- Laboratory of Endocrine Physiology, Department of Physiological Sciences, Roberto Alcantara Gomes Biology Institute, State University of Rio de Janeiro, Rio de Janeiro, Brazil
Obesity is a global epidemic, and maternal smoking has been shown to be associated with the development of childhood obesity. Overall, approximately 40% of children worldwide are exposed to tobacco smoke at home. It is well known that environmental changes within a critical window of development, such as gestation or lactation, can initiate permanent alterations in metabolism that lead to diseases in adulthood, a phenomenon called programming. It is known that programming is based on epigenetic alterations (changes in DNA methylation, histone acetylation, or small interfering RNA expression) that change the expression pattern of several genes. However, little is known concerning the mechanisms by which smoke exposure in neonatal life programs the adipose tissue and endocrine function. Here, we review several epidemiological and experimental studies that confirm the association between maternal nicotine or tobacco exposure during gestation or lactation and the development of obesity and endocrine dysfunction. For example, a positive correlation was demonstrated in rodents between increased serum leptin in the neonatal period and exposure of the mothers to nicotine during lactation, and the further development of leptin and insulin resistance, and thyroid and adrenal dysfunction, in adulthood in the same offspring. Thus, a smoke-free environment during the lactation period is essential to improving health outcomes in adulthood and reducing the risk for future diseases. An understanding of the pathophysiological mechanisms underlying the effects of smoking on programming can provide new insights into therapeutic strategies for obesity.
Worldwide Epidemics of Overweight and Obesity
Obesity among adults, children, and adolescents has become an urgent problem and reached significant levels in recent years. The World Health Organization (WHO) (2011a) declared obesity a public health problem in 1997. Approximately 65% of the world’s population lives in countries where overweight and obesity kills more people than malnutrition. In 2010, the latest analysis of the IASO/IOTF (International Obesity Taskforce, 2010) estimates that approximately one billion adults are currently overweight and 475 million are obese. Globally, the IASO/IOTF estimates that up to 150 million school-aged children are overweight and 50 million are obese. Nearly 43 million children under 5 years old were overweight in 2010, particularly in urban settings (International Obesity Taskforce, 2010).
A higher body weight is associated with an increased risk of developing chronic diseases, including diabetes mellitus, cardiovascular disease, non-alcoholic fatty liver disease, dyslipidemia, and some of the most common cancers, alongside increased risk of disability (Walls et al., 2012). Although obesity is highly related to genetic factors, other factors may contribute to the high prevalence. A strong association has been shown between changes caused by epigenetic insults that occur in utero or early childhood (especially during the lactation period) that impact the regulation of metabolic pathways related to adipogenesis and complications, including diabetes, hypertension, and dyslipidemia (Monteiro et al., 2004; Doak et al., 2005; Ozanne et al., 2011).
Worldwide Tobacco Consumption
One of the most common pollutants is exposure to cigarette smoke. In 2011, the WHO reported on the Global Tobacco Epidemic, warning about the dangers of tobacco and the impact of smoking cessation interventions (World Health Organization (WHO), 2011b). This same study predicted that if current trends continue, by 2030, smoking will kill one in six people, and the number of female smokers will significantly increase. In addition, this study showed that tobacco is a risk factor for six of the eight leading causes of death worldwide, including cardiac ischemia, pulmonary obstruction, and cerebrovascular diseases, and that most of the second-hand exposures were at home (54 vs. 37% at workplaces).
Globally, between 80,000 and 100,000 children start smoking every day [World Health Organization (WHO), 2009]. Given these data, it is impossible not to consider the effects of cigarette smoke on children. Approximately 40% of children worldwide are exposed to cigarette smoke at home, and 43% have at least one parent who smokes (World Health Organization (WHO), 2009). This early exposure to constituents of cigarette smoke makes children susceptible to respiratory infections, ear infections, growth retardation, and an increased frequency of hospitalizations due to infections (Ladomenou et al., 2009; Yilmaz et al., 2009a). Moreover, these children have decreased serum antioxidant vitamins (A, C, and E), which can contribute to an oxy-cellular redox imbalance and, consequently, increased lipid peroxidation and protein and DNA damage by free radicals present in cigarette smoke (Yilmaz et al., 2009b). Cigarette smoke exposure during pregnancy is related to reduced birth weight, a higher prevalence of sudden infant death syndrome, and impaired child growth, including decreased body mass, length, and head circumference at 3 months of age (Hegaard et al., 2006; Fenercioglu et al., 2009). When cigarette smoke exposure occurs during lactation, changes in milk composition, such as lower total lipid content during the first 6 months of lactation, are observed. Maternal tobacco use also changes cytokine concentrations in human milk; for example, the IL-1 concentration in the colostrum is lower in mothers who smoke compared to non-smoking mothers (Zanardo et al., 2005).
Cigarettes contain numerous toxic compounds, the most important of which are polycyclic aromatic hydrocarbons, nicotine, thiocyanate, and carbon monoxide (Passey et al., 1971). Nicotine, the main addictive compound of tobacco smoke, is an alkaloid produced in the roots of the tobacco plant and has a half-life of 2 h in humans. When nicotine is inhaled, it is quickly transported through the bloodstream across the blood brain barrier and cellular membranes (Rosemberg, 2002). Nicotine binds to nicotinic receptors present in neuromuscular junctions, synapses of the central nervous system and peripheral ganglion (Taylor, 1996).
In adulthood, smoking has controversial effects on body weight and adiposity (Chiolero et al., 2008). It has been shown that smoking decreases body weight, and the smoker becomes heavier after smoke cessation (Molarius et al., 1997). However, some studies have shown the contrary; heavy and chronic smokers had an increased BMI (body mass index), visceral adiposity, and insulin resistance (Ronnemaa et al., 1996; John et al., 2005). The increased visceral adiposity may be explained by the observations that cigarette smoke induces corticosterone secretion (Friedman et al., 1987), decreases testosterone in males (Meikle et al., 1988), and increases androgen in females (Khaw et al., 1988), which are conditions associated with higher visceral fat.
During pregnancy, nicotine crosses the placenta and affects fetal development (DiFranza and Lew, 1995). During lactation, nicotine is transferred through the maternal milk, and the main metabolite, cotinine, can be found in the urine of neonates (Laurberg et al., 2004).
Programming
Stimuli or events that occur during critical periods of life, such as gestation and lactation, can shape future health and disease (Barker, 2003). This phenomenon was first defined as metabolic programming and is now known as developmental plasticity due to its probabilistic rather than deterministic nature (Gluckman and Hanson, 2007; Gluckman et al., 2010). Programming appears to play an important role in the development of obesity, which has raised concerns throughout the international scientific community and led to the creation of a society for the study of health maintenance and origin of diseases during development (DOHaD – Developmental Origins of Health and Disease). Obesity is considered a multifactorial disease, where events, which occur in a critical window of life can act as triggers or adjuncts, making the subject more susceptible to developing metabolic diseases (Walls et al., 2012). Among these events, maternal exposure to different factors, such as nutrients, hormones, and environmental pollutants, plays an important role in obesity development of the offspring (Moura and Passos, 2005; de Moura et al., 2008). In fact, some environmental and dietary chemicals that can mimic or interfere with the normal action of several hormones are termed “endocrine disruptors.” Studies predict the existence of chemical “obesogens,” molecules that inappropriately regulate lipid metabolism and adipogenesis to promote obesity (Grun and Blumberg, 2006; Tabb and Blumberg, 2006).
As schematized in Figure 1, different priming factors could act through different epigenetic mechanisms. It appears that the obesogens could activate the processes of DNA methylation and histone acetylation or increase the levels of some small interference RNAs (siRNA), which can suppress genes related to normal adipogenesis, thereby affecting the developmental plasticity of the adipose tissue depending on the cumulative nutritional status during life (Gluckman et al., 2007; Guilloteau et al., 2009). Epidemiological studies of adults exposed in utero to calorie restriction during the Dutch Hunger in World War II were the first in which DNA hypomethylation at the imprinted IGF2 region in mononuclear cells was demonstrated (Heijmans et al., 2008). Those undernourished babies developed into overweight adults who had a higher cardiovascular risk, confirming the hypothesis that the early life environment can cause epigenetic changes that persist throughout life and provide a mechanistic base for the DOHaD theory. In addition, Suter et al. (2010, 2011a) showed that in utero, tobacco exposure epigenetically modifies placental CYP1A1 expression through a decrease in DNA methylation, and this gene imprinting could explain the lower birth weight of babies of smoking mothers. More recently, the same group (Suter et al., 2011b) showed that a high fat maternal diet in non-human primates during gestation increases histone acetylation at the liver Npas2 gene promoter, which is related to circadian rhythms of lipid and glucose metabolism. Studies have suggested that perinatal tobacco exposure, likely due to the effects of nicotine, induces higher histone acetylation through decreases in the expression and activity of the sirtuins, mainly sirtuin 1, leading to a change in the oxidative stress and inflammatory profile in the offspring (Yang et al., 2007; Volkow, 2011). More information on epigenetic issues has been presented in several recent reviews (Waterland et al., 2008; Hussain, 2012).
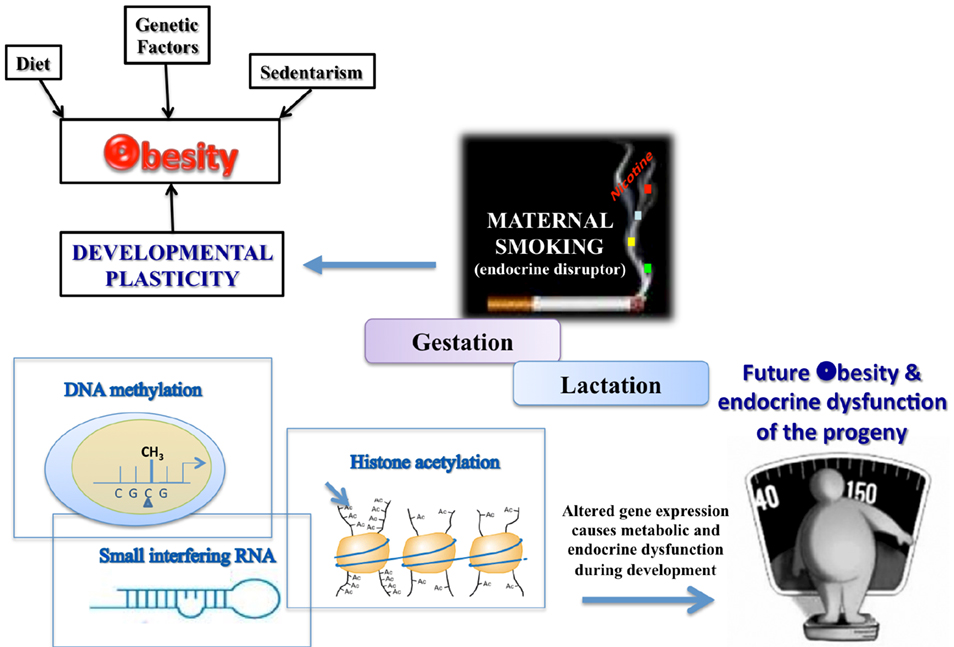
Figure 1. TOBACCO – one of the environmental disruptors in obesity and endocrine dysfunction development of the offspring.
Tobacco and Programming
Epidemiological and Clinical Data
Approximately 250 billion women smoke daily worldwide. Despite the well known harmful effects, many women continue to smoke throughout pregnancy. In the USA, 12% of women continue to smoke during pregnancy, resulting in the birth of at least 400,000 smoke-exposed children per year [Centers for Disease Control and Prevention (CDC), 2012; Martin et al., 2003]. Smoking has been associated with changes in gestation time, miscarriages, and shorter breastfeeding duration (Mello et al., 2001; Einarson and Riordan, 2009). During pregnancy, tobacco smoking is considered a risk factor for perinatal mortality, low birth weight, and neurological abnormalities (DiFranza and Lew, 1995). Nicotine acts as a potent vasoconstrictor, reducing the oxygen supply to the fetus and affecting development. During lactation, nicotine is transferred through the breast milk (Luck and Nau, 1987; Dahlström et al., 2004) and can act directly on the newborn’s metabolism. In fact, cotinine can be detected in the urine of neonates from smoking mothers. Suckling from a smoking mother has been shown to cause tachycardia in an infant, suggesting an adrenergic effect and demonstrating that nicotine causes important changes in breastfeeding infants (Laurberg et al., 2004).
Several reports have demonstrated an association between maternal smoking during pregnancy and the development of obesity and hypertension in childhood and adolescence (Von Kries et al., 2002; Wideroe et al., 2003; Goldani et al., 2007). However, it is not clear how different effects of maternal smoking can be synergistic or additive in the development of obesity in the offspring of smoking mothers. In a Chinese population, some parameters of metabolic syndrome, such as hypertriglyceridemia, central obesity, a decreased HDL-c and an increased level of fasting serum insulin in the adult offspring, have been associated with maternal cigarette smoke exposure (Xie et al., 2010). Metabolic changes in children exposed to cigarette smoke are related to the risk of developing atherosclerosis and diabetes. Epidemiological studies have shown decreased serum HDL-c in children exposed to cigarette smoke and increased markers of cardiovascular risk, including leptin, C-reactive protein, and IL-6, and decreased adiponectin concentrations (Nagel et al., 2009). Smoke exposure is also associated with the presence of autoantibodies to pancreatic islet cells, which would be the first step in the development of type 1 diabetes (Skrodenienė et al., 2008).
Additionally, tobacco smoke affects hormonal secretion, an effect that can program late endocrine dysfunctions. After the consumption of a few cigarettes, increases in ADH, GH, glucocorticoids, epinephrine, and leptin can be observed (Yeh and Barbieri, 1989; Grassi et al., 1994; Walker et al., 1999; Reseland et al., 2005). Even when non-smokers inhale tobacco smoke, it can act on different endocrine-metabolic parameters. In studies in which volunteers were subjected to environmental tobacco smoke, similar to a profile found in a bar/restaurant, increases of resting energy expenditure and serum thyroid hormones were observed with only 1 h of exposure (Metsios et al., 2007). In a similar study, the same increase in thyroid hormones was observed, along with increased production of the pro-inflammatory cytokine IL-1β and increased systolic blood pressure (Flouris et al., 2008). These hormonal changes should be further investigated because children of smoker mothers may have a higher risk of developing endocrine diseases later in life.
Experimental Data
For a better understanding of the role of tobacco smoke in the pathophysiology of obesity, researchers have used experimental models that allow the study of each tobacco component that may be involved in the development of obesity and, thus, provide a mechanistic basis prevention and treatment.
Experimental data have confirmed the epidemiological data associating maternal smoking during pregnancy with the development of obesity (Gao et al., 2005; Holloway et al., 2005; Somm et al., 2008). Williams and Kanagasabai (1984) showed that fetal nicotine exposure in rats increases body fat in the fetus on the 20th day of gestation (birth on the 21st day), suggesting that fetal nicotine exposure results in increased adiposity in the offspring. Newman et al. (1999) observed that rats exposed to nicotine in utero are heavier at 9 weeks of age compared with controls. In rodents, nicotine exposure from gestation to the 10th day of lactation increased body weight in offspring at 35 days of age. In male offspring, this effect was transient, but in female offspring, the higher body weight persisted until 90 days of age (Chen and Kelly, 2005). In addition, rats whose mothers were treated with nicotine for 14 days before mating and during pregnancy until weaning were heavier at 70 days of age compared with controls and had higher body weight and visceral adipose tissue at 180 days of age (Gao et al., 2005). In addition to obesity, adult animals whose mothers were exposed to nicotine during both gestation and lactation also display insulin resistance, glucose intolerance, cold intolerance, a reduction in spontaneous physical activity, and higher risk of developing cardiovascular diseases (Bruin et al., 2007; Somm et al., 2008, 2009).
It is interesting to note that most studies have investigated the effects of nicotine in rodents during gestation, which is equivalent in terms of neural development to the first two trimesters of gestation in humans. Considering neural development, lactation in rats is thought to be equivalent to the third trimester of human gestation (Quinn, 2005; Clancy et al., 2007). For eutherian mammals, the function of lactation is to provide the primary source of nutrition and immune protection to the offspring after birth (Golding et al., 1997). Because this period is critical to cognitive and neurological development, adverse environmental changes can predispose newborns to the development of some diseases in adulthood (Mott et al., 1991; Symonds, 2007). Nevertheless, despite experimental data of smoking/nicotine programming during pregnancy or gestation and lactation, to the best of our knowledge, there are few studies that have evaluated the effects of nicotine or tobacco exposure exclusively during the early postnatal period. This may be of particular relevance because there is a high rate of smoking relapse among women who quit smoking during pregnancy (McBride and Pirie, 1990). In fact, our group suggested that the postnatal period is critical for nicotine programming of body weight and fat distribution. Despite the differences in the neural development of rats and humans, studies that focus exclusively on the lactation period are important in evaluating the possible programming effects of neonatal exposure to cigarette smoke.
Hormonal changes associated with nicotine/tobacco exposure during lactation
Our group has shown that maternal nicotine exposure (6 mg/kg/day) from day 2 to 16 of lactation causes neonatal hyperleptinemia and primary hypothyroidism and programs for overweight, higher visceral adiposity, adipocyte hypertrophy, hyperleptinemia, leptin and insulin resistance, and secondary hypothyroidism in adult rat offspring (Oliveira et al., 2009; de Oliveira et al., 2010).
Increased body fat is associated with higher levels of leptin (Friedman and Halaas, 1998); however, the association between tobacco/nicotine and leptin levels is still controversial because both hyperleptinemia (Hodge et al., 1997; Eliasson and Smith, 1999; Nicklas et al., 1999) and hypoleptinemia (Wei et al., 1997; Donahue et al., 1999) have been found in tobacco smokers. The significance of higher serum leptin levels and the effect on leptin action are unclear because in most cases, it is associated with leptin resistance. Nevertheless, leptin resistance can be tissue-specific. It has been shown (Rahmouni et al., 2008) that in some knockout mice models of Bardet–Biedl obesity syndrome, leptin resistance has anorexigenic effects; however, leptin preserves its effects on renal sympathetic nerve activity. Our group also demonstrated that even in the sympathetic nervous system (SNS), a peripheral adrenal medulla leptin resistance is present, while the central leptin action upon the SNS is preserved (Trevenzoli et al., 2010).
Some leptin effects on energy balance may be mediated by the hypothalamus-pituitary-thyroid axis and peripheral metabolism of thyroid hormones (Ahima et al., 1996; Légradi et al., 1997; Friedman and Halaas, 1998; Seoane et al., 2000; Nowak et al., 2002; Ortiga-Carvalho et al., 2002; Lisboa et al., 2003). In fact, disturbances of thyroid function are associated with marked changes in both energy expenditure and body weight, with leptin and thyroid hormones appearing to play mutually important roles. It is well known that tobacco can affect the thyroid gland (Christensen et al., 1984; Ericsson and Lindgrade, 1991; Fisher et al., 1997; Utiger, 1998), and although there are few studies concerning the effect of tobacco compounds on thyroid function, thiocyanate, not nicotine, is frequently related to hypothyroidism and goiter (Muller et al., 1995; Dai et al., 1996; Fukata et al., 1996; Eskandari et al., 1997). Maternal smoking influences the thyroid function of the newborn differently, likely according to the levels of iodine-intake. In newborns of normal iodine-intake mothers, it appears that smoke is related to neonatal hyperthyroidism suppressing TSH (Meberg and Marstein, 1986), while in borderline iodine-intake mothers, smoke exposure is associated with goiter (Chanoine et al., 1991). According to Laurberg et al. (2004), smoking mothers have lower iodide content in breast milk, and their offspring have lower urinary iodide. This study suggests that nicotine decreases maternal milk iodide transfer.
As we previously described thyroid hypofunction in rat pups after maternal exposure to nicotine, we decided to evaluate the transfer of iodine through milk in this model (de Oliveira et al., 2011). As schematized in Figure 2, during nicotine exposure, mothers showed decreased serum T4 and mammary radioiodine uptake (RAIU) and increased serum TSH and thyroid RAIU, followed by higher T3 content in milk. In addition to decreased T3 and T4 and increased TSH levels, pups from nicotine mothers showed lower thyroid RAIU. Interestingly, at weaning after nicotine withdrawal, the pups recovered thyroid function, likely due to the increased lactational transfer of T3. Therefore, we demonstrated that the thyroid function of both mothers and pups was affected by early nicotine exposure. The likely mechanism of the pups’ primary hypothyroidism is decreased leptin signaling in the thyroid. However, long-term thyroid hypofunction is related to central and peripheral leptin resistance in adulthood (Santos-Silva et al., 2010).
In another study (Pinheiro et al., 2011), we investigated the association of leptin and adrenal hormones in adult male offspring whose mothers were treated with nicotine during lactation. These progeny presented with higher production of adrenal catecholamine but lower catecholamine secretion. They also displayed hypothalamic-pituitary-adrenal axis hyperfunction, characterized by an increase in both CRH and ACTH immunostaining in the hypothalamus and pituitary and higher serum corticosterone levels. Because obesity depends on SNS and hypothalamic-pituitary-adrenal function, these findings could be associated with a higher probability of developing obesity due to lower catecholamine secretion (reducing the lipolytic effects of SNS) and higher corticosterone action (increasing visceral adipogenesis).
Thus, our studies identified lactation as a critical period for nicotine programming for obesity, with both thyroid and adrenal dysfunctions being possible contributing factors. In addition, through the effects of nicotine, perinatal maternal smoking can be responsible for the future development of some components of the metabolic syndrome in offspring.
Table 1 presents some findings concerning a sex dimorphism in a model of maternal nicotine exposure during lactation. Adrenal function in adult offspring was programmed only in male offspring, while female offspring showed only changes in leptin content in muscle and adipocytes (Pinheiro et al., 2011). Thus, males may be more susceptible to maternal smoke-induced obesity than females. It is possible that the resistance to the programming found in female rats is an evolutionary advantage because the environmental changes are not fully transmitted to the progeny. However, there is lack of epidemiological studies to support this hypothesis.
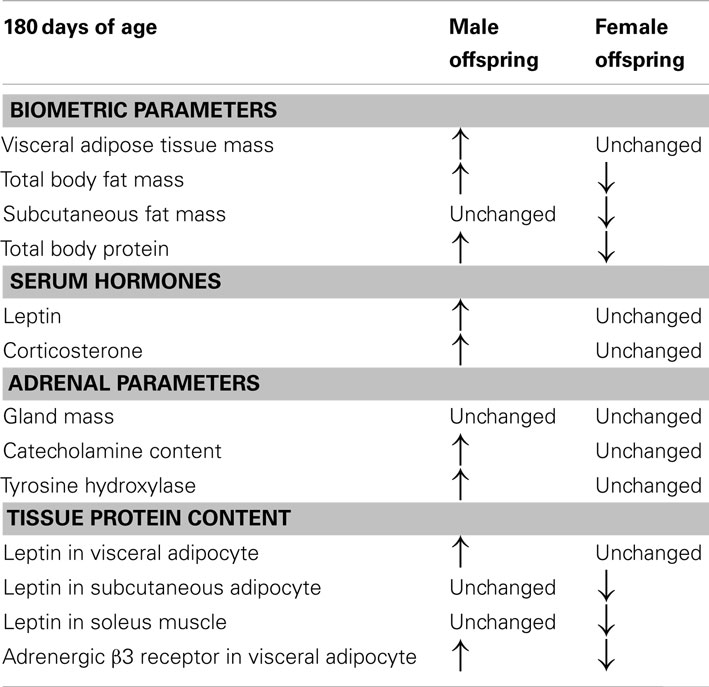
Table 1. Comparison between some parameters of adult (180 days of age) male and female rat progeny whose mothers were nicotine-treated only during lactation period.
A cigarette contains more than 4,500 compounds in addition to nicotine. Therefore, it is interesting to evaluate the effects of total cigarette exposure during lactation. To evaluate this possible combined programming effect of cigarette compounds, we more recently developed a model of maternal cigarette smoking exposure during lactation to investigate some nutritional, biochemical, and hormonal parameters in dams and pups. In this experiment, lactating rats were submitted to a smoking machine 4 times/day/h for each exposure (1.7 mg nicotine/cigarette) during the lactation period without pups to attempt to reproduce the human conditions in which the lactating mother only smokes far from her baby. Different from the pups whose mothers were exposed solely to nicotine, the pups from smoke-exposed mothers did not exhibit neonatal thyroid dysfunction and had lower body adiposity. In addition, they had lower adrenal catecholamine content and lower serum corticosterone levels at weaning (Santos-Silva et al., 2011). The only programming effect noted in the offspring was decreased catecholamine production in the adrenal gland at adulthood, a dysfunction that can lead to long-lasting changes in the stress response. However, these adult offspring surprisingly had no changes in body adiposity or serum hormone profile (Santos-Silva et al., 2012). Other cigarette components may inhibit the obesogenic effect of nicotine, or more likely, the time period of exposure (4 h/day) was not adequate to transfer enough nicotine to the pups. Another point to consider is that the newborn may be exposed to cigarette smoke in the environment because relatives other than the mother, such the father, can also smoke. Thus, we are studying another model in which the pups stay with the mother during the full period of cigarette smoke exposure in the smoke chamber.
To clearly elucidate the effect of tobacco programming, Tables 2 and 3 depict the major changes found into two models of smoke exposure during lactation in rodents, namely, maternal nicotine exposure (Oliveira et al., 2010) and cigarette smoke exposure (Santos-Silva et al., 2011). Our comparison of these two experimental models suggest that some changes result specifically from nicotine exposure, such as thyroid and adrenal dysfunction, serum HDL-c and leptinemia. Surprisingly, some of the nicotine effects were blunted when the animals were exposed to the cigarette smoke, such as thyroid dysfunction and hyperleptinemia. In addition, components of tobacco other than nicotine may be responsible for the alterations in triglycerides and LDL-c.
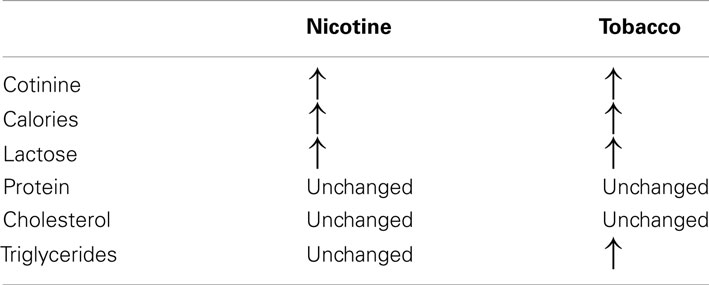
Table 2. Comparison between milk composition in the models of maternal nicotine and tobacco smoke-exposed during lactation.
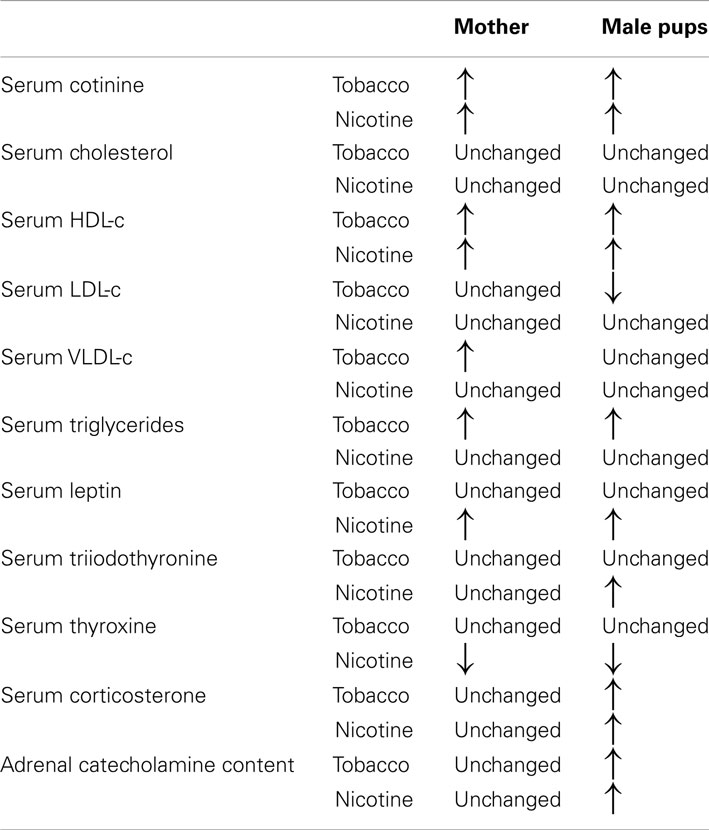
Table 3. Comparison between metabolic and endocrine changes in the models of maternal nicotine and tobacco smoke-exposed during lactation.
Our experimental data and data from the literature (experimental, clinical, and epidemiological) show that maternal smoking, mainly through nicotine/tobacco smoke exposure in early life, can significantly influence neonate development, with an emphasis on obesity.
Concluding Remarks – Perspectives
Smoking during pregnancy and lactation remains a major scientific and public health concern and are associated with numerous short- and long-term adverse effects. We can learn from the developmental programming induced by maternal smoking, and its future impact on endocrine dysfunction and obesity that in addition to its clear toxic effects, tobacco smoke has long-term effects contributing to the obesity epidemic.
We do not yet know all of the mechanisms by which this early life exposure leads to the observed changes in adipogenesis or hormonal changes, including epigenetic alterations. Unanswered questions remain concerning the role of hormonal or transcript factors, such as PPARgamma, on the adipogenesis in this condition and how the different factors that are changed during the neonatal period combine to increase the probability of these reported developmental changes.
The possibility that tobacco compounds can make children who are exposed to cigarette smoke during the gestation and/or lactation periods, through blood cord, milk, or environment, more susceptible to obesity and other metabolic and endocrine disorders in adulthood warrants clinical, epidemiological, prospective, and experimental studies that support a more assertive health policy by governments toward education about the risk of cigarette smoke, at least in vulnerable periods of life, such gestation, lactation, and infancy.
Conflict of Interest Statement
The authors declare that the research was conducted in the absence of any commercial or financial relationships that could be construed as a potential conflict of interest.
Acknowledgments
Patricia Cristina Lisboa, Elaine de Oliveira, and Egberto Gaspar de Moura are researchers from State University of Rio de Janeiro, which have research projects supported by the “National Council for Scientific and Technological Development” (Conselho Nacional de Desenvolvimento Científico e Tecnológico-CNPq), the “Carlos Chagas Filho Research Foundation of the State of Rio de Janeiro” (Fundação Carlos Chagas Filho de Amparo à Pesquisa do Estado do Rio de Janeiro-FAPERJ) and Coordination for the Enhancement of Higher Education Personnel (Coordenação de Aperfeiçoamento de Pessoal de Nível Superior – CAPES).
References
Ahima, R. S., Prabakaran, D., Mantzoros, C., Qu, D., Lowell, B., Maratos-Flier, E., et al. (1996). Role of leptin in the neuroendocrine response to fasting. Nature 382, 250–252.
Bruin, J. E., Kellenberger, L. D., Gerstein, H. C., Morrison, K., and Holloway, A. C. (2007). Fetal and neonatal nicotine exposure and postnatal glucose: identifying critical windows of exposure. J. Endocrinol. 194, 171–178.
Centers for Disease Control and Prevention (CDC). (2012). Smoking and Tobacco Use. Available at: http://www.cdc.gov/tobacco/ [accessed May 8, 2012]
Chanoine, J. P., Toppet, V., Bourdoux, P., Spehl, M., and Delange, F. (1991). Smoking during pregnancy: a significant cause of neonatal thyroid enlargement. BJOG 98, 65–68.
Chen, W. J., and Kelly, R. B. (2005). Effect of prenatal or perinatal nicotine exposure o neonatal thyroid status and offspring growth in rats. Life Sci. 76, 1249–1258.
Chiolero, A., Faeh, D., Paccaud, F., and Cornuz, J. (2008). Consequences of smoking for body weight, body distribution and insulin resistance. Am. J. Clin. Nutr. 87, 801–809.
Christensen, S. B., Ericsson, U. B., Janzon, L., Tibblin, S., and Melander, A. (1984). Influence of cigarette smoking on goiter formation, thyroglobulin, and thyroid hormone levels in women. J. Clin. Endocrinol. Metab. 58, 615–618.
Clancy, B., Finlay, B. L., Darlington, R. B., and Anand, K. J. (2007). Extrapolating brain development from experimental species to humans. Neurotoxicology 28, 931–937.
Dahlström, A., Ebersjö, C., and Lundell, B. (2004). Nicotine exposure in breastfed infants. Acta Paediatr. 93, 810–816.
Dai, Z., Gao, J., An, K., Lee, J. M., Edwards, G. E., and An, G. (1996). Promoter elements controlling developmental and environmental regulation of a tobacco ribosomal protein gene L34. Plant Mol. Biol. 32, 1055–1065.
de Moura, E. G., Lisboa, P. C., and Passos, M. C. (2008). Neonatal programming of neuroimmunomodulation role of adipocytokines and neuropeptides. Neuroimmunomodulation 15, 176–188.
de Oliveira, E., de Moura, E. G., Santos-Silva, A. P., Pinheiro, C. R., Claudio-Neto, S., Christian Manhães, A., et al. (2011). Neonatal hypothyroidism caused by maternal nicotine exposure is reversed by higher T3 transfer by milk after nicotine withdraw. Food Chem. Toxicol. 49, 2068–2073.
de Oliveira, E., Moura, E. G., Santos-Silva, A. P., Pinheiro, C. R., Lima, N. S., Nogueira-Neto, J. F., et al. (2010). Neonatal nicotine exposure causes insulin and leptin resistance and inhibits hypothalamic leptin signaling in adult rats offspring. J. Endocrinol. 206, 55–63.
DiFranza, J. R., and Lew, R. A. (1995). Effect of maternal cigarette smoking on pregnancy complications and sudden infant death syndrome. J. Fam. Pract. 40, 385–394.
Doak, C. M., Adair, L. S., Bentley, M., Monteiro, C., and Popkin, B. M. (2005). The dual burden household and the nutrition transition paradox. Int. J. Obes. 29, 129–136.
Donahue, R. P., Zimmet, P., Bean, J. A., Decourten, M., DeCarlo Donahue, R. A., Collier, G., et al. (1999). Cigarette smoking, alcohol use, and physiological activity in relation to serum leptin levels in a multiethnic population: The Miami Community Health Study. Ann Epidemiol 9, 108–113.
Einarson, A., and Riordan, S. (2009). Smoking in pregnancy and lactation: a review of risks and cessation strategies. Eur. J. Clin. Pharmacol. 65, 325–330.
Eliasson, B., and Smith, U. (1999). Leptin levels in smokers and long-term users of nicotine gum. Eur. J. Clin. Invest. 29, 145–152.
Ericsson, U. B., and Lindgrade, F. (1991). Effect of cigarette smoking on thyroid function and the prevalence of goitre, thyrotoxicosis and autoimmune thyroiditis. J. Intern. Med. 229, 67–71.
Eskandari, S., Loo, D. D. F., Dai, G., Levy, O., Wright, E. M., and Carrasco, N. (1997). Thyroid Na/I symporter. J. Biol. Chem. 43, 27230–27238.
Fenercioglu, A. K., Tamer, I., Karatekin, G., and Nuhoglu, A. (2009). Impaired postnatal growth of infants prenatally exposed to cigarette smoking. Tohoku J. Exp. Med. 218, 221–228.
Fisher, C. L., Mannino, D. M., Herman, W. H., and Frumkin, H. (1997). Cigarette smoking and thyroid hormone levels in males. Int. J. Epidemiol. 26, 972–977.
Flouris, A. D., Metsios, G. S., Jamurtas, A. Z., and Koutedakis, Y. (2008). Sexual dimorphism in the acute effects of secondhand smoke on thyroid hormone secretion, inflammatory markers and vascular function. Am. J. Physiol. Endocrinol. Metab. 294, E456–E462.
Friedman, A. J., Ravnikar, V. A., and Barbieri, R. L. (1987). Serum steroid hormone profiles in postmenopausal smokers and nonsmokers. Fertil. Steril. 47, 398–401.
Friedman, J. M., and Halaas, J. L. (1998). Leptin and the regulation of body weight in mammals. Nature 395, 763–770.
Fukata, S., Kuma, K., and Sugawara, M. (1996). Relationship between cigarette smoking and hypothyroidism in patients with Hashimoto’s thyroiditis. J. Endocrinol. Invest. 19, 607–612.
Gao, Y. J., Holloway, A. C., Zeng, Z. H., Lim, G. E., Petrik, J. J., Foster, W. G., et al. (2005). Prenatal exposure to nicotine causes postnatal obesity and altered perivascular adipose tissue function. Obes. Res. 13, 687–692.
Gluckman, P. D., and Hanson, M. A. (2007). Developmental plasticity and human disease: research directions. J. Intern. Med. 261, 461–471.
Gluckman, P. D., Hanson, M. A., and Beedle, A. S. (2007). Early life events and their consequences for later disease: a life history and evolutionary perspective. Am. J. Hum. Biol. 19, 1–19.
Gluckman, P. D., Hanson, M. A., and Buklijas, T. (2010). A conceptual framework for the developmental origins of health and disease. J. Dev. Orig. Health Dis. 1, 6–18.
Goldani, M. Z., Haeffner, L. S. B., Agranonik, M., Barbieri, M. A., Bettiol, H., and Silva, A. A. M. (2007). Do early life factors influence body mass index in adolescents? Braz. J. Med. Biol. Res. 40, 1231–1236.
Golding, J., Rogers, I. S., and Emmett, P. M. (1997). Association between breast feeding, child development and behaviour. Early Hum. Dev. 29, S175–S184.
Grassi, G., Seravalle, G., Calhoun, D. A., Bolla, G. B., Giannattasio, C., Marabini, M., et al. (1994). Mechanisms responsible for sympathetic activation by cigarette smoking in humans. Circulation 90, 248–253.
Grun, F., and Blumberg, B. (2006). Environmental obesogens: organotins and endocrine disruption via nuclear receptor signaling. Endocrinology 147, S50–S55.
Guilloteau, P., Zabielski, R., Hammon, H. M., and Metges, C. C. (2009). Adverse effects of nutritional programming during prenatal and early postnatal life, some aspects of regulation and potential prevention and treatments. J. Physiol. Pharmacol. 60(Suppl. 3), 17–35.
Hegaard, H. K., Kjaergaard, H., Møller, L. F., Wachmann, H., and Ottesen, B. (2006). The effects of environmental tobacco smoke during pregnancy on birth weight. Acta Obstet. Gynecol. Scand. 85, 675–681.
Heijmans, B. T., Tobi, E. W., Stein, A. D., Putter, H., Gerard, J. B., Susser, E. S., et al. (2008). Persistent epigenetic differences associated with prenatal exposure to famine in humans. Proc. Natl. Acad. Sci. U.S.A. 105, 17046–17049.
Hodge, A. M., Westerman, R. A., de Courten, M. P., Collier, G. R., Zimmet, P. Z., and Alberti, K. G. (1997). Is leptin sensitivity the link between smoking cessation and weight gain? Int. J. Obes. Relat. Metab. Disord. 21, 50–53.
Holloway, A. C., Lim, G. E., Petrik, J. J., Foster, W. G., Morrison, K. M., and Gerstein, H. C. (2005). Fetal and neonatal exposure to nicotine in Wistar rats results in increased beta cell apoptosis at birth and postnatal endocrine and metabolic changes associated with type 2 diabetes. Diabetologia 48, 2661–2666.
Hussain, N. (2012). Epigenetic influences that modulate infant growth, development, and disease (Review). Antioxid. Redox Signal. 17, 224–236.
International Obesity Taskforce. (2010). The Global Epidemic. IASO/IOTB. Available at: http://www.iaso.org/iotf/obesity/obesitytheglobalepidemic/ [accessed May, 2012]
John, U., Hanke, M., Rumpf, H. J., and Thyrian, J. R. (2005). Smoking status, cigarettes per day, and their relationship to overweight and obesity among former and current smokers in a national adult general population sample. Int. J. Obes. Relat. Metab. Disord. 29, 1289–1294.
Khaw, K. T., Tazuke, S., and Barrett-Connor, E. (1988). Cigarette smoking and levels of adrenal androgens in postmenopausal women. N. Engl. J. Med. 318, 1705–1709.
Ladomenou, F., Kafatos, A., and Galanakis, E. (2009). Environmental tobacco smoke exposure as a risk factor for infections in infancy. Acta Paediatr. 98, 1137–1141.
Laurberg, P., Nøhr, S. B., Pedersen, K. M., and Fuglsang, E. J. (2004). Iodine nutrition in breast-fed infants is impaired by maternal smoking. J. Clin. Endocrinol. Metab. 89, 181–187.
Légradi, G., Emerson, C. H., Ahima, R. S., Flier, J. S., and Lechan, M. (1997). Leptin prevents fasting-induced suppression of prothyrotropin-releasing hormone messenger ribonucleic acid in neurons of the hypothalamic paraventicular nucleus. Endocrinology 138, 2569–2576.
Lisboa, P. C., Oliveira, K. J., Cabanelas, A., Ortiga-Carvalho, T. M., and Pazos-Moura, C. C. (2003). Acute cold exposure, leptin, and somatostatin analog (octreotide) modulate thyroid 5′-deiodinase activity. Am. J. Physiol. Endocrinol. Metab. 284, E1172–E1176.
Luck, W., and Nau, H. (1987). Nicotine and cotinine concentrations in the milk of smoking mothers: influence of cigarette consumption and diurnal variation. Eur. J. Pediatr. 146, 21–26.
Martin, J. A., Hamilton, B. E., Sutton, P. D., Ventura, S. J., Menacker, F., and Munson, M. L. (2003). Births: final data for 2002. Natl. Vital. Stat. Rep. 52, 1–113.
Meberg, A., and Marstein, S. (1986). Smoking during pregnancy – effects on the fetal thyroid function. Acta Paediatr. Scand. 75, 762–766.
Meikle, A. W., Liu, X. H., Taylor, G. N., and Stringham, J. D. (1988). Nicotine and cotinine effects on 3 alpha hydroxysteroid dehydrogenase in canine prostate. Life Sci. 43, 1845–1850.
Mello, P. R. B., Pinto, G. R., and Botelho, C. (2001). The influence of smoking on fertility, pregnancy and lactation. J. Pediatr. 77, 257–264.
Metsios, G. S., Flouris, A. D., Jamurtas, A. Z., Carrillo, A. E., Kouretas, D., Germenis, A. E., et al. (2007). A brief exposure to moderate passive smoke increases metabolism and 507 thyroid hormone secretion. J. Clin. Endocrinol. Metab. 92, 208–211.
Molarius, A., Seidell, J. C., Kuulasmaa, K., Dobson, A. J., and Sans, S. (1997). Smoking and relative body weight: an international perspective from the WHO MONICA Project. J. Epidemiol. Community Health 51, 252–260.
Monteiro, C. A., Conde, W. L., Lu, B., and Popkin, M. B. (2004). Obesity and inequities in health in the developing world. Int. J. Obes. (Lond.) 28, 1181–1186.
Mott, G. E., Lewis, D. S., and McGill, H. C. Jr. (1991). Programming of cholesterol metabolism by breast or formula feeding. Ciba Found. Symp. 156, 56–66.
Moura, E. G., and Passos, M. C. (2005). Neonatal programming of body weight regulation and energetic metabolism. Biosci. Rep. 25, 251–269.
Muller, B., Zulewski, H., Huber, P., Ratcliffe, J. G., and Staub, J. J. (1995). Impaired action of thyroid hormone associated with smoking in women with hypothyroidism. N. Engl. J. Med. 333, 964–969.
Nagel, G., Arnold, F. J., Wilhelm, M., Link, B., Zoellner, I., and Koenig, W. (2009). Environment tobacco smoke and cardiometabolic risk in young children: results from a survey in south-west Germany. Eur. Heart J. 30, 1885–1893.
Newman, M. B., Shytle, R. D., and Sanberg, P. R. (1999). Locomotor behavioral effects of prenatal and postnatal nicotine exposure in rat offspring. Behav. Pharmacol. 10, 699–706.
Nicklas, B. J., Tomoyasu, N., Muir, J., and Goldberg, A. P. (1999). Effects of cigarette smoking and its cessation on body weight and plasma leptin levels. Metab. Clin. Exp. 48, 804–808.
Nowak, K. M., Kaczmarek, P., Mackowiak, P., Ziolkowska, A., Albertin, G., Ginda, W. J., et al. (2002). Rat thyroid gland expresses the long form of leptin receptors, and leptin stimulates the function of the gland in euthyroid non-fasted animals. Int. J. Mol. Med. 9, 31–34.
Oliveira, E., Moura, E., Santos-Silva, A., Fagundes, A., Rios, A., Abreu-Villaca, Y., et al. (2009). Short and long-term effects of maternal nicotine exposure during lactation on body adiposity, lipid profile and thyroid function of rat offspring. J. Endocrinol. 202, 397–405.
Oliveira, E., Pinheiro, C. R., Santos-Silva, A. P., Trevenzoli, I. H., Abreu-Villaça, Y., Nogueira-Neto, J. F., et al. (2010). Nicotine exposure affects mother’s and pup’s nutritional, biochemical, and hormonal profiles during lactation in rats. J. Endocrinol. 205, 159–170.
Ortiga-Carvalho, T. M., Oliveira, K. L., Soares, B. A., and Pazos-Moura, C. C. (2002). The role of leptin in the regulation of TSH secretion in the fed state: in vivo and in vitro studies. J. Endocrinol. 174, 121–125.
Ozanne, S. E., Sandovici, I., and Constância, M. (2011). Maternal diet, aging and diabetes meet at a chromatin loop. Aging 3, 548–554.
Passey, R. D., Blackmore, M., Warbrick-Smith, D., and Jones, R. (1971). Smoking risks of different tobaccos. Br. Med. J. 4, 198–201.
Pinheiro, C. R., Oliveira, E., Trevenzoli, I. H., Manhães, A. C., Santos-Silva, A. P., Younes-Rapozo, V., et al. (2011). Developmental plasticity in adrenal function and leptin production primed by nicotine exposure during lactation: gender differences in rats. Horm. Metab. Res. 43, 693–701.
Quinn, R. (2005). Comparing rat’s to human’s age: how old is my rat in people years? Nutrition 21, 775–777.
Rahmouni, K., Fath, M. A., Seo, S., Thedens, D. R., Berry, C. J., Weiss, R., et al. (2008). Leptin resistance contributes to obesity and hypertension in mouse models of Bardet-Biedl syndrome. J. Clin. Invest. 118, 1458–1467.
Reseland, J. E., Mundal, H. H., Hollung, K., Haugen, F., Zahid, N., Anderssen, S. A., et al. (2005). Cigarette smoking may reduce plasma leptin concentration via catecholamines. Prostaglandins Leukot. Essent. Fatty Acids 73, 43–49.
Ronnemaa, T., Ronnemaa, E. M., Puukka, P., Pyorala, K., and Laakso, M. (1996). Smoking is independently associated with high plasma insulin levels in nondiabetic men. Diabetes Care 19, 1229–1232.
Rosemberg, J. (2002). Pandemia do tabagismo – Enfoques Históricos e Atuais. São Paulo: Secretaria de Saúde do Estado de Sao Paulo – SES – CIP e CVE, 184.
Santos-Silva, A. P., Lisboa, P. C., Pinheiro, C. R., Maia, L. A., Peixoto-Silva, N., Abreu-Villaça, Y., et al. (2012). Maternal tobacco smoke exposure during lactation inhibits catecholamine production by adrenal medullae in adult rat offspring. Horm. Metab. Res. 44, 550–554.
Santos-Silva, A. P., Moura, E. G., Pinheiro, C. R., Rios, A. S., Abreu-Villaça, Y., Passos, M. C., et al. (2010). Neonatal nicotine exposure alters leptin signaling in the hypothalamus-pituitary-thyroid axis in the late postnatal period and adulthood in rats. Life Sci. 87, 187–195.
Santos-Silva, A. P., Oliveira, E., Pinheiro, C. R., Nunes Freitas, A. L., Abreu-Villaça, Y., Santana, A., et al. (2011). Effects of tobacco smoke exposure during lactation on nutritional and hormonal profiles in mothers and offspring. J. Endocrinol. 209, 75–84.
Seoane, L. M., Carro, E., Tovar, S., Casanueva, F. F., and Dieguez, C. (2000). Regulation in vivo TSH secretion by leptin. Regul. Pept. 92, 25–29.
Skrodenienė, E., Marciulionyte, D., Padaiga, Z., Jašinskienė, E., Sadauskaite-Kuehne, V., and Ludvigsson, J. (2008). Environmental risk factors in prediction of childhood prediabetes. Medicina (Kaunas) 44, 56–63.
Somm, E., Schwitzgebel, V. M., Vauthay, D. M., Aubert, M. L., and Hüppi, P. S. (2009). Prenatal nicotine exposure and the programming of metabolic and cardiovascular disorders. Mol. Cell. Endocrinol. 304, 69–77.
Somm, E., Schwitzgebel, V. M., Vauthay, D. M., Camm, E. J., Chen, C. Y., Giacobino, J. P., et al. (2008). Prenatal nicotine exposure alters early pancreatic islet and adipose tissue development with consequences and the control of body weight and glucose metabolism later in life. Endocrinology 149, 6289–6299.
Suter, M., Abramovici, A., Showalter, L., Hu, M., Shope, C., Varner, M., et al. (2010). In utero tobacco exposure epigenetically modifies placental cyp1a1 expression. Metab. Clin. Exp. 59, 1481–1490.
Suter, M., Ma, J., Harris, A., Patterson, L., Brown, K. A., Shope, C., et al. (2011a). Maternal tobacco use modestly alters correlated epigenome-wide placental DNA methylation and gene expression. Epigenetics 6, 1284–1294.
Suter, M., Bocock, P., Showalter, L., Hu, M., Shope, C., McKnight, R., et al. (2011b). Epigenomics: maternal high-fat diet exposure in utero disrupts peripheral circadian gene expression in nonhuman primates. FASEB J. 25, 714–726.
Symonds, M. E. (2007). Integration of physiological and molecular mechanisms of the developmental origins of adult disease: new concepts and insights. Proc. Nutr. Soc. 66, 442–450.
Tabb, M. M., and Blumberg, B. (2006). New modes of action for endocrine-disrupting chemicals. Mol. Endocrinol. 20, 475–482.
Taylor, P. (1996). “Agents acting at the neuromuscular junction and autonomic, neuromuscular junction and autonomic ganglia,” in The Pharmacological Basis of Therapeutics, 9th Edn, eds J. G. Hardman, L. E. Limbird, P. B. Molinoff, R. W. Ruddon, and A. G. Gilman (New York: McGraw-Hill), 177–197.
Trevenzoli, I. H., Pinheiro, C. R., Conceição, E. P., Oliveira, E., Passos, M. C., Lisboa, P. C., et al. (2010). Programming of rat adrenal medulla by neonatal hyperleptinemia: adrenal morphology, catecholamine secretion, and leptin signaling pathway. Am. J. Physiol. Endocrinol. Metab. 298, E941–E949.
Volkow, N. D. (2011). Epigenetics of nicotine: another nail in the coughing. Sci. Transl. Med. 3, 107–143.
Von Kries, R., Toschke, A. M., Koletzko, B., and Slikker, M. Jr. (2002). Maternal smoking during pregnancy and childhood obesity. Am. J. Epidemiol. 156, 954–961.
Walker, J. F., Collins, L. C., Rowell, P. P., Goldsmith, L. J., Moffatt, R. J., and Stamford, B. A. (1999). The effect of smoking on energy expenditure and plasma catecholamine and nicotine levels during light physical activity. Nicotine Tob. Res. 1, 365–370.
Walls, H. L., Backholer, K., Proietto, J., and McNeil, J. J. (2012). Obesity and trends in life expectancy. J. Obes. doi:10.1155/2012/107989
Waterland, R. A., Travisano, M., Tahiliani, K. G., Rached, M. T., and Mirza, S. (2008). Methyl donor supplementation prevents transgenerational amplification of obesity. Int. J. Obes. (Lond.) 32, 1373–1379.
Wei, M., Stern, M. P., and Haffner, S. M. (1997). Serum leptin levels in Mexican Americans and non-Hispanic whites: association with body mass index and cigarette smoking. Ann. Epidemiol. 7, 81–86.
Wideroe, M., Vik, T., Jacobsen, G., and Bakketeig, L. S. (2003). Does maternal smoking during pregnancy cause childhood overweight? Paediatr. Perinat. Epidemiol. 17, 422.
Williams, C. M., and Kanagasabai, T. (1984). Maternal adipose tissue response to nicotine administration in the pregnant rat: effects on fetal body fat and cellularity. Br. J. Nutr. 51, 7–13.
World Health Organization (WHO). (2009). Report on the Global Tobacco Epidemic, 2009: Implementing Smoke-Free Environments. Geneva: World Health Organization.
World Health Organization (WHO). (2011a). WHO Report on the Global Tobacco Epidemic, 2011 – Warning About the Dangers of Tobacco. Available at: http://www.who.int/tobacco/global_report/2011/en/ [accessed May, 2012]
World Health Organization (WHO). (2011b). What are Overweight and Obesity? Geneva: World Health Organization.
Xie, B., Palmer, P. H., Pang, Z., Sun, P., Duan, H., and Johnson, C. A. (2010). Environmental tobacco use and indicators of metabolic syndrome in Chinese adults. Nicotine Tob. Res. 12, 198–206.
Yang, S., Wright, J., Bauter, M., Seweryniak, K., Kode, A., and Rahman, I. (2007). Sirtuin regulates cigarette smoke-induced proinflammatory mediator release via RelA/p65 NF-_B in macrophages in vitro and in rat lungs in vivo: implications for chronic inflammation and aging. Am. J. Physiol. Lung Cell Mol. Physiol. 292, L567–L576.
Yeh, J., and Barbieri, R. L. (1989). Twenty-four-hour urinary-free cortisol in premenopausal cigarette smokers and nonsmokers. Fertil. Steril. 52, 1067–1069.
Yilmaz, G., Hizli, S., Karacan, C., Yurdakok, K., Coskun, T., and Dilmen, U. (2009a). Effect of passive smoking on growth and infection rates of breast-fed and non-breast-fed infants. Pediatr. Int. 51, 352–358.
Yilmaz, G., Isik Agras, P., Hizli, S., Karacan, C., Besler, H. T., Yurdakok, K., et al. (2009b). The effect of passive smoking and breast feeding on serum antioxidant vitamin (A, C, E) levels in infants. Acta Paediatr. 98, 531–536.
Keywords: gestation, lactation, programming, tobacco, nicotine, obesity, hormones
Citation: Lisboa PC, de Oliveira E and de Moura EG (2012) Obesity and endocrine dysfunction programmed by maternal smoking in pregnancy and lactation. Front. Physio. 3:437. doi: 10.3389/fphys.2012.00437
Received: 14 July 2012; Accepted: 30 October 2012;
Published online: 19 November 2012.
Edited by:
Catalina Pico, University of the Balearic Islands, SpainReviewed by:
Karen Moritz, The University of Queensland, AustraliaJacqueline K. Phillips, Macquarie University, Australia
Marlon E. Cerf, South African Medical Research Council, South Africa
Copyright: © 2012 Lisboa, de Oliveira and de Moura. This is an open-access article distributed under the terms of the Creative Commons Attribution License, which permits use, distribution and reproduction in other forums, provided the original authors and source are credited and subject to any copyright notices concerning any third-party graphics etc.
*Correspondence: Patricia Cristina Lisboa, Laboratório de Fisiologia Endócrina, Departamento de Ciências Fisiológicas, Instituto de Biologia Roberto Alcantara Gomes, Universidade do Estado do Rio de Janeiro, Av. 28 de setembro, 87 Fundos, PAPC 5°. andar, Rio de Janeiro 20551-030, Brazil. e-mail:cGNsaXNib2FAdWVyai5icg==