- 1Université de Nice, UMR 7277, Nice, France
- 2Institut de Biologie de Valrose, CNRS UMR 7277, INSERM U1091, Université de Nice, Nice, France
Originally mistaken as an opioid receptor, the sigma-1 receptor (Sig1R) is a ubiquitous membrane protein that has been involved in many cellular processes. While the precise function of Sig1R has long remained mysterious, recent studies have shed light on its role and the molecular mechanisms triggered. Sig1R is in fact a stress-activated chaperone mainly associated with the ER-mitochondria interface that can regulate cell survival through the control of calcium homeostasis. Sig1R functionally regulates ion channels belonging to various molecular families and it has thus been involved in neuronal plasticity and central nervous system diseases. Interestingly, Sig1R is frequently expressed in tumors but its function in cancer has not been yet clarified. In this review, we discuss the current understanding of Sig1R. We suggest herein that Sig1R shapes cancer cell electrical signature upon environmental conditions. Thus, Sig1R may be used as a novel therapeutic target to specifically abrogate pro-invasive functions of ion channels in cancer tissue.
Introduction
The concept of “Sigma receptors” arose 40 years ago in a pharmacological study postulating the existence of three types of opioid receptors on the basis of the psychomimetic effects induced by several opioid compounds (μ, κ and σ receptor respectively accounting for the effects produced by morphine, ketacyclazocine and SKF 10,047) (Martin et al., 1976). Further pharmacological studies revealed the existence of two binding sites, namely Sigma 1 (Sig1R) and Sigma 2 receptors (Sig2R) (Quirion et al., 1992). The Sig1R was cloned in 1996 (gene names: SIGMAR1 or OPRS1) and the gene is located on 9p13 (Hanner et al., 1996; Prasad et al., 1998). Sig1R is a 25-kDa protein anchored in the endoplasmic reticulum (ER) with no similarity with other known mammalian proteins, thus definitely ruling out any connection with a classical receptor family. Sig1R possesses two transmembrane regions and two steroid binding domains (SBD). These domains form a pocket which is the binding site for cholesterol, steroids, sphingolipids (Palmer et al., 2007; Fontanilla et al., 2008), and also for a wide panel of synthetic or natural compounds (sigma ligands) from different classes such as opioids, antipsychotics, psychostimulants, alkaloids or antidepressants (Pal et al., 2008; Maurice and Su, 2009) (Figure 1). In vivo, endogenous dimethyl tryptamine (DMT) interacts with Sig1R in the brain but its physiological significance as an endogenous sigma ligand is not yet clarified (Fontanilla et al., 2009; Mavlyutov et al., 2012).
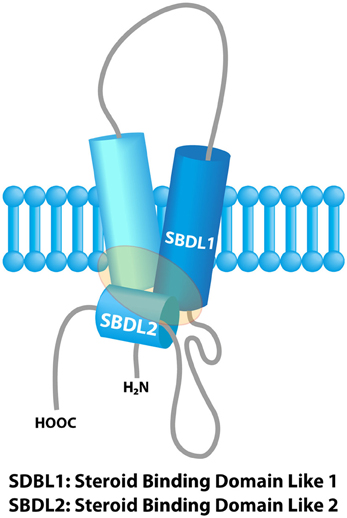
Figure 1. Model of the sigma-1 receptor binding region from previous photolabeling studies. The shaded area represents the ligand binding region. Adapted with permission from Chu et al. (2013).
The molecular nature of Sig2R has long been questioned. A recent work proposed the progesterone receptor membrane component 1 (Pgrmc1) as the putative sigma 2 binding site (Xu et al., 2011). This cytochrome-related protein binds several P450 proteins and various chemical compounds and it participates to cholesterol synthesis. However, while this putative Sig2R shares some pharmacological properties with Sig1R, the two proteins belong to distinct families. This review will focus on Sig1R.
Sig1Rs have been associated with many diseases including stroke, cocaine addiction, Alzheimer's disease, amnesia, amyotrophic lateral sclerosis, retinal degeneration, and cancer (Romieu et al., 2004; Aydar et al., 2006; Renaudo et al., 2007; Maurice and Su, 2009; Luty et al., 2010; Mavlyutov et al., 2011; Ruscher et al., 2011; Kourrich et al., 2013). Nonetheless, the way Sig1R operates in such diseases is still poorly understood. Su and colleagues'work on neurons and CHO cells have shed light on the molecular mechanisms underlying Sig1R functions. Sig1R is mainly located at the ER, in close contact with the mitochondria, in the so-called mitochondria-associated-ER membrane domains (MAM). In resting condition, Sig1R resides in ceramide- and cholesterol-rich lipid microdomains associated with the ER-resident chaperone GRP78 (BiP) (Hayashi and Su, 2007; Hayashi and Fujimoto, 2010). Under cellular stress leading to ER injury, Sig1R dissociates from BiP and binds IP3 receptors, enhancing in turn cell survival through the control of calcium signaling between the ER and mitochondria. In addition, Sig1R translocates to other cell compartments and binds to different membrane proteins. The stimulation with sigma “agonists” mimicks stress-induced Sig1R dissociation from BiP and Sig1R delocalization, while sigma ligands classified as “antagonists” impede this process (Hayashi and Su, 2007). Altogether, these results have led to a model in which Sig1R is “silent” in normal physiological conditions, whereas in case of a disease, Sig1R behaves as a chaperone that binds client protein to the benefit of cell survival (Su et al., 2010). This exciting hypothesis has been validated by recent studies demonstrating that Sig1R molecular silencing reduces brain recovery after experimental stroke (Ruscher et al., 2011) and promotes retina degeneration after acute damage to the optic nerve (Mavlyutov et al., 2011).
The question of client proteins targeted by Sig1R is of importance. Beyond the coupling with IP3 receptors, a number of studies mainly based on the effects of exogenous sigma ligands have shown that Sig1R interferes with dopamine and acetylcholine systems and modulates the function of ion channels belonging to various families. Recent studies have described a molecular interaction between Sig1R and ion channels, suggesting that ion channels represent a major client protein family for the Sig1R chaperone (Carnally et al., 2010; Crottes et al., 2011; Balasuriya et al., 2012; Kourrich et al., 2012). Over the past decades, ion channels have been integrated to the main cellular processes underlying the hallmarks of cancer: tumors often express ion channels and transporters that are absent from the corresponding tissue. It is suggested that these channels and transporters enhance the cell's capacity to adapt themselves to restraint metabolic conditions encountered within the tumor tissue (low pH and PO2, poor nutrient supply, etc… ) (Wulff et al., 2009; Prevarskaya et al., 2010; Arcangeli, 2011). Transport proteins therefore participate to the adaptive cancer cells'response to environmental stress, conferring them with greater aggressiveness. This review attempts to draw together the knowledge about ion channel regulation by Sig1R and the recent discoveries on the function of ion channels in cancer. We suggest that upon environmental challenging conditions within the tumor, Sig1R may participate in the electrical remodeling of cancer cell electrical properties to enhance their survival and aggressiveness.
Sigma 1 Receptors in Cancer
Binding experiment studies realized in the 90's revealed that sigma receptors are highly expressed in many human and rodent tumor cell lines including breast, lung, prostate, colon, melanoma, neuroblastoma and glioma (John et al., 1995; Vilner et al., 1995b; Aydar et al., 2004). However, most of the sigma ligands used in these studies are not selective enough between Sig1R and Sig2R to draw a definitive conclusion on the density of each binding site in the explored cancer cell types. Using a specific Sig1R antibody, a high expression of Sig1R was found in lung, breast and prostate cancer cell lines whereas low levels were found in normal counterpart cells. Interestingly, the Sig1R density was increased in high metastatic potential cancer cells suggesting a link between Sig1R expression and aggressiveness (Aydar et al., 2006). In another study, the expression of Sig1R was explored by imunohistochemistry in 58 breast cancer patients and 51 normal breasts. Sig1R positive epithelial cell staining was detected in 60 or 41% of invasive or in situ cancers respectively, in 75% of ductal hyperplasia and in 33% of normal breast (Wang et al., 2004). Accordingly, scintigraphy with a moderately-selective Sig1R ligand (N-[2-(1′-Piperidinyl) Ethyl]-3-123I-Iodo-4-Methoxybenzamide) on patients with primary breast cancer revealed that the ligand was specifically retained within the tumor site, but not in healthy tissues (Caveliers et al., 2001). Several reports indicate that the use of Sig1R ligands to target therapeutic nanoparticles dramatically enhances the delivery of siRNA or drugs at the tumor site in melanoma, prostate, lung and breast cancer (Li and Huang, 2006; Chen et al., 2010; Guo et al., 2012; Kim and Huang, 2012).
Altogether, these studies strongly suggest that Sig1R is over expressed in many cancer cells and an extensive exploration of Sig1R expression in biopsies from various cancers is now required to determine whether Sig1R could be proposed as a diagnosis or prognosis marker.
The effects of sigma ligands on cancer cells' behavior have been assessed by many groups in vitro and in vivo. Early descriptive works showed that cell treatment with sigma ligands causes rounding, detachment and growth inhibition of C6 glioma (Vilner et al., 1995a), breast and colon carcinoma and melanoma cells (Brent and Pang, 1995; Aydar et al., 2004). Further works by Spruce and coll. showed that the moderately selective ligand rimcazole provokes in vitro and in vivo (mouse xenograft model) a tumor-selective, caspase-dependent apoptosis of breast and colon cancer (Spruce et al., 2004; Achison et al., 2007). Rimcazole was shown to antagonize a Sig1R-dependent mechanism involving a calcium-dependent activation of phospholipase C, a calcium-independent inhibition of phosphatidylinositol 3′-kinase pathway signaling and the accumulation of HIF-1α. While Sig1R agonists (+) pentazocine and (+) SKF10,047 had no effect per se, these ligands could abrogate rimacazole-induced apoptosis, suggesting that in cancer cells, Sig1R is in an activated state and enhances survival. In agreement with this hypothesis, transfection of Sig1R in HEK293 cells reverses apoptosis induced by the over-expression of Bax or by staurospaurine (Spruce et al., 2004; Achison et al., 2007; Crottes et al., 2011). However, whether Sig1R protects cancer cells from death through a chaperoning activity has not yet been addressed.
Sig1R has also been connected to cell/matrix interaction. Aydar et al. have demonstrated that in breast cancer cells, Sig1R is associated with β1 integrin in lipid cholesterol-enriched rafts. Silencing Sig1R with siRNA chased β1 integrin from lipid rafts, reducing cell adhesion to matrix component such as fibronectin and vitronectin. Interestingly, treatment with the Sig1R agonist SKF10,047 also reduced β1 integrin density within lipid rafts and cell adhesion, an effect that was mimicked by the depletion of membrane cholesterol by methyl-β-cyclodextrin (Palmer et al., 2007).
From these data, it is clear that Sig1R participates on several facets of cancer cell biology. Recently, mutations in Sig1R have been found to cause a form of ALS and frontotemporal lobar degeneration (Luty et al., 2010; Al-Saif et al., 2011; Prause et al., 2013). Whether mutations in Sig1R also occur in cancer tissues is a question that remains to be explored. So far, the common mechanism by which Sig1R or sigma ligands drives cancer cell behavior is not clear. An exciting hypothesis arises from converging studies describing Sig1R as a sterol-dependent, stress-activated chaperone controlling lipid raft formation in the ER and the plasma membrane (PM) [extensively reviewed in Tsai et al. (2009), Hayashi and Su (2010)]. In response to environmental conditions encountered in cancer tissue (hypoxia, nutrient and growth factor deprivation) Sig1R may dynamically trigger various adaptation mechanisms, the nature of which being tightly dependent on the client protein available in a given tumor cell type. At this stage, it is noteworthy that ion channels emerge from the literature as the main client protein family for Sig1R (Hayashi and Su, 2007; Crottes et al., 2011; Balasuriya et al., 2012; Kourrich et al., 2012, 2013).
Sig1R: A Modulator of Ion Channels
Voltage-Gated Ion Channels
Voltage-gated ion channels (VGIC) are mainly involved in the initiation and shaping of action potentials and global cell excitability (Hodgkin and Huxley, 1952; Hille, 1984). The progress made during the past decade in characterizing the electrical signature of cancer cell has intriguingly extended the initial function of VGIC far beyond the field of exciting cells. Indeed, VGIC are involved in a number of tumor cell processes including mitosis (Weber et al., 2006; Becchetti, 2011), migration (Gillet et al., 2009; Becchetti and Arcangeli, 2010), apoptosis (Lang et al., 2004), adhesion to ECM (Pillozzi and Arcangeli, 2010), angiogenesis (Pillozzi et al., 2007), homing and drug resistance (Pillozzi and Arcangeli, 2010). Interestingly, Sig1R has been shown to interact with K+, Ca2+, Cl− and Na+ channels (Renaudo et al., 2004; Kourrich et al., 2012). Very recent studies have provided some clues about these interactions.
1- Voltage-gated K+ channels (VGKC)
Numerous studies have reported the inhibition of VGKC by sigma ligands in a wide range of cell types (Kennedy and Henderson, 1990; Soriani et al., 1998, 1999a,b; Lupardus et al., 2000; Kourrich et al., 2012). In particular, sigma ligands decrease current density and provoke a leftward shift in the voltage-dependency inactivation (Zera et al., 1996; Soriani et al., 1999a; Aydar et al., 2002). In a study performed in frogs'pituitary cells, it was nonetheless shown that sigma ligands depress the M-current by a rightward shift of the activation curve (Soriani et al., 1999b). The mechanism by which sigma ligands modulate Kv channels has been proposed to be either direct or indirect, depending on the model used. Inside-out patch clamp experiments suggested a direct effect of sigma ligands on Kv channels in rodent neurohypophysal terminals and in small cell lung carcinoma (Wilke et al., 1999; Lupardus et al., 2000). However, in frogs'pituitary cells, the inhibitory effects of the selective Sig1R ligand (+) pentazocine, on both delayed-rectifier and IA currents, were abolished in the presence of cholera toxin, GTPγS or GDPβS suggesting the involvement of a Gs-protein dependent pathway (Soriani et al., 1998, 1999a). How could these two sets of observation be interpreted? In a recent study, Mei et al. showed that the sigma ligand Cyproheptadine stimulates the Kv2.1-dependent current in cortical neurons in a Sig1R and Gi/o-dependent manner. The study indeed describes a functional interaction between Sig1R, Kv2.1 and a G-protein coupled receptor (GPCR): the mu-opoid receptor (He et al., 2012). It can then been proposed that sigma ligands, either alter directly the Sig1R/VGKC coupling or modulate functional complexes that integrate Sig1R, VGKC and GPCR. This last hypothesis is strengthened by recent reports demonstrating that Sig1R modulates several GPCR in the brain (i.e. opioid and muscarinic acetyl choline receptors) (Kim et al., 2010), and forms a complex with D1 and D2 dopamine receptors (Navarro et al., 2010, 2013).
Whether Sig1R requires an endogenous/exogenous sigma ligand to modulate VGKC is a crucial question that has been addressed in a few but important reports focusing on the molecular interaction between Sig1R and its partners. In Xenopus oocytes, it has been shown that the co-expression of Sig1R with Kv1.4 or Kv1.3 accelerates the inactivation kinetic parameters (Aydar et al., 2002; Kinoshita et al., 2012). In human leukaemic cells, our group found that the silencing of Sig1R by shRNA reduces the endogenous human ether-à-gogo-related gene (hERG; Kv11.1) current density without altering channel voltage dependency or kinetic parameters. Delving into the molecular mechanisms, we observed that the silencing of Sig1R decreases hERG maturation efficiency and diminishes the α-subunit channel stability at the plasma membrane, in turn reducing the number of ion channels available (Crottes et al., 2011). Inasmuch Sig1R co-immunoprecipitates with hERG, these observations are consistent with the idea of a Sig1R protein behaving either like a chaperone or a channel regulatory β-subunit through a protein/protein interaction (Aydar et al., 2002; Crottes et al., 2011; Kinoshita et al., 2012). This hypothesis was further strengthened by a recent report showing that cocaine exposure induces in nucleus accumbens a persistent protein/protein association between Sig-1Rs and Kv1.2 channels. This phenomenon is associated with a redistribution of both proteins from the intracellular compartments to the plasma membrane (Kourrich et al., 2013).
The presence of VGKC is linked to cell proliferation in various cancer types, through the regulation of both the resting (controlling the Ca2+ driving force) and cell volume, both phenomenon participating in the cell cycle checkpoints (for review; Wulff et al., 2009; Becchetti, 2011; Felipe et al., 2012). The connection between VGKC, Sig1R and cancer cell proliferation has been first addressed by Renaudo et al. (2004). We observed that selective Sig1R ligands provoke a cell cycle arrest in small cell lung carcinoma and T-ALL cells by blocking the delayed-rectifier and Kv1.3 channels, respectively. In both cases, Sig1R-dependent inhibition of potassium currents resulted in an accumulation of the cyclin inhibitor p27Kip1 and a reduction in cyclin A contents, leading to an arrest at the end of the G1 phase of the cell cycle (Renaudo et al., 2004).
VGKC of the ether-à-gogo family, i.e., hERG and EAG channels, represent a source of highly promising therapeutic targets for cancer. hERG is mainly expressed in the heart, the central nervous system and the endocrine system were it regulates the frequency of action potentials (for review: Vandenberg et al., 2012). In a series of excellent papers, Arcangeli's group has demonstrated that hERG is a tumor marker of myeloid and lymphoid leukaemias, colon and breast carcinoma, ovarian cancer and glioblastoma (Pillozzi et al., 2007, 2011; Pillozzi and Arcangeli, 2010; Arcangeli, 2011). Importantly, they demonstrated that upon β1 integrin stimulation, hERG forms signaling macro-complexes with β1-integrin, the VEGF receptor Flt-1 or the cytokine receptor CXCR4 in lipid rafts. The channel in turn participates in a crosstalk between cancer cells and their microenvironment to promote invasive processes such as motility, angiogenesis, homing and chemoresistance (Pillozzi et al., 2007; Pillozzi and Arcangeli, 2010). As stated above, we recently showed that Sig1R expression stimulates hERG maturation and membrane stability in the chronic myeloid leukaemia cell line K562. Inasmuch Sig1R co-immunoprecipitates with both immature and mature forms of the channel α-subunits, it is suggested that Sig1R not only associates with hERG in the ER, but also drives it to the plasma membrane (Crottes et al., 2011). The question of whether Sig1R is involved in the formation of such hERG-dependent signaling complexes with β-integrins and other partners is an interesting one but has not been addressed yet. This hypothesis deserves further consideration in vitro and in vivo knowing that both Sig1R silencing and treatment with the sigma ligand igmesine decrease K562 cell adhesion capacity to fibronectin in a hERG-dependent manner (Crottes et al., 2011).
Channels of the EAG family are present in a number of tumor types. Stuhmer's group nicely demonstrated that CHO cells transfected with EAG exhibit a cancerous invasive phenotype in vitro and in vivo (Hemmerlein et al., 2006; Gomez-Varela et al., 2007; Pardo and Suhmer, 2008). Further signaling studies revealed that EAG-1 (Kv10.1) enhances cell resistance to hypoxia by increasing HIF-1 levels, thus stimulating VEGF secretion (Downie et al., 2008). The over expression of EAG-2 has been recently shown in human medulloblastoma (MB). In this cancer, EAG-2 promotes the progression of the MB tumor by regulating cell volume dynamics, in turn inhibiting the tumor suppressor p38 MAPK pathway (Huang et al., 2012). While the putative link between EAG channels and Sig1R has not been addressed so far, it is tempting to speculate such an interaction considering the molecular and structural proximity between EAG and hERG channels.
2-Voltage-gated Na+ channels (VGNC)
The existence of VGNC has been first speculated by Hodgkin and Huxley to account for the fast depolarizing phase of the action potential of excitable cells (Hodgkin and Huxley, 1952). To date, the family of VGNC includes nine members mainly involved in the encoding of neuronal signaling, cardiac rhythm, muscle contraction and endocrine secretion (Hille, 1984; Harmar et al., 2009). Intriguingly, VGNC are expressed in metastatic cells of many cancers. In these cells, the sodium current driven by VGNC α subunits enhances the invasion and metastasis in vivo (Brisson et al., 2011, 2012; Yang et al., 2012). Expression of the cardiac Nav1.5 α subunit (SCN5A) is correlated with a poor prognosis in breast cancer specimens, suggesting that VGSCs may be used as prognosis marker in cancer progression (House et al., 2010; Yang et al., 2012). The mechanical link between Nav1.5 and cancer progression has been recently documented: in breast cancer cells, Nav1.5 associates with the Na+/H+ exchanger NHE1 in caveolae; Nav1.5 stimulates NHE1 activity, contributing to the acidification of the pericellular space. The low extracellular pH in turn potentiates the activity of different cathepsins involved in ECM degradation, a fundamental step for cancer cell invasion process (Gillet et al., 2009; Brisson et al., 2011, 2012). In recent studies, Jackson and Ruoho's groups have shown that sigma ligands reduce Nav1.5-dependent currents in cardiomyocytes of wild type mice. Interestingly, Nav1.5 current sensitivity to sigma ligands was lost in cardiomyocytes of knock-out mice for Sig1R (Fontanilla et al., 2009; Johannessen et al., 2011). The nature of the interaction occurring between Sig1R and Nav1.5 has been scrutinized in 2012 by atomic force microscopy (AFM) which revealed that Sig1R directly binds the channel with a four-fold symmetry in human embryonic kidney cell (HEK) heterologous expression system (Balasuriya et al., 2012). Because the Nav1.5 channel includes the four pore-forming α subunits within a single protein, this result suggests that Sig1R neither interacts with C- nor N-terminus, but rather with the transmembrane domains. This hypothesis is strengthened by the fact that deletions in the transmembrane domain of Kv1.3 subunits abolish their co-immunoprecipitation with Sig1R in Xenopus oocytes (Kinoshita et al., 2012). While the suppression of Sig1R expression in mice cardiomyocytes fails to alter any parameter of the native Nav1.5 current (Fontanilla et al., 2009), the Sig1R silencing in the highly aggressive MDA-MB-231 breast cancer cell line results in a strong reduction in current density, suggesting that Sig1R controls Nav1.5 trafficking in cancer cells but not in healthy cardiac cells (Balasuriya et al., 2012). From these observations, it can be hypothesized that Sig1R, by enhancing Nav1.5 membrane expression in breast cancer cells, modulates NHE1 activity, resulting in greater aggressiveness potency.
3-Voltage-gated calcium channels (VGCC)
VGCC are principally involved in fast synaptic transmission, cardiomyocyte and striated muscle contraction, as well as stimulus-secretion coupling. The low threshold T-type channel (Cav-3) has however been involved in proliferation and differentiation in several cancer cell lines (Gackiere et al., 2008; Prevarskaya et al., 2010; Becchetti, 2011). While no data support an interaction between Sig1R and the T-type channel in the literature, it has been shown that Sig1R co-immunoprecipitate with high-threshold L-type channels in retinal ganglion and that the sigma ligand SKF 10.047 inhibits the corresponding current (Tchedre et al., 2008). These observations suggest that VGCC in cancer cells might be a client for Sig1R.
Calcium-Activated Potassium Channels (KCa)
KCa channels are involved in many physiological processes by regulating calcium entry through the control of the membranes'resting potential and Ca2+ driving force. A link between Sig1R and small-conductance KCa channels has been recently proposed in synaptic activity and plasticity in the hippocampus (Martina et al., 2007). In this report, the authors showed that Sig1R ligands potentiate the N-Methyl-D-Aspartate (NMDA) receptor responses and long-term potentiation (LTP) by inhibiting a small conductance Ca2+-activated K+ current (SK channel). Interestingly, SK3 channels play a predominant role in melanoma and breast cancer cell migration and are considered as potent targets for cancer therapy (Potier et al., 2006; Chantome et al., 2009; Girault et al., 2012). On the other hand, SK4 channels have been involved in the migration potency of glioblastoma stem cells (Ruggieri et al., 2012). The putative interaction between SK channels and Sig1R thus constitutes an interesting hypothesis that remains to be explored.
Volume-Regulated Chloride Channels (VRCC)
VRCC, functionally coupled with K+ channels, drive cell volume regulation by controlling chloride salt-associated water efflux (Hoffmann et al., 2009). Cell volume regulation and regulatory volume decrease (RVD) participate to at least three main aspects of cancer progression, i.e., cell cycle (G1/S and G2/M volume checkpoints) (Lang et al., 2000; Rouzaire-Dubois et al., 2000; Becchetti, 2011; Hoffmann, 2011; Huang et al., 2012), motility (control of cell shape dynamics through salt and water fluxes) (Cuddapah and Sontheimer, 2011) and apoptosis (Apoptosis Volume Decrease occurring at an early signaling step of programmed cell death) (Bortner and Cidlowski, 2011; Bortner et al., 2012). Our group has shown that sigma ligands strongly inhibit both VRCC and VGKC in T leukaemic and small cell lung carcinoma cells in a Sig1R-dependent manner. VRCC and VGKC inhibition lead to a strong reduction in RVD potency after a hypotonic shock. In isotonic conditions, cell treatment with sigma ligands lead to cell swelling, underlying an arrest of cell division at the late G1 phase. These results indicate that the pharmacological alteration of Sig1R, by inhibiting channels involved in RVD, can block the cell division process (Renaudo et al., 2004, 2007). In these studies we also questioned the function of Sig1R in cancer cells in the absence of exogenous ligands. We observed that the over-expression of Sig1R in HEK cells was sufficient per se to significantly reduce the activation kinetics of VRCC upon hypotonic shock. We proposed that the presence of Sig1R induces a tonic reduction of VRCC activity, not sufficient to impede the cell cycle, but strong enough to protect cells from apoptosis by delaying AVD. This result was confirmed by showing that cells over-expressing Sig1R are less sensitive to staurospaurine-induced apoptosis than normal cells (Renaudo et al., 2007). Together with other reports, this study has unveiled the function of Sig1R as a protein involved in cell protection against environmental stress by modulating ion channels (Hayashi and Su, 2007; Renaudo et al., 2007).
Calcium Signaling and Ion Channels at the MAM
Cell fate largely depends on calcium exchanges occurring between ER and mitochondria. These exchanges generally take place at specific membrane localization, the MAMs, which were originally described as sites for lipid synthesis and lipid transfer between ER and mitochondria membranes (Rusinol et al., 1994) for review: (Parys et al., 2012). Calcium fluxes between the two compartments involve various chaperones and signaling proteins as well as ion channels and transporters including IP3 receptors, voltage-dependent anion channels (VDAC) or the translocon. The regulation of calcium entry in the mitochondria participates in the control of the energy state and cell response to ER-mediated stress. Calcium homeostasis at MAM therefore constitutes a crossroad decision for cell engagement toward apoptosis, survival or autophagy (Tsai et al., 2009; Parys et al., 2012; Hammadi et al., 2013). Not surprisingly, MAM-associated transport machinery is disregulated in the context of environmental challenges in cancer such as hypoxia, low pH and dramatic nutrient deprivation (Moenner et al., 2007; Raturi and Simmen, 2013). As stated above, Hayashi and Su have demonstrated in CHO cells that the Sig1R chaperone plays a fundamental role in regulating the Ca2+ transport machinery within the MAMs, leading to a reinforced cell survival in response to environmental stress (Hayashi and Su, 2007). In particular, stress-activated Sig1R chaperones IP3 receptor and prevents its degradation. Moreover a recent report has shown that Sig1R is physically associated to VDAC2, a mitochondrial channel involved in cholesterol import into the mitochodria for metabolic regulation (Marriott et al., 2012). While no data is available on the function of MAM-associated Sig1R in tumors, it is conceivable that Sig1R contributes to the adaptation of cancer cells in restrictive environment.
Conclusion and Perspectives
In summary, Sig1R is a stress-activated chaperone which controls, through different mechanisms, several families of ion channels at the plasma membrane and at the MAM. Studies realized in the retina, brain and heart strongly suggest that Sig1R participates in cell resistance to tissue injury, for instance infarction, stroke or ischemia (Kourrich et al., 2012). Several reports indicate that Sig1R exerts a role only in conditions of stress and remains generally “silent” in healthy organs or in steady-state conditions (Maurice and Su, 2009; Tsai et al., 2009). In good agreement with this idea, Sig1R KO mice present a normal development and behavior but are less resistant to experimental stroke (Ruscher et al., 2011). Moreover, the absence of side effects of Sig1R ligands in clinical trials in human suffering psychiatric disorders, improves the hypothesis of a dynamic and protective role of Sig1R in stressing conditions (Volz and Stoll, 2004; Banister and Kassiou, 2012). Thus, it is tempting to speculate that tumor cells hijack the primary protective function of Sig1R to enhance their survival/growing/invasive potency in restrictive metabolic conditions encountered within the tumor tissue. As demonstrated by many authors, the aberrant expression of ion channels confers selective advantages for cancer cells to adapt their behavior and survival in the tumor environment. While research studies mainly focus on the function of one ion channel in a cancer type, it is important to consider that many ion channels are deregulated in the same cancer cell. Because a variety of ion channels are client proteins for Sig1R, we speculate that the Sig1R chaperone controls cancer cells'electrical plasticity by putatively “driving” ion channels to potentiate their function in proliferation, apoptosis resistance, migration and angiogenesis (Figure 2). At the time being, there is no real explanation on the process that controls the expression of all these ion channels in cancer cells and it is often postulated that this is due to the acquisition of an embryonic or developmental phenotype. The possibility that Sig1R expression might participate to this phenotype is an interesting hypothesis that has not been explored so far.
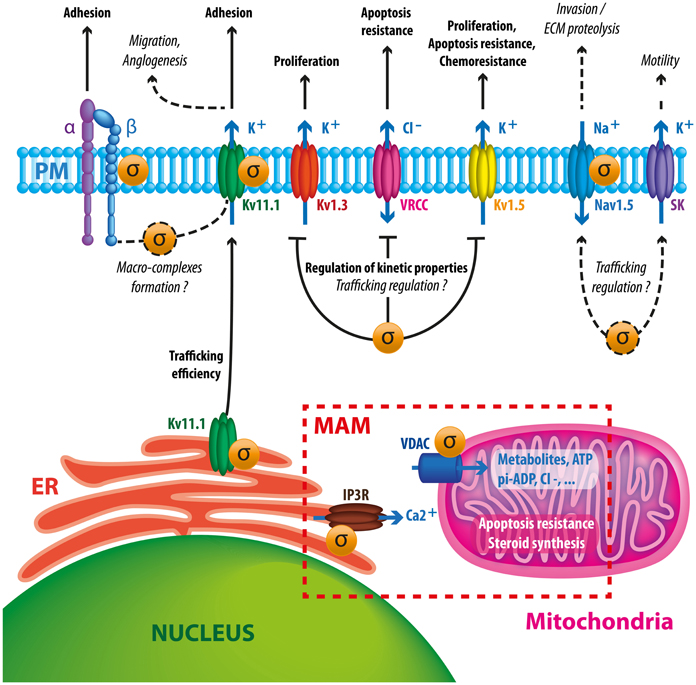
Figure 2. Schematic diagram summarizing putative and documented interactions between Sig1R and ion channels in cancer cells. Sig1R promotes hERG maturation and membrane stability which may potentiate channel interaction with β1 integrin macrocomplexes, leading to enhanced migration and angiogenesis. Sig1R also directly binds to other VGIC such as Kv1.3, Kv1.5, and Nav1.5 controlling either current density or kinetic properties of channels. These interactions may have consequences on cell proliferation, apoptosis resistance, chemoresistance and invasive properties. The presence of Sig1R in cancer cells tonically reduces VRCC activity via a yet unknown mechanism, leading to a better resistance to apoptotic signals. Sigma receptors have also been associated to SK channels, suggesting that they may participate to cancer cell motility. At the MAM, Sig1R may enhance cell survival by regulating calcium fluxes between ER and mitochondria by chaperoning IP3 receptors and VDAC channels.
The literature strongly argues for a close interaction between Sig1R and ion channels that are already expressed in the cell. An alternative mechanism should however be considered: because of the spatial dynamics of Sig1R within the cell, the protein could also behave as a transcriptional factor controlling either directly or indirectly a kit of genes encoding ion channels. While no data supports the presence of Sig1R in the nucleus, many reports have shown the involvement of Sig1R in a number of signaling pathways potentially targeting transcriptional activity (i.e., MAP kinases, PKA, PI3K/AKT, NFk-B, c-Fos, CREB) (for review: Hayashi et al., 2011).
It is noteworthy that ion channels expressed in cancer cells play important functions in healthy organs as well such as in the heart and brain. As a consequence, therapies based on toxins and drugs directly targeting ion channels present major drawbacks for cancer treatment. The unique properties of Sig1R may pave a new avenue to alter ion channels specifically within tumors. In this regard, many outstanding questions need to be addressed to unravel the importance of Sig1R in cancer such as the consequences of Sig1R silencing on the electrical signature of cancer cells and subsequent alteration of their behavior in vitro and in vivo. Promising anti-tumoral effects have been obtained in vivo with exogenous sigma ligands, but the innate function of Sig1R in cancer remains undetermined. Moreover, the molecular mechanisms of Sig1R ligands on Sig1R/ion channel complexes remain to be addressed.
Answers to these questions will open new strategies based on the targeting of Sig1R to target ion channels and associated cancer progression.
Conflict of Interest Statement
The authors declare that the research was conducted in the absence of any commercial or financial relationships that could be construed as a potential conflict of interest.
Acknowledgments
This work was supported by the University of Nice-Sophia Antipolis, the CNRS and the Association Ti'Toine Normandie. David Crottès has a fellowship from Région PACA and CNRS. We thank Ms. Elodie Busson-Crottès (http://www.coeurlyweb.com) for the realisation of the figure and Stéphanie Simonet for English corrections.
References
Achison, M., Boylan, M. T., Hupp, T. R., and Spruce, B. A. (2007). HIF-1alpha contributes to tumour-selective killing by the sigma receptor antagonist rimcazole. Oncogene 26, 1137–1146. doi: 10.1038/sj.onc.1209890
Al-Saif, A., Al-Mohanna, F., and Bohlega, S. (2011). A mutation in sigma-1 receptor causes juvenile amyotrophic lateral sclerosis. Ann. Neurol. 70, 913–919. doi: 10.1002/ana.22534
Arcangeli, A. (2011). Ion channels and transporters in cancer. 3. Ion channels in the tumor cell-microenvironment cross talk. Am. J. Physiol. Cell Physiol. 301, C762–C771. doi: 10.1152/ajpcell.00113.2011
Aydar, E., Onganer, P., Perrett, R., Djamgoz, M. B., and Palmer, C. P. (2006). The expression and functional characterization of sigma (sigma) 1 receptors in breast cancer cell lines. Cancer Lett. 242, 245–257. doi: 10.1016/j.canlet.2005.11.011
Aydar, E., Palmer, C. P., and Djamgoz, M. B. (2004). Sigma receptors and cancer: possible involvement of ion channels. Cancer Res. 64, 5029–5035. doi: 10.1158/0008-5472.CAN-03-2329
Aydar, E., Palmer, C. P., Klyachko, V. A., and Jackson, M. B. (2002). The sigma receptor as a ligand-regulated auxiliary potassium channel subunit. Neuron 34, 399–410. doi: 10.1016/S0896-6273(02)00677-3
Balasuriya, D., Stewart, A. P., Crottes, D., Borgese, F., Soriani, O., and Edwardson, J. M. (2012). The sigma-1 receptor binds to the Nav1.5 voltage-gated Na+ channel with 4-fold symmetry. J. Biol. Chem. 287, 37021–37029. doi: 10.1074/jbc.M112.382077
Banister, S. D., and Kassiou, M. (2012). The therapeutic potential of sigma (sigma) receptors for the treatment of central nervous system diseases: evaluation of the evidence. Curr. Pharm. Des. 18, 884–901. doi: 10.2174/138161212799436539
Becchetti, A. (2011). Ion channels and transporters in cancer. 1. Ion channels and cell proliferation in cancer. Am. J. Physiol. Cell Physiol. 301, C255–C265. doi: 10.1152/ajpcell.00047.2011
Becchetti, A., and Arcangeli, A. (2010). Integrins and ion channels in cell migration: implications for neuronal development, wound healing and metastatic spread. Adv. Exp. Med. Biol. 674, 107–123. doi: 10.1007/978-1-4419-6066-5_10
Bortner, C. D., and Cidlowski, J. A. (2011). Life and death of lymphocytes: a volume regulation affair. Cell. Physiol. Biochem. 28, 1079–1088. doi: 10.1159/000335864
Bortner, C. D., Scoltock, A. B., Sifre, M. I., and Cidlowski, J. A. (2012). Osmotic stress resistance imparts acquired anti-apoptotic mechanisms in lymphocytes. J. Biol. Chem. 287, 6284–6295. doi: 10.1074/jbc.M111.293001
Brent, P. J., and Pang, G. T. (1995). Sigma binding site ligands inhibit cell proliferation in mammary and colon carcinoma cell lines and melanoma cells in culture. Eur. J. Pharmacol. 278, 151–160. doi: 10.1016/0014-2999(95)00115-2
Brisson, L., Gillet, L., Calaghan, S., Besson, P., Le Guennec, J. Y., Roger, S., and et al. (2011). Na(V)1.5 enhances breast cancer cell invasiveness by increasing NHE1-dependent H(+) efflux in caveolae. Oncogene 30, 2070–2076. doi: 10.1038/onc.2010.574
Brisson, L., Reshkin, S. J., Gore, J., and Roger, S. (2012). pH regulators in invadosomal functioning: proton delivery for matrix tasting. Eur. J. Cell Biol. 91, 847–860. doi: 10.1016/j.ejcb.2012.04.004
Carnally, S. M., Johannessen, M., Henderson, R. M., Jackson, M. B., and Edwardson, J. M. (2010). Demonstration of a direct interaction between sigma-1 receptors and acid-sensing ion channels. Biophys. J. 98, 1182–1191. doi: 10.1016/j.bpj.2009.12.4293
Caveliers, V., Everaert, H., Lahoutte, T., Dierickx, L. O., John, C. S., and Bossuyt, A. (2001). Labelled sigma receptor ligands: can their role in neurology and oncology be extended? Eur. J. Nucl. Med. 28, 133–135. doi: 10.1007/s002590000368
Chantome, A., Girault, A., Potier, M., Collin, C., Vaudin, P., Pages, J. C., and et al. (2009). KCa2.3 channel-dependent hyperpolarization increases melanoma cell motility. Exp. Cell Res. 315, 3620–3630. doi: 10.1016/j.yexcr.2009.07.021
Chen, Y., Bathula, S. R., Yang, Q., and Huang, L. (2010). Targeted nanoparticles deliver siRNA to melanoma. J. Invest. Dermatol. 130, 2790–2798. doi: 10.1038/jid.2010.222
Chu, U. B., Ramachandran, S., Hajipour, A. R., and Ruoho, A. E. (2013). Photoaffinity labeling of the sigma-1 receptor with N-[3-(4-nitrophenyl)propyl]-N-dodecylamine: evidence of receptor dimers. Biochemistry 52, 859–868. doi: 10.1021/bi301517u
Crottes, D., Martial, S., Rapetti-Mauss, R., Pisani, D. F., Loriol, C., Pellissier, B., and et al. (2011). Sig1R protein regulates hERG channel expression through a post-translational mechanism in leukemic cells. J. Biol. Chem. 286, 27947–27958. doi: 10.1074/jbc.M111.226738
Cuddapah, V. A., and Sontheimer, H. (2011). Ion channels and transporters [corrected] in cancer. 2. Ion channels and the control of cancer cell migration. Am. J. Physiol. Cell Physiol. 301, C541–C549. doi: 10.1152/ajpcell.00102.2011
Downie, B. R., Sanchez, A., Knotgen, H., Contreras-Jurado, C., Gymnopoulos, M., Weber, C., and et al. (2008). Eag1 expression interferes with hypoxia homeostasis and induces angiogenesis in tumors. J. Biol. Chem. 283, 36234–36240. doi: 10.1074/jbc.M801830200
Felipe, A., Bielanska, J., Comes, N., Vallejo, A., Roig, S., Ramon, Y. C. S., and et al. (2012). Targeting the voltage-dependent K(+) channels Kv1.3 and Kv1.5 as tumor biomarkers for cancer detection and prevention. Curr. Med. Chem. 19, 661–674. doi: 10.2174/092986712798992048
Fontanilla, D., Hajipour, A. R., Pal, A., Chu, U. B., Arbabian, M., and Ruoho, A. E. (2008). Probing the steroid binding domain-like I (SBDLI) of the sigma-1 receptor binding site using N-substituted photoaffinity labels. Biochemistry 47, 7205–7217. doi: 10.1021/bi800564j
Fontanilla, D., Johannessen, M., Hajipour, A. R., Cozzi, N. V., Jackson, M. B., and Ruoho, A. E. (2009). The hallucinogen N,N-dimethyltryptamine (DMT) is an endogenous sigma-1 receptor regulator. Science 323, 934–937. doi: 10.1126/science.1166127
Gackiere, F., Bidaux, G., Delcourt, P., Van Coppenolle, F., Katsogiannou, M., Dewailly, E., and et al. (2008). CaV3.2 T-type calcium channels are involved in calcium-dependent secretion of neuroendocrine prostate cancer cells. J. Biol. Chem. 283, 10162–10173. doi: 10.1074/jbc.M707159200
Gillet, L., Roger, S., Besson, P., Lecaille, F., Gore, J., Bougnoux, P., and et al. (2009). Voltage-gated sodium channel activity promotes cysteine cathepsin-dependent invasiveness and colony growth of human cancer cells. J. Biol. Chem. 284, 8680–8691. doi: 10.1074/jbc.M806891200
Girault, A., Haelters, J. P., Potier-Cartereau, M., Chantome, A., Jaffres, P. A., Bougnoux, P., and et al. (2012). Targeting SKCa channels in cancer: potential new therapeutic approaches. Curr. Med. Chem. 19, 697–713. doi: 10.2174/092986712798992039
Gomez-Varela, D., Zwick-Wallasch, E., Knotgen, H., Sanchez, A., Hettmann, T., Ossipov, D., and et al. (2007). Monoclonal antibody blockade of the human Eag1 potassium channel function exerts antitumor activity. Cancer Res. 67, 7343–7349. doi: 10.1158/0008-5472.CAN-07-0107
Guo, J., Ogier, J. R., Desgranges, S., Darcy, R., and O'Driscoll, C. (2012). Anisamide-targeted cyclodextrin nanoparticles for siRNA delivery to prostate tumours in mice. Biomaterials 33, 7775–7784. doi: 10.1016/j.biomaterials.2012.07.012
Hammadi, M., Oulidi, A., Gackiere, F., Katsogiannou, M., Slomianny, C., Roudbaraki, M., and et al. (2013). Modulation of ER stress and apoptosis by endoplasmic reticulum calcium leak via translocon during unfolded protein response: involvement of GRP78. FASEB J. 27, 1600–1609. doi: 10.1096/fj.12-218875
Hanner, M., Moebius, F. F., Flandorfer, A., Knaus, H. G., Striessnig, J., Kempner, E., and et al. (1996). Purification, molecular cloning, and expression of the mammalian sigma1-binding site. Proc. Natl. Acad. Sci. U.S.A. 93, 8072–8077. doi: 10.1073/pnas.93.15.8072
Harmar, A. J., Hills, R. A., Rosser, E. M., Jones, M., Buneman, O. P., Dunbar, D. R., and et al. (2009). IUPHAR-DB: the IUPHAR database of G protein-coupled receptors and ion channels. Nucleic Acids Res. 37, D680–D685. doi: 10.1093/nar/gkn728
Hayashi, T., and Fujimoto, M. (2010). Detergent-resistant microdomains determine the localization of sigma-1 receptors to the endoplasmic reticulum-mitochondria junction. Mol. Pharmacol. 77, 517–528. doi: 10.1124/mol.109.062539
Hayashi, T., and Su, T. P. (2007). Sigma-1 receptor chaperones at the ER-mitochondrion interface regulate Ca(2+) signaling and cell survival. Cell 131, 596–610. doi: 10.1016/j.cell.2007.08.036
Hayashi, T., and Su, T. P. (2010). Cholesterol at the endoplasmic reticulum: roles of the sigma-1 receptor chaperone and implications thereof in human diseases. Subcell. Biochem. 51, 381–398. doi: 10.1007/978-90-481-8622-8_13
Hayashi, T., Tsai, S. Y., Mori, T., Fujimoto, M., and Su, T. P. (2011). Targeting ligand-operated chaperone sigma-1 receptors in the treatment of neuropsychiatric disorders. Expert Opin. Ther. Targets 15, 557–577.
He, Y. L., Zhang, C. L., Gao, X. F., Yao, J. J., Hu, C. L., and Mei, Y. A. (2012). Cyproheptadine enhances the I(K) of mouse cortical neurons through sigma-1 receptor-mediated intracellular signal pathway. PLoS ONE 7:e41303. doi: 10.1371/journal.pone.0041303
Hemmerlein, B., Weseloh, R. M., Mello De Queiroz, F., Knotgen, H., Sanchez, A., Rubio, M. E., and et al. (2006). Overexpression of Eag1 potassium channels in clinical tumours. Mol. Cancer 5:41. doi: 10.1186/1476-4598-5-41
Hodgkin, A. L., and Huxley, A. F. (1952). A quantitative description of membrane current and its application to conduction and excitation in nerve. J. Physiol. 117, 500–544.
Hoffmann, E. K. (2011). Ion channels involved in cell volume regulation: effects on migration, proliferation, and programmed cell death in non adherent EAT cells and adherent ELA cells. Cell. Physiol. Biochem. 28, 1061–1078. doi: 10.1159/000335843
Hoffmann, E. K., Lambert, I. H., and Pedersen, S. F. (2009). Physiology of cell volume regulation in vertebrates. Physiol. Rev. 89, 193–277. doi: 10.1152/physrev.00037.2007
House, C. D., Vaske, C. J., Schwartz, A. M., Obias, V., Frank, B., Luu, T., and et al. (2010). Voltage-gated Na+ channel SCN5A is a key regulator of a gene transcriptional network that controls colon cancer invasion. Cancer Res. 70, 6957–6967. doi: 10.1158/0008-5472.CAN-10-1169
Huang, X., Dubuc, A. M., Hashizume, R., Berg, J., He, Y., Wang, J., and et al. (2012). Voltage-gated potassium channel EAG2 controls mitotic entry and tumor growth in medulloblastoma via regulating cell volume dynamics. Genes Dev. 26, 1780–1796. doi: 10.1101/gad.193789.112
Johannessen, M., Fontanilla, D., Mavlyutov, T., Ruoho, A. E., and Jackson, M. B. (2011). Antagonist action of progesterone at sigma-receptors in the modulation of voltage-gated sodium channels. Am. J. Physiol. Cell Physiol. 300, C328–C337. doi: 10.1152/ajpcell.00383.2010
John, C. S., Bowen, W. D., Varma, V. M., McAfee, J. G., and Moody, T. W. (1995). Sigma receptors are expressed in human non-small cell lung carcinoma. Life Sci. 56, 2385–2392. doi: 10.1016/0024-3205(95)00232-U
Kennedy, C., and Henderson, G. (1990). Inhibition of potassium currents by the sigma receptor ligand (+)-3-(3-hydroxyphenyl)-N-(1-propyl)piperidine in sympathetic neurons of the mouse isolated hypogastric ganglion. Neuroscience 35, 725–733. doi: 10.1016/0306-4522(90)90343-3
Kim, F. J., Kovalyshyn, I., Burgman, M., Neilan, C., Chien, C. C., and Pasternak, G. W. (2010). Sigma 1 receptor modulation of G-protein-coupled receptor signaling: potentiation of opioid transduction independent from receptor binding. Mol. Pharmacol. 77, 695–703. doi: 10.1124/mol.109.057083
Kim, S. K., and Huang, L. (2012). Nanoparticle delivery of a peptide targeting EGFR signaling. J. Control. Release 157, 279–286. doi: 10.1016/j.jconrel.2011.08.014
Kinoshita, M., Matsuoka, Y., Suzuki, T., Mirrielees, J., and Yang, J. (2012). Sigma-1 receptor alters the kinetics of Kv1.3 voltage gated potassium channels but not the sensitivity to receptor ligands. Brain Res. 1452, 1–9. doi: 10.1016/j.brainres.2012.02.070
Kourrich, S., Hayashi, T., Chuang, J. Y., Tsai, S. Y., Su, T. P., and Bonci, A. (2013). Dynamic interaction between sigma-1 receptor and Kv1.2 shapes neuronal and behavioral responses to cocaine. Cell 152, 236–247. doi: 10.1016/j.cell.2012.12.004
Kourrich, S., Su, T. P., Fujimoto, M., and Bonci, A. (2012). The sigma-1 receptor: roles in neuronal plasticity and disease. Trends Neurosci. 35, 762–771. doi: 10.1016/j.tins.2012.09.007
Lang, F., Gulbins, E., Szabo, I., Lepple-Wienhues, A., Huber, S. M., Duranton, C., and et al. (2004). Cell volume and the regulation of apoptotic cell death. J. Mol. Recognit. 17, 473–480. doi: 10.1002/jmr.705
Lang, F., Ritter, M., Gamper, N., Huber, S., Fillon, S., Tanneur, V., and et al. (2000). Cell volume in the regulation of cell proliferation and apoptotic cell death. Cell. Physiol. Biochem. 10, 417–428. doi: 10.1159/000016367
Li, S. D., and Huang, L. (2006). Targeted delivery of antisense oligodeoxynucleotide and small interference RNA into lung cancer cells. Mol. Pharm. 3, 579–588. doi: 10.1021/mp060039w
Lupardus, P. J., Wilke, R. A., Aydar, E., Palmer, C. P., Chen, Y., Ruoho, A. E., and et al. (2000). Membrane-delimited coupling between sigma receptors and K+ channels in rat neurohypophysial terminals requires neither G-protein nor ATP. J. Physiol. 526, 527–539. doi: 10.1111/j.1469-7793.2000.00527.x
Luty, A. A., Kwok, J. B., Dobson-Stone, C., Loy, C. T., Coupland, K. G., Karlstrom, H., and et al. (2010). Sigma nonopioid intracellular receptor 1 mutations cause frontotemporal lobar degeneration-motor neuron disease. Ann. Neurol. 68, 639–649. doi: 10.1002/ana.22274
Marriott, K. S., Prasad, M., Thapliyal, V., and Bose, H. S. (2012). Sigma-1 receptor at the mitochondrial-associated endoplasmic reticulum membrane is responsible for mitochondrial metabolic regulation. J. Pharmacol. Exp. Ther. 343, 578–586. doi: 10.1124/jpet.112.198168
Martin, W. R., Eades, C. G., Thompson, J. A., Huppler, R. E., and Gilbert, P. E. (1976). The effects of morphine- and nalorphine- like drugs in the nondependent and morphine-dependent chronic spinal dog. J. Pharmacol. Exp. Ther. 197, 517–532.
Martina, M., Turcotte, M. E., Halman, S., and Bergeron, R. (2007). The sigma-1 receptor modulates NMDA receptor synaptic transmission and plasticity via SK channels in rat hippocampus. J. Physiol. 578, 143–157. doi: 10.1113/jphysiol.2006.116178
Maurice, T., and Su, T. P. (2009). The pharmacology of sigma-1 receptors. Pharmacol. Ther. 124, 195–206. doi: 10.1016/j.pharmthera.2009.07.001
Mavlyutov, T. A., Epstein, M. L., Liu, P., Verbny, Y. I., Ziskind-Conhaim, L., and Ruoho, A. E. (2012). Development of the sigma-1 receptor in C-terminals of motoneurons and colocalization with the N,N'-dimethyltryptamine forming enzyme, indole-N-methyl transferase. Neuroscience 206, 60–68. doi: 10.1016/j.neuroscience.2011.12.040
Mavlyutov, T. A., Nickells, R. W., and Guo, L. W. (2011). Accelerated retinal ganglion cell death in mice deficient in the Sigma-1 receptor. Mol. Vis. 17, 1034–1043.
Moenner, M., Pluquet, O., Bouchecareilh, M., and Chevet, E. (2007). Integrated endoplasmic reticulum stress responses in cancer. Cancer Res. 67, 10631–10634. doi: 10.1158/0008-5472.CAN-07-1705
Navarro, G., Moreno, E., Aymerich, M., Marcellino, D., McCormick, P. J., Mallol, J., and et al. (2010). Direct involvement of sigma-1 receptors in the dopamine D1 receptor-mediated effects of cocaine. Proc. Natl. Acad. Sci. U.S.A. 107, 18676–18681. doi: 10.1073/pnas.1008911107
Navarro, G., Moreno, E., Bonaventura, J., Brugarolas, M., Farre, D., Aguinaga, D., and et al. (2013). Cocaine inhibits dopamine D2 receptor signaling via sigma-1-D2 receptor heteromers. PLoS ONE 8:e61245. doi: 10.1371/journal.pone.0061245
Pal, A., Chu, U. B., Ramachandran, S., Grawoig, D., Guo, L. W., Hajipour, A. R., and et al. (2008). Juxtaposition of the steroid binding domain-like I and II regions constitutes a ligand binding site in the sigma-1 receptor. J. Biol. Chem. 283, 19646–19656. doi: 10.1074/jbc.M802192200
Palmer, C. P., Mahen, R., Schnell, E., Djamgoz, M. B., and Aydar, E. (2007). Sigma-1 receptors bind cholesterol and remodel lipid rafts in breast cancer cell lines. Cancer Res. 67, 11166–11175. doi: 10.1158/0008-5472.CAN-07-1771
Pardo, L. A., and Suhmer, W. (2008). Eag1 as a cancer target. Expert Opin. Ther. Targets 12, 837–843. doi: 10.1517/14728222.12.7.837
Parys, J. B., Decuypere, J. P., and Bultynck, G. (2012). Role of the inositol 1,4,5-trisphosphate receptor/Ca2+-release channel in autophagy. Cell Commun. Signal. 10:17. doi: 10.1186/1478-811X-10-17
Pillozzi, S., and Arcangeli, A. (2010). Physical and functional interaction between integrins and hERG1 channels in cancer cells. Adv. Exp. Med. Biol. 674, 55–67. doi: 10.1007/978-1-4419-6066-5_6
Pillozzi, S., Brizzi, M. F., Bernabei, P. A., Bartolozzi, B., Caporale, R., Basile, V., and et al. (2007). VEGFR-1 (FLT-1), beta1 integrin, and hERG K+ channel for a macromolecular signaling complex in acute myeloid leukemia: role in cell migration and clinical outcome. Blood 110, 1238–1250. doi: 10.1182/blood-2006-02-003772
Pillozzi, S., Masselli, M., De Lorenzo, E., Accordi, B., Cilia, E., Crociani, O., and et al. (2011). Chemotherapy resistance in acute lymphoblastic leukemia requires hERG1 channels and is overcome by hERG1 blockers. Blood 117, 902–914. doi: 10.1182/blood-2010-01-262691
Potier, M., Joulin, V., Roger, S., Besson, P., Jourdan, M. L., Leguennec, J. Y., and et al. (2006). Identification of SK3 channel as a new mediator of breast cancer cell migration. Mol. Cancer Ther. 5, 2946–2953. doi: 10.1158/1535-7163.MCT-06-0194
Prasad, P. D., Li, H. W., Fei, Y. J., Ganapathy, M. E., Fujita, T., Plumley, L. H., and et al. (1998). Exon-intron structure, analysis of promoter region, and chromosomal localization of the human type 1 sigma receptor gene. J. Neurochem. 70, 443–451. doi: 10.1046/j.1471-4159.1998.70020443.x
Prause, J., Goswami, A., Katona, I., Roos, A., Schnizler, M., Bushuven, E., and et al. (2013). Altered localization, abnormal modification and loss of function of Sigma receptor-1 in amyotrophic lateral sclerosis. Hum. Mol. Genet. 22, 1581–1600. doi: 10.1093/hmg/ddt008
Prevarskaya, N., Skryma, R., and Shuba, Y. (2010). Ion channels and the hallmarks of cancer. Trends Mol. Med. 16, 107–121. doi: 10.1016/j.molmed.2010.01.005
Quirion, R., Bowen, W. D., Itzhak, Y., Junien, J. L., Musacchio, J. M., Rothman, R. B., and et al. (1992). A proposal for the classification of sigma binding sites. Trends Pharmacol. Sci. 13, 85–86. doi: 10.1016/0165-6147(92)90030-A
Raturi, A., and Simmen, T. (2013). Where the endoplasmic reticulum and the mitochondrion tie the knot: the mitochondria-associated membrane (MAM). Biochim. Biophys. Acta 1833, 213–224. doi: 10.1016/j.bbamcr.2012.04.013
Renaudo, A., L'hoste, S., Guizouarn, H., Borgese, F., and Soriani, O. (2007). Cancer cell cycle modulated by a functional coupling between sigma-1 receptors and Cl- channels. J. Biol. Chem. 282, 2259–2267. doi: 10.1074/jbc.M607915200
Renaudo, A., Watry, V., Chassot, A. A., Ponzio, G., Ehrenfeld, J., and Soriani, O. (2004). Inhibition of tumor cell proliferation by sigma ligands is associated with K+ channel inhibition and p27kip1 accumulation. J. Pharmacol. Exp. Ther. 311, 1105–1114. doi: 10.1124/jpet.104.072413
Romieu, P., Meunier, J., Garcia, D., Zozime, N., Martin-Fardon, R., Bowen, W. D., and et al. (2004). The sigma1 (sigma1) receptor activation is a key step for the reactivation of cocaine conditioned place preference by drug priming. Psychopharmacology (Berl.) 175, 154–162. doi: 10.1007/s00213-004-1814-x
Rouzaire-Dubois, B., Milandri, J. B., Bostel, S., and Dubois, J. M. (2000). Control of cell proliferation by cell volume alterations in rat C6 glioma cells. Pflugers Arch. 440, 881–888. doi: 10.1007/s004240000371
Ruggieri, P., Mangino, G., Fioretti, B., Catacuzzeno, L., Puca, R., Ponti, D., and et al. (2012). The inhibition of KCa3.1 channels activity reduces cell motility in glioblastoma derived cancer stem cells. PLoS ONE 7:e47825. doi: 10.1371/journal.pone.0047825
Ruscher, K., Shamloo, M., Rickhag, M., Ladunga, I., Soriano, L., Gisselsson, L., and et al. (2011). The sigma-1 receptor enhances brain plasticity and functional recovery after experimental stroke. Brain 134, 732–746. doi: 10.1093/brain/awq367
Rusinol, A. E., Cui, Z., Chen, M. H., and Vance, J. E. (1994). A unique mitochondria-associated membrane fraction from rat liver has a high capacity for lipid synthesis and contains pre-Golgi secretory proteins including nascent lipoproteins. J. Biol. Chem. 269, 27494–27502.
Soriani, O., Foll, F. L., Roman, F., Monnet, F. P., Vaudry, H., and Cazin, L. (1999a). A-current down-modulated by sigma receptor in frog pituitary melanotrope cells through a G protein-dependent pathway. J. Pharmacol. Exp. Ther. 289, 321–328.
Soriani, O., Le Foll, F., Galas, L., Roman, F., Vaudry, H., and Cazin, L. (1999b). The sigma-ligand (+)-pentazocine depresses M current and enhances calcium conductances in frog melanotrophs. Am. J. Physiol. 277, E73–E80.
Soriani, O., Vaudry, H., Mei, Y. A., Roman, F., and Cazin, L. (1998). Sigma ligands stimulate the electrical activity of frog pituitary melanotrope cells through a G-protein-dependent inhibition of potassium conductances. J. Pharmacol. Exp. Ther. 286, 163–171.
Spruce, B. A., Campbell, L. A., McTavish, N., Cooper, M. A., Appleyard, M. V., O'Neill, M., and et al. (2004). Small molecule antagonists of the sigma-1 receptor cause selective release of the death program in tumor and self-reliant cells and inhibit tumor growth in vitro and in vivo. Cancer Res. 64, 4875–4886. doi: 10.1158/0008-5472.CAN-03-3180
Su, T. P., Hayashi, T., Maurice, T., Buch, S., and Ruoho, A. E. (2010). The sigma-1 receptor chaperone as an inter-organelle signaling modulator. Trends Pharmacol. Sci. 31, 557–566. doi: 10.1016/j.tips.2010.08.007
Tchedre, K. T., Huang, R. Q., Dibas, A., Krishnamoorthy, R. R., Dillon, G. H., and Yorio, T. (2008). Sigma-1 receptor regulation of voltage-gated calcium channels involves a direct interaction. Invest. Ophthalmol. Vis. Sci. 49, 4993–5002. doi: 10.1167/iovs.08-1867
Tsai, S. Y., Hayashi, T., Mori, T., and Su, T. P. (2009). Sigma-1 receptor chaperones and diseases. Cent. Nerv. Syst. Agents Med. Chem. 9, 184–189. doi: 10.2174/1871524910909030184
Vandenberg, J. I., Perry, M. D., Perrin, M. J., Mann, S. A., Ke, Y., and Hill, A. P. (2012). hERG K(+) channels: structure, function, and clinical significance. Physiol. Rev. 92, 1393–1478. doi: 10.1152/physrev.00036.2011
Vilner, B. J., De Costa, B. R., and Bowen, W. D. (1995a). Cytotoxic effects of sigma ligands: sigma receptor-mediated alterations in cellular morphology and viability. J. Neurosci. 15, 117–134.
Vilner, B. J., John, C. S., and Bowen, W. D. (1995b). Sigma-1 and sigma-2 receptors are expressed in a wide variety of human and rodent tumor cell lines. Cancer Res. 55, 408–413.
Volz, H. P., and Stoll, K. D. (2004). Clinical trials with sigma ligands. Pharmacopsychiatry 37(Suppl. 3), S214–S220. doi: 10.1055/s-2004-832680
Wang, B., Rouzier, R., Albarracin, C. T., Sahin, A., Wagner, P., Yang, Y., and et al. (2004). Expression of sigma 1 receptor in human breast cancer. Breast Cancer Res. Treat. 87, 205–214. doi: 10.1007/s10549-004-6590-0
Weber, C., Mello De Queiroz, F., Downie, B. R., Suckow, A., Stuhmer, W., and Pardo, L. A. (2006). Silencing the activity and proliferative properties of the human EagI potassium channel by RNA Interference. J. Biol. Chem. 281, 13030–13037. doi: 10.1074/jbc.M600883200
Wilke, R. A., Mehta, R. P., Lupardus, P. J., Chen, Y., Ruoho, A. E., and Jackson, M. B. (1999). Sigma receptor photolabeling and sigma receptor-mediated modulation of potassium channels in tumor cells. J. Biol. Chem. 274, 18387–18392. doi: 10.1074/jbc.274.26.18387
Wulff, H., Castle, N. A., and Pardo, L. A. (2009). Voltage-gated potassium channels as therapeutic targets. Nat. Rev. Drug Discov. 8, 982–1001. doi: 10.1038/nrd2983
Xu, J., Zeng, C., Chu, W., Pan, F., Rothfuss, J. M., Zhang, F., and et al. (2011). Identification of the PGRMC1 protein complex as the putative sigma-2 receptor binding site. Nat. Commun. 2:380. doi: 10.1038/ncomms1386
Yang, M., Kozminski, D. J., Wold, L. A., Modak, R., Calhoun, J. D., Isom, L. L., and et al. (2012). Therapeutic potential for phenytoin: targeting Na(v)1.5 sodium channels to reduce migration and invasion in metastatic breast cancer. Breast Cancer Res. Treat. 134, 603–615. doi: 10.1007/s10549-012-2102-9
Zera, E. M., Molloy, D. P., Angleson, J. K., Lamture, J. B., Wensel, T. G., and Malinski, J. A. (1996). Low affinity interactions of GDPbetaS and ribose- or phosphoryl-substituted GTP analogues with the heterotrimeric G protein, transducin. J. Biol. Chem. 271, 12925–12931. doi: 10.1074/jbc.271.22.12919
Keywords: sigma-1 receptor, chaperones, ion channels modulation, stress, physiological, cancer
Citation: Crottès D, Guizouarn H, Martin P, Borgese F and Soriani O (2013) The sigma-1 receptor: a regulator of cancer cell electrical plasticity? Front. Physiol. 4:175. doi: 10.3389/fphys.2013.00175
Received: 31 May 2013; Accepted: 21 June 2013;
Published online: 16 July 2013.
Edited by:
Andrea Becchetti, University of Milano-Bicocca, ItalyCopyright © 2013 Crottès, Guizouarn, Martin, Borgese and Soriani. This is an open-access article distributed under the terms of the Creative Commons Attribution License, which permits use, distribution and reproduction in other forums, provided the original authors and source are credited and subject to any copyright notices concerning any third-party graphics etc.
*Correspondence: Olivier Soriani, Faculté des Sciences, Institut de Biologie de Valrose, CNRS UMR7277, INSERM U1091, Université de Nice Sophia Antipolis, Parc Valrose, 06108 Nice 2, France e-mail: soriani@unice.fr