhosh, S., Sini, T. K., Anandan, R., and Mathew, P. T. (2006). Effect of chitosan supplementation on antitubercular drugs-induced hepatotoxicity in rats. Toxicology 219, 53–59. doi: 10.1016/j.tox.2005.11.001
Saraswathy, S. D., and Shyamala Devi, C. S. (2001). Modulating effect of Liv.100, an ayurvedic formulation on antituberculosis drug-induced alterations in rat liver microsomes. Phytother. Res. 15, 501–505. doi: 10.1002/ptr.770
Sass, G., Soares, M. C., Yamashita, K., Seyfried, S., Zimmermann, W. H., Eschenhagen, T., et al. (2003). Heme oxygenase-1 and its reaction product, carbon monoxide, prevent inflammation-related apoptotic liver damage in mice. Hepatology 38, 909–918. doi: 10.1002/hep.1840380417
Saukkonen, J. J., Cohn, D. L., Jasmer, R. M., Schenker, S., Jereb, J. A., Nolan, C. M., et al. (2006). ATS (American Thoracic Society) hepatotoxicity of antituberculosis therapy subcommittee. An official ATS statement: hepatotoxicity of antituberculosis therapy. Am. J. Respir. Crit. Care Med. 174, 935–952. doi: 10.1164/rccm.200510-1666ST
Scambia, G., De Vincenzo, R., Ranelletti, F. O., Panici, P. B., Ferrandina, G., D'Agostino, G., et al. (1996). Antiproliferative effect of silybin on gynaecological malignancies: synergism with cisplatin and doxorubicin. Eur. J. Cancer 32A, 877–882. doi: 10.1016/0959-8049(96)00011-1
Schneider, R., Welt, K., Aust, W., Löster, H., and Fitzl, G. (2008). Cardiac ischemia and reperfusion in spontaneously diabetic rats with and without application of EGb 761: I. cardiomyocytes. Histol. Histopathol. 23, 807–817.
Shah, R. R. (1999). Drug-induced hepatotoxicity: pharmacokinetic perspectives and strategies for risk reduction. Adv. Drug React. Toxicol. Rev. 18, 181–233.
Shapiro, M. A., and Lewis, J. H. (2007). Causality assessment of drug-induced hepatotoxicity: promises and pitfalls. Clin. Liver Dis. 11, 477. doi: 10.1016/j.cld.2007.06.003
Sheu, S. Y., Lai, C. H., and Chiang, H. C. (1998). Inhibition of xanthine oxidase by purpurogallin and silymarin group. Anticancer Res. 18, 263–268.
Shimizu, I. (2001). Antifibrogenic therapies in chronic HCV infection. Curr. Drug Targets Infect. Disord. 1, 227–240. doi: 10.2174/1568005014606053
Singh, V., Ahmad, S., and Rao, G. S. (1994). Prooxident antioxident properties of iron-hydroquinone and iron-1,2,4-benzenetriol complex. Implications for benzene toxicity. Toxicology 89, 25–33. doi: 10.1016/0300-483X(94)90130-9
Sinha, B. K. (1987). Activation of hydrazine derivatives of free radicals in the per fused rat liver: a spin trapping study. Biochim. Biophys. Acta 924, 261–269. doi: 10.1016/0304-4165(87)90021-3
Snawder, J. E., Roe, A. L., Benson, R. W., and Roberts, D. W. (1994). Loss of CYP2E1 and CYP1A2 activity as a function of acetaminophen dose: relation to toxicity. Biochem. Biophys. Res. Commun. 203, 532–539. doi: 10.1006/bbrc.1994.2215
Sodhi, C. P., Rana, S. F., Attari, S., Metha, S., Vaiphei, K., and Metha, S. K. (1998). Oxidative hepatic injury of isoniazid-rifampicin in young rats subjected to protein energy malnutrition. Drug Chem. Toxicol. 21, 305–317. doi: 10.3109/01480549809002207
Sodhi, C. P., Rana, S. V., Mehta, S. K., Vaiphei, K., Attri, S., Thakur, S., et al. (1996). Study of oxidative stress in isoniazid-induced hepatic injury in young rats with and without protein-energy malnutrition. J. Biochem. Toxicol. 11, 139–146.
Soliman, M. M., Abdo Nassan, M., and Ismail, T. A. (2014). Immunohistochemical and molecular study on the protective effect of curcumin against hepatictoxicity induced by paracetamol in wistar rats. BMC Complement. Altern. Med. 29, 14:457. doi: 10.1186/1472-6882-14-457
Soliman, M. M., Baiomy, A. A., and Yassin, M. H. (2015). Molecular and histopathological study on the ameliorative effects of curcumin against lead acetate-induced hepatotoxicity and nephrototoxicity in wistar rats. Biol. Trace Elem. Res. 167, 91–102. doi: 10.1007/s12011-015-0280-0
Song, I. S., Lee, Y. M., Chung, S. J., and Shim, C. K. (2003). Multiple alterations of canalicular membrane transport activities in rats with CCl(4)-induced hepatic injury. Drug Metab. Dispos. 31, 482–490. doi: 10.1124/dmd.31.4.482
Sonnenbichler, J., Scalera, F., Sonnenbichler, I., and Weyhenmeyer, R. (1999). Stimulatory effects of silibinin and silicristin from the milk thistle Silybum marianum on kidney cells. J. Pharmacol. Exp. Ther. 290, 1375–1383.
Sonnenbichler, J., and Zetl, I. (1986). Biochemical effects of the flavolignane silibinin on RNA, protein and DNA synthesis in rat liver. Progr. Clin. Biol. Res. 213, 319–331.
Sonoda, J., Rosenfeld, J. M., Xu, L., Evans, R. M., and Xie, W. (2003). A nuclear receptor-mediated xenobiotic response and its implication in drug metabolism and host protection. Curr. Drug Metab. 4, 59–72. doi: 10.2174/1389200033336739
Srivastava, P., Prabhu, V. V., Yadav, N., Gogada, R., and Chandra, D. (2013). “Effect of dietary resveratrol in the treatment of cancer,” in Cancer Chemoprevention and Treatment by Diet Therapy, ed W. C. Cho (New York, NY: Springer), 1–22.
Staudinger, J. L., Ding, X., and Lichti, K. (2006). Pregnane X receptor and natural products: beyond drug-drug interactions. Expert Opin. Drug Metab. Toxicol. 2, 847–857. doi: 10.1517/17425255.2.6.847
Steele, V. E., Kelloff, G. J., Wilkinson, B. P., and Arnold, J. T. (1990). Inhibition of transformation in cultured rat tracheal epithelial cells by potential chemopreventive agents. Cancer Res. 50, 2068–2074.
Stickel, F., and Shouval, D. (2015). Hepatotoxicity of herbal and dietary supplements: an update. Arch. Toxicol. 89, 851–865. doi: 10.1007/s00204-015-1471-3
Surh, Y.-J. (1999). Molecular mechanisms of chemopreventive effects of selected dietary and medicinal phenolic substances. Mutat. Res. 428, 305–327. doi: 10.1016/S1383-5742(99)00057-5
Tanaka, S., Haruma, K., Kunihiro, M., Nagata, S., Kitadai, Y., Manabe, N., et al. (2004). Effects of aged garlic extract (AGE) on colorectal adenomas: a double-blinded study. Hiroshima J. Med. Sci. 53, 39–45.
Tang, Y., Lou, F., Wang, J., Li, Y., and Zhuang, S. (2001). Coumaroyl flavonol glycosides from the leaves of Ginkgo biloba. Phytochemistry 58, 1251–1256. doi: 10.1016/S0031-9422(01)00320-X
Tasduq, S. A., Peerzada, K., Koul, S., Bhat, R., and Johri, R. K. (2005). Biochemical manifestations of anti-tuberculosis drugs induced hepatotoxicity and the effect of silymarin. Hepatol. Res. 31, 132–135. doi: 10.1016/j.hepres.2005.01.005
Thor, H., and Orrenius, S. (1980). The mechanism of bromobenzene-induced cytotoxicity studied with isolated hepatocytes. Arch. Toxicol. 44, 31–43. doi: 10.1007/BF00303181
Trackshel, G. M., and Maines, M. D. (1988). Characterization of glutathione S- transferase in rat kidney. Biochem. J. 252, 127–136. doi: 10.1042/bj2520127
Tseng, S. H., Chien, T. Y., Tzeng, C. F., Lin, Y. H., Wu, C. H., and Wang, C. C. (2007). Prevention of hepatic oxidative injury by Xiao-Chen-Chi-Tang in mice. J. Ethnopharmacol. 111, 232–239. doi: 10.1016/j.jep.2006.11.030
Tseng, T. H., Chu, C. Y., Huang, J. M., Shiow, S. J., and Wang, C. J. (1995). Crocetin protects against oxidative damage in rat primary hepatocytes. Cancer Lett. 97, 61–67. doi: 10.1016/0304-3835(95)03964-X
Upadhyay, G., Gupta, S. P., Prakash, O., and Singh, M. P. (2010a). Pyrogallol-mediated toxicity and natural antioxidants: triumphs and pitfalls of preclinical findings and their translational limitations. Chem. Biol. Interact. 183, 333–340. doi: 10.1016/j.cbi.2009.11.028
Upadhyay, G., Kumar, A., and Singh, M. P. (2007). Effect of silymarin on pyrogallol- and rifampicin-induced hepatotoxicity in mouse. Eur. J. Pharmacol. 565, 190–201. doi: 10.1016/j.ejphar.2007.03.004
Upadhyay, G., Singh, A. K., Kumar, A., Prakash, O., and Singh, M. P. (2008). Resveratrol modulates pyrogallol-induced changes in hepatic toxicity markers, xenobiotic metabolizing enzymes and oxidative stress. Eur. J. Pharmacol. 596, 146–152. doi: 10.1016/j.ejphar.2008.08.019
Upadhyay, G., Tiwari, M. N., Prakash, O., Jyoti, A., Shanker, R., and Singh, M. P. (2010b). Involvement of multiple molecular events in pyrogallol-induced hepatotoxicity and silymarin-mediated protection: evidence from gene expression profiles. Food Chem. Toxicol. 48, 1660–1670. doi: 10.1016/j.fct.2010.03.041
Urquhart, B. L., Tirona, R. G., and Kim, R. B. (2007). Nuclear receptors and the regulation of drug-metabolizing enzymes and drug transporters: implications for interindividual variability in response to drugs. J. Clin. Pharmacol. 47, 566–578. doi: 10.1177/0091270007299930
Vajdovich, P., Szilagyi, A., and Gall, T. (1995). Evaluating of blood lipid peroxidation parameters in carbon tetrachloride (CCl4) toxicity in sheep. Acta Vet. Hung. 43, 423–429.
Valenzuela, A., Guerra, R., and Garrido, A. (1987). Silybin dihemisuccinate protects rat erythrocytes against phenylhydrazine-induced lipoperoxidation and hemolysis. Planta Med. 53, 402–405. doi: 10.1055/s-2006-962757
Van Beek, T. A. (2002). Chemical analysis of Ginkgo biloba leaves and extracts. J. Chromatogr. 967, 21–55. doi: 10.1016/S0021-9673(02)00172-3
Vessey, D. A. (1996). “Metabolism of xenobiotics by the human liver,” in Hepatology: A Textbook of Liver Disease, 3rd Edn., eds D. Zakin and T. D. Boyer (Philadelphia: WB Saunders), 257–305.
Wang, R., Kong, J., Wang, D., Lin-min Lien, L., and Lien, E. J. (2007). A survey of Chinese herbal ingredients with liver protection activities. Chin. Med. 2:5. doi: 10.1186/1749-8546-2-5
Wang, Y., Jiang, Y., Fan, X., Tan, H., Zeng, H., Wang, Y., et al. (2015). Hepato-protective effect of resveratrol against acetaminophen-induced liver injury is associated with inhibition of CYP-mediated bioactivation and regulation of SIRT1–p53 signaling pathways. Toxicol. Lett. 236, 82–89. doi: 10.1016/j.toxlet.2015.05.001
Waseem, M., Pandey, P., Tomar, B., Raisuddin, S., and Parvez, S. (2014). Ameliorative action of curcumin in cisplatin-mediated hepatotoxicity: an in vivo study in Wistar rats. Arch. Med. Res. 45, 462–468. doi: 10.1016/j.arcmed.2014.07.006
Watanabe, C. M., Wolffram, S., Ader, P., Rimbach, G., Packer, L., Maguire, J. J., et al. (2001). The in vivo neuromodulatory effects of the herbal medicine ginkgo biloba. Proc. Natl. Acad. Sci. U.S.A. 98, 6577–6580. doi: 10.1073/pnas.111126298
Waxman, D. J. (1999). P450 gene induction by structurally diverse xenochemicals: central role of nuclear receptors CAR, PXR, and PPAR. Arch. Biochem. Biophys. 369, 11–23. doi: 10.1006/abbi.1999.1351
Wellington, K., and Jarvis, B. (2001). Silymarin: a review of its clinical properties in the management of hepatic disorders. BioDrugs 15, 465–489. doi: 10.2165/00063030-200115070-00005
Wesnes, K. A., Ward, T., McGinty, A., and Petrini, O. (2000). The memory enhancing effects of a Ginkgo biloba/Panax ginseng combination in healthy middle-aged volunteers. Psychopharmacology 152, 353–361. doi: 10.1007/s002130000533
Wolf, K. K., Wood, S. G., Allard, J. L., Hunt, J. A., Gorman, N., Walton-Strong, B. W., et al. (2007). Role of CYP3A and CYP2E1 in alcohol-mediated increases in acetaminophen hepatotoxicity: comparison of wild-type and Cyp2e1(-/-) mice. Drug Metab. Dispos. 35, 1223–1231. doi: 10.1124/dmd.107.014738
Woods, C. G., Vanden Heuvel, J. P., and Rusyn, I. (2007). Genomic profiling in nuclear receptor-mediated toxicity. Toxicol. Pathol. 35, 474–494. doi: 10.1080/01926230701311351
Wood, G. C., and Woodcock, B. G. (1970). Effects of dietary protein deficiency on the conjugation of foreign compounds in rat liver. J. Pharm. Pharmacol. Suppl:60S+. doi: 10.1111/j.2042-7158.1970.tb08581.x
Wu, S. J., Wang, J. S., Lin, C. C., and Chang, C. H. (2001). Evaluation of hepatoprotective activity of legumes. Phytomedicine 8, 213–219. doi: 10.1078/0944-7113-00033
Wu, Y. G., Xia, L. L., Lin, H., Zhou, D., Qian, H., and Lin, S. T. (2007b). Prevention of early liver injury by breviscapine in streptozotocin-induced diabetic rats. Planta Med. 73, 433–438. doi: 10.1055/s-2007-967182
Wu, Y., Li, L., Wen, T., and Li, Y. Q. (2007a). Protective effects of echinacoside on carbon tetrachloride-induced hepatotoxicity in rats. Toxicology 232, 50–56. doi: 10.1016/j.tox.2006.12.013
Xu, J. K., Hiroshi, K., Zheng, J. J., Jiang, T., and Yao, X. S. (2006). Protective effect of tanshinones against liver injury in mice loaded with restraint stress. Yao Xue Xue Bao 41, 631–635.
Yamazaki, Y., Kakizaki, S., Horiguchi, N., Takagi, H., Mori, M., and Negishi, M. (2005). Role of nuclear receptor CAR in carbon tetrachloride-induced hepatotoxicity. World J. Gastroenterol. 11, 5966–5972. doi: 10.3748/wjg.v11.i38.5966
Yang, L., Wang, C. Z., Ye, J. Z., and Li, H. T. (2011). Hepatoprotective effects of polyprenols from Ginkgo biloba L. leaves on CCl4-induced hepatotoxicity in rats. Fitoterapia 82, 834–840. doi: 10.1016/j.fitote.2011.04.009
Yang, M., Xu, D. D., Zhang, Y., Liu, X., Hoeven, R., and Cho, W. C. (2014a). A systematic review on natural medicines for the prevention and treatment of Alzheimer's disease with meta-analyses of intervention effect of ginkgo. Am. J. Chin. Med. 42, 505–521. doi: 10.1142/S0192415X14500335
Yang, Z., Zhuang, L., Lu, Y., Xu, Q., and Chen, X. (2014b). Effects and tolerance of silymarin (milk thistle) in chronic hepatitis C virus infection patients: a meta-analysis of randomized controlled trials. Biomed Res Int. 2014:941085. doi: 10.1155/2014/941085
Yarnell, E., and Abascal, K. (2007). Interaction of herbal constituents with cytochrome P450 Enz. Alternat. Complement. Ther. 13, 239–247. doi: 10.1089/act.2007.13508
Yin, F., Liu, J., Ji, X., Wang, Y., Zidichouski, J., and Zhang, J. (2011). Silibinin: a novel inhibitor of Aβ aggregation. Neurochem. Int. 58, 399–403. doi: 10.1016/j.neuint.2010.12.017
Young, J. F., Dragsted, L. O., Daneshvar, B., Lauridsen, S. T., Hansen, M., and Sandström, B. (2000). The effect of grape-skin extract on oxidative status. Br. J. Nutr. 84, 505–513.
Young, S. C., Wang, C. J., Lin, J. J., Peng, P. L., Hsu, J. L., and Chou, F. P. (2007). Protection effect of piper betel leaf extract against carbon tetrachloride-induced liver fibrosis in rats. Arch. Toxicol. 81, 45–55. doi: 10.1007/s00204-006-0106-0
Yu, C. Y., Kim, S. H., Lim, J. D., Kim, M. J., and Chung, I. M. (2003). Intraspecific relationship analysis by DNA markers and in vitro cytotoxic and antioxidant activity in Eleutherococcus senticosus. Toxicol. In Vitro 17, 229–236. doi: 10.1016/S0887-2333(03)00008-0
Zhang, Z., Gao, L., Cheng, Y., Jiang, J., Chen, Y., Jiang, H., et al. (2014). Resveratrol, a natural antioxidant, has a protective effect on liver injury induced by inorganic arsenic exposure. Biomed. Res. Int. 2014:617202. doi: 10.1155/2014/617202
Zhong, M. M., Chen, F. H., Yuan, L. P., Wang, X. H., Wu, F. R., Yuan, F. L., et al. (2007). Protective effect of total flavonoids from Bidens bipinnata L. against carbon tetrachloride-induced liver injury in mice. J. Pharm. Pharmacol. 59, 1017–1025. doi: 10.1211/jpp.59.7.0015
Zi, X., and Agarwal, R. (1999). Silibinin decreases prostate-specific antigen with cell growth inhibition via G1 arrest, leading to differentiation of prostate carcinoma cells: implications for prostate cancer intervention. Proc. Natl. Acad. Sci. U.S.A. 96, 7490–7495. doi: 10.1073/pnas.96.13.7490
Zi, X., Grasso, A. W., Kung, H.-J., and Agarwal, R. (1998). A flavonoid antioxidant, silymarin, inhibits activation of erbB1 signaling and induces cyclindependent kinase inhibitors, G1 arrest, and anticarcinogenic effects in human prostate carcinoma DU145 cells. Cancer Res. 58, 1920–1929.
Zi, X., Mukhtar, H., and Agarwal, R. (1997). Novel cancer chemopreventive effects of a flavonoid antioxidant silymarin: inhibition of m RNA expression of an endogenous tumor promoter TNF-α. Biochem. Biophys. Res. Commun. 239, 334–339. doi: 10.1006/bbrc.1997.7375
Keywords: antioxidant, hepatoprotection, hepatotoxicity, herbal medicine
Citation: Singh D, Cho WC and Upadhyay G (2016) Drug-Induced Liver Toxicity and Prevention by Herbal Antioxidants: An Overview. Front. Physiol. 6:363. doi: 10.3389/fphys.2015.00363
Received: 02 September 2015; Accepted: 16 November 2015;
Published: 26 January 2016.
Edited by:
Honglei Weng, University of Heidelberg, GermanyReviewed by:
Sandeep Kumar, State University of New York College of Optometry, USAIsrar Ahmad, University of Alabama at Birmingham, USA
Copyright © 2016 Singh, Cho and Upadhyay. This is an open-access article distributed under the terms of the Creative Commons Attribution License (CC BY). The use, distribution or reproduction in other forums is permitted, provided the original author(s) or licensor are credited and that the original publication in this journal is cited, in accordance with accepted academic practice. No use, distribution or reproduction is permitted which does not comply with these terms.
*Correspondence: William C. Cho, d2lsbGlhbWNzY2hvQGdtYWlsLmNvbQ==;
Ghanshyam Upadhyay, dXBhZGh5YXlpaXRyQGdtYWlsLmNvbQ==