- 1Biological Physics Group, School of Physics and Astronomy, University of Manchester, Manchester, UK
- 2School of Computer Science and Technology, Harbin Institute of Technology, Harbin, China
- 3Multidisciplinary Cardiovascular Research Centre, Institute for Membrane and Systems Biology, University of Leeds, Leeds, UK
- 4Faculty of Medical and Human Sciences, University of Manchester, Manchester, UK
- 5School of Physiology and Pharmacology and Cardiovascular Research Laboratories, Medical Sciences Building, University Walk, Bristol, UK
Introduction: β-adrenergic stimulation increases the heart rate by accelerating the electrical activity of the pacemaker of the heart, the sinoatrial node (SAN). Ionic mechanisms underlying the actions of β-adrenergic stimulation are not yet fully understood. Isoprenaline (ISO), a β-adrenoceptor agonist, shifts voltage-dependent If activation to more positive potentials resulting in an increase of If, which has been suggested to be the main mechanism underlying the effect of β-adrenergic stimulation. However, ISO has been found to increase the firing rate of rabbit SAN cells when If is blocked. ISO also increases ICaL, Ist, IKr, and IKs; and shifts the activation of IKr to more negative potentials and increases the rate of its deactivation. ISO has also been reported to increase the intracellular Ca2+ transient, which can contribute to chronotropy by modulating the “Ca2+ clock.” The aim of this study was to analyze the ionic mechanisms underlying the positive chronotropy of β-adrenergic stimulation using two distinct and well established computational models of the electrical activity of rabbit SAN cells. Methods and results: We modified the Boyett et al. (2001) and Kurata et al. (2008) models of electrical activity for the central and peripheral rabbit SAN cells by incorporating equations for the known dose-dependent actions of ISO on various ionic channel currents (ICaL, Ist, IKr, and IKs), kinetics of IKr and If, and the intracellular Ca2+ transient. These equations were constructed from experimental data. To investigate the ionic basis of the effects of ISO, we simulated the chronotropic effect of a range of ISO concentrations when ISO exerted all its actions or just a subset of them. Conclusion: In both the Boyett et al. and Kurata et al. SAN models, the chronotropic effect of ISO was found to result from an integrated action of ISO on ICaL, If, Ist, IKr, and IKs, among which an increase in the rate of deactivation of IKr plays a prominent role, though the effect of ISO on If and [Ca2+]i also plays a role.
Introduction
β-Adrenergic stimulation increases the heart rate through accelerating the spontaneous activity of the pacemaker of the heart, the sinoatrial node (SAN; Abramochkin et al., 2009). It is believed that this occurs through β-adrenoceptor mediated modulation of ionic currents that contribute to pacemaker activity; however the precise ionic mechanisms underlying the effect of β-adrenergic stimulation are not yet fully elucidated. Experiments have shown that isoprenaline (ISO), a β-adrenergic agonist, increases the L-type calcium current (ICaL; Noma et al., 1980; Walsh et al., 1988; Zaza et al., 1996; Vinogradova et al., 2002; Ke et al., 2007; Alig et al., 2009), delayed rectifier potassium current (both IKr and IKs; Walsh et al., 1988; Duchatelle-Gourdon et al., 1989; Giles et al., 1989; Yazawa and Kameyama, 1990; Freeman and Kass, 1993;Lei et al., 2000, 2002; Ke et al., 2007; Vinogradova et al., 2008), and shifts voltage-dependent activation of the hyperpolarization activated current (If) toward positive potentials resulting in an increase of If (Brown et al., 1979; Cai et al., 1995; DiFrancesco, 1995; Zaza et al., 1996; Accili et al., 1997a,b; Bucchi et al., 2003; Barbuti et al., 2007; Alig et al., 2009; Baruscotti et al., 2010; Liao et al., 2010). As If has been regarded to be a major pacemaker current in mammalian pacemaker cells, an increase in If has been suggested to be the main mechanism underlying the positive chronotropic effect of β-adrenergic stimulation (DiFrancesco, 1995, 2010; Zaza et al., 1996; DiFrancesco and Borer, 2007; Liao et al., 2010). However, ISO has been found to increase the firing rate of the rabbit SAN when If was blocked by Cs+ (Cai et al., 1995), suggesting that If enhancement may not be predominantly responsible for the positive chronotropic action of ISO. Previous studies have shown that ISO increases the amplitude of the systolic rise of intracellular Ca2+ concentration ([Ca2+]i) in cardiac cells (Ju and Allen, 1999; Huser et al., 2000; Shannon et al., 2004; Maltsev and Lakatta, 2009; Wu et al., 2009). This raises the possibility that changes to [Ca2+]i with ISO might contribute to the increase in firing rate via the “Ca2+ clock” mechanism (Vinogradova et al., 2002, 2008; Maltsev and Lakatta, 2009). A number of studies have shown that interventions altering [Ca2+]i change the firing rate of pacemaker cells (Hagiwara, 1989; Ju and Allen, 1999; Huser et al., 2000; Shannon et al., 2004; Vinogradova et al., 2008; Maltsev and Lakatta, 2009; Wu et al., 2009). In both mammalian cardiac cells (Hagiwara, 1989; Huser et al., 2000; Vinogradova et al., 2002, 2008; Maltsev and Lakatta, 2009; Wu et al., 2009) and amphibian pacemaker cells in which If is absent (Ju and Allen, 1999), it has been found that the firing rate was dependent on the amplitude of the [Ca2+]i transient: agents modifying SR Ca2+ release consequently affect the firing rate. β-Adrenergic stimulation increases the amplitude of the [Ca2+]i transient. It has been argued that much of the increase in firing rate caused by β-stimulation seems to occur through the “Ca2+ clock” mechanism (Vinogradova et al., 2002, 2008; Maltsev and Lakatta, 2009). ISO has also been reported to increase the amplitude of the inward sustained current (Ist; Guo et al., 1995, 1997; Shinagawa and Noma, 2000; Toyoda et al., 2005), and the amplitude of the IKr and IKs (Lei et al., 2000, 2002). It has also been found to shift the activation curve of IKr toward more negative membrane potentials and to increase its rate of deactivation (Yazawa and Kameyama, 1990; Lei et al., 2000, 2002; Ke et al., 2007). As Ist is activated over a membrane potential range between −70 and −50 mV (Guo et al., 1995, 1997; Shinagawa and Noma, 2000), increases in Ist might play a role in accelerating the rate of pacemaker activity. An increase in the rate of deactivation of IKr decreases the outward current, resulting in a relatively larger net inward current during diastolic depolarization, which could also contribute to the increase of the rate of spontaneous activity. In this study, we modified existing models of the electrical activity of central and peripheral rabbit SAN cells (Boyett et al., 2001; Kurata et al., 2008) in order to simulate the positive chronotropic effect of ISO and study the underlying ionic basis for the actions of ISO using a computational approach. We have incorporated equations for concentration-dependent actions of ISO on the macroscopic conductance of ICaL, IKr and IKs, Ist and to describe changes in the kinetics of If and IKr, and in [Ca2+]i. These equations have been constructed from experimental data. Using the models, the simulated effect of ISO shows concentration-dependence, which is quantitatively consistent with experimental recordings. To investigate the underlying ionic basis the effect of ISO, we examined its effects over a range of concentrations on the currents responsible for the pacemaker activity of the SAN, and we have investigated the individual role of actions of ISO on currents of ICaL, If, IKr, Ist, and [Ca2+]i. We found that the chronotropic effect of ISO reflects integrated actions on ionic currents ICaL, If, IKr, IKs, Ist, and [Ca2+]i, amongst which the increase in the rate of deactivation of IKr appears to be particularly important, whilst the effect of ISO on If and [Ca2+]i also plays a role.
Model Development
Based on the voltage clamp experimental data on the kinetics of ionic channels and regional differences in the ionic current densities, well established action potentials models of the center and periphery of the rabbit SAN cells have been developed (Zhang et al., 2000; Boyett et al., 2001; Kurata et al., 2008). The Zhang et al. (2000) models were upgraded by incorporating an inward sustained current (Ist) and intracellular Ca2+ regulation equations (Boyett et al., 2001). In this study, we modified the models of Boyett et al. (2001) and Kurata et al. (2008) by including equations describing the actions of ISO on ICaL, If, IKr, IKs, Ist, and [Ca2+]i.
Increase of ICaL
Isoprenaline was found, experimentally, to increase ICaL without a significant change in its kinetics (Zaza et al., 1996). The increase in ICaL is dose dependent. The equation describing the dose-dependent increase of ICaL was constructed from experimental data of Zaza et al. (1996) obtained from rabbit SAN cells, which take the form:
Where fCa is the percentage of increase of ICaL. By fitting Eq. 1 to the data of Zaza et al. (1996; circles) and Vinogradova et al. (2002; triangles) as shown in Figure 1A, we obtained the best fit values for fCa,max (the maximum percentage increase ICaL) and K0.5,Ca (the ISO concentration required to half-maximally increase of ICaL) as 0.54 (i.e., 54%) and 7 nM, respectively. The obtained K0.5,Ca (7 nM) matches to the experimental data of EC50 of ISO on ICaL (Zaza et al., 1996). The solid line in Figure 1A shows the relationship between the increase in ICaL and the ISO concentration predicted by Eq. 1.
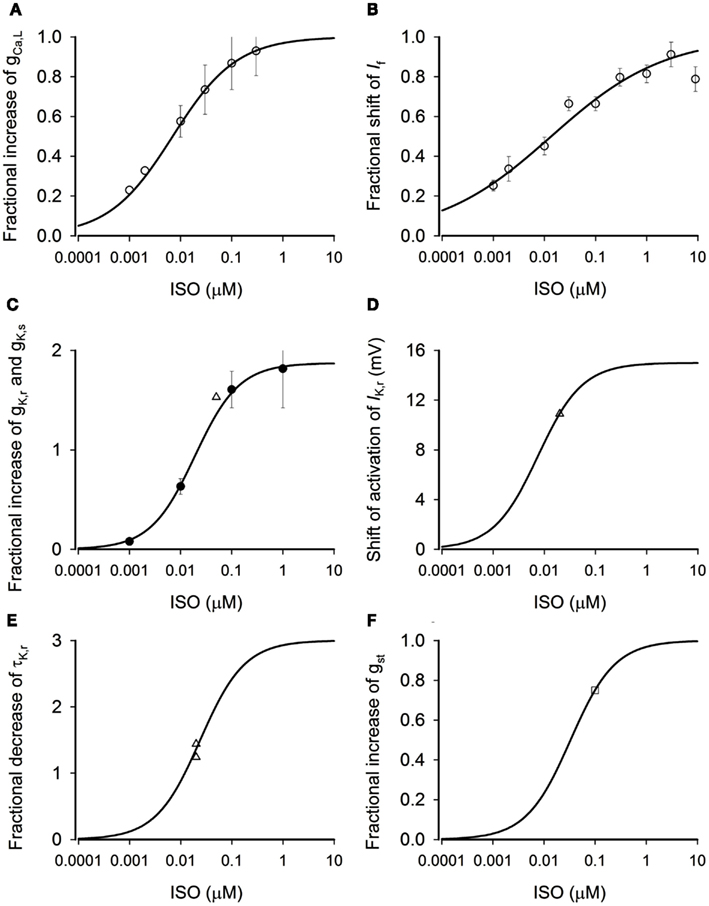
Figure 1. Concentration-dependent effects of ISO. (A) Dose-dependent fractional increase of gCaL. Open circles: experimental data from Zaza et al. (1996) from rabbit SAN cells. Solid line: computed from model. (B) Dose-dependent fractional shift of the If activation curve. Open circles: data from Zaza et al. (1996) from rabbit SAN cells. Solid line: computed from model. (C) Dose-dependent fractional increase of gKr. Solid circles: data from Yazawa and Kameyama (1990) from guinea-pig SAN cells. Open triangle: data from Lei et al. (2000) from rabbit SAN cells. Solid line: computed from model. (D) Dose-dependent shift of IKr activation curve. Open triangle: data from Lei et al. (2000) from rabbit SAN cells. Solid line: computed from model. (E) Dose-dependent fractional decrease of time constant of deactivation of IKr. Open triangles: data from Lei et al. (2000) from rabbit SAN cells. Solid line: computed from model. (F) Dose-dependent fractional increase of gst. Open square: data from Guo et al. (1995) from rabbit SAN cells. Solid line: computed from model.
Shift of the Activation Curve of If
Isoprenaline increases If by shifting its voltage-dependent activation curve toward more positive membrane potentials (Zaza et al., 1996; Accili et al., 1997a,b). The equation to describe the shift, Sf (in mV), was constructed from the experimental data of Zaza et al. (1996) obtained from rabbit SAN cells as shown below:
By fitting the Eq. 2 to the data of Zaza et al. (1996; Figure 1B, circles), we obtained the best fit values for Sf,max (the maximum shift), K0.5,f (the ISO concentration required to half-maximally shift the activation curve), and nf (the Hill coefficient) as 9.62 mV, 13.5 nM, and 0.392, respectively, which are close to those obtained by Zaza et al. (1996). The solid line in Figure 1B shows the relationship between the shift of If activation curve and the ISO concentration predicted by Eq. 2.
Actions on IKr and IKs
Isoprenaline has complex actions on IK. β-adrenergic agonists have been reported to increase IK in rabbit SAN cells (Lei et al., 2000, 2002), small multi-cellular preparations of rabbit SAN (Walsh et al., 1988), guinea-pig SAN cells (Freeman and Kass, 1993), guinea-pig ventricular myocytes (Yazawa and Kameyama, 1990), and frog atrial cells (Giles et al., 1989). In rabbit SAN cells, IK consists of two components, a rapidly activating IKr and a slowly activating IKs, of which IKr is normally the predominant component (Habuchi et al., 1995; Ito and Ono, 1995; Lei and Brown, 1996; Lei et al., 2000, 2002). ISO has been found to increase the current amplitude of IKr and IKs (Lei et al., 2000, 2002), increase the rate of deactivation of IKr in rabbit SAN cells (Lei et al., 2000; Ke et al., 2007), and shift the activation curve of IKr toward more negative membrane potentials in guinea-pig ventricular myocytes (Yazawa and Kameyama, 1990), frog atrial cells (Giles et al., 1989), and rabbit SAN cells (Lei et al., 2000).
Increase of gKr and gKs (IKr and IKs)
The equation for concentration-dependent increase in gKr was constructed from experimental data of Lei et al. (2000, 2002) obtained from rabbit SAN cells shown as in Figure 1C (triangles) and data of Yazawa and Kameyama (1990) obtained from guinea-pig ventricular myocytes shown as in Figure 1C (circles). The equation takes the form:
Where fK is the percentage increase of IKr. By fitting Eq. 3 to the experimental data of Lei et al. (2000, 2002) and Yazawa and Kameyama (1990), we obtained the best fit values for fK,max (the maximum percentage increase IK) and K0.5,Ca (the ISO concentration required to half-maximally increase of gKr) as 1.87 (i.e., 187%) and 19 nM, respectively. The solid line in Figure 1C shows the relationship between the increase in gKr and the ISO concentration predicted by Eq. 3.
Isoprenaline has also been found to increase IKs: experimental data have shown that 10 nM ISO increased IKs by about 20% (Lei et al., 2002), which is comparable to the increase of IKr with a similar ISO concentration. Due both to this similarity and to the lack of availability of complete experimental concentration-response data, in this study we assumed a similar concentration-dependent modulation by ISO of gKs and gKr, both of which were modeled by Eq. 3.
Shift in Activation of IKr
The equation to describe the shift in the voltage-dependent activation relation of IKr (SK) was constructed from experimental data of Lei et al. (2000) obtained from rabbit SAN cells. As there are not sufficient experimental data available in respect of concentration-dependence of the effect, we assumed that the concentration-dependent shift of the IKr activation curve is the same as dose-dependent increase of gKr, which takes the form:
By fitting Eq. 4 to the experimental data of Lei et al. (2000) as shown in Figure 1D (triangle), we obtained the best fit value of SK,max (the maximal shift of IK, in mV) as −15 mV and K0.5,Kacti as 7.5 nM.
Increase in the Rate of Deactivation of IKr
The equation describing the increase in the rate of deactivation of IKr was constructed from experimental data on IK from Lei et al. (2000) obtained from rabbit SAN cells, shown in Figure 1E (triangles). In the Figure, data are presented as the decrease of the time constant of deactivation (τKr). Once again, we assumed that the dose-dependent change of τKr is as the same as the dose-dependent increase of gKr. The equation takes the form:
is the fractional decrease of τKr. By fitting the equation to the experimental data of Lei et al. (2000), we obtained the best fit value of as 3.0 and K0.5, τKr as 24 nM.
Increase of Ist
Isoprenaline has been reported to increase Ist (Guo et al., 1995, 1997; Shinagawa and Noma, 2000; Toyoda et al., 2005). The equation for the increase of Ist was constructed from experimental data obtained from rabbit SAN cells (Guo et al., 1997). Due to a lack of availability of sufficient experimental data, we assumed that the concentration-dependent increase of Ist is the same as the concentration-dependent increase of gKr. The equation takes the form:
By fitting Eq. 6 to the experimental data of Guo et al. (1997) as shown in Figure 1F (open square), we obtained the best fit value of fst,max as 1.0 and K0.5,st as 33 nM.
Ca2+ Handling
Isoprenaline was found to increase the amplitude and minimal diastolic level of [Ca2+]i in mammalian (Hagiwara, 1989; Huser et al., 2000; Vinogradova et al., 2002, 2008; Shannon et al., 2004; Maltsev and Lakatta, 2009; Wu et al., 2009) and amphibian (Ju and Allen, 1999) pacemaker cells. It has been found that ISO altered SR Ca2+ uptake and release by stimulation of calmodulin kinase II (CamKII; Shannon et al., 2005; Maltsev and Lakatta, 2009). In simulations of the effect of ISO, we adopted the approach of Kharche et al. (2011) to modify the Ca2+ handling equations to increase the amplitude and minimal diastolic level of [Ca]i, as observed in experimental studies (Ju and Allen, 1999; Vinogradova et al., 2002) by increasing the maximal SR Ca2+ release (by 20%) and reducing the SR Ca2+ release (by 20%) fluxes.
Equations 1–6 were incorporated into the action potential mathematical models of Boyett et al. (2001) and Kurata et al. (2008) for central and peripheral rabbit SAN cells to simulate the chronotropic effect of ISO and to investigate the ionic basis underlying such a chronotropic effect.
Results
Chronotropic Effect of Isoprenaline
The simulated effects of ISO on spontaneous action potentials are shown in Figure 2. In the figure, the left-hand panels show the results computed from the central (A) and peripheral (C) Boyett et al. cell models, the right-hand panels show the results computed from the central (B) and peripheral (D) Kurata et al. cell models. For each cell-type and each model, the effects of ISO with a concentration of 0.05 μM are shown. In all cell models, ISO affected the shape of action potentials: it accelerated the firing rate, shortened the action potential duration, increased the amplitude of action potentials, and the maximal diastolic potential (the maximal diastolic potential became more negative). These simulated effects of ISO on action potentials are consistent with experimental observations on rabbit SAN cells (Zaza et al., 1996; Lei et al., 2000).
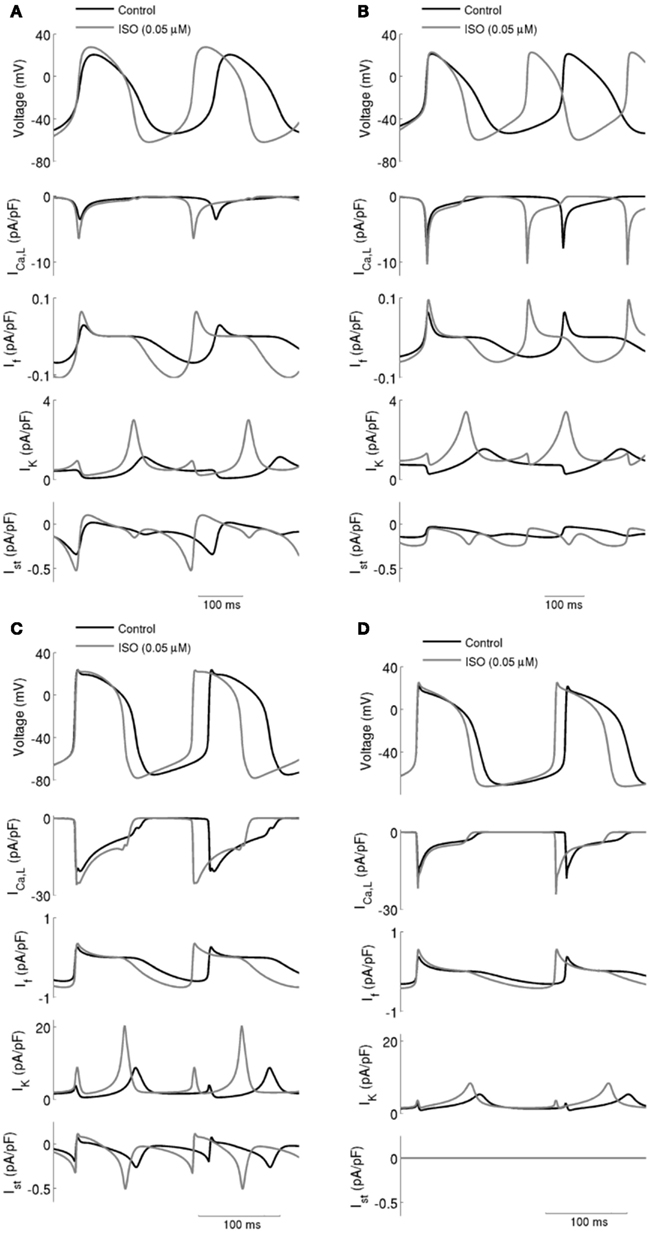
Figure 2. ICaL, If, IK (sum of IKr and IKs), and Ist during action potentials in control and with ISO. Data in control and with 0.05 μM ISO are shown for: (A) Boyett et al.; (B) Kurata et al. central cell models. (C) Boyett et al.; (D) Kurata et al. peripheral cell models. In all four cell models, ISO caused an increase in spontaneous firing rate and this was accompanied by increases in ICaL, If, IK, and Ist.
Effects of ISO on ionic currents underlying action potentials (upper panel in each of Figure 2; Left panels for the Boyett et al. central (Figure 2A) and peripheral (Figure 2C) cell models; and right panels for the Kurata et al. central (Figure 2B) and peripheral (Figure 2D) of cell model were then studied [namely on ICaL, If, IK (the sum of IKr and IKs), and Ist]. The profiles of ICaL (the second panel), If (the third panel), IK (the fourth panel), and Ist (the fifth panel) are shown in Figure 2 under control conditions and in the presence of 0.05 μM ISO. In the simulated presence of ISO the inward currents ICaL, If, and Ist were increased, while net IK was increased in the repolarization phase but decreased in the diastolic depolarization phase. The increased IK during action potential repolarization sped up the rate of repolarization (thereby shortening action potential duration) and increased the maximal diastolic potential. Increases of the inward currents ICaL, If, and Ist, together with a decrease of the outward current IK in the depolarization phase accelerated the rate of depolarization and therefore the rate of the spontaneous activity. The increase in action potential amplitude can be attributed to an increase in ICaL.
The simulated effects of ISO exhibited clear concentration-dependence: the higher the simulated ISO concentrations, the greater the observed chronotropic effect. This is shown in Figure 3, in which action potentials computed under control conditions are superimposed on those computed with different ISO concentrations for the central (A) and peripheral (C) Boyett et al. cell models (left-hand panels), and the central (B) and peripheral (D) Kurata et al. (right-hand panels) cell models. In simulations using the Boyett et al. (2001) models, 0.005 μM ISO increased the rate by 7% for the central cell model and by 6% for the peripheral cell model. 0.05 μM ISO increased the rate by 20% for the central cell model and 15% for the peripheral cell model. 0.5 μM ISO increased the rate by 21% for the central cell model and 16% for the peripheral cell model. In simulations using the Kurata et al. (2008) models, 0.005 μM ISO increased the rate by 13% for the central cell model but did not significantly change rate for the peripheral cell model. 0.05 μM ISO increased the rate by 25% for the central cell model and 7% for the peripheral cell model; 0.5 μM ISO increased the rate by 29% for the central cell model and 16% for the peripheral cell model. The results summarizing the simulated chronotropic effects of ISO are shown in Figure 4, in which the percentage of decrease of pacemaking cycle length (BCL) is plotted against ISO concentration. The data computed from the central cell models of Boyett et al. and Kurata et al. are shown in Figures 4A,B, respectively. The data computed from the peripheral cell models of Boyett et al. and Kurata et al. are shown in Figures 4C,D, respectively. The simulated data (solid line) were compared with the experimental data obtained from rabbit isolated SAN cells by Lei et al. (2000; open circle), Zaza et al., 1996; open right-facing triangle), Choi et al. (1999; open diamond), and Barbuti et al. (2007; open inverted triangle). Experimentally, 0.02 μM ISO increased the rate by 13% (Lei et al., 2000), similar to simulated response of all four cell models to the same ISO concentration. The discrepancy between the simulations and the experimental data of Zaza et al. (1996) or the Choi et al. (1999) is slight and could be attributable to model limitations (see Discussion). Note that the simulation data from the central cell models matched better to experimental data than the peripheral cell models as in those experimental studies only primary SAN cells were considered without distinguish between central and peripheral cells. The roles of ICaL, If, IKr, Ist, and [Ca2+]i in the ISO-produced positive chronotropy were also considered, as shown in Figure 5.
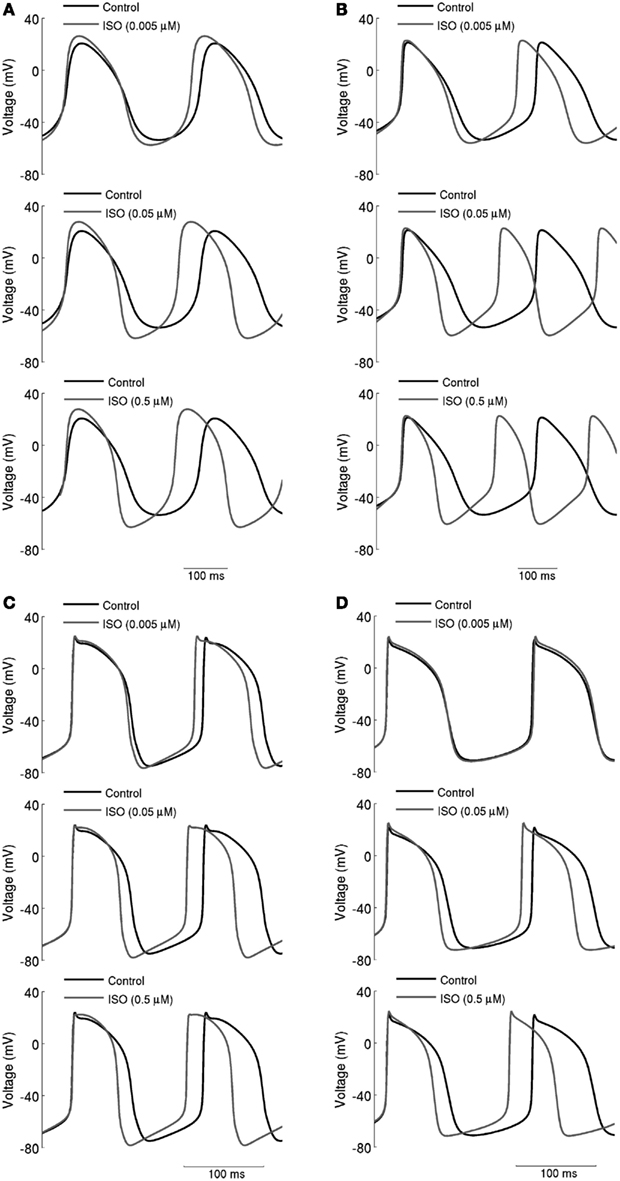
Figure 3. Concentration-dependent effects of ISO on action potentials. (A) Boyett et al.; (B) Kurata et al. central cell models. (C) Boyett et al.; (D) Kurata et al. peripheral cell models. Action potentials are shown under control conditions and in the presence 0.005, 0.05, and 0.5 μM ISO. Spontaneous firing rate was increased by ISO in a dose-dependent manner.
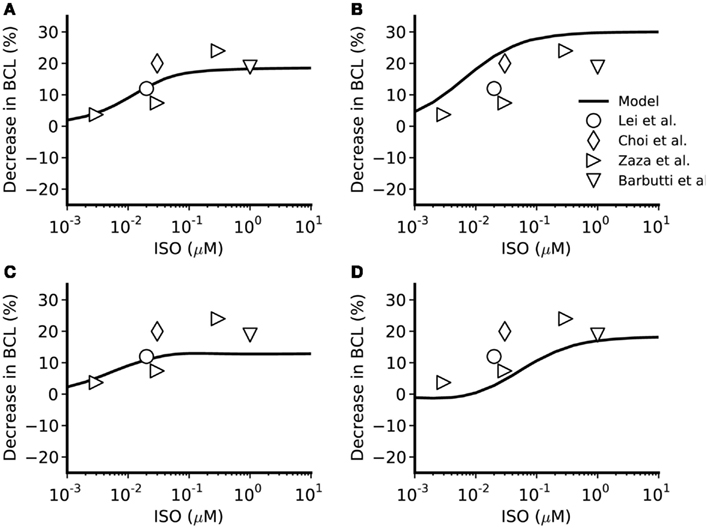
Figure 4. Concentration-dependent percentage decrease of pacemaking cycle length (BCL) by ISO. (A,B) Boyett et al. and Kurata et al. central cell models. (C,D) Boyett et al. and Kurata et al. peripheral cell models. The figure incorporates concentration-response data from the models and relevant experimental data. Black line: data from the standard configuration of model in which effects of ISO are incorporated for all of ICaL, If, IKr, IKs, Ist, and Ca2+ transient. Open circles: experimental data of Lei et al. (2000) from rabbit SAN cells. Open right-facing triangle: data of Zaza et al. (1996) from rabbit SAN cells. Open diamonds: data of Choi et al. (1999) from rabbit SAN cells. Open inverted triangles: data of Barbuti et al. (2007) from rabbit SAN cells.
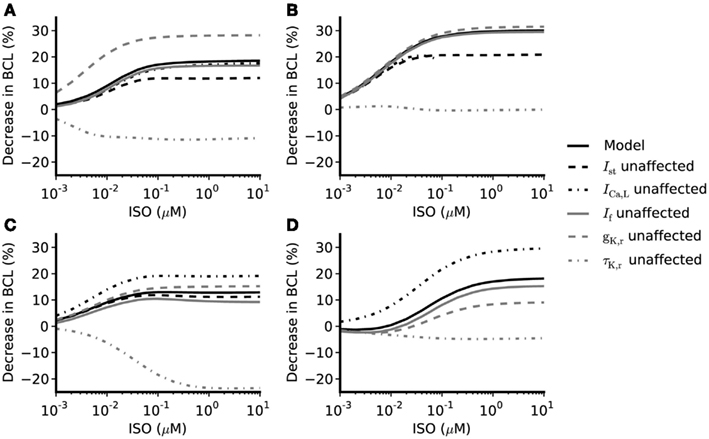
Figure 5. Concentration-dependent percentage decrease of pacemaking cycle length (BCL) by ISO under different simulation conditions. (A,B) Boyett et al. and Kurata et al. central cell models (C,D) Boyett et al. and Kurata et al. peripheral cell models. Black solid line: data from the standard configuration of model in which effects of ISO are incorporated for all of ICaL, If, IKr, IKs, Ist, and Ca2+ transient. Black dash-dotted line: data from model when effects on ICaL omitted. Gray solid line: data from model when effects on If omitted. Gray dashed line: data from model when gKr not affected by ISO. Gray dash-dotted line: data from model when time constant of deactivation of τKr not affected by ISO. Black dashed line: data from model when effects of ISO on Ist omitted.
The Role of ICaL
The role of ICaL in ISO-induced positive chronotropy was investigated by comparing the effect of ISO when its action on ICaL was incorporated into the models with that observed when this action was removed. The concentration-dependence of the change in rate (when ICaL is not affected by ISO while If, IKr, IKs, [Ca]i, and Ist are affected by ISO) is shown in Figure 5 (black dash-dotted line), whilst the standard response [obtained when all actions of ISO were considered (solid black line)] is also shown. In the central cell models, without affecting ICaL, the concentration-dependent change of rate was similar to the standard response. For the peripheral cell models, when ICaL was unaltered by ISO, the concentration-dependent change of rate was found always to be larger than the standard value in the whole range of ISO concentration considered. This implies that the increase of ICaL did not contribute to the ISO increase of the rate under our simulation conditions; indeed, if anything, it decreased rate.
The Role of If
Isoprenaline shifts the voltage dependence of activation curve of If to more positive membrane potentials with a maximal shift of about +15 mV in rabbit SAN cells (Zaza et al., 1996; Accili et al., 1997a,b; and about +7 to +18 mV in murine SAN cells; Alig et al., 2009; Baruscotti et al., 2010; Liao et al., 2010). This shift results in an increase in If over voltages relevant to the pacemaker potential range. Adopting an approach similar to that taken above for ICaL, the role of If in ISO-induced positive chronotropy was investigated by comparing simulations incorporating the effects of ISO on If with those in which this action was not incorporated. The simulated data are shown in Figure 5 (solid gray line) and compared with the standard simulations (solid black line). In both the central and peripheral cell models the concentration-dependent changes of the rate were reduced compared with the standard value, which suggests If contributes to the ISO-induced positive chronotropy.
The computed action potentials and If under different conditions are presented in Figure 6, in order to illustrate more clearly the role of If in the chronotropic effect of ISO. The left-hand panels show the results computed from the Boyett et al. models, whilst the right-hand panels show the results computed from the Kurata et al. models. “control” traces were obtained without simulating effects of ISO, traces labeled “ISO” were obtained from incorporating all actions of 0.05 μM ISO, whilst traces labeled “ISO, no If shift” omit actions on If. In these simulations, ISO accelerated the pacemaking rate by reducing the cycle length of spontaneous activity for both the central (Figures 6A,B) and peripheral (Figures 6C,D) cell models. In the case of “ISO, no If shift,” the pacemaker activities of both models are slowed down, but by less than 3% compared to the “ISO” condition. The positive shift in voltage-dependent activation for If generated a significant increase in the magnitude of If as shown in the lower panels in Figures 6A,B (central models) and Figures 6C,D (peripheral models). However, our simulations indicate that the increase of If was not exclusively the result of this shift, as when it was absent there was still an increase in If, attributable to the increase of the maximal diastolic potential.
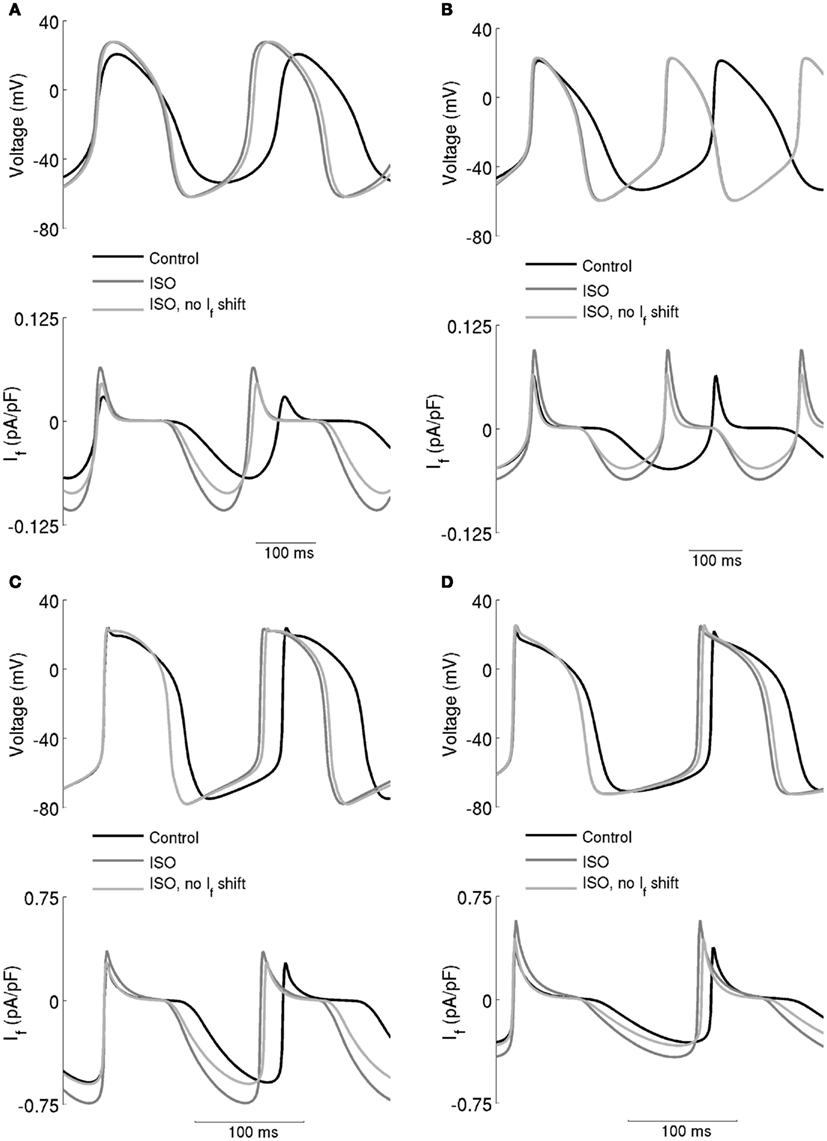
Figure 6. Effect of the ISO-induced increase of If on spontaneous activity. (A) Boyett et al. and (B) Kurata et al. central cell models. (C) Boyett et al. and (D) Kurata et al. cell models. Each panel shows action potentials (upper traces) and records of If (lower traces) under control conditions and in the presence of 0.05 μM ISO. Two simulations with ISO are shown – in one, If was affected and in the other it was not. This shows that the role of If in the chronotropic effect of ISO is small.
The Role of IKr
The role of IKr in the chronotropic effect of ISO was comprised of three components: the increase of gKr, the shift of the IKr activation curve, and the increase of the rate of deactivation of IKr. By adopting a simulation approach, it was possible to investigate in turn the contribution of each of these elements to the action of ISO. The computed concentration-dependent changes in spontaneous rate of the central and peripheral models when the action of ISO on gKr was omitted are shown in Figure 5 (dashed gray line) and compared with the standard ISO simulation (solid black line). In these simulations, the Boyett et al. and the Kurata et al. central cell models responded in a qualitatively similar fashion. In both Boyett et al. and Kurata et al. central SAN models (Figures 5A,B), removing the ISO action on gKr accelerated pacemaking as demonstrated by a greater decrease in pacemaking cycle length as compared to the standard condition. Such an enhanced chronotropic effect without ISO modification of gKr was also observed in the Boyett et al. peripheral cell model (Figure 5C), though data from the Kurata et al. peripheral cell model (Figure 5D) showed a different response. In the Kurata et al. peripheral cell model, removing the ISO action on gKr resulted in a reduced decrease in the pacemaking cycle length as compared to the standard value.
In simulations, we also computed concentration-dependent change in spontaneous rate when the action of ISO on voltage dependence of activation of IKr was omitted, and compared the results with the standard value (data not shown). In both the Boyett et al. and Kurata et al. central cell models, the computed values are larger than the standard values, which suggests that the shift in voltage-dependent activation of IKr does not contribute to ISO-stimulated increase in pacemaking rate (in contrast, it appeared actually to reduce pacemaking rate). Omitting this action of ISO had a negligible effect on the response of the peripheral cell models. Similarly, the concentration-dependent change of the pacemaking rate when the action of ISO on τKr was omitted is also shown in Figure 5 (gray dash-dotted line). In both central and peripheral cell models, removal of the action of ISO on τKr had a dramatic influence of the chronotropic effect of ISO. In the central cell models, when effects on τKr were omitted ISO decreased the pacemaking rate (more so in Boyett et al. model, compared to that of Kurata et al.). Similar effects were seen for the peripheral cell models, for which ISO significantly slowed down the pacemaking rate at high concentrations. The results of these simulations indicate that the effect of ISO on τKr plays an important role in the chronotropic effect of ISO.
The underlying basis for the influence on spontaneous rate of τKr modification by ISO is shown in Figure 7. This shows the action potentials for Boyett et al. central (A) and peripheral (C) cell models (left-hand panels), and the Kurata et al. central cell (B) and peripheral (D) cell models (right-hand panels). The traces labeled “control” were obtained in the absence of ISO, the traces labeled “ISO” were obtained from simulations in which all effects of 0.05 μM ISO were considered. The traces labeled “τKr affected only” were obtained from simulations in which only the effect of 0.05 μM ISO on “τKr” was considered. Also shown in the figure, in the lower panels, are the corresponding IKr records. In the Boyett et al. model simulations, 0.05 μM ISO increased the pacemaking rate by 20 and 15% for the central and peripheral cell models, respectively. In both models, ISO increased the maximal diastolic potential (i.e., become more negative; see Table 1). However, when only τKr was affected, ISO increased the rate by 33 and 10% for the central and peripheral cell models, respectively, each with an elevated maximal diastolic potential (see Table 1). Compared with the “control” condition, in both central and peripheral cell models ISO increased IKr during the initial repolarization phase, but reduced IKr in later repolarization and the depolarization phase. These changes in IKr result from altered τKr. When τKr only was affected, an increase of IKr in the early repolarization phase was observed, whilst a decrease of IKr in late repolarization period and subsequent depolarization phase was seen. The decreased IKr during in the depolarization phase contributed significantly to the increase of the pacemaking rate. Similar observations were seen with the Kurata et al. models (Table 1). 0.05 μM ISO increased the pacemaking rate by 25% for the central cell model and 7% for the peripheral cell model. When τKr only was affected, ISO increased the rate by 20% for the central cell model and by 9% for the peripheral cell model.
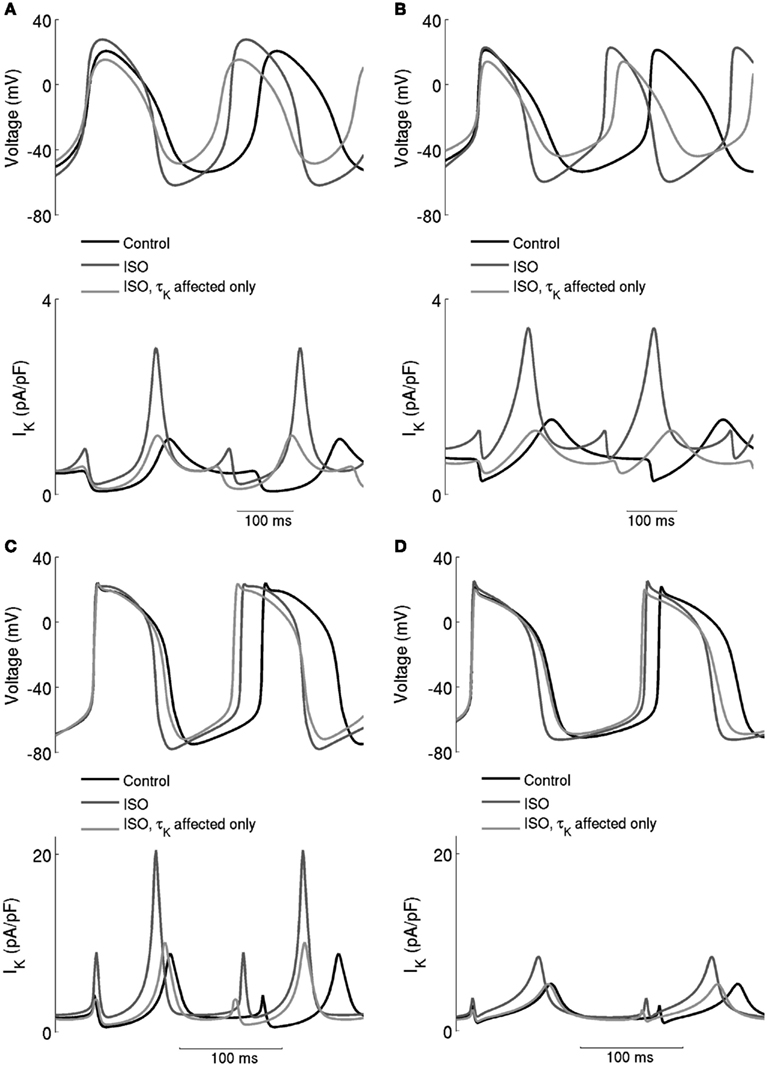
Figure 7. Effects of ISO-induced increase of the time constant of deactivation of IKr. Spontaneous activity in the central (A) Boyett et al. (B) Kurata et al. cell models and peripheral (C) Boyett et al. (D) Kurata et al. cell models. Each panel shows action potentials and records of IKr under control conditions and in the presence of 0.05 μM ISO. Two simulation conditions with ISO are shown – in one, only τKr was affected and in the other modulatory effects on currents were incorporated. When only τK was affected, there was a substantial increase of the firing rate, but there was also a large decrease of the maximum diastolic potential, which is not observed experimentally.

Table 1. Effects of ISO and ISO-induced change in the deactivation rate of IKr on the characteristics of pacemaking action potentials.
In simulations, we also computed concentration-dependent change in spontaneous rate when the action of ISO on increasing gKs was omitted. As compared to the results obtained in the standard condition, omitting ISO action on gKs produced negligible effect on the pacemaking rate (data not shown).
In a previous experimental study, Lei et al. (2002) have shown that blocking IKs produced a negligible effect on spontaneous rate of rabbit SAN cells under control condition. However, with application of 10 nM ISO, blocking IKs produced ~10% prolongation of pacemaking cycle length. This implies that IKs plays a more important role in generating SAN pacemaking with ISO present than in its absence (Lei et al., 2002). This experimental observation on the role of IKs in the pacemaking potentials of rabbit SAN cells was partially reproduced by the model. In simulations, blocking IKs in the control condition produced negligible alteration to the pacemaking cycle length in both of the Boyett et al. central and peripheral cell models. With 10 nM ISO, blocking IKs produced an increase of ~3 and 2% in the pacemaking cycle length for the central and peripheral cell model, respectively. These simulation results are qualitatively similar to the experimental observations of Lei et al. (2002). Quantitative differences between the model simulation and the experimental data of Lei et al. (2002) on the role of IKs in SAN cell activity under control and ISO conditions may be due to the intrinsic limitations of the model as discussed in detail in Zhang et al. (2000), or due to limitations in simulating ISO (see Discussion).
The Role of Ist
Similar to other currents investigated, the role of Ist in the positive chronotropic effect of ISO was investigated by comparing simulations of the effect of ISO that incorporated its effects on Ist with those in which this action was omitted. The computed data are shown in Figure 5 (black dashed line) and can be compared with the standard response incorporating all effects of ISO (solid black line). By removing the action of ISO on Ist, the computed dose-dependent changes in the Boyett et al. central cell model were only slightly reduced compared with the standard values. In the Boyett et al. peripheral cell model, the computed concentration-dependent changes of rate were also close to the standard values. These results suggest that Ist does not play an important role in ISO-induced positive chronotropy under our simulation conditions. As there is no Ist in the Kurata et al. cell models, the role of augmented Ist in the positive chronotropic effect of ISO was not analyzed using the Kurata et al. cell models.
The Role of Ca2+ Handling and Na+-Ca2+ Exchange
Experimental studies have shown that that ISO increases the systolic and diastolic levels of intracellular Ca2+ ([Ca2+]i) (Ju and Allen, 1999; Vinogradova et al., 2002, 2008). Here we considered how ISO-induced changes in INaCa consequent upon ISO-induced changes in [Ca2+]i contribute to the positive chronotropic action of ISO, when effects on other currents are excluded. The results from Boyett et al. models are shown in Figure 8. In the figure the time traces of action potentials (Figures 8Ai,Bi), [Ca2+]i (Figures 8Aii,Bii), and INaCa (Figures 8Aiii,Biii) were superimposed in different conditions. The traces labeled “control” were obtained in the absence of ISO, the traces labeled “ISO” were obtained from simulations in which the ISO-induced changes in systolic and diastolic [Ca2+]i alone were considered. In both the central and peripheral cell models, the systolic and diastolic levels of [Ca2+]i were doubled by ISO. In simulations using the Boyett et al. models, changes in [Ca2+]i increased the rate by 10% for the central model, and 1% for the peripheral cell model. The accelerated pacemaking rate was attributable to an increased INaCa during the diastolic pacemaking phase (Figures 8Aiii,Biii) that arose from an elevated diastolic [Ca2+]i level due to an integral action of an increased the SR Ca2+ release and reduced SR Ca2+ uptake. Further simulations were also performed to investigate the individual role of an increased SR Ca2+ release and a reduced SR Ca2+ uptake. By either increasing the SR Ca2+ release alone or reducing the SR Ca2+ uptake alone, the diastolic [Ca2+]i level was elevated, resulting in an increased INaCa leading to accelerated pacemaking rates. Results from Kurata et al. cell models were qualitatively similar to the results from the Boyett et al. models.
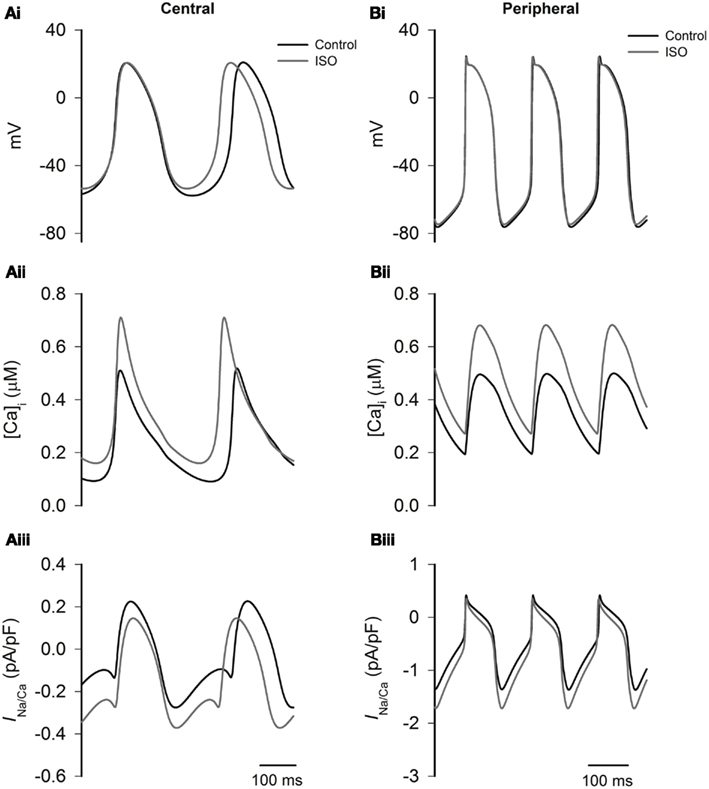
Figure 8. Effects of ISO-induced changes of the Ca2+ transient on spontaneous activity. Left panels show central cell models, whilst right panels show peripheral cell models (Boyett et al. models) for action potentials (Ai,Bi), intracellular Ca2+ transients (Aii,Bii), and INaCa(Aiii,Biii) under control conditions and in the presence of ISO. In ISO simulations, all effects except modulation of the intracellular Ca2+ transient are omitted. The simulations show that the increase in the Ca2+ transient plays an important role in response of the central cell model to ISO.
Discussion
In this study, we have incorporated a set of equations of the known actions of ISO on ICaL, If, IKr and IKs, Ist, and intracellular Ca2+ handling into two established action potential models of central and peripheral rabbit SAN cells to simulate the chronotropic effect of ISO. Use of these models has enabled us to manipulate the actions of ISO and dissect out the relative contributions of different ionic currents to the modulatory actions of β-adrenergic stimulation.
The simulated effect of ISO on the spontaneous activity in the SAN cell models studied here is very similar to that seen experimentally in rabbit SAN cells (Zaza et al., 1996; Lei et al., 2000, 2002). ISO accelerated the pacemaking rate and affected the shape of action potentials in both models used here. The computed concentration-dependent increases of the rate are comparable to experimental data obtained from rabbit SAN (Zaza et al., 1996; Lei et al., 2000). With both Boyett et al. (2001) and Kurata et al. (2008) models, ISO increases the overshoot and maximal diastolic potential, and shortens the duration of action potentials. These changes in action potential shape are consistent with experimental observations (Zaza et al., 1996; Lei et al., 2000).
In our simulations, we were able to show that an increase in ICaL contributes to the increase of the overshoot of action potential with ISO, however it contributes very little to the acceleration of firing rate. In the peripheral cell model, when the action of increasing ICaL alone is considered, the firing rate was actually slowed down. Consistent with this, blocking ICaL by nifedipine has been found to increase the pacemaking rate experimentally in small balls cut from the periphery of the rabbit SAN (Kodama et al., 1997). Presumably, the underlying mechanism is related to the effect of increased ICaL on action potential duration, whereby an increase of ICaL leads to an increase in the time interval between two successive action potentials.
The role of If in the chronotropic effect of ISO has been controversial. If has been considered by some as the main pacemaking current in rabbit SAN (DiFrancesco, 1995, 2010; DiFrancesco and Borer, 2007) and increased If has been considered as the main ionic basis of the chronotropic effect of ISO (Zaza et al., 1996; Liao et al., 2010). However, the role of If in β-adrenoceptor mediated chronotropy in the SAN is not unequivocal: Cai et al. (1995) have shown that the positive chronotropic effect of ISO was not significantly affected when If is blocked by Cs+. Also, in the amphibian sinus venosus, in which there is no If, ISO still accelerated the firing rate (Ju and Allen, 1999), whilst mice lacking the predominant If channel gene (HCN4) exhibit physiological heart rate responses to isoproterenol (Herrmann et al., 2007). In our simulations, we have observed that If does contribute to the chronotropic effect of ISO but that its contribution is small, as removal of the action of ISO on If produces a small alteration to the simulated concentration-response relation for the rate increase.
The role of Ist in the chronotropy was also considered. In both models removal of the action of ISO on Ist does not affect the dose-dependent increase of rate significantly. In simulations, removal of the action of ISO had a relatively larger effect on the chronotropy in the central cell model than in the peripheral cell model. This is because in the development of the models, due to the absence of experimental data about the regional difference of Ist in rabbit SAN, the same magnitude (current density) of Ist was assumed for both central and peripheral cell models. Consequently the central cell models were relatively more sensitive to Ist, due to relatively smaller current densities of other depolarizing channel currents, such as If, INa, and ICaT, when compared to peripheral cell models (Zhang et al., 2000).
In this study, we have shown that an increased [Ca2+]i level in the simulated presence of ISO contributed to the increase in rate by reducing the pacemaking cycle length in the central cell model. This observation is qualitatively similar to the results of Maltsev and Lakatta (2009), which provide evidence that the “Ca2+ clock” plays an important role in generating sinoatrial nodal pacemaking. Quantitative differences between our results and those of Maltsev and Lakatta (2009) on the “Ca2+ clock” contribution to pacemaking are likely to reflect differences in the paramaterization of the various currents involved in the membrane clock between the Boyett et al. (2001) model and the Maltsev and Lakatta (2009) model. The parameters for ionic channel currents in the Boyett et al. (2001) are inherited from the Zhang et al. (2000) cell models, which were based on and validated against experimental data (Zhang et al., 2000). The difference in the response to raised [Ca2+]i between the central and peripheral Boyett et al. (2001) models also reflected the experimentally observed intrinsic difference in the current densities of some depolarizing currents between two different cell types, as summarized in Boyett et al. (2000) and Zhang et al. (2000).
The chronotropic effect of ISO reflects the combined actions of ISO on ionic currents ICaL, If, IKr, IKs, and Ist, among which action on IKr plays a relatively more important role. Removal of the action of ISO on the rate of deactivation of IKr reduced the chronotropic effect of ISO significantly in both Boyett et al. (2001) and Kurata et al. (2008) models, and even reverses the chrontropy of ISO with high concentration in the peripheral model. An increase in the rate of deactivation of IKr increased the magnitude of IKr in the early action potential repolarization phase, but decreased the magnitude of IKr in the late repolarization phase and the depolarization phase. A decrease in outward IKr in the depolarization period enables the net inward current to be relatively larger, which speeds up the depolarization and thus the pacemaking rate.
The important contribution of IKr to cardiac pacemaking activities has been noted in previous experimental studies. In their work Sato et al. (2000) showed that blocking IKr partially by ibutilide (though ibutilide is not a completely selective IKr channel blocker) slowed down rabbit SAN pacemaking rate modestly, but blocking IKr completely at a higher concentration abolished its pacemaking action potentials. Ono and Ito (1995) also noted the important role of E−4031 sensitive IKr in rabbit SAN cardiac pacemaking. Further studies demonstrated that partial block of IKr (around 50%) by E−4031 almost abolished spontaneous pacemaking activity in central rabbit SAN tissue, though the pacemaking activity in peripheral SAN tissue persisted (Kodama et al., 1999). Data from mouse SAN cells also showed an important contribution of IKr to cardiac pacemaking action potentials as block IKr by E−4031 prolonged the pacemaking cycle length by about 68% (Nikmaram et al., 2008). Our simulation data added to these experimental data in showing an important role of IKr in SAN normal pacemaking, especially in elucidating the role of altered deactivation rate of IKr in cardiac pacemaking action potentials, which it is not easily possible to get from a pharmacological study.
Assumptions, Limitations, and Conclusion
In the present study, due to the lack of available experimental data, we assumed that the concentration-dependent action of ISO on the current amplitude of sinoatrial IKs and Ist was the same as that of IKr. This assumption requires to be re-evaluated when more experimental data become available. In addition, where data were not available from rabbit SAN cells, data from other species were used (e.g., some equation parameters for Ist were based on multiple data sources from guinea-pig and rat SAN cells (Guo et al., 1995, 1997; Shinagawa and Noma, 2000; Toyoda et al., 2005). Intracellular Ca2+ cycling was found to modulate pacemaking rate in the present model, but not as prominently as in a prior simulation study of Maltsev and Lakatta (2009). As the role of the Ca2+ clock in SAN pacemaking is debated (e.g., Honjo et al., 2003; Lancaster et al., 2004; DiFrancesco and Borer, 2007; Herrmann et al., 2007; Joung et al., 2009; DiFrancesco, 2010; Gao et al., 2010; Himeno et al., 2011; Sosunov and Anyukhovsky, 2012), further experimental quantification of SAN cellular intracellular Ca2+ handling mechanisms and their contribution to electrogenesis would be useful. Also due to the limited availability of experimental data on rabbit SAN cells, simulations of concentration-dependent ISO action on If were based on the experimental data of Zaza et al. (1996), which showed a maximal shift of the steady-state activation curve by +9.62 mV. Though this is relatively smaller as compared to the ISO-induced shift (about +7 to +18 mV) of the If activation curve in murine SAN cells (Alig et al., 2009; Baruscotti et al., 2010; Liao et al., 2010), it is close to observed values of 8.8 mV (Accili et al., 1997a) and 5.3 mV (Accili et al., 1997b) for a 1 μM ISO-induced shift in voltage-dependent activation of If in adult rabbit SAN cells. As there are no experimental data to show other ISO-induced changes in the kinetics of If, in simulations, the slope of the steady-state activation curve and the voltage-dependent time constant of activation process were assumed to be unchanged in the ISO condition.
Whilst it is important that potential limitations of the models used in this investigation are made explicit, it is still likely that the findings of our study are valid in showing that the chronotropic effect of ISO involves an integrated action of ISO on ICaL If, Ist, IKr, IKs, and [Ca2+]i) in order to match prior experimental data of Zaza et al. (1996) and Lei et al. (2000). Our study is significant in highlighting the relative contributions of If, Ca2+ handling, and modulation of IKr deactivation kinetics to the overall response to ISO.
Conflict of Interest Statement
The authors declare that the research was conducted in the absence of any commercial or financial relationships that could be construed as a potential conflict of interest.
Acknowledgments
This work was supported by projects grants from Engineering and Physical Science Research Council UK (EP/J00958X/1; EP/I029826/1), the British Heart Foundation (FS/08/021), and the Natural Science Foundation of China (61179009).
References
Abramochkin, D. V., Kuzmin, V. S., Sukhova, G. S., and Rosenshtraukh, L. V. (2009). Modulation of rabbit sinoatrial node activation sequence by acetylcholine and isoproterenol investigated with optical mapping technique. Acta Physiol. (Oxf.) 196, 385–394.
Accili, E. A., Redaelli, G., and DiFrancesco, D. (1997a). Differential control of the hyperpolarization-activated current (i(f)) by cAMP gating and phosphatase inhibition in rabbit sino-atrial node myocytes. J. Physiol. (Lond.) 500(Pt 3), 643–651.
Accili, E. A., Robinson, R. B., and DiFrancesco, D. (1997b). Properties and modulation of If in newborn versus adult cardiac SA node. Am. J. Physiol. 272(3 Pt 2), H1549–H1552.
Alig, J., Marger, L., Mesirca, P., Ehmke, H., Mangoni, M. E., and Isbrandt, D. (2009). Control of heart rate by cAMP sensitivity of HCN channels. Proc. Natl. Acad. Sci. U.S.A. 106, 12189–12194.
Barbuti, A., Terragni, B., Brioschi, C., and DiFrancesco, D. (2007). Localization of f-channels to caveolae mediates specific beta2-adrenergic receptor modulation of rate in sinoatrial myocytes. J. Mol. Cell. Cardiol. 42, 71–78.
Baruscotti, M., Bucchi, A., Viscomi, C., Mandelli, G., Consalez, G., Gnecchi-Rusconi, T., Montano, N., Casali, K. R., Micheloni, S., Barbuti, A., and DiFrancesco, D. (2010). Deep bradycardia and heart block caused by inducible cardiac-specific knockout of the pacemaker channel gene Hcn4. Proc. Natl. Acad. Sci. U.S.A. 108, 1705–1710.
Boyett, M. R., Honjo, H., and Kodama, I. (2000). The sinoatrial node, a heterogeneous pacemaker structure. Cardiovasc. Res. 47, 658–687.
Boyett, M. R., Zhang, H., Garny, A., and Holden, A. V. (2001). Control of the pacemaker activity by the intracellular Ca2+: experiments and simulation. Philos. Trans. R. Soc. Lond. B Biol. Sci. 359, 1091–1110.
Brown, H. F., DiFrancesco, D., and Noble, S. J. (1979). How does adrenaline accelerate the heart? Nature 280, 235–236.
Bucchi, A., Baruscotti, M., Robinson, R. B., and DiFrancesco, D. (2003). I(f)-dependent modulation of pacemaker rate mediated by cAMP in the presence of ryanodine in rabbit sino-atrial node cells. J. Mol. Cell. Cardiol. 35, 905–913.
Cai, Q., Lei, M., and Brown, H. F. (1995). Responses of guinea-pig SA node/atria to acetylcholine and adrenaline in the presence of blockers of if and iK,ACh. J. Physiol. (Lond.) 483, 21.
Choi, H. S., Wang, D. Y., Noble, D., and Lee, C. O. (1999). Effect of isoprenaline, carbachol, and Cs+ on Na+ activity and pacemaker potential in rabbit SA node cells. Am. J. Physiol. 276(1 Pt 2), H205–H214.
DiFrancesco, D. (1995). The onset and autonomic regulation of cardiac pacemaker activity: relevance of the f current. Cardiovasc. Res. 29, 449–456.
DiFrancesco, D. (2010). The role of the funny current in pacemaker activity. Circ. Res. 106, 434–446.
DiFrancesco, D., and Borer, J. S. (2007). The funny current: cellular basis for the control of heart rate. Drugs 67(Suppl. 2), 15–24.
Duchatelle-Gourdon, I., Hartzell, H. C., and Lagrutta, A. A. (1989). Modulation of the delayed rectifier potassium current in frog cardiomyocytes by beta-adrenergic agonists and magnesium. J. Physiol. (Lond.) 415, 251–274.
Freeman, L. C., and Kass, R. S. (1993). Delayed rectifier potassium channels in ventricle and sinoatrial node of the guinea pig: molecular and regulatory properties. Cardiovasc. Drugs Ther. 7(Suppl. 3), 627–635.
Gao, Z., Chen, B., Joiner, M. L., Wu, Y., Guan, X., Koval, O. M., Chaudhary, A. K., Cunha, S. R., Mohler, P. J., Martins, J. B., Song, L. S., and Anderson, M. E. (2010). I(f) and SR Ca(2+) release both contribute to pacemaker activity in canine sinoatrial node cells. J. Mol. Cell. Cardiol. 49, 33–40.
Giles, W., Nakajima, T., Ono, K., and Shibata, E. F. (1989). Modulation of the delayed rectifier K+ current by isoprenaline in bull-frog atrial myocytes. J. Physiol. (Lond.) 415, 233–249.
Guo, J., Mitsuiye, T., and Noma, A. (1997). The sustained inward current in sino-atrial node cells of guinea-pig heart. Pflugers Arch. 433, 390–396.
Guo, J., Ono, K., and Noma, A. (1995). A sustained inward current activated at the diastolic potential range in rabbit sino-atrial node cells. J. Physiol. (Lond.) 483(Pt 1), 1–13.
Habuchi, Y., Han, X., and Giles, W. R. (1995). Comparison of the hyperpolarisation-activated and delayed rectifier currents in rabbit atrioventricular node and sinoatrial node. Heart Vessels Suppl. 9, 203–206.
Hagiwara, N. I. H. (1989). Modulation by intracellular Ca2+ of the hyperpolarisation-activated inward currents in rabbit single sino-atrial node cells. J. Physiol. (Lond.) 409, 121–141.
Herrmann, S., Stieber, J., Stockl, G., Hofmann, F., and Ludwig, A. (2007). HCN4 provides a “depolarization reserve” and is not required for heart rate acceleration in mice. EMBO J. 26, 4423–4432.
Himeno, Y., Toyoda, F., Satoh, H., Amano, A., Cha, C. Y., Matsuura, H., and Noma, A. (2011). Minor contribution of cytosolic Ca2+ transients to the pacemaker rhythm in guinea pig sinoatrial node cells. Am. J. Physiol. Heart Circ. Physiol. 300, H251–H261.
Honjo, H., Inada, S., Lancaster, M. K., Yamamoto, M., Niwa, R., Jones, S. A., Shibata, N., Mitsui, K., Horiuchi, T., Kamiya, K., Kodama, I., and Boyett, M. R. (2003). Sarcoplasmic reticulum Ca2+ release is not a dominating factor in sinoatrial node pacemaker activity. Circ. Res. 92, e41–e44.
Huser, J., Blatter, L. A., and Lipsius, S. L. (2000). Intracellular Ca2+ release contributes to automaticity in cat atrial pacemaker cells. J. Physiol. (Lond.) 524(Pt 2), 415–422.
Ito, H., and Ono, K. (1995). A rapidly activating delayed rectifier K+ channel in rabbit sinoatrial node cells. Am. J. Physiol. 269(2 Pt 2), H443–H452.
Joung, B., Tang, L., Maruyama, M., Han, S., Chen, Z., Stucky, M., Jones, L. R., Fishbein, M. C., Weiss, J. N., Chen, P. S., and Lin, S. F. (2009). Intracellular calcium dynamics and acceleration of sinus rhythm by beta-adrenergic stimulation. Circulation 119, 788–796.
Ju, Y. K., and Allen, D. G. (1999). How does beta-adrenergic stimulation increase the heart rate? The role of intracellular Ca2+ release in amphibian pacemaker cells. J. Physiol. (Lond.) 516(Pt 3), 793–804.
Ke, Y., Lei, M., Collins, T. P., Rakovic, S., Mattick, P. A., Yamasaki, M., Brodie, M. S., Terrar, D. A., and Solaro, R. J. (2007). Regulation of L-type calcium channel and delayed rectifier potassium channel activity by p21-activated kinase-1 in guinea pig sinoatrial node pacemaker cells. Circ. Res. 100, 1317–1327.
Kharche, S., Yu, J., Lei, M., and Zhang, H. (2011). A mathematical model of action potentials of mouse sinoatrial node cells with molecular bases. Am. J. Physiol. Heart Circ. Physiol. 301, H945–H963.
Kodama, I., Boyett, M. R., Nikmaram, M. R., Yamamoto, M., Honjo, H., and Niwa, R. (1999). Regional differences in effects of E-4031 within the sinoatrial node. Am. J. Physiol. 276, H793–H802.
Kodama, I., Nikmaram, M. R., Boyett, M. R., Suzuki, R., Honjo, H., and Owen, J. M. (1997). Regional differences in the role of the Ca2+ and Na+ currents in pacemaker activity in the sinoatrial node. Am. J. Physiol. 272(6 Pt 2), H2793–H2806.
Kurata, Y., Matsuda, H., Hisatome, I., and Shibamoto, T. (2008). Regional difference in dynamical property of sinoatrial node pacemaking: role of Na+ channel current. Biophys. J. 95, 951–977.
Lancaster, M. K., Jones, S. A., Harrison, S. M., and Boyett, M. R. (2004). Intracellular Ca2+ and pacemaking within the rabbit sinoatrial node: heterogeneity of role and control. J. Physiol. (Lond.) 556(Pt 2), 481–494.
Lei, M., and Brown, H. F. (1996). Two components of the delayed rectifier potassium current, IK, in rabbit sino-atrial node cells. Exp. Physiol. 81, 725–741.
Lei, M., Brown, H. F., and Terrar, D. A. (2000). Modulation of delayed rectifier potassium current, iK, by isoprenaline in rabbit isolated pacemaker cells. Exp. Physiol. 85, 27–35.
Lei, M., Cooper, P. J., Camellitti, P., and Kohl, P. (2002). Role of the 293b-sensitive, slowly activating rectifier potassium current, i(Ks), in pacemaker activity of rabbit isolated sinoatrial node cells. Cardiovasc. Res. 53, 68–79.
Liao, Z., Lockhead, D., Larson, E. D., and Proenza, C. (2010). Phosphorylation and modulation of hyperpolarization-activated HCN4 channels by protein kinase A in the mouse sinoatrial node. J. Gen. Physiol. 136, 247–258.
Maltsev, V. A., and Lakatta, E. G. (2009). Synergism of coupled subsarcolemmal Ca2+ clocks and sarcolemmal voltage clocks confers robust and flexible pacemaker function in a novel pacemaker cell model. Am. J. Physiol. Heart Circ. Physiol. 296, H594–H615.
Nikmaram, M. R., Liu, J., Abdelrahman, M., Dobrzynski, H., Boyett, M. R., and Lei, M. (2008). Characterization of the effects of ryanodine, TTX, E-4031 and 4-AP on the sinoatrial and atrioventricular nodes. Prog. Biophys. Mol. Biol. 96, 452–464.
Noma, A., Kotake, H., and Irisawa, H. (1980). Slow inward current and its role mediating the chronotropic effect of epinephrine in the rabbit sinoatrial node. Pflugers Arch. 388, 1–9.
Ono, K., and Ito, H. (1995). Role of rapidly activating delayed rectifier K+ current in sinoatrial node pacemaker activity. Am. J. Physiol. 269, H453–H62.
Sato, N., Tanaka, H., Habuchi, Y., and Giles, W. R. (2000). Electrophysiological effects of ibutilide on the delayed rectifier K(+) current in rabbit sinoatrial and atrioventricular node cells. Eur. J. Pharmacol. 22, 281–288.
Shannon, T. R., Wang, F., and Bers, D. M. (2005). Regulation of cardiac sarcoplasmic reticulum Ca release by luminal [Ca] and altered gating assessed with a mathematical model. Biophys. J. 89, 4096–4110.
Shannon, T. R., Wang, F., Puglisi, J., Weber, C., and Bers, D. M. (2004). A mathematical treatment of integrated Ca dynamics within the ventricular myocyte. Biophys. J. 87, 3351–3371.
Shinagawa, Y. S. H., and Noma, A. (2000). The sustained inward current and inward rectifier K+ current in pacemaker cells dissociated from rat sinoatrial. J. Physiol. (Lond.) 523, 593–605.
Sosunov, E. A., and Anyukhovsky, E. P. (2012). Differential effects of ivabradine and ryanodine on pacemaker activity in canine sinus node and Purkinje fibers. J. Cardiovasc. Electrophysiol. (in press). doi: 10.1111/j.1540-8167.2011.02285.x
Toyoda, F., Ding, W. G., and Matsuura, H. (2005). Responses of the sustained inward current to autonomic agonists in guinea-pig sino-atrial node pacemaker cells. Br. J. Pharmacol. 144, 660–668.
Vinogradova, T. M., Bogdanov, K. Y., and Lakatta, E. G. (2002). beta-Adrenergic stimulation modulates ryanodine receptor Ca(2+) release during diastolic depolarization to accelerate pacemaker activity in rabbit sinoatrial nodal cells. Circ. Res. 90, 73–79.
Vinogradova, T. M., Sirenko, S., Lyashkov, A. E., Younes, A., Li, Y., Zhu, W., Yang, D., Ruknudin, A. M., Spurgeon, H., and Lakatta, E. G. (2008). Constitutive phosphodiesterase activity restricts spontaneous beating rate of cardiac pacemaker cells by suppressing local Ca2+ releases. Circ. Res. 102, 761–769.
Walsh, K. B., Begenisich, T. B., and Kass, R. S. (1988). Beta-adrenergic modulation in the heart. Independent regulation of K and Ca channels. Pflugers Arch. 411, 232–234.
Wu, Y., Gao, Z., Chen, B., Koval, O. M., Singh, M. V., Guan, X., Hund, T. J., Kutschke, W., Sarma, S., Grumbach, I. M., Wehrens, X. H., Mohler, P. J., Song, L. S., and Anderson, M. E. (2009). Calmodulin kinase II is required for fight or flight sinoatrial node physiology. Proc. Natl. Acad. Sci. U.S.A. 106, 5972–5977.
Yazawa, K., and Kameyama, M. (1990). Mechanism of receptor-mediated modulation of the delayed outward potassium current in guinea-pig ventricular myocytes. J. Physiol. (Lond.) 421, 135–150.
Zaza, A., Robinson, R. B., and DiFrancesco, D. (1996). Basal responses of the L-type Ca2+ and hyperpolarization-activated currents to autonomic agonists in the rabbit sino-atrial node. J. Physiol. (Lond.) 491(Pt 2), 347–355.
Keywords: sinoatrial node, isoprenaline, action potential
Citation: Zhang H, Butters T, Adeniran I, Higham J, Holden AV, Boyett MR and Hancox JC (2012) Modeling the chronotropic effect of isoprenaline on rabbit sinoatrial node. Front. Physio. 3:241. doi: 10.3389/fphys.2012.00241
Received: 30 March 2012; Accepted: 13 June 2012;
Published online: 09 July 2012.
Edited by:
Peter Taggart, University College London, UKReviewed by:
Christian Zemlin, SUNY Upstate Medical University, USAThomas Hund, Ohio State University, USA
Copyright: © 2012 Zhang, Butters, Adeniran, Higham, Holden, Boyett and Hancox. This is an open-access article distributed under the terms of the Creative Commons Attribution License, which permits use, distribution and reproduction in other forums, provided the original authors and source are credited and subject to any copyright notices concerning any third-party graphics etc.
*Correspondence: Henggui Zhang, Biological Physics Group, School of Physics and Astronomy, University of Manchester, Manchester M13 9PL, UK. e-mail: henggui.zhang@manchester.ac.uk