- Department of Physiology and Developmental Biology, Brigham Young University, Provo, UT, USA
Chronic obstructive pulmonary disease (COPD) is a progressive condition characterized by chronic airway inflammation and airspace remodeling, leading to airflow limitation that is not completely reversible. Smoking is the leading risk factor for compromised lung function stemming from COPD pathogenesis. First- and second-hand cigarette smoke contain thousands of constituents, including several carcinogens and cytotoxic chemicals that orchestrate chronic lung inflammation and destructive alveolar remodeling. Receptors for advanced glycation end-products (RAGE) are multi-ligand cell surface receptors primarily expressed by diverse lung cells. RAGE expression increases following cigarette smoke exposure and expression is elevated in the lungs of patients with COPD. RAGE is responsible in part for inducing pro-inflammatory signaling pathways that culminate in expression and secretion of several cytokines, chemokines, enzymes, and other mediators. In the current review, new transgenic mouse models that conditionally over-express RAGE in pulmonary epithelium are discussed. When RAGE is over-expressed throughout embryogenesis, apoptosis in the peripheral lung causes severe lung hypoplasia. Interestingly, apoptosis in RAGE transgenic mice occurs via conserved apoptotic pathways also known to function in advanced stages of COPD. RAGE over-expression in the adult lung models features of COPD including pronounced inflammation and loss of parenchymal tissue. Understanding the biological contributions of RAGE during cigarette smoke-induced inflammation may provide critically important insight into the pathology of COPD.
Introduction
Chronic obstructive pulmonary disease (COPD) is defined by airflow obstruction that is not fully reversible (Carp and Janoff, 1978). In particular, COPD involves chronic airway inflammation and pulmonary emphysema, which is defined anatomically via pathology samples as an abnormal permanent enlargement of airspaces distal to the terminal bronchioles accompanied by destruction of their walls without obvious fibrosis (Pauwels et al., 2001). COPD morbidity and mortality continue to rise as physician diagnoses of COPD increased from approximately 7 million in 1980 to approximately 13.1 million in 2004 (Adams and Barnes, 2006). COPD was responsible for 8 million outpatient visits, 1.5 million emergency room visits, and 672,000 hospitalizations in the U.S. in 2006 (US Department of Health and Human Services, 2009) and compared to 1980, deaths in 2007 increased 74% to over 124,000 people (American Lung Association COPD Fact Sheet, 2011). While as recent as 2010 the cost associated with COPD was $49.9 billion (Dalal et al., 2010), the precise pathobiochemical basis of COPD exacerbated by voluntary or involuntary tobacco smoke exposure remains enigmatic.
Cigarette smoking is currently the most considerable risk factor for the development of COPD, consisting of emphysema and chronic obstructive bronchitis (Anderson et al., 1964; Fletcher and Peto, 1977; Thun et al., 2000; Hogg, 2004). Notwithstanding, only one quarter of cigarette smokers develop clinically detectible airflow limitation and other symptoms of COPD, suggesting an important role for genetic susceptibility (Sethi and Rochester, 2000; Stockley et al., 2009). Although most people that develop COPD currently smoke cigarettes or have smoked in the past, COPD also develops in individuals that have never smoked (Higgins, 1991). This harmful outcome is due in part to exposure to second-hand smoke (Janson, 2004; Wakefield et al., 2005; Eisner et al., 2006). Furthermore, because some former smokers still live with active smokers and are observed to develop COPD later in life, passive smoke exposure is likely to contribute to disease progression.
First- and second-hand smokers diagnosed with moderate COPD have altered expression of several genes, including transcription factors, growth factors, and extracellular matrix proteins (Ning et al., 2004). These and other gene products likely function to stimulate the recruitment of inflammatory cells, cytokine secretion, cell death, and elevated protease production observed after prolonged cigarette smoke exposure (Carp and Janoff, 1978; Wright and Churg, 1990; Kuschner et al., 1996; Hautamaki et al., 1997; Sopori, 2002). As such, it is critical to examine how genes influence disease presentation so that precise mechanisms through which passive and active cigarette smoke contribute to COPD/emphysema can be identified.
General Mechanisms of COPD Pathogenesis
Numerous reviews that address COPD pathogenesis, its impact, and plausible therapies have been composed (Bridevaux and Rochat, 2011; Budinger and Mutlu, 2011; Caramori et al., 2011; Lugade et al., 2011; Rooney and Sethi, 2011). The intent of the current work is to concisely provide a foundational summary of conserved COPD modalities and discuss the plausible influence of receptors for advanced glycation end-products (RAGE) signaling. The prevailing pathogenic concept states that COPD is associated with chronic inflammation, imbalances between proteases/antiproteases, oxidative stress, and an elevated apoptotic index. Inflammation arising predominantly from neutrophilic contributions has been proposed due to enhanced neutrophil abundance in bronchoalveolar lavage (BAL) and sputum from COPD patients (Thompson et al., 1989; Stanescu et al., 1996; O'Donnell et al., 2004). Levels of chemoattractants that recruit neutrophils and other potent inflammatory mediators are also elevated in COPD, including leukotriene B4 (Beeh et al., 2003), CXCL2 and 8 (Keatings et al., 1996; Tanino et al., 2002; Beeh et al., 2003), CXCL1 (Keatings et al., 1996), CXCL5 (Tanino et al., 2002), IFN-γ (Hodge et al., 2007), IL-1β (Thacker, 2006; Churg et al., 2009), and TNF-α (Barnes and Karin, 1997). Matrix metalloproteinases (MMPs) produced by macrophages and neutrophils are also misregulated in COPD (Shapiro, 1994). In particular, levels of MMP-1, MMP-2, MMP-7, MMP-9, and MMP-12 are all up-regulated in pulmonary tissue, BAL, and/or sputum of patients with COPD (Shapiro et al., 1993; Hautamaki et al., 1997; Ohnishi et al., 1998; Pratico et al., 1998; Shaykhiev et al., 2009), however because smoke exposed MMP-9 knockout mice are protected from emphysema, MMP-9 may require cooperation with other proteases during adverse lung remodeling (Atkinson et al., 2011) The chemical assessment of tobacco smoke reveals that it contains high levels of reactive oxygen species (ROS) that are in excess of intrinsic antioxidant defense mechanisms (Pauwels et al., 2001; Barnes et al., 2003). Generated in the airways, oxidants lead to cell dysfunction and/or death and also influence inflammatory signaling and protease augmentation via NF-κB-mediated mechanisms (Moodie et al., 2004). During the last decade, enhanced apoptosis stemming from diverse signaling pathways has also been implicated in alveolar septal cell loss observed in COPD patients (Kasahara et al., 2000, 2001; Tuder et al., 2003; Petrache et al., 2006). As a programmed event of removing unwanted cells and debris, apoptosis occurs via extrinsic signaling processes (Degterev et al., 2003), and intrinsic mitochondria or endoplasmic reticulum-mediated processes (Darmon et al., 1995; Slee et al., 1999). In summary, COPD is characterized by progressive destruction of the distal lung and small airway obstruction resulting from chronic inflammation and elevated cell death.
Constituents of Tobacco Smoke
Tobacco smoke is a toxic and carcinogenic mixture of more than 5000 chemicals (Talhout et al., 2011). Of these, around 400 have been quantified, at least 200 are toxic to humans and/or experimental animals, and over 50 have been identified as known, probable, or possible human carcinogens (Kirsti, 2004). Studies indicate that compared with mainstream smoke collected under standard FTC/ISO smoking parameters, sidestream smoke has higher levels of PAHs (Grimmer et al., 1987; Evans et al., 1993), nitrosamines (Brunnemann et al., 1977, 1980; Hoffmann et al., 1979a; Ruhl et al., 1980), aza-arenes (Dong et al., 1978; Grimmer et al., 1987), aromatic amines (Patrianakos and Hoffmann, 1979), carbon monoxide (Hoffmann et al., 1979b; Rickert et al., 1984), nicotine (Rickert et al., 1984; Pakhale and Maru, 1998), ammonia (Brunnemann and Hoffmann, 1975), pyridine (Johnson et al., 1973; Brunnemann and Hoffmann, 1978), and the gas phase components 1,3-butadiene, acrolein, isoprene, benzene, and toluene (Brunnemann et al., 1990). In addition to these deleterious compounds, other factors such as the type of tobacco, the chemicals added to the tobacco, the way the tobacco product is smoked, and, for cigarettes and cigars, the material in which the tobacco is wrapped can also affect second-hand smoke chemical composition (International Agency for Research on Cancer, 2002; National Toxicology Program, 2005; US Department of Health and Human Services, 2006).
Cigarette smoke is also an important exogenous source of reactive glycation products capable of promoting formation of AGEs, advanced glycation end-products, which are irreversibly glycated proteins that efficiently bind RAGE (Cerami et al., 1997). Studies have shown that both aqueous extracts of tobacco and cigarette smoke contain glycotoxins, highly reactive glycation products that can rapidly induce AGE formation on proteins in vitro and in vivo (Nicholl and Bucala, 1998; Nicholl et al., 1998). These activities can be eliminated by passing the samples through a dry packed column of aminoguanidine, a potent and specific inhibitor of AGE formation. Additional studies have shown that serum AGEs and apolipoprotein B-linked AGE levels are significantly elevated in cigarette smokers relative to non-smokers and AGEs or immunochemically related molecules are present at higher levels in the tissues of smokers compared to non-smokers, regardless of the presence of diabetes (Nicholl et al., 1998).
Receptor for Advanced Glycation End-Products
RAGE are cell-surface receptors of the immunoglobulin superfamily expressed in many cell types including endothelial and vascular smooth muscle cells, fibroblasts, macrophages/monocytes, and epithelium (Brett et al., 1993). RAGE expression is most abundant in the lung, from which it was initially isolated, and is selectively localized to well-differentiated alveolar type I (ATI) epithelial cells (Schmidt, 2001). Identification in respiratory epithelium (Dahlin et al., 2004; Koslowski et al., 2004) and studies that document RAGE-mediated adherence to collagen IV (Demling et al., 2006) have led to the implication of RAGE in important developmental processes such as the spreading, thinning, and adherence that characterize the transitioning of ATII cells to squamous ATI cells. RAGE was first described as a progression factor in cellular responses induced by AGEs that accumulate in hyperglycemia and oxidant stress. Subsequent studies have distinguished RAGE as a pattern recognition receptor that also binds S100/calgranulins, amyloid-β-peptide, and HMGB-1 (or amphoterin), to influence gene expression via divergent signal transduction pathways (Reddy et al., 2006; Hudson et al., 2008; Kim et al., 2008; Toure et al., 2008). Because RAGE expression can also increase when ligands accumulate (Schmidt, 2001), RAGE-ligand interaction may contribute to chronic pathological states where ligands are common including diabetic complications, neurodegenerative disorders, atherosclerosis, and inflammation (Hofmann et al., 1999; Taguchi et al., 2000). Specifically, a host of pro-inflammatory responses such as those coordinated by MAP kinases (ERK, JNK, and p38), NF-κB, ROS, and other pro-inflammatory mediators such as TNF and IL-1 (Bianchi et al., 2010) result from RAGE-ligand interactions (Figure 1). In contrast to short-lived cellular activation mediated by LPS, engagement of RAGE by its ligands results in prolonged inflammation (Lin et al., 2009). If left unchecked, such chronic inflammation results in severe tissue injury.
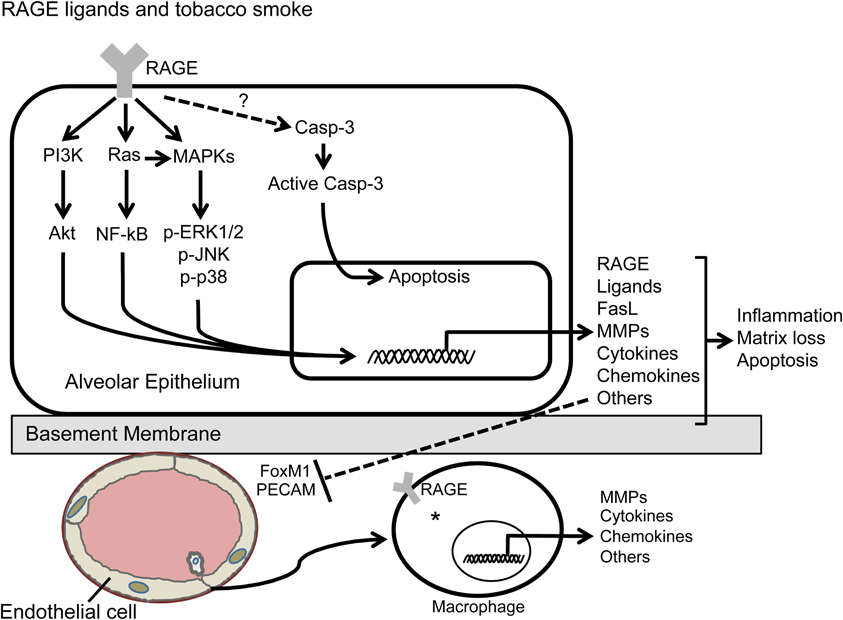
Figure 1. Deleterious effects characteristic of COPD are elicited via several pro-inflammatory signaling pathways observed in RAGE-expressing alveolar epithelial cells and resident alveolar macrophages (*). Direct stimulation of RAGE by tobacco smoke, de novo AGE generation in a tobacco smoke environment, or genetic up-regulation of RAGE in the lungs of conditional bi-transgenic mice results in characteristics of COPD including inflammation, matrix destabilization, and apoptosis.
The full length membrane bound form of RAGE (mRAGE) contains an extracellular variable V-region-like immunoglobulin domain crucial for ligand binding and two constant C-region-like immunoglobulin domains, a single-pass hydrophobic transmembrane domain and a short, 43 amino acid, highly charged cytoplasmic domain essential for intracellular signaling (Buckley and Ehrhardt, 2010). The cytoplasmic domain of RAGE contains four possible phosphorylation sites, S391, S399, S400, and T401, of which only S391 is conserved among humans, mice, guinea pigs, rats, rabbits, dogs, and cats (Sakaguchi et al., 2011). Replacement of S391 to alanine was sufficient to abrogate PKCζ-dependent phosphorylation and subsequent signal transduction in vitro (Sakaguchi et al., 2011). Although not explicitly stated, RAGE behaves similarly to a receptor tyrosine kinase (RTK) cell surface receptor, requiring homodimerization to effectively potentiate intracellular signaling cascades (Zong et al., 2010). Distinct alternative isoforms also exist for the receptor due to differential splicing variants of the RAGE message. Dominant negative RAGE (dn-RAGE) is a membrane anchored splice variant of RAGE capable of ligand binding but lacking the intracellular domain necessary for signal transduction. Endogenous secreted RAGE (esRAGE) is generated via alternative splicing at exon 9 yielding the same V and C-regions of the full length-RAGE but lacks both the hydrophobic transmembrane and the intracellular domains (Buckley and Ehrhardt, 2010). Additionally, full-length RAGE can be cleaved by MMPs to render sRAGE, a non-splice variant of RAGE closely resembling esRAGE in structure and function (Yamakawa et al., 2011). These altered variants of RAGE incapable of transducing signals are thought to function as decoy receptors that prevent the interaction of mRAGE with its ligands.
The pro-inflammatory role of RAGE in cardiovascular diseases is well documented (Yan et al., 2009). Furthermore, several studies strongly suggest that RAGE signaling is a key regulator of inflammation in major pulmonary diseases. A study demonstrated that abrogation of RAGE signaling (using RAGE null mice) attenuated pulmonary ischemia and reperfusion injury associated with decreased NF-κB activation and IL-8 production (Sternberg et al., 2008). Another important role for RAGE signaling in lung disease shows that RAGE-deficient mice under hyperoxic conditions survived longer than wild type controls and the mice had less airway cellularity and diminished alveolar damage compared to wild type controls (Reynolds et al., 2010). RAGE has been implicated in the fibrotic process in a number of tissues, including the peritoneum, kidney, and liver (Li et al., 2004; De Vriese et al., 2006; Xia et al., 2008), where it has been shown to promote fibrosis. In the lung, evidence continues to accumulate suggesting an important role for RAGE in pulmonary fibrosis, yet conflicting data portray RAGE as having both protective and destabilizing functions. Acute lung injury (ALI) and a more severe condition known as acute respiratory distress syndrome (ARDS) are characterized by deterioration of the alveolar-capillary barrier and impaired alveolar fluid clearance (Lucas et al., 2009). ALI and ARDS are associated with damage to ATI cells, a population of cells with significant RAGE expression, and several different animal models of ALI express increased RAGE levels in BALF (Uchida et al., 2006; Su et al., 2007, 2009; Zhang et al., 2008). A published study from our laboratory considered the effects of smoke exposure on RAGE expression both in lung cells and mice (Reynolds et al., 2008). The research revealed that RAGE and its ligands were up-regulated in lung epithelial cells cultured with cigarette smoke extract (CSE) and that mice exposed to cigarette smoke for 6 months had elevated RAGE expression in pulmonary epithelium (Reynolds et al., 2008). While the full extent of RAGE function in smoke-induced COPD has not been sufficiently examined, these studies demonstrate that RAGE may play a role in COPD pathogenesis.
Contributions of Rage to COPD Progression
RAGE and two of its ligands S100A12 and HMGB-1 were up-regulated in a rat alveolar type I-like cell line (R3/1), a human alveolar type 1I-like epithelial cell line (A549), and a macrophage-like murine cell line (RAW 264.7) following exposure to CSE (Reynolds et al., 2008). S100A12 is a calcium-binding pro-inflammatory modulator and HMGB-1 is a non-histone nuclear protein that acts as a potent pro-inflammatory mediator when secreted. In human lungs with smoke-related lesions, widespread RAGE expression has been documented in bronchiolar epithelia, small respiratory airways, reactive ATI cells, and alveolar macrophages (AMs; Morbini et al., 2006). The same study identified elevated S100A12 in polymorphonuclear granulocytes and in extracellular fluid and the number and intensity of carboxymethyl-lysine positive cells (cells that stain for AGEs) were measurably enhanced in epithelial and inflammatory cells of the lungs of smokers (Morbini et al., 2006).
Another factor highly expressed in the lungs of smokers with COPD is early growth response gene 1 (Egr-1), a zinc finger-containing, hypoxia-inducible transcription factor (Ning et al., 2004). Egr-1 expression significantly increased in lung cell lines following CSE exposure in vitro and it activated the RAGE promoter (Reynolds et al., 2006, 2008). Because the RAGE gene also contains NF-κB and SP-1 promoter response elements (Li and Schmidt, 1997) and is transcriptionally regulated by cis-acting Egr-1 (Reynolds et al., 2006), a possible auto-inflammatory loop may be triggered suggesting cooperation between Egr-1 and RAGE in chronic smoke-related inflammatory disease states. More recently, it was discovered that Ras, a small GTPase that functions as a molecular switch in the control of diverse signaling cascades, was induced in R3/1 cells following exposure to CSE, resulting in up-regulation of NF-κB-mediated secretion of TNF-α, IL-1β, and IL-8 (Figure 1; Reynolds et al., 2011a).
Our lab has recently expanded research into the biology of smoke-exposed primary mouse AMs also known to express RAGE. Studies document that low levels of RAGE are expressed by mouse primary macrophages during normal conditions and that RAGE overexpression by these primary macrophages is associated with inflammation and the coordination of lung damage (Morbini et al., 2006). Our studies indicate that acute exposure of mice to CSE via nasal instillation resulted in diminished BAL cellularity and fewer AMs in RAGE null mice compared to controls. Additionally, AMs isolated from wild type mice exposed to CSE significantly increased RAGE expression (Robinson et al., 2012). This recently published work also demonstrated for the first time that RAGE null AMs exposed to CSE experienced reduced Ras and p38 MAPK activation, less NF-κB translocation, and diminished expression of TNF-α and IL-1β when compared to CSE exposed wild type AMs (Figure 1). Evidence suggests that primary AMs coordinate CSE-induced inflammation, at least in part, via RAGE-mediated mechanisms and that cooperation with alveolar epithelium in coordinated inflammatory responses is likely.
Use of Rage Transgenic Mice in Modeling Characteristics of COPD
Several animal models that seek to recapitulate various aspects of COPD have been presented within the past decade. These models include mouse IL-1β over-expressers (Lappalainen et al., 2005), rat VEGF signaling nulls (VEGF or VEGFR2 blockers: Kasahara et al., 2000), intratracheal administration of active caspase-3 (Aoshiba et al., 2003) and several others that aim to elucidate inflammatory and other destructive mechanisms during smoke-less and smoke-exposed disease progression (Petrache et al., 2005; Giordano et al., 2008; Kang et al., 2012). The vast majority of these models present emphysema-like anatomical characteristics and inflammatory indexes in the presence of room-air and notable exacerbation in the presence of cigarette smoke. Although RAGE has been shown to be a marker for many inflammatory diseases including COPD, a genetic mouse model for COPD had not been previously examined.
We generated a bi-transgenic in vivo mouse model that utilizes two transgenes to conditionally up-regulate RAGE (Figure 2). One transgenic mouse line employs surfactant protein C (SP-C) to drive expression of rtTA (reverse tetracycline transactivator) and another transgenic line contains binding sites for a complex between rtTA and doxycycline (dox; Reynolds et al., 2011b). Although COPD is an adult lung disease, we initially sought to characterize RAGE bi-transgenic mice during development with the realization that aspects of COPD may be detected during organogenesis. Our model was thought to compliment research that centers on bronchopulmonary dysplasia (BPD), an embryonic disease highly correlated with emphysema in terms of oxidative stress, pulmonary inflammation, increased apoptosis, protease/antiprotease imbalance and altered microvasculature (Hargitai et al., 2001; Danan et al., 2002; Saugstad, 2003; Ekekezie et al., 2004; Speer, 2006). While COPD is characterized by sustained inflammation and alveolar destruction, remarkably similar mechanisms are implicated in the altered branching and impaired alveolarization observed in BPD (Bourbon et al., 2009).
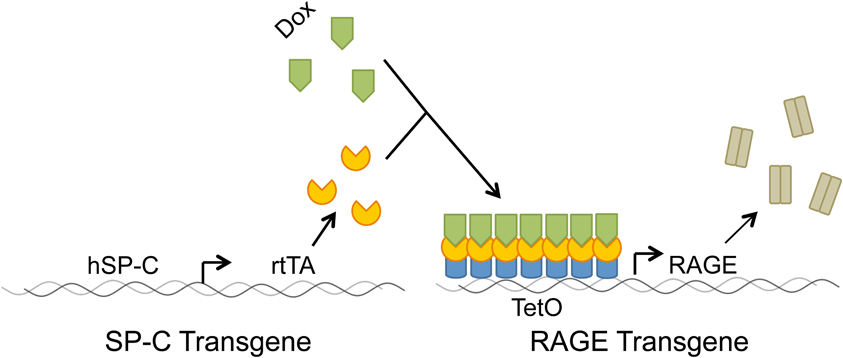
Figure 2. Full length RAGE was over-expressed in alveolar type (AT) II cells by obtaining progeny from two transgenic lines of mice. The reverse tetracycline transactivator (rtTA) was produced under the control of the human surfactant protein C (hSP-C) promoter in distal respiratory epithelium. Following the administration of doxycycline (dox), a dox-rtTA complex activates the TetO promoter, thereby expressing RAGE.
Embryonic Rage Bi-Transgenic Mice have Perturbed Distal Epithelium
Complete perinatal lethality was observed when dox was supplied to RAGE bi-transgenic mice throughout embryogenesis. At embryonic day (E) 18.5, pulmonary tissues were severely hypoplastic and only minimal respiratory surface area near the visceral pleura remained. Several immunohistochemical and flow cytometric experiments demonstrated diminished abundance of differentiated distal lung cell types, most notably ATI and ATII cells (Reynolds et al., 2011b).
Altered cellular differentiation has not sufficiently been characterized in the distal lung of COPD patients; however, new research has emerged demonstrating that human ciliated cells can respond to cigarette smoke by promoting GDF15, a factor capable of driving Muc5A expression in goblet cells (Wu et al., 2011). RAGE and RAGE ligands have been implicated in altered cellular differentiation of several cell types including smooth muscle cells, skeletal myocytes and developing neural tissue (Suga et al., 2011; Kim et al., 2012; Riuzzi et al., 2012). Thyroid transcription factor 1 (TTF-1; also known as Nkx2.1) is a key regulator of pulmonary development and present in distal lung epithelium that can negatively regulate RAGE expression (Reynolds et al., 2008) and SP-1 positively regulates the active promoter region of TTF-1 in surfactant producing cells (Das et al., 2011). Because NF-κB (a crucial intermediate of RAGE signaling) can interfere with SP-1 binding (Benjamin et al., 2010), RAGE may play a role in inhibited surfactant synthesis observed when ATII cells are abnormally regulated.
Embryonic Rage Bi-Transgenic Mice have Abnormal Distal Pulmonary Endothelial Cell Growth
In addition to the decreased cellularity of the lungs, RAGE over-production disturbed capillary growth and maintenance through the inhibition of FoxM1 (a critical transcription factor necessary for endothelial expansion) and PECAM (a marker for endothelial cells) expression (Geyer et al., 2011). Endothelial cell apoptosis has been observed in COPD patients using TUNEL, immunohistochemistry and DNA ligation techniques that coincided with the reduction of endothelial markers including VEGF and VEGFR2 (Kasahara et al., 2001). Additionally Dinh-Xuan et al. and Peinado et al. both showed that resected lung samples from COPD patients had extensive endothelial dysfunction, which they proposed to contribute to hypertension (Dinh-Xuan et al., 1991; Peinado et al., 1998). It is hypothesized that vascular tone in the lung can be regulated by direct stimulation of the vascular compartment by cigarette smoke and indirect stimulation stemming from smoke-exposed epithelial cells. Our discoveries relating to pulmonary endothelium in the RAGE bi-transgenic mouse correlate with numerous studies that demonstrate RAGE signaling in cases of depressed endothelial function and increased barrier disruption (Sun et al., 2009; Pollreisz et al., 2010; Wolfson et al., 2011; Chen et al., 2012; Huang et al., 2012).
Embryonic Rage Bi-Transgenic Mice have Extracellular Matrix Abnormalities
We also demonstrated that MMP-9 secretion is increased, coincident with diminished collagen IV (a principle component of the alveolar basement membrane) deposition and production (Bukey et al., 2011). COPD is characterized by an increase in several MMPs including MMP-1, 2, 9, and 12 (Ohnishi et al., 1998; Geraghty et al., 2011). Other research groups have also demonstrated AGE-RAGE dependent mechanisms in MMP-9 production (Ishibashi et al., 2010; Zhang et al., 2010; Zhu et al., 2012). While not yet evaluated in our embryonic RAGE bi-transgenic mouse model, MMPs 1 and 2 have been implicated as RAGE targets (Kamioka et al., 2011; Du et al., 2012; Yu et al., 2012). Interestingly, MMP-1 has been shown to be up-regulated not only in the lungs of COPD patients but in osteoarthritis as well, a chronic inflammatory disease affecting articular cartilage (Steenvoorden et al., 2006). Ongoing research seeks to test hypotheses related to matrix-targeting protease imbalances such as those that involve α1-antitrypsin.
Embryonic Rage Bi-Transgenic Mice have Elevated Parenchymal Cell Apoptosis
Thorough evaluations of apoptosis were performed in order to ascertain causes for the hypoplastic lung phenotype in the embryonic RAGE bi-transgenic mouse. RAGE over-expressing lungs detrimentally declined during the canalicular phase, a period identified by terminal bronchiole branching, initial alveolarization, and microvascular organization. The abrupt loss of tissue was observed in tandem with a significant increase in pro-apoptotic Fas ligand (FasL), a decrease in the anti-apoptotic factor Bcl-2, elevated cleaved active caspase-3 (a critical mediator of cell death), and quantifiable apoptosis by TUNEL assessment (Stogsdill et al., 2012). Electron microscopy also confirmed apoptosis via the detection of numerous bleb-like structures within cells that were physically separated from the underlying basement membrane. Importantly, cellular proliferation was not changed, suggesting there was no feedback mechanism to compensate for elevated cell death. Evidence is mounting that demonstrates active apoptosis of epithelial and endothelial cells in human COPD patients (Segura-Valdez et al., 2000; Kasahara et al., 2001; Majo et al., 2001; Yokohori et al., 2004; Hodge et al., 2005; Imai et al., 2005). Lending support for FasL-mediated apoptosis observed in RAGE bi-transgenic mice was research by Mahali et al. that demonstrated FasL elaboration is a direct product of AGE-RAGE ligation (Mahali et al., 2011). Furthermore, RAGE and its ligands have been shown to promote apoptosis in other tissue types, including myocytes (Tsoporis et al., 2010), endothelial cells (Chen et al., 2010), neuronal cells (Kim et al., 2011), epithelial cells (Jin et al., 2011), and pancreatic β-cells (Lee et al., 2010). Our studies have shown for the first time that increased expression of RAGE using transgenic mouse technology directly activates apoptosis in lung parenchyma. In fact, sustained RAGE expression during development is capable of modeling disorders characterized by cell loss including BPD. Furthermore, these data reveal important RAGE-mediated mechanisms that control cell quantity possibly introduced at the initiation of smoke-induced COPD pathogenesis.
Adult Rage Over-Expression Yields an Emphysematous Lung
Conditional up-regulation of RAGE for 2 to 3 months in the adult bi-transgenic mouse lung lead to incremental dilation of alveolar spaces, assessed by standard H&E staining (Stogsdill et al., 2011). Quantification of the mean chord length of the airspace revealed progressive dilation of alveolar spaces as RAGE over-expression persisted (unpublished data). The adult RAGE bi-transgenic mice had increased MMP-9 and decreased elastin expression consistent with other COPD models. Furthermore, RAGE bi-transgenic mice manifested significant inflammation measured by elevated BALF protein, leukocyte infiltration, and secreted cytokines (MIP-2, IFN-γ; Stogsdill et al., 2011). These data support the concept that innovative transgenic mice that over-express RAGE may model pulmonary inflammation and alveolar destabilization independent of tobacco smoke. Furthermore, it validates RAGE signaling as a target pathway in the prevention or attenuation of smoke-related inflammatory lung diseases.
Conclusions
Despite the progression in the field of RAGE biology in the context of lung disease, the full extent of RAGE localization, the molecular mechanisms that control its expression and its downstream effects should remain topics of focused investigation. While a great deal is known about COPD, relatively little is known about factors that perpetuate inflammation or modalities that sustain them. Our research has shown that mechanisms of COPD progression including chronic inflammation, imbalances involving proteases, oxidative stress, and elevated apoptosis may be mediated by RAGE. Several endogenous (S100/calgranulins, HMGB-1, AGEs) and exogenous ligands (cigarette smoke) may be responsible for the sustained activation of RAGE leading to disease progression (Figure 1). As such, it remains possible that targeting RAGE may, at least in part, provide successful opportunities in the therapeutic alleviation of debilitating inflammatory lung disease exacerbated by tobacco smoke.
Conflict of Interest Statement
The authors declare that the research was conducted in the absence of any commercial or financial relationships that could be construed as a potential conflict of interest.
Acknowledgments
The authors greatly appreciate the advice and support of fellow undergraduates in the Reynolds Lab. This work was supported by a grant from the Flight Attendant's Medical Research Institute (Paul R. Reynolds) and a Brigham Young University Mentoring Environment grant (Paul R. Reynolds).
References
Adams, P. F., and Barnes, P. M. (2006). Summary health statistics for the U.S. population: National Health Interview Survey, 2004. Vital Health Stat. 10, 1–104.
American Lung Association COPD Fact Sheet. (2011). Available online at: http://www.lungusa.org/lung-disease/copd/resources/facts-figures/COPD-Fact-Sheet.html (Accessed February 19, 2011).
Anderson, A. E. Jr., Hernandez, J. A., Eckert, P., and Foraker, A. G. (1964). Emphysema in lung macrosections correlated with smoking habits. Science 144, 1025–1026.
Aoshiba, K., Yokohori, N., and Nagai, A. (2003). Alveolar wall apoptosis causes lung destruction and emphysematous changes. Am. J. Respir. Cell Mol. Biol. 28, 555–562.
Atkinson, J. J., Lutey, B. A., Suzuki, Y., Toennies, H. M., Kelley, D. G., Kobayashi, D. K., Ijem, W. G., Deslee, G., Moore, C. H., Jacobs, M. E., Conradi, S. H., Gierada, D. S., Pierce, R. A., Betsuyaku, T., and Senior, R. M. (2011). The role of matrix metalloproteinase-9 in cigarette smoke-induced emphysema. Am. J. Respir. Crit. Care Med. 183, 876–884.
Barnes, P. J., and Karin, M. (1997). Nuclear factor-kappaB: a pivotal transcription factor in chronic inflammatory diseases. N. Engl. J. Med. 336, 1066–1071.
Barnes, P. J., Shapiro, S. D., and Pauwels, R. A. (2003). Chronic obstructive pulmonary disease: molecular and cellular mechanisms. Eur. Respir. J. 22, 672–688.
Beeh, K. M., Kornmann, O., Buhl, R., Culpitt, S. V., Giembycz, M. A., and Barnes, P. J. (2003). Neutrophil chemotactic activity of sputum from patients with COPD: role of interleukin 8 and leukotriene B4. Chest 123, 1240–1247.
Benjamin, J. T., Carver, B. J., Plosa, E. J., Yamamoto, Y., Miller, J. D., Liu, J. H., Van Der Meer, R., Blackwell, T. S., and Prince, L. S. (2010). NF-kappaB activation limits airway branching through inhibition of Sp1-mediated fibroblast growth factor-10 expression. J. Immunol. 185, 4896–4903.
Bianchi, R., Giambanco, I., and Donato, R. (2010). S100B/RAGE-dependent activation of microglia via NF-κB and AP-1, Co-regulation of COX-2 expression by S100B, IL-1β and TNF-α. Neurobiol. Aging 31, 665–677.
Bourbon, J. R., Boucherat, O., Boczkowski, J., Crestani, B., and Delacourt, C. (2009). Bronchopulmonary dysplasia and emphysema: in search of common therapeutic targets. Trends Mol. Med. 15, 169–179.
Brett, J., Schmidt, A. M., Yan, S. D., Zou, Y. S., Weidman, E., Pinsky, D., Nowygrod, R., Neeper, M., Przysiecki, C., Shaw, A., Migheli, A., and Stern, D. (1993). Survey of the distribution of a newly characterized receptor for advanced glycation end products in tissues. Am. J. Pathol. 143, 1699–1712.
Bridevaux, P. O., and Rochat, T. (2011). COPD 2011, are there other risk factors than tobacco? Rev. Med. Suisse 7, 2232–2235.
Brunnemann, K. D., Fink, W., and Moser, F. (1980). Analysis of volatile N-nitrosamines in mainstream and sidestream smoke from cigarettes by GLC-TEA. Oncology 37, 217–222.
Brunnemann, K. D., and Hoffmann, D. (1975). Chemical studies on tobacco smoke XXXIV. Gas chromatographic determination of ammonia in cigarette and cigar smoke. J. Chromatogr. Sci. 13, 159–163.
Brunnemann, K. D., and Hoffmann, D. (1978). Chemical studies on tobacco smoke LIX. Analysis of volatile nitrosamines in tobacco smoke and polluted indoor environments. IARC Sci. Publ. 19, 343–356.
Brunnemann, K. D., Kagan, M. R., Cox, J. E., and Hoffmann, D. (1990). Analysis of 1, 3-butadiene and other selected gas-phase components in cigarette mainstream and sidestream smoke by gas chromatography-mass selective detection. Carcinogenesis 11, 1863–1868.
Brunnemann, K. D., Yu, L., and Hoffmann, D. (1977). Assessment of carcinogenic volatile N-nitrosamines in tobacco and in mainstream and sidestream smoke from cigarettes. Cancer Res. 37, 3218–3222.
Buckley, S. T., and Ehrhardt, C. (2010). The receptor for advanced glycation end products (RAGE) and the lung. J. Biomed. Biotechnol. 2010, 917108.
Budinger, G. R., and Mutlu, G. M. (2011). Update in environmental and occupational medicine 2010. Am. J. Respir. Crit. Care Med. 183, 1614–1619.
Bukey, B. R., Porter, J. L., Hancock, J. M., Stogsdill, J. A., and Reynolds, P. R. (2011). Increased MMP-9 activity in mice that over-express RAGE in alveolar epithelium destabilizes the basement membrane by degrading collagen type IV. Dev. Biol. 356, 143–144.
Caramori, G., Casolari, P., Cavallesco, G. N., Giuffre, S., Adcock, I., and Papi, A. (2011). Mechanisms involved in lung cancer development in COPD. Int. J. Biochem. Cell Biol. 43, 1030–1044.
Carp, H., and Janoff, A. (1978). Possible mechanisms of emphysema in smokers. In vitro suppression of serum elastase-inhibitory capacity by fresh cigarette smoke and its prevention by antioxidants. Am. Rev. Respir. Dis. 118, 617–621.
Cerami, C., Founds, H., Nicholl, I., Mitsuhashi, T., Giordano, D., Vanpatten, S., Lee, A., Alabed, Y., Vlassara, H., Bucala, R., and Cerami, A. (1997). Tobacco smoke is a source of toxic reactive glycation products. Proc. Natl. Acad. Sci. U.S.A. 94, 13915–13920.
Chen, J., Jin, J., Song, M., Dong, H., Zhao, G., and Huang, L. (2012). C-reactive protein down-regulates endothelial nitric oxide synthase expression and promotes apoptosis in endothelial progenitor cells through receptor for advanced glycation end-products. Gene 496, 128–135.
Chen, J., Song, M., Yu, S., Gao, P., Yu, Y., Wang, H., and Huang, L. (2010). Advanced glycation endproducts alter functions and promote apoptosis in endothelial progenitor cells through receptor for advanced glycation endproducts mediate overpression of cell oxidant stress. Mol. Cell. Biochem. 335, 137–146.
Churg, A., Zhou, S., Wang, X., Wang, R., and Wright, J. L. (2009). The role of interleukin-1B in murine cigarette smoke-induced emphysema and small airway remodeling. Am. J. Respir. Cell Mol. Biol. 40, 482–490.
Dahlin, K., Mager, E. M., Allen, L., Tigue, Z., Goodglick, L., Wadehra, M., and Dobbs, L. (2004). Identification of genes differentially expressed in rat alveolar type I cells. Am. J. Respir. Cell Mol. Biol. 31, 309–316.
Dalal, A. A., Christensen, L., Liu, F., and Riedel, A. A. (2010). Direct costs of chronic obstructive pulmonary disease among managed care patients. Int. J. Chron. Obstruct. Pulmon. Dis. 5, 341–349.
Danan, C., Jarreau, P. H., Franco, M. L., Dassieu, G., Grillon, C., Abd Alsamad, I., Lafuma, C., Harf, A., and Delacourt, C. (2002). Gelatinase activities in the airways of premature infants and development of bronchopulmonary dysplasia. Am. J. Physiol. Lung Cell. Mol. Physiol. 283, L1086–L1093.
Darmon, A. J., Nicholson, D. W., and Bleackley, R. C. (1995). Activation of the apoptotic protease CPP32 by cytotoxic T-cell-derived granzyme B. Nature 377, 446–448.
Das, A., Acharya, S., Gottipati, K. R., McKnight, J. B., Chandru, H., Alcorn, J. L., and Boggaram, V. (2011). Thyroid transcription factor-1 (TTF-1) gene: identification of ZBP-89, Sp1, and TTF-1 sites in the promoter and regulation by TNF-alpha in lung epithelial cells. Am. J. Physiol. Lung Cell. Mol. Physiol. 301, L427–L440.
Demling, N., Ehrhardt, C., Kasper, M., Laue, M., Knels, L., and Rieber, E. P. (2006). Promotion of cell adherence and spreading: a novel function of RAGE, the highly selective differentiation marker of human alveolar epithelial type I cells. Cell Tissue Res. 323, 475–488.
De Vriese, A. S., Tilton, R. G., Mortier, S., and Lameire, N. H. (2006). Myofibroblast transdifferentiation of mesothelial cells is mediated by RAGE and contributes to peritoneal fibrosis in uraemia. Nephrol. Dial. Transplant. 21, 2549–2555.
Dinh-Xuan, A. T., Higenbottam, T. W., Clelland, C. A., Pepke-Zaba, J., Cremona, G., Butt, A. Y., Large, S. R., Wells, F. C., and Wallwork, J. (1991). Impairment of endothelium-dependent pulmonary-artery relaxation in chronic obstructive lung disease. N. Engl. J. Med. 324, 1539–1547.
Dong, M., Schmeltz, I., Jacobs, E., and Hoffmann, D. (1978). Aza-Arenes in tobacco smoke. J. Anal. Toxicol. 2, 21–25.
Du, H., Li, P., Wang, J., Qing, X., and Li, W. (2012). The interaction of amyloid beta and the receptor for advanced glycation endproducts induces matrix metalloproteinase-2 expression in brain endothelial cells. Cell. Mol. Neurobiol. 32, 141–147.
Eisner, M. D., Balmes, J., Yelin, E. H., Katz, P. P., Hammond, S. K., Benowitz, N., and Blanc, P. D. (2006). Directly measured secondhand smoke exposure and COPD health outcomes. BMC Pulm. Med. 6, 12.
Ekekezie, I. I., Thibeault, D. W., Simon, S. D., Norberg, M., Merrill, J. D., Ballard, R. A., Ballard, P. L., and Truog, W. E. (2004). Low levels of tissue inhibitors of metalloproteinases with a high matrix metalloproteinase-9/tissue inhibitor of metalloproteinase-1 ratio are present in tracheal aspirate fluids of infants who develop chronic lung disease. Pediatrics 113, 1709–1714.
Evans, W. H., Thomas, N. C., Boardman, M. C., and Nash, S. J. (1993). Relationships of polycyclic aromatic hydrocarbon yields with particulate matter (water and nicotine free) yields in mainstream and sidestream cigarette smoke. Sci. Total Environ. 136, 101–109.
Fletcher, C., and Peto, R. (1977). The natural history of chronic airflow obstruction. Br. Med. J. 1, 1645–1648.
Geraghty, P., Dabo, A. J., and D'Armiento, J. (2011). TLR4 protein contributes to cigarette smoke-induced matrix metalloproteinase-1 (MMP-1) expression in chronic obstructive pulmonary disease. J. Biol. Chem. 286, 30211–30218.
Geyer, A. J., Ferguson, N. T., and Reynolds, P. R. (2011). Conditional embryonic over-expression of RAGE in the mouse lung diminishes pulmonary endothelium expression. Dev. Biol. 356, 143–143.
Giordano, R. J., Lahdenranta, J., Zhen, L., Chukwueke, U., Petrache, I., Langley, R. R., Fidler, I. J., Pasqualini, R., Tuder, R. M., and Arap, W. (2008). Targeted induction of lung endothelial cell apoptosis causes emphysema-like changes in the mouse. J. Biol. Chem. 283, 29447–29460.
Grimmer, G., Naujack, K. W., and Dettbarn, G. (1987). Gaschromatographic determination of polycyclic aromatic hydrocarbons, aza-arenes, aromatic amines in the particle and vapor phase of mainstream and sidestream smoke of cigarettes. Toxicol. Lett. 35, 117–124.
Hargitai, B., Szabo, V., Hajdu, J., Harmath, A., Pataki, M., Farid, P., Papp, Z., and Szende, B. (2001). Apoptosis in various organs of preterm infants: histopathologic study of lung, kidney, liver, and brain of ventilated infants. Pediatr. Res. 50, 110–114.
Hautamaki, R. D., Kobayashi, D. K., Senior, R. M., and Shapiro, S. D. (1997). Requirement for macrophage elastase for cigarette smoke-induced emphysema in mice. Science 277, 2002–2004.
Higgins, M. (1991). Risk factors associated with chronic obstructive lung disease. Ann. N.Y. Acad. Sci. 624, 7–17.
Hodge, G., Nairn, J., Holmes, M., Reynolds, P. N., and Hodge, S. (2007). Increased intracellular T helper 1 proinflammatory cytokine production in peripheral blood, bronchoalveolar lavage and intraepithelial T cells of COPD subjects. Clin. Exp. Immunol. 150, 22–29.
Hodge, S., Hodge, G., Holmes, M., and Reynolds, P. N. (2005). Increased airway epithelial and T-cell apoptosis in COPD remains despite smoking cessation. Eur. Respir. J. 25, 447–454.
Hoffmann, D., Adams, J. D., Brunnemann, K. D., and Hecht, S. S. (1979a). Assessment of tobacco-specific N-nitrosamines in tobacco products. Cancer Res. 39, 2505–2509.
Hoffmann, D., Adams, J. D., and Wynder, E. L. (1979b). Formation and analysis of carbon monoxide in cigarette mainstream and sidestream smoke. Prev. Med. 8, 344–350.
Hofmann, M. A., Drury, S., Fu, C., Qu, W., Taguchi, A., Lu, Y., Avila, C., Kambham, N., Bierhaus, A., Nawroth, P., Neurath, M. F., Slattery, T., Beach, D., McClary, J., Nagashima, M., Morser, J., Stern, D., and Schmidt, A. M. (1999). RAGE mediates a novel proinflammatory axis: a central cell surface receptor for S100/calgranulin polypeptides. Cell 97, 889–901.
Hogg, J. C. (2004). Pathophysiology of airflow limitation in chronic obstructive pulmonary disease. Lancet 364, 709–721.
Huang, W., Liu, Y., Li, L., Zhang, R., Liu, W., Wu, J., Mao, E., and Tang, Y. (2012). HMGB1 increases permeability of the endothelial cell monolayer via RAGE and Src family tyrosine kinase pathways. Inflammation 35, 350–362.
Hudson, B. I., Kalea, A. Z., Del Mar Arriero, M., Harja, E., Boulanger, E., D'Agati, V., and Schmidt, A. M. (2008). Interaction of the RAGE cytoplasmic domain with diaphanous-1 is required for ligand-stimulated cellular migration through activation of Rac1 and Cdc42. J. Biol. Chem. 283, 34457–34468.
Imai, K., Mercer, B. A., Schulman, L. L., Sonett, J. R., and D'Armiento, J. M. (2005). Correlation of lung surface area to apoptosis and proliferation in human emphysema. Eur. Respir. J. 25, 250–258.
International Agency for Research on Cancer. (2002). Tobacco Smoke and Involuntary Smoking. IARC Monographs on the Evaluation of Carcinogenic Risks to Humans, Vol. 83. IARC: Lyon, France.
Ishibashi, T., Kawaguchi, M., Sugimoto, K., Uekita, H., Sakamoto, N., Yokoyama, K., Maruyama, Y., and Takeishi, Y. (2010). Advanced glycation end product-mediated matrix metallo-proteinase-9 and apoptosis via renin-angiotensin system in type 2 diabetes. J. Atheroscler. Thromb. 17, 578–589.
Janson, C. (2004). The effect of passive smoking on respiratory health in children and adults. Int. J. Tuberc. Lung Dis. 8, 510–516.
Jin, Q., Chen, H., Luo, A., Ding, F., and Liu, Z. (2011). S100A14 stimulates cell proliferation and induces cell apoptosis at different concentrations via receptor for advanced glycation end products (RAGE). PLoS ONE 6:e19375. doi: 10.1371/journal.pone.0019375
Johnson, W. R., Hale, R. W., Clough, S. C., and Chen, P. H. (1973). Chemistry of the conversion of nitrate nitrogen to smoke products. Nature 243, 223–225.
Kamioka, M., Ishibashi, T., Ohkawara, H., Nagai, R., Sugimoto, K., Uekita, H., Matsui, T., Yamagishi, S., Ando, K., Sakamoto, T., Sakamoto, N., Takuwa, Y., Wada, I., Shiomi, M., Maruyama, Y., and Takeishi, Y. (2011). Involvement of membrane type 1-matrix metalloproteinase (MT1-MMP) in RAGE activation signaling pathways. J. Cell. Physiol. 226, 1554–1563.
Kang, M. J., Choi, J. M., Kim, B. H., Lee, C. M., Cho, W. K., Choe, G., Kim, D. H., Lee, C. G., and Elias, J. A. (2012). IL-18 induces emphysema, and airway and vascular remodeling via IFN-gamma, IL-17A and IL-13. Am. J. Respir. Crit. Care Med. 185, 1205–1217.
Kasahara, Y., Tuder, R. M., Cool, C. D., Lynch, D. A., Flores, S. C., and Voelkel, N. F. (2001). Endothelial cell death and decreased expression of vascular endothelial growth factor and vascular endothelial growth factor receptor 2 in emphysema. Am. J. Respir. Crit. Care Med. 163, 737–744.
Kasahara, Y., Tuder, R. M., Taraseviciene-Stewart, L., Le Cras, T. D., Abman, S., Hirth, P. K., Waltenberger, J., and Voelkel, N. F. (2000). Inhibition of VEGF receptors causes lung cell apoptosis and emphysema. J. Clin. Invest. 106, 1311–1319.
Keatings, V. M., Collins, P. D., Scott, D. M., and Barnes, P. J. (1996). Differences in interleukin-8 and tumor necrosis factor-alpha in induced sputum from patients with chronic obstructive pulmonary disease or asthma. Am. J. Respir. Crit. Care Med. 153, 530–534.
Kim, J., Wan, C. K., J O'Carroll, S., Shaikh, S. B., and Nicholson, L. F. (2012). The role of receptor for advanced glycation end products (RAGE) in neuronal differentiation. J. Neurosci. Res. 90, 1136–1147.
Kim, S. W., Lim, C. M., Kim, J. B., Shin, J. H., Lee, S., Lee, M., and Lee, J. K. (2011). Extracellular HMGB1 released by NMDA treatment confers neuronal apoptosis via RAGE-p38 MAPK/ERK signaling pathway. Neurotox. Res. 20, 159–169.
Kim, V., Rogers, T. J., and Criner, G. J. (2008). New concepts in the pathobiology of chronic obstructive pulmonary disease. Proc. Am. Thorac. Soc. 5, 478–485.
Kirsti, H.-P. (2004). Genotoxicity of environmental tobacco smoke: a review. Mutat. Res. 567, 427–445.
Koslowski, R., Barth, K., Augstein, A., Tschernig, T., Bargsten, G., Aufderheide, M., and Kasper, M. (2004). A new rat type I-like alveolar epithelial cell line R3/1, bleomycin effects on caveolin expression. Histochem. Cell Biol. 121, 509–519.
Kuschner, W. G., D'Alessandro, A., Wong, H., and Blanc, P. D. (1996). Dose-dependent cigarette smoking-related inflammatory responses in healthy adults. Eur. Respir. J. 9, 1989–1994.
Lappalainen, U., Whitsett, J. A., Wert, S. E., Tichelaar, J. W., and Bry, K. (2005). Interleukin-1beta causes pulmonary inflammation, emphysema, and airway remodeling in the adult murine lung. Am. J. Physiol. Respir. Cell Mol. Physiol. 32, 311–318.
Lee, B. W., Chae, H. Y., Kwon, S. J., Park, S. Y., Ihm, J., and Ihm, S. H. (2010). RAGE ligands induce apoptotic cell death of pancreatic beta-cells via oxidative stress. Int. J. Mol. Med. 26, 813–818.
Li, J., and Schmidt, A. M. (1997). Characterization and functional analysis of the promoter of RAGE, the receptor for advanced glycation end products. J. Biol. Chem. 272, 16498–16506.
Li, J. H., Wang, W., Huang, X. R., Oldfield, M., Schmidt, A. M., Cooper, M. E., and Lan, H. Y. (2004). Advanced glycation end products induce tubular epithelial-myofibroblast transition through the RAGE-ERK1/2 MAP kinase signaling pathway. Am. J. Pathol. 164, 1389–1397.
Lin, L., Park, S., and Lakatta, E. G. (2009). RAGE signaling in inflammation and arterial aging. Front. Biosci. 14, 1403–1413.
Lucas, R., Verin, A. D., Black, S. M., and Catravas, J. D. (2009). Regulators of endothelial and epithelial barrier integrity and function in acute lung injury. Biochem. Pharmacol. 77, 1763–1772.
Lugade, A. A., Bogner, P. N., and Thanavala, Y. (2011). Murine model of chronic respiratory inflammation. Adv. Exp. Med. Biol. 780, 125–141.
Mahali, S., Raviprakash, N., Raghavendra, P. B., and Manna, S. K. (2011). Advanced glycation end products (AGEs) induce apoptosis via a novel pathway: involvement of Ca2+ mediated by interleukin-8 protein. J. Biol. Chem. 286, 34903–34913.
Majo, J., Ghezzo, H., and Cosio, M. G. (2001). Lymphocyte population and apoptosis in the lungs of smokers and their relation to emphysema. Eur. Respir. J. 17, 946–953.
Moodie, F. M., Marwick, J. A., Anderson, C. S., Szulakowski, P., Biswas, S. K., Bauter, M. R., Kilty, I., and Rahman, I. (2004). Oxidative stress and cigarette smoke alter chromatin remodeling but differentially regulate NF-kappaB activation and proinflammatory cytokine release in alveolar epithelial cells. FASEB J. 18, 1897–1899.
Morbini, P., Villa, C., Campo, I., Zorzetto, M., Inghilleri, S., and Luisetti, M. (2006). The receptor for advanced glycation end products and its ligands: a new inflammatory pathway in lung disease? Mod. Pathol. 19, 1437–1445.
National Toxicology Program. (2005). Report on Carcinogens, 11th Edn. US Department of Health and Human Services, Public Health Service, National Toxicology Program.
Nicholl, I. D., and Bucala, R. (1998). Advanced glycation endproducts and cigarette smoking. Cell. Mol. Biol. 44, 1025–1033.
Nicholl, I. D., Stitt, A. W., Moore, J. E., Ritchie, A. J., Archer, D. B., and Bucala, R. (1998). Increased levels of advanced glycation endproducts in the lenses and blood vessels of cigarette smokers. Mol. Med. 4, 594–601.
Ning, W., Li, C. J., Kaminski, N., Feghali-Bostwick, C. A., Alber, S. M., Di, Y. P., Otterbein, S. L., Song, R., Hayashi, S., Zhou, Z., Pinsky, D. J., Watkins, S. C., Pilewski, J. M., Sciurba, F. C., Peters, D. G., Hogg, J. C., and Choi, A. M. (2004). Comprehensive gene expression profiles reveal pathways related to the pathogenesis of chronic obstructive pulmonary disease. Proc. Natl. Acad. Sci. U.S.A. 101, 14895–14900.
O'Donnell, R. A., Peebles, C., Ward, J. A., Daraker, A., Angco, G., Broberg, P., Pierrou, S., Lund, J., Holgate, S. T., Davies, D. E., Delany, D. J., Wilson, S. J., and Djukanovic, R. (2004). Relationship between peripheral airway dysfunction, airway obstruction, and neutrophilic inflammation in COPD. Thorax 59, 837–842.
Ohnishi, K., Takagi, M., Kurokawa, Y., Satomi, S., and Konttinen, Y. T. (1998). Matrix metalloproteinase-mediated extracellular matrix protein degradation in human pulmonary emphysema. Lab. Invest. 78, 1077–1087.
Pakhale, S. S., and Maru, G. B. (1998). Distribution of major and minor alkaloids in tobacco, mainstream and sidestream smoke of popular Indian smoking products. Food Chem. Toxicol. 36, 1131–1138.
Patrianakos, C., and Hoffmann, D. (1979). Chemical studies on tobacco smoke LXIV. On the analysis of aromatic amines in cigarette smoke. J. Anal. Toxicol. 3, 150–154.
Pauwels, R. A., Buist, A. S., Calverley, P. M., Jenkins, C. R., and Hurd, S. S. (2001). Global strategy for the diagnosis, management, and prevention of chronic obstructive pulmonary disease. NHLBI/WHO Global Initiative for Chronic Obstructive Lung Disease (GOLD) Workshop summary. Am. J. Respir. Crit. Care Med. 163, 1256–1276.
Peinado, V. I., Barbera, J. A., Ramirez, J., Gomez, F. P., Roca, J., Jover, L., Gimferrer, J. M., and Rodriguez-Roisin, R. (1998). Endothelial dysfunction in pulmonary arteries of patients with mild COPD. Am. J. Physiol. 274, L908–L913.
Petrache, I., Fijalkowska, I., Zhen, L., Medler, T. R., Brown, E., Cruz, P., Choe, K. H., Taraseviciene-Stewart, L., Scerbavicius, R., Shapiro, L., Zhang, B., Song, S., Hicklin, D., Voelkel, N. F., Flotte, T., and Tuder, R. M. (2006). A novel antiapoptotic role for alpha1-antitrypsin in the prevention of pulmonary emphysema. Am. J. Respir. Crit. Care Med. 173, 1222–1228.
Petrache, I., Natarajan, V., Zhen, L., Medler, T. R., Richter, A. T., Cho, C., Hubbard, W. C., Berdyshev, E. V., and Tuder, R. M. (2005). Ceramide upregulation causes pulmonary cell apoptosis and emphysema-like disease in mice. Nat. Med. 11, 491–498.
Pollreisz, A., Hudson, B. I., Chang, J. S., Qu, W., Cheng, B., Papapanou, P. N., Schmidt, A. M., and Lalla, E. (2010). Receptor for advanced glycation endproducts mediates pro-atherogenic responses to periodontal infection in vascular endothelial cells. Atherosclerosis 212, 451–456.
Pratico, D., Basili, S., Vieri, M., Cordova, C., Violi, F., and Fitzgerald, G. A. (1998). Chronic obstructive pulmonary disease is associated with an increase in urinary levels of isoprostane F2alpha-III, an index of oxidant stress. Am. J. Respir. Crit. Care Med. 158, 1709–1714.
Reddy, M. A., Li, S. L., Sahar, S., Kim, Y. S., Xu, Z. G., Lanting, L., and Natarajan, R. (2006). Key role of Src kinase in S100B-induced activation of the receptor for advanced glycation end products in vascular smooth muscle cells. J. Biol. Chem. 281, 13685–13693.
Reynolds, P. R., Cosio, M. G., and Hoidal, J. R. (2006). Cigarette smoke-induced Egr-1 upregulates proinflammatory cytokines in pulmonary epithelial cells. Am. J. Respir. Cell Mol. Biol. 35, 314–319.
Reynolds, P. R., Kasteler, S. D., Cosio, M. G., Sturrock, A., Huecksteadt, T., and Hoidal, J. R. (2008). RAGE: developmental expression and positive feedback regulation by Egr-1 during cigarette smoke exposure in pulmonary epithelial cells. Am. J. Physiol. Lung Cell. Mol. Physiol. 294, L1094–L1101.
Reynolds, P. R., Kasteler, S. D., Schmitt, R. E., and Hoidal, J. R. (2011a). Receptor for advanced glycation end-products signals through Ras during tobacco smoke-induced pulmonary inflammation. Am. J. Respir. Cell Mol. Biol. 45, 411–418.
Reynolds, P. R., Stogsdill, J. A., Stogsdill, M. P., and Heimann, N. B. (2011b). Up-regulation of RAGE by alveolar epithelium influences cytodifferentiation and causes severe lung hypoplasia. Am. J. Respir. Cell Mol. Biol. 45, 1195–1202.
Reynolds, P. R., Schmitt, R. E., Kasteler, S. D., Sturrock, A., Sanders, K., Bierhaus, A., Nawroth, P. P., Paine, R. 3rd., and Hoidal, J. R. (2010). Receptors for advanced glycation end-products targeting protect against hyperoxia-induced lung injury in mice. Am. J. Respir. Cell Mol. Biol. 42, 545–551.
Rickert, W. S., Robinson, J. C., and Collishaw, N. (1984). Yields of tar, nicotine, and carbon monoxide in the sidestream smoke from 15 brands of Canadian cigarettes. Am. J. Public Health 74, 228–231.
Riuzzi, F., Sorci, G., Beccafico, S., and Donato, R. (2012). S100B engages RAGE or bFGF/FGFR1 in myoblasts depending on its own concentration and myoblast density. Implications for muscle regeneration. PLoS ONE 7:e28700. doi: 10.1371/journal.pone.0028700
Robinson, A. B., Johnson, K. D., Bennion, B. G., and Reynolds, P. R. (2012). RAGE signaling by alveolar macrophages influences tobacco smoke-induced inflammation. Am. J. Physiol. Lung Cell. Mol. Physiol. 302, L1192–L1199.
Rooney, C., and Sethi, T. (2011). The epithelial cell and lung cancer: the link between chronic obstructive pulmonary disease and lung cancer. Respiration 81, 89–104.
Ruhl, C., Adams, J. D., and Hoffmann, D. (1980). Chemical studies on tobacco-specific N-nitrosamines in the smoke of selected cigarettes from the U.S.A., West Germany, and France. J. Anal. Toxicol. 4, 255–259.
Sakaguchi, M., Murata, H., Yamamoto, K., Ono, T., Sakaguchi, Y., Motoyama, A., Hibino, T., Kataoka, K., and Huh, N. H. (2011). TIRAP, an adaptor protein for TLR2/4, transduces a signal from RAGE phosphorylated upon ligand binding. PLoS ONE 6:e23132. doi: 10.1371/journal.pone.0023132
Saugstad, O. D. (2003). Bronchopulmonary dysplasia-oxidative stress and antioxidants. Semin. Neonatol. 8, 39–49.
Schmidt, A. M. (2001). The multiligand receptor RAGE as a progression factor amplifying immune and inflammatory responses. J. Clin. Invest. 108, 949–955.
Segura-Valdez, L., Pardo, A., Gaxiola, M., Uhal, B. D., Becerril, C., and Selman, M. (2000). Upregulation of gelatinases A and B, collagenases 1 and 2, and increased parenchymal cell death in COPD. Chest 117, 684–694.
Sethi, J. M., and Rochester, C. L. (2000). Smoking and chronic obstructive pulmonary disease. Clin. Chest Med. 21, 67–86. viii.
Shapiro, S. D. (1994). Elastolytic metalloproteinases produced by human mononuclear phagocytes. Potential roles in destructive lung disease. Am. J. Respir. Crit. Care Med. 150, S160–S164.
Shapiro, S. D., Kobayashi, D. K., and Ley, T. J. (1993). Cloning and characterization of a unique elastolytic metalloproteinase produced by human alveolar macrophages. J. Biol. Chem. 268, 23824–23829.
Shaykhiev, R., Krause, A., Salit, J., Strulovici-Barel, Y., Harvey, B. G., O'Connor, T. P., and Crystal, R. G. (2009). Smoking-dependent reprogramming of alveolar macrophage polarization: implication for pathogenesis of chronic obstructive pulmonary disease. J. Immunol. 183, 2867–2883.
Slee, E. A., Harte, M. T., Kluck, R. M., Wolf, B. B., Casiano, C. A., Newmeyer, D. D., Wang, H. G., Reed, J. C., Nicholson, D. W., Alnemri, E. S., Green, D. R., and Martin, S. J. (1999). Ordering the cytochrome c-initiated caspase cascade: hierarchical activation of caspases-2, -3, -6, -7, -8, and -10 in a caspase-9-dependent manner. J. Cell Biol. 144, 281–292.
Speer, C. P. (2006). Inflammation and bronchopulmonary dysplasia: a continuing story. Semin. Fetal Neonatal Med. 11, 354–362.
Stanescu, D., Sanna, A., Veriter, C., Kostianev, S., Calcagni, P. G., Fabbri, L. M., and Maestrelli, P. (1996). Airways obstruction, chronic expectoration, and rapid decline of FEV1 in smokers are associated with increased levels of sputum neutrophils. Thorax 51, 267–271.
Steenvoorden, M. M., Huizinga, T. W., Verzijl, N., Bank, R. A., Ronday, H. K., Luning, H. A., Lafeber, F. P., Toes, R. E., and Degroot, J. (2006). Activation of receptor for advanced glycation end products in osteoarthritis leads to increased stimulation of chondrocytes and synoviocytes. Arthritis Rheum. 54, 253–263.
Sternberg, D. I., Gowda, R., Mehra, D., Qu, W., Weinberg, A., Twaddell, W., Sarkar, J., Wallace, A., Hudson, B., D'Ovidio, F., Arcasoy, S., Ramasamy, R., D'Armiento, J., Schmidt, A. M., and Sonett, J. R. (2008). Blockade of receptor for advanced glycation end product attenuates pulmonary reperfusion injury in mice. J. Thorac. Cardiovasc. Surg. 136, 1576–1585.
Stockley, R. A., Mannino, D., and Barnes, P. J. (2009). Burden and pathogenesis of chronic obstructive pulmonary disease. Proc. Am. Thorac. Soc. 6, 524–526.
Stogsdill, J. A., Stogsdill, M. P., Porter, J. L., Hancock, J. M., Robinson, A. B., and Reynolds, P. R. (2012). Embryonic over-expression of RAGE by alveolar epithelium induces an imbalance between proliferation and apoptosis. Am. J. Respir. Cell Mol. Biol. 47, 60–66.
Stogsdill, M. P., Stogsdill, J. A., Porter, J. L., Bodine, G., and Reynolds, P. R. (2011). Persistent over-expression of RAGE in adult mouse lung causes airspace enlargement coincident with emphysema. FASEB J. 25, 114.
Su, X., Lee, J. W., Matthay, Z. A., Mednick, G., Uchida, T., Fang, X., Gupta, N., and Matthay, M. A. (2007). Activation of the alpha7 nAChR reduces acid-induced acute lung injury in mice and rats. Am. J. Respir. Cell Mol. Biol. 37, 186–192.
Su, X., Looney, M. R., Gupta, N., and Matthay, M. A. (2009). Receptor for advanced glycation end-products (RAGE) is an indicator of direct lung injury in models of experimental lung injury. Am. J. Physiol. Lung Cell. Mol. Physiol 297, L1–L5.
Suga, T., Iso, T., Shimizu, T., Tanaka, T., Yamagishi, S., Takeuchi, M., Imaizumi, T., and Kurabayashi, M. (2011). Activation of receptor for advanced glycation end products induces osteogenic differentiation of vascular smooth muscle cells. J. Atheroscler. Thromb. 18, 670–683.
Sun, C., Liang, C., Ren, Y., Zhen, Y., He, Z., Wang, H., Tan, H., Pan, X., and Wu, Z. (2009). Advanced glycation end products depress function of endothelial progenitor cells via p38 and ERK 1/2 mitogen-activated protein kinase pathways. Basic Res. Cardiol. 104, 42–49.
Taguchi, A., Blood, D. C., Del Toro, G., Canet, A., Lee, D. C., Qu, W., Tanji, N., Lu, Y., Lalla, E., Fu, C., Hofmann, M. A., Kislinger, T., Ingram, M., Lu, A., Tanaka, H., Hori, O., Ogawa, S., Stern, D. M., and Schmidt, A. M. (2000). Blockade of RAGE-amphoterin signalling suppresses tumour growth and metastases. Nature 405, 354–360.
Talhout, R., Schulz, T., Florek, E., Van Benthem, J., Wester, P., and Opperhuizen, A. (2011). Hazardous compounds in tobacco smoke. Int. J. Environ. Res. Public Health 8, 613–628.
Tanino, M., Betsuyaku, T., Takeyabu, K., Tanino, Y., Yamaguchi, E., Miyamoto, K., and Nishimura, M. (2002). Increased levels of interleukin-8 in BAL fluid from smokers susceptible to pulmonary emphysema. Thorax 57, 405–411.
Thompson, A. B., Daughton, D., Robbins, R. A., Ghafouri, M. A., Oehlerking, M., and Rennard, S. I. (1989). Intraluminal airway inflammation in chronic bronchitis. Characterization and correlation with clinical parameters. Am. Rev. Respir. Dis. 140, 1527–1537.
Thun, M. J., Apicella, L. F., and Henley, S. J. (2000). Smoking vs other risk factors as the cause of smoking-attributable deaths. JAMA 284, 706–712.
Toure, F., Zahm, J. M., Garnotel, R., Lambert, E., Bonnet, N., Schmidt, A. M., Vitry, F., Chanard, J., Gillery, P., and Rieu, P. (2008). Receptor for advanced glycation end-products (RAGE) modulates neutrophil adhesion and migration on glycoxidated extracellular matrix. Biochem. J. 416, 255–261.
Tsoporis, J. N., Izhar, S., Leong-Poi, H., Desjardins, J. F., Huttunen, H. J., and Parker, T. G. (2010). S100B interaction with the receptor for advanced glycation end products (RAGE): a novel receptor-mediated mechanism for myocyte apoptosis postinfarction. Circ. Res. 106, 93–101.
Tuder, R. M., Petrache, I., Elias, J. A., Voelkel, N. F., and Henson, P. M. (2003). Apoptosis and emphysema: the missing link. Am. J. Respir. Cell Mol. Biol. 28, 551–554.
Uchida, T., Shirasawa, M., Ware, L. B., Kojima, K., Hata, Y., Makita, K., Mednick, G., Matthay, Z. A., and Matthay, M. A. (2006). Receptor for advanced glycation end-products is a marker of type I cell injury in acute lung injury. Am. J. Respir. Crit. Care Med. 173, 1008–1015.
US Department of Health and Human Services. (2006). The Health Consequences of Involuntary Exposure to Tobacco Smoke: A Report of the Surgeon General. Rockville, MD: US Department of Health and Human Services, Centers for Disease Control and Prevention, Coordinating Center for Health Promotion, National Center for Chronic Disease Prevention and Health Promotion, Office on Smoking and Health.
US Department of Health and Human Services. (2009). Morbidity and Mortality: 2009 Chart Book on Cardiovascular, Lung and Blood Diseases. National Institutes of Health. National Heart Lung and Blood Institute.
Wakefield, M., Cameron, M., Inglis, G., Letcher, T., and Durkin, S. (2005). Secondhand smoke exposure and respiratory symptoms among casino, club, and office workers in Victoria, Australia. J. Occup. Environ. Med. 47, 698–703.
Wolfson, R. K., Chiang, E. T., and Garcia, J. G. (2011). HMGB1 induces human lung endothelial cell cytoskeletal rearrangement and barrier disruption. Microvasc. Res. 81, 189–197.
Wright, J. L., and Churg, A. (1990). Cigarette smoke causes physiologic and morphologic changes of emphysema in the guinea pig. Am. Rev. Respir. Dis. 142, 1422–1428.
Wu, Q., Jiang, D., and Chu, H. W. (2011). Cigarette smoke induces growth differentiation factor 15 production in human lung epithelial cells: implication in mucin over-expression. Innate Immun. 18, 617–626.
Xia, J. R., Liu, N. F., and Zhu, N. X. (2008). Specific siRNA targeting the receptor for advanced glycation end products inhibits experimental hepatic fibrosis in rats. Int. J. Mol. Sci. 9, 638–661.
Yamakawa, N., Uchida, T., Matthay, M. A., and Makita, K. (2011). Proteolytic release of the receptor for advanced glycation end products from in vitro and in situ alveolar epithelial cells. Am. J. Physiol. Lung Cell. Mol. Physiol. 300, L516–L525.
Yan, S. F., Ramasamy, R., and Schmidt, A. M. (2009). The receptor for advanced glycation endproducts (RAGE) and cardiovascular disease. Expert Rev. Mol. Med. 11, e9.
Yokohori, N., Aoshiba, K., and Nagai, A. (2004). Increased levels of cell death and proliferation in alveolar wall cells in patients with pulmonary emphysema. Chest 125, 626–632.
Yu, S., Li, H., Ma, Y., and Fu, Y. (2012). Matrix metalloproteinase-1 of gingival fibroblasts influenced by advanced glycation end products (AGEs) and their association with receptor for AGEs and nuclear factor-kappaB in gingival connective tissue. J. Periodontol. 83, 119–126.
Zhang, F., Banker, G., Liu, X., Suwanabol, P. A., Lengfeld, J., Yamanouchi, D., Kent, K. C., and Liu, B. (2010). The novel function of advanced glycation end products in regulation of MMP-9 production. J. Surg. Res. 171, 871–876.
Zhang, H., Tasaka, S., Shiraishi, Y., Fukunaga, K., Yamada, W., Seki, H., Ogawa, Y., Miyamoto, K., Nakano, Y., Hasegawa, N., Miyasho, T., Maruyama, I., and Ishizaka, A. (2008). Role of soluble receptor for advanced glycation end products on endotoxin-induced lung injury. Am. J. Respir. Crit. Care Med. 178, 356–362.
Zhu, P., Ren, M., Yang, C., Hu, Y. X., Ran, J. M., and Yan, L. (2012). Involvement of RAGE, MAPK and NF-kappaB pathways in AGEs-induced MMP-9 activation in HaCaT keratinocytes. Exp. Dermatol. 21, 123–129.
Keywords: RAGE, COPD, tobacco, mouse model
Citation: Robinson AB, Stogsdill JA, Lewis JB, Wood TT and Reynolds PR (2012) RAGE and tobacco smoke: insights into modeling chronic obstructive pulmonary disease. Front. Physio. 3:301. doi: 10.3389/fphys.2012.00301
Received: 19 April 2012; Paper pending published: 29 May 2012;
Accepted: 10 July 2012; Published online: 25 July 2012.
Edited by:
Laima Taraseviciene-Stewart, University of Colorado Denver, USACopyright © 2012 Robinson, Stogsdill, Lewis, Wood and Reynolds. This is an open-access article distributed under the terms of the Creative Commons Attribution License, which permits use, distribution and reproduction in other forums, provided the original authors and source are credited and subject to any copyright notices concerning any third-party graphics etc.
*Correspondence: Paul R. Reynolds, Department of Physiology and Developmental Biology, Brigham Young University, 375A Widtsoe Building, Provo, UT 84602, USA. e-mail: paul_reynolds@byu.edu