- Department of Engineering and Sustainable Development, and Swedish Winter Sports Research Centre, Mid Sweden University, Östersund, Sweden
Humans share with seals the ability to contract the spleen and increase circulating hematocrit, which may improve apneic performance by enhancing gas storage. Seals have large spleens and while human spleen size is small in comparison, it shows great individual variation. Unlike many marine mammals, human divers rely to a great extent on lung oxygen stores, but the impact of lung volume on competitive apnea performance has never been determined. We studied if spleen- and lung size correlated with performance in elite apnea divers. Volunteers were 14 male apnea world championship participants, with a mean (SE) of 5.8 (1.2) years of previous apnea training. Spleen volume was calculated from spleen length, width, and thickness measured via ultrasound during rest, and vital capacity via spirometry. Accumulated competition scores from dives of maximal depth, time, and distance were compared to anthropometric measurements and training data. Mean (SE) diving performance was 75 (4) m for constant weight depth, 5 min 53 (39) s for static apnea and 139 (13) m for dynamic apnea distance. Subjects’ mean height was 184 (2) cm, weight 82 (3) kg, vital capacity (VC) 7.3 (0.3) L and spleen volume 336 (32) mL. Spleen volume did not correlate with subject height or weight, but was positively correlated with competition score (r = 0.57; P < 0.05). Total competition score was also positively correlated with VC (r = 0.54; P < 0.05). The three highest scoring divers had the greatest spleen volumes, averaging 538 (53) mL, while the three lowest-scoring divers had a volume of 270 (71) mL (P < 0.01). VC was also greater in the high-scorers, at 7.9 (0.36) L as compared to 6.7 (0.19) L in the low scorers (P < 0.01). Spleen volume was reduced to half after 2 min of apnea in the highest scoring divers, and the estimated resting apnea time gain from the difference between high and low scorers was 15 s for spleen volume and 60 s for VC. We conclude that both spleen- and lung volume predict apnea performance in elite divers.
Introduction
Humans have the ability to contract the spleen during diving and increase circulating hematocrit (Hurford et al., 1990) which seems to prolong apneas when performed in series (Schagatay et al., 2001). This response is well known in seals, where spleen size varies greatly between species and in some deep divers like the Weddell seal it is exceptionally large with the ability to store 2/3 of the body’s erythrocytes during rest (Qvist et al., 1986). In highly aerobic species like the horse and the dog, the spleen contracts and elevates hematocrit during exercise (Barcroft and Stevens, 1927). The human spleen also contracts, increasing circulating Hb and Hct during both apnea (Schagatay et al., 2001) and exercise (Laub et al., 1993; Stewart and McKenzie, 2002), which will increase blood gas storage capacity. The spleen contraction is probably active, i.e., not due to reduced blood flow to the organ (Bakovic et al., 2003) and the reversible elevations of Hct and Hb are not due to hemoconcentration by extravasation of plasma (Schagatay et al., 2001). Spleen size shows great individual variation in humans (Prassopoulos et al., 1997), and also its ability to contract appears to vary between individuals (Schagatay et al., 2005) but it has never been determined whether these factors correlate with individual apneic diving ability.
The sport Apnea has developed during the past 15 years, and involves disciplines with the aim to reach the greatest possible depth, immersion time and horizontal distance under water, and serves as a good model for studying maximal apneic diving performance in humans. The ability to perform extended apnea depends mainly on three factors: (1) the total body gas storage capacity in lungs, blood, and tissues; (2) asphyxia tolerance, or the tolerable levels of hypoxia and hypercapnia as determined by, e.g., brain hypoxia tolerance and the CO2 buffering capacity; and (3) metabolic rate, which is determined mainly by work economy and the ability to restrict metabolism via the diving response. A large spleen with the ability to eject a great quantity of stored erythrocytes into circulation would be beneficial for apneic duration both by increasing blood oxygen storage and enhancing buffering capacity, and an important part of oxygen storage in humans is the lung supply.
A way to increase gas storage capacity would be to maximize lung volume, thereby increasing oxygen stores and also allowing more CO2 to be transferred to the lungs during apnea via dilution effect. In addition, large lungs with small residual volume (RV) also help the diver to attain greater depth without risking “squeeze,” as the depth at which compression to RV is reached would be greater. Increased lung volume furthermore facilitates equalization of middle ears and sinuses. Breath-hold divers are known to overfill their lungs before diving via buccal pumping or “lung packing” (Örnhagen et al., 1998), i.e., by pumping air into the lungs via a swallowing motion. However, there are also negative effects of large lung volume including increased buoyancy as well as a high intra-thoracic pressure, with a negative effect on venous return and increased risk of syncope (Andersson et al., 1998). The diving response, known to limit metabolism during diving, may also be decreased by a large inspired lung volume (Andersson and Schagatay, 1998a,b), and over filling of the lungs could, by counteracting the diving response, elevate metabolism during diving, at least while the diver is not at depth. Trained apnea divers have previously been reported to have large lungs (Carey et al., 1956) suggesting that the positive effects may outweigh the disadvantages. However, the lungs of typical marine mammals are no larger than those of terrestrial, and, e.g., the deep diving Weddell seal exhales before diving in order to avoid excess nitrogen loading, and relies mainly on blood- and tissue (mainly muscle myoglobin) oxygen storage during diving (Butler, 2001). Conversely, competitive apneists are known to train to increase lung volume, by stretching and lung packing maneuvers (Örnhagen et al., 1998), but to our knowledge no systematic study of the effect of lung volume on competitive apnea diving performance in elite apneists has been performed.
We therefore aimed to compare spleen and lung volume, two possible dive-prolonging characteristics, to the individual scores in an apnea world championship competition. We hypothesized that scores in the competition would be positively correlated with both spleen and lung volume.
Materials and Methods
Participants and Setting
This study complied with the Declaration of Helsinki and the methods used had been approved by the local human ethics board. Information about the study was posted in the competition area of the championship. Fourteen male subjects from 10 different nations volunteered for the study and, after receiving more detailed information about the study, signed an informed consent form. Their mean (SE) age was 29 (2) years, and several were individual world record holders. The participants had an average of 5.8 (1.2) years of experience in apnea training. Their total apnea training load averaged 6.2 (0.6) h/week and other physical training 6.3 (0.7) h/week in the month leading up to the competition. Apnea training varied between individuals, but always included serial and maximal static dry and immersed apneas, walking apnea and dynamic apneas in a pool, and, for the weeks leading up to the competition, dynamic deep diving on a line in the sea or a lake. Physical training mainly included endurance training like swimming, running and cycling, which some divers combined with strength training of major swimming muscles. Anthropometric and training data is presented in Table 1.
The apnea competition involved accumulation of points from dives of maximal depth, time and horizontal distance for a total score (Table 2). In competitive apnea, points are gained in direct relation to depth, time, and distance in the respective disciplines, producing an overall score that renders the winner by adding up the scores from three disciplines. The three disciplines were Constant weight deep diving with fins, where 1 m depth generates 1 point, Static apnea, where 5 s generates 1 point, and Dynamic apnea with fins, where 2 m yields 1 point. The total score was used as a representation of individual overall diving performance.
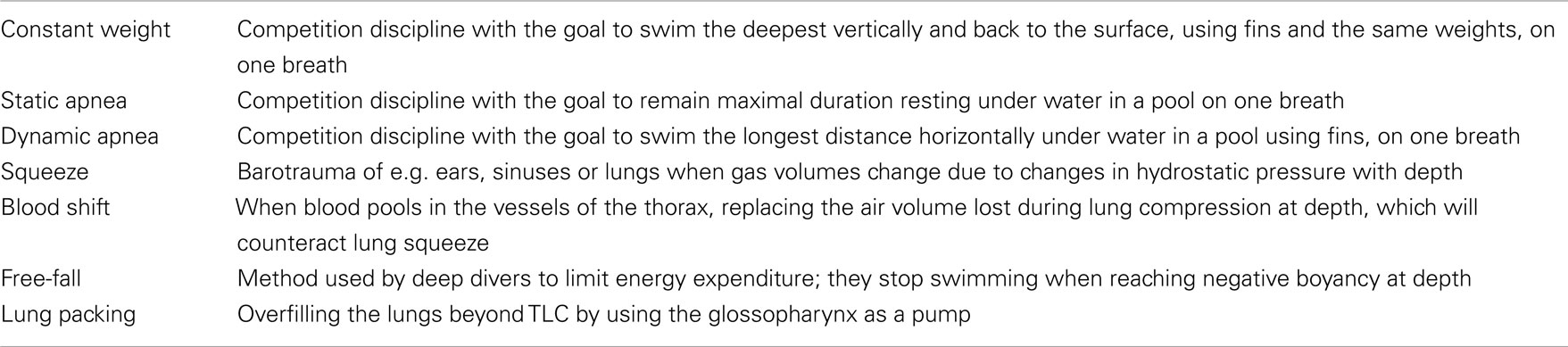
Table 2. Disciplines and explanation of some special terms used in sports apnea (see also Schagatay, 2009, 2010, 2011).
Measurements
Subjects arrived at the laboratory after at least 45 min without apnea, physical activity, or eating. Ambient temperature was 23–25°C. Vital capacity (VC) in the standing position, without lung packing, was measured three times (Microlab spirometer, Micro Medical Ltd., Kent, UK). Subjects’ height and weight were also measured and training data collected. Spleen measurements were obtained via ultrasonic imaging (Mindray DP-6600, Shenzhen Mindray Bio-Medical Electronics Co., Ltd., Shenzhen, China) using the following protocol: Divers were semi-seated vertically on an elevated stool for approximately 3 min while the site for spleen measurements was identified from the dorsal side, followed by measurement of spleen maximal length, width, and thickness every minute for 7 min. From these triaxial measurements of length (L), width (W), and thickness (T), spleen volume was calculated using the Pilström formula: Lπ (WT − T2)/3 (Schagatay et al., 2005).
In order to estimate the contribution of the expelled blood volume to resting apneic duration, the three highest scoring divers were asked to perform a time limited apnea of 2 min, to determine their magnitude of spleen contraction, in addition to the baseline measurements. This short apnea was performed in the sitting position without prior hyperventilation. Triaxial spleen measurements were obtained every minute before the apnea and after the apnea to obtain a recovery profile.
Analysis
To obtain an individual baseline measurement of spleen volume, the mean of the two largest subsequent volume measurements recorded during the 7 min resting period was used, this was done to minimize the influence of measurement error due to slight changes in probe placement, and to limit the influence of the pulsatile changes in spleen volume. With measurements of VC the largest of the three measurements was used in the analysis, which is the standard procedure to limit the influence of the unaccustomed subject’s failure to fill or empty lungs completely in each test. Spleen and lung volume, height, weight and training data from the divers were compared with the individual total scores in the competition via Pearson product-moment correlation. For an estimation of the relative contribution to apneic duration of spleen- and lung volumes, the mean values of spleen and lung volumes from the three subjects with the highest competition scores were compared to those of the three lowest-scoring divers. Between-group comparisons were completed using unpaired Student’s t-tests and within-group tests using paired t-tests. The accepted level for differences was P < 0.05.
A statistical multivariable regression partial least squares model, with cross-validation, was established using the software SIMCA-P+ v.12. The degree of fit (R2) of the model was 63% and the model validity (Q2) 55%, where the variables spleen volume, lung volume, height, number of years of apnea training and the amount of physical training per week were described by the model. A permutation test was conducted to validate the results.
Results
Diving Performance
Mean (SE) competition dive performances of participating subjects were 75 (4) m for constant weight deep diving, 5 min 53 (39) s for static apnea and 139 (13) m for dynamic apnea, which are to our knowledge the highest mean performances reported in any subject group studied in apnea physiology. The mean total competition score for the tested divers was 211 (8) points.
Correlations between Anthropometric Data and Performance
Spleen Volume
Mean (SE) spleen volume was 336 (32) mL with range 215–598 mL. Spleen volume was not correlated with individual height or weight, but was positively correlated with total competition score (r = 0.57; P < 0.05; Figure 1A), and also after correction for height (r = 0.55; P < 0.05) and weight (r = 0.57; P < 0.05; Table 1).
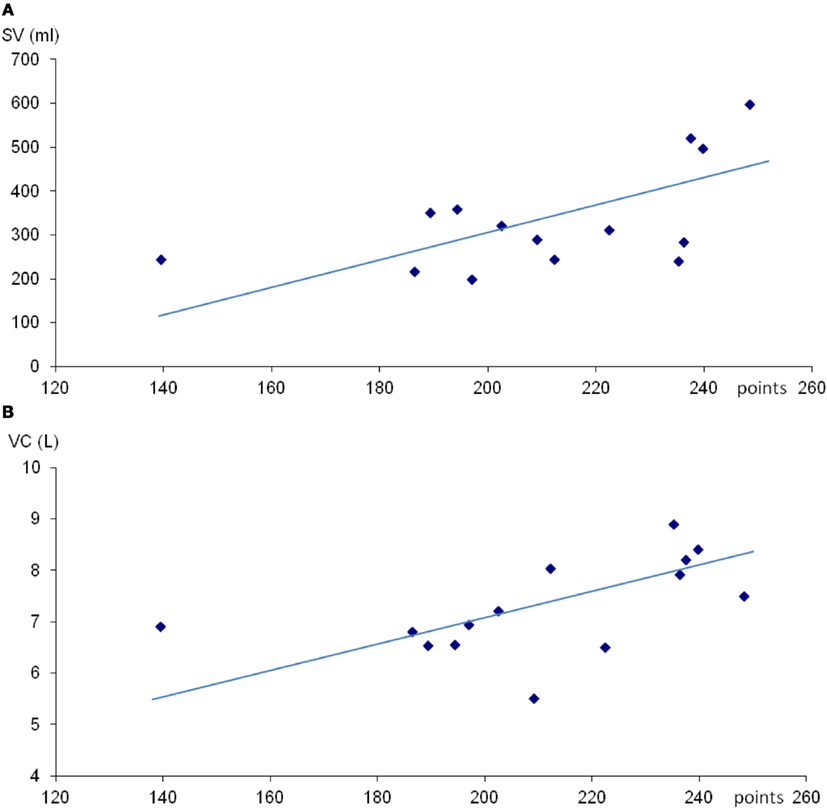
Figure 1. Scatter diagram of spleen volume (SV; A) and vital capacity (VC; B) against total points in apnea competition. For (A), r = 0.57 and for (B), r = 0.54 (both P < 0.05).
Vital capacity
Mean (SE) VC was 7.3 (0.25) L with a range from 5.5 to 8.9 L. No correlation between VC and subjects height or weight was observed. There was a positive correlation between VC and total competition score (r = 0.54; P < 0.05; Figure 1B).
Other factors
Subject height, weight, and BMI were not correlated with performance (Table 1). There was no correlation between performance and hours of apnea training during the month prior to the competition, but there was a trend toward negative correlation between physical training and performance (r = −0.48; P < 0.1; Table 1).
Multivariable analysis
Spleen- and lung volume were significantly (99%) related to the total sum of scores the divers accomplished from the three disciplines. The variables height, number of years of apnea training and the amount of apnea training or physical training per week were not significantly related to the total sum of scores. The result was confirmed by the permutation test.
Estimated Contributions from Spleen and Lung Volumes to Apneic Performance
Effect of spleen volume
The three highest scoring divers (mean 242 pts) had a mean (SD) spleen volume of 538 (53) mL and the three lowest-scoring divers (mean 172 pts) a volume of 270 (71) mL; (P < 0.01; Figure 2) while height and weight of the two groups were similar, at 183 (4.7) and 183 (4.0) cm, and 77 (3) and 91 (23) kg, respectively (NS).
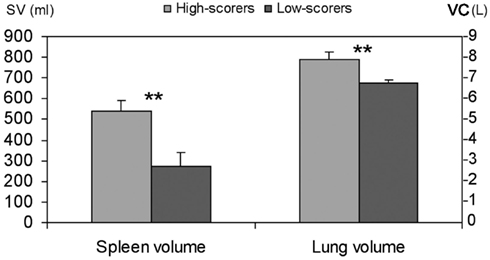
Figure 2. Mean (SD) spleen volume (SV) and lung volumes vital capacity (VC) in highest (n = 3) and lowest (n = 3) scoring divers. Significance at P < 0.01 is indicated by **.
When the highest scoring subjects performed a 2 min apnea, their mean spleen volume decreased by 260 mL (P < 0.05; Figure 3). Based on the spleen volume reduction observed, the splenic blood contribution to apneic duration in high performing divers was estimated. Spleen blood contains at least double the normal hematocrit (Laub et al., 1993; Stewart and McKenzie, 2002) and with 260 mL of spleen blood being added to the circulation, equivalent in Hb content to at least 500 mL of whole blood, it could bind another 100 mL of O2. Estimating during resting apnea, based on RMR measurements via indirect calorimetry (Byrne et al., 2005), 2.67 mL O2/kg/min, as approximately 200 mL O2/min, the extra O2 stored in the three top-scoring divers would last for 30 s. The smaller spleens in the three lowest-scoring divers would, assuming a similar 50% spleen volume reduction, contribute extra O2 lasting approximately 15 s. Thus, the three best divers could extend apneic duration by 15 s in comparison to the three lowest-scoring divers, due to the extra O2 storage capacity resulting from the Hb expelled by their larger spleens.
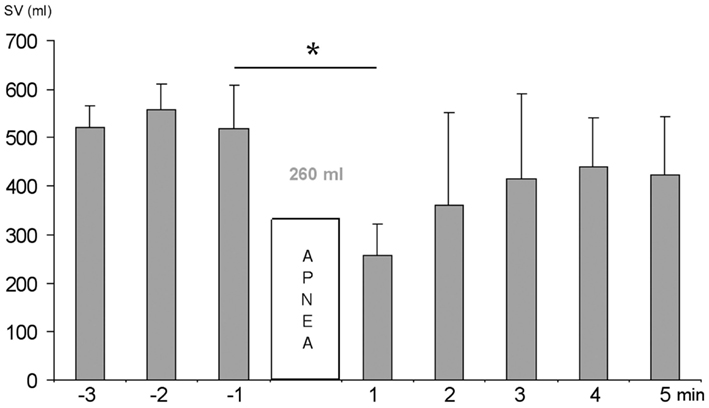
Figure 3. Minute to minute mean (SD) spleen volume (SV) before and after 2 min apnea in the three highest scoring divers. Significance at P < 0.05 is indicated by *.
Effect of vital capacity
Mean (SD) VC was 7.9 (0.36) L in the three top-scorers and 6.7 (0.19; P < 0.01) in the three lowest-scoring divers (Figure 2). The mean VC in the three best divers was thus more than 1 L greater than in the three lowest-scoring divers, equivalent to approximately 200 mL of extra O2 storage, allowing for an extension of apneic duration by approximately 1 min.
Discussion
A significant correlation was found between accumulated competition score and both spleen volume and lung volume, despite the relatively small number of subjects investigated in this study, suggesting that both these factors may predict competitive apneic performance. There was a large span of individual variation in spleen- and lung volume among divers, showing that specific values of such traits are not necessarily prerequisite for participation at the elite level. However, from the group correlation analysis it is clear that both these factors were associated with the production of top results. This is a novel finding, which may be useful in predicting performance in apneic divers. An interesting question arising from these findings is whether apnea-specific training can increase spleen and lung size, or if successful divers self-recruit to the sport by virtue of this physiological predisposition.
Spleen Volume
Previously reported spleen volumes in the general population vary greatly, and the average in 140 healthy Caucasians of both genders was found to be 215 mL, spanning from 107 to 342 mL (Prassopoulos et al., 1997). These values appear to be lower than the mean of 336 mL observed in our study’s elite apneists. The span 215–598 mL in our studied divers starts with the normal value reported by Prassopoulos et al. (1997). In accordance with that study, we found no correlation between subject height or weight and spleen volume, and they also did not find any difference between genders or age groups. Geraghty and associates reported a mean value of 238 mL in 47 mid-aged males, spanning 76–400 mL, but a volume of only 180 mL in females (Geraghty et al., 2004). They had corrected values for height and weight using a standardized body size. When standardized for height and weight, our divers’ mean spleen volume was in the 90th percentile of Geraghty and associates’ observed range and the values of our three best divers more than 100 mL above their recorded maximum. Our lowest-scoring divers were in the 60th percentile, thus near the median presented by Geraghty and associates. It cannot be ruled out that differences in methods between the studies cited above and the present study could yield different results. However, in a study on 10 non-divers of both genders, using the same method for spleen measurements as in the present study but with subjects in a prone position, we found a mean spleen volume of 275 mL, with a range of 185–391 mL (Schagatay et al., 2005). This is similar to the other values reported for non-divers, but clearly less than the 336 mL found in the elite divers in the present study. Bakovic et al. (2003) reported a mean spleen volume in 10 elite divers of 344 mL, which corresponds well to the divers in the present study, but spleen volume in their control group was only slightly smaller.
This is the first report of competitive apneists’ spleen volumes in relation to their performance. The finding supports a previous indication that trained apneists may have larger spleens or more pronounced spleen contraction, or possibly a higher spleen hematocrit than non-divers; a greater elevation of Hb was observed after three maximal apneas in trained apneic divers compared to both non-diving athletes and sedentary non-divers (Richardson et al., 2005). Studies by Hurford et al. (1990), Bakovic et al. (2003) and Prommer et al. (2007) also suggest that spleen contraction could be at least somewhat augmented in apneic divers. However, in previous studies the apneas performed by the divers have been longer than for non-divers, which could possibly account for their more powerful spleen contraction.
Seal species performing deep and long during dives appear to have larger spleens that average suggesting that benefits of such traits are present in diving mammals (Qvist et al., 1986). It is not known if training can increase mammalian spleen volume, but the spleen appears to have a high regenerative ability. After the experimental removal of 2/3 of the spleen, the remaining tissue showed compensatory growth in baboons (van Rensburg et al., 1991). In humans, growth of a small, accessory spleen after removal of the main spleen has been demonstrated (Hong et al., 1963) and the intact human spleen increased in volume to 179% its original size within a month in parallel with liver regeneration after part of the liver had been surgically removed (Kotton and Fine, 2008), suggesting that the same growth factors are involved. Whether our findings indicate training-stimulated growth or reflects individual predisposition can not be determined in this study, but the spleen volume of the three most successful divers is clearly in the upper range of that observed in healthy humans, which may indicate this possibility.
Lung Volume
Previously reported mean VC in the general adult male population was 4.1 L for 225 Caucasian subjects with a mean age of 19 years and height of 162 cm (Chiba et al., 1984; Laub et al., 1993) and after correction for differences in height, our subjects’ mean VC was 158% of this reference group. Thus, the 7.3 L mean lung volume, with a span from 5.5 to 8.9 L in our study’s elite divers, was well above normal. Previously reported mean VC in eight male divers was 6.1 L (span 5.6–6.7 L; Andersson et al., 2002) and 6.3 L in another group of 15 male divers (span 5.0–7.1 L; Andersson et al., 2004), where both groups were of similar age, height, and weight as in the present study but also included recreational apnea divers. No comparison between competition results and VC among divers has been published previously, but the results are in line with studies comparing groups of non-divers with trained divers and reporting greater lung volumes in the divers (see review by Ferretti and Costa, 2003). There is also a report of similar lung volumes between apnea divers and non-divers (Bakovic et al., 2003).
Large lungs in skilled divers could be due to individual predisposition, increased respiratory muscle strength or chest flexibility, or it could be due to training-stimulated lung growth. A direct increase in lung volume as a response to training is suggested by the longitudinal changes in divers observed by Carey et al. (1956). Other research suggests an enlarging effect on the lungs by high altitude exposure and by swimming, but most likely not by other sports (Gaultier and Crapo, 1997). The presence of stem cells in lung tissue (Kotton and Fine, 2008), as well as the observation that lobular removal of lung lobes in children may lead to regeneration to normal size within 2 years (Nakajima et al., 1998) suggests that lung growth may be induced in man, at least at a young age. It is also suggested by statements from elite divers that lung volume has been increased substantially since they started training in adult age by specific lung training protocols (personal communication), and while only minor (3%) increases in VC were observed in a study using lung packing alone in previously untrained subjects (Nygren-Bonnier et al., 2007) we have seen a larger increase in lung volume after training, using the complex training programs developed by elite divers (unpublished results).
While many mammalian divers have lung volumes comparable to terrestrial mammals, there are also semiaquatic species with large lungs, e.g., the sea otter (Lenfant et al., 1970). With a diving pattern resembling the natural diving in humans (Bodkin et al., 2004; Schagatay et al., 2011) it is likely that sea otters also benefit from maximizing lung oxygen storage, while deep diving species may on the contrary exhale before submersing and allow lung collapse at depth.
Contributions from Spleen and Lung to Apneic Duration in Top Athletes
Extended apneic duration is required for good performance in all disciplines of competitive apnea. Previous research has shown an apnea-prolonging effect by repeated apneas in intact but not in splenectomized subjects, ascribed to splenic contraction (Schagatay et al., 2001; Bakovic et al., 2003). A larger spleen with the ability to eject more stored red blood cells would be beneficial for the apnea diver, allowing enhancement of both body oxygen stores and asphyxia tolerance via increased buffering of CO2, and subsequent prolonged apneic duration. The estimated contribution of the spleen blood to apneic duration in the most successful divers, compared to the least successful divers was 15 s, based on the estimated difference in spleen volume reduction between these groups. Reported spleen volume reductions of between 18 and 35% in other studies (Hurford et al., 1990; Bakovic et al., 2003; Schagatay et al., 2005; Richardson et al., 2006) shows that the spleen contractions seen in our top three divers was indeed high, and suggests that the low scorers would probably not display a more powerful contraction. With less relative spleen contraction in low scorers the time gain in high-scorers would be even greater. It should further be noted that the single, non-maximal apnea used in this study would, perhaps not have elicited the full spleen response (Schagatay et al., 2001, 2005). Metabolic rate during apnea performance is most likely lower than in any other sport, and apnea performance is often preceded by meditation-like stages. The VO2 during resting apnea in 78 healthy males was found to be 2.67 mL O2/kg/min via indirect calorimetry (Byrne et al., 2005) which is lower than most RMR values reported. As divers have low metabolic rate, we estimated that our divers would use about 200 mL O2/min. It is even possible that apneic is lower, as >20% reduction of the resting aerobic metabolism during apnea on account of the diving response has been demonstrated (Andersson et al., 2008). In addition to the increased oxygen storage, the increased Hb during apnea would enhance the CO2 buffering capacity and delay the urge to breathe and the individual breaking point. In competitive apnea, the differences in splenic contribution may thus play a decisive role for competition results, and spleen contraction may be initiated during the warm up procedures often seen (Schagatay, 2010).
The mean VC in the three most successful divers studied was more than 1 L greater than in the three lowest-scoring divers, likely affecting apneic duration by adding about 200 mL of O2 to the total body stores corresponding an additional 60 s of apnea, which shows that lung volume is a major factor for determining human apneic duration. Aside from enhanced O2 storage, the CO2 storage capacity would be increased by the larger VC by a dilution effect which could prolong apneas further. An enlarged VC could furthermore contribute to faster recovery after apnea by reducing relative dead space and increasing alveolar gas exchange by enlarging minute ventilation at a given respiratory frequency. This could provide additional benefits to competitive apneists, and also be important in repeated diving in for example fishing divers. However, lung volume is not known to be large among diving mammals, and some seals dive after exhalation, likely reducing buoyancy and avoiding decompression illness by limiting alveolar gas exchange when the lungs are allowed to collapse during diving (Butler, 2001). This may be compared to professional Ama divers, diving with only 85% of VC for repeated sea harvesting at moderate depths (Hong et al., 1963). Thus not only positive effects result from using large lung volumes and for sub-maximal dives the optimal volume may be below total lung capacity (TLC). Competitive apneists instead fill their lungs beyond TLC by “lung packing” (Örnhagen et al., 1998) which has been shown to prolong apneas compared to diving at TLC, which in turn yielded longer apneas than using 85% of TLC (Overgaard et al., 2006). This demonstrates the great importance of lung gas storage capacity for extended human diving. The TLC to RV ratio is also important for reaching great depths and increasing TLC without increasing RV would thus be beneficial for deep diving (Schaefer et al., 1968).
Other Factors
Training
The tendency for a negative correlation between time spent with general physical training and apneic performance could possibly reflect a time limitation when divers spent more time with apnea related training during the weeks before the competition, but it is also in line with the previous findings that there is no increase of the diving response with increasing physical fitness (Strømme and Ingjer, 1978; Schagatay et al., 2000). The lack of correlation between time invested in apnea training and competition result was somewhat surprising (Table 1), as previous findings have found that apnea training enhanced diving bradycardia and prolonged apneic duration (Schagatay et al., 2000). It is clear, however, that specific apnea training, with effects on several systems essential for apneic diving, is necessary at this level of performance, as the average time spent on apnea training in the tested group was >6 h/week.
Physiological responses and techniques
Many physiological factors aside from spleen- and lung volume are likely involved in setting the limits in competitive apnea diving (reviewed in Schagatay, 2009, 2010, 2011). In the swimming disciplines, in addition to the tolerance to hypoxia, technique, and work economy are important (Schagatay, 2010). Also the magnitude of diving response (Andersson and Schagatay, 1998a; Schagatay and Andersson, 1998) anaerobic capacity and tolerance of accumulation of hypoxia induced factors are likely factors involved in determining individual performance (Schagatay, 2010). Baseline hematocrit is known to be high in divers (Richardson et al., 2005), a condition which may be induced by apnea training (de Bruijn et al., 2008). Depth disciplines are dependent on advanced training and use of specific equalization techniques to avoid squeeze when the lung is compressed below RV, and factors such as the capacity for “blood shift” (Ferrigno et al., 1987) and the ability to use free-fall (Table 2) in order to conserve energy will affect the depth reached (Schagatay, 2011). For depth, also the neuropsychological tolerance to nitrogen narcosis (Ridgway and McFarland, 2006) will be increasingly important, and with depths increasing beyond 100 m there is also an increasing risk of developing decompression sickness on ascent (Schagatay, 2011) and a negative effect of a large lung air supply may become evident; divers may eventually have to allow lung collapse to reach further (Fitz-Clarke, 2007).
Not only physiological features and individual results determine who participates in the world championship in a young sport like apnea. There is little financial incentive in apneic diving, and the sport is generally not supported by national talent development programs. Therefore, socioeconomic factors may also determine the circumstances for training and participation in competitions. Despite limited recruitment, there have been dramatic and continual improvements in elite apneic performance in recent years, showing that human diving capacity may approach that of some semiaquatic species, e.g., the sea otter, once the full capacity is explored. Individual spleen- and lung volume measurement may be an effective method of identifying “hidden” talent on performance. Longitudinal study is necessary to determine whether these features are inherent or acquired by apnea-specific training.
Conclusion
This study suggests that spleen volume as well as lung volume may contribute to successful apnea performance in humans. A correlation of spleen volume with apneic performance has never been described before but is functionally logical, as ejection of additional red blood cells to circulation will both enhance oxygen storage and CO2 buffering capacity. Lung volume has previously been reported to be greater in divers than in non-divers, but this is the first report connecting VC to apnea competition results on the individual level. Studies of performance-predicting factors in competitive apnea will provide a functional evaluation of human apneic diving ability in a mammalian context and, from a sports perspective, it may help to identify talent and aid divers in improving training techniques and performance.
Conflict of Interest Statement
The authors declare that the research was conducted in the absence of any commercial or financial relationships that could be construed as a potential conflict of interest.
Acknowledgments
We thank the volunteer divers for participation in our study, and coaches and staff around the competition site for help with recruitment and with building a temporary laboratory. The study was supported by funds from the Swedish Centre of Research in Sports (CIF).
References
Andersson, J., and Schagatay, E. (1998a). Arterial oxygen desaturation during apnea in humans. Undersea Hyperb. Med. 25, 21–25.
Andersson, J., and Schagatay, E. (1998b). Effects of lung volume and involuntary breathing movements on the human diving response. Eur. J. Appl. Physiol. 77, 19–24.
Andersson, J., Schagatay, E., Gustafsson, P., and Örnhagen, H. (1998). “Cardiovascular effects of ‘buccal pumping’ in breath-hold divers,” in 24th Annual Scientific Meeting of the European Underwater and Baromedical Society, Stockholm, 84–87.
Andersson, J. P., Biasoletto-Tjellstrom, G., and Schagatay, E. K. (2008). Pulmonary gas exchange is reduced by the cardiovascular diving response in resting humans. Respir. Physiol. Neurobiol. 160, 320–324.
Andersson, J. P., Liner, M. H., Runow, E., and Schagatay, E. K. (2002). Diving response and arterial oxygen saturation during apnea and exercise in breath-hold divers. J. Appl. Physiol. 93, 882–886.
Andersson, J. P. A., Liner, M. H., Fredsted, A., and Schagatay, E. K. A. (2004). Cardiovascular and respiratory responses to apneas with and without face immersion in exercising humans. J. Appl. Physiol. 96, 1005–1010.
Bakovic, D., Valic, Z., Eterovic, D., Vukovic, I., Obad, A., Marinovic-Terzic, I., and Dujic, Z. (2003). Spleen volume and blood flow response to repeated breath-hold apneas. J. Appl. Physiol. 95, 1460–1466.
Barcroft, J., and Stevens, J. G. (1927). Observations upon the size of the spleen. J. Physiol. 64, 1–22.
Bodkin, J. L., Esslinger, G. G., and Monson, D. H. (2004). Foraging depths of sea otters and implications to coastal marine communities. Mar. Mamm. Sci. 20, 305–321.
Byrne, N. M., Hills, A. P., Hunter, G. R., Weinsier, R. L., and Schutz, Y. (2005). Metabolic equivalent: one size does not fit all. J. Appl. Physiol. 99, 1112–1119.
Carey, C. R., Schaefer, K. E., and Alvis, H. J. (1956). Effect of skin diving on lung volume. J. Appl. Physiol. 8, 519–523.
Chiba, T., Boles, E. T. Jr., and Staples, G. (1984). Experimental studies on the rat-model for partial splenectomy and on spleen regeneration. Nippon Geka Gakkai Zasshi 85, 49–55.
de Bruijn, R., Richardson, M., and Schagatay, E. (2008). Increased erythropoietin concentration after repeated apneas in humans. Eur. J. Appl. Physiol. 102, 609–613.
Ferretti, G., and Costa, M. (2003). Diversity in and adaptation to breath-hold diving in humans. Comp. Biochem. Physiol. Part A Mol. Integr. Physiol. 136, 205–213.
Ferrigno, M., Hickey, D. D., Linér, M. H., and Lundgren, C. E. G. (1987). Simulated breath-hold diving to 20 meters: cardiac performance in humans. J. Appl. Physiol. 62, 2160–2167.
Fitz-Clarke, J. (2007). Mechanisms of airway, and alveolar collapse in human breath-hold diving. Respir. Physiol. Neurobiol. 159, 202–210.
Gaultier, C., and Crapo, R. (1997). Effects of nutrition, growth hormone disturbances, training, altitude and sleep on lung volumes. Eur. Respir. J. 10, 2913–2919.
Geraghty, E. M., Boone, J. M., McGahan, J. P., and Jain, K. (2004). Normal organ volume assessment from abdominal ct. Abdom. Imaging 29, 482–490.
Hong, S. K., Rahn, H., Kang, D. H., Song, S. H., and Kang, B. S. (1963). Diving pattern, lung volumes, and alveolar gas of the Korean diving woman (ama). J. Appl. Physiol. 18, 457–465.
Hurford, W. E., Hong, S. K., Park, Y. S., Ahn, D. W., Shiraki, K., Mohri, M., and Zapol, W. M. (1990). Splenic contraction during breath-hold diving in the korean ama. J. Appl. Physiol. 69, 932–936.
Laub, M., Hvid-Jacobsen, K., Hovind, P., Kanstrup, I. L., Christensen, N. J., and Nielsen, S. L. (1993). Spleen emptying and venous hematocrit in humans during exercise. J. Appl. Physiol. 74, 1024–1026.
Lenfant, C., Johansen, K., and Torrance, J. D. (1970). Gas transport and oxygen storage capacity in some pinnipeds and the sea otter. Respir. Physiol. 9, 277–286.
Nakajima, C., Kijimoto, C., Yokoyama, Y., Miyakawa, T., Tsuchiya, Y., Kuroda, T., Nakano, M., and Saeki, M. (1998). Longitudinal follow-up of pulmonary function after lobectomy in childhood – factors affecting lung growth. Pediatr. Surg. Int. 13, 341–345.
Nygren-Bonnier, M., Lindholm, P., Markström, A., Skedinger, M., Mattsson, E., and Kelfbeck, B. (2007). Effects of glossopharyngeal pistoning for lung insufflation on vital capacity in healthy women. Am. J. Phys. Med. Rehabil. 86, 290–294.
Örnhagen, H., Schagatay, E., Andersson, J., Bergsten, E., Gustafsson, P., and Sandström, S. (1998). “Mechanisms of ‘buccal pumping’ (‘lung packing’) and its pulmonary effects,” in 24th Annual Scientific Meeting of the European Underwater and Baromedical Society, Stockholm, 80–83.
Overgaard, K., Friis, S., Pedersen, R. B., and Lykkeboe, G. (2006). Influence of lung volume, glossopharyngeal inhalation and p(et) O2 and p(et) CO2 on apnea performance in trained breath-hold divers. Eur. J. Appl. Physiol. 97, 158–164.
Prassopoulos, P., Daskalogiannaki, M., Raissaki, M., Hatjidakis, A., and Gourtsoyiannis, N. (1997). Determination of normal splenic volume on computed tomography in relation to age, gender and body habitus. Eur. Radiol. 7, 246–248.
Prommer, N., Ehrmann, U., Schmidt, W., Steinacker, J. M., Radermacher, P., and Muth, C. M. (2007). Total haemoglobin mass and spleen contraction: a study on competitive apnea divers, non-diving athletes and untrained control subjects. Eur. J. Appl. Physiol. 101, 753–759.
Qvist, J., Hill, R. D., Schneider, R. C., Falke, K. J., Liggins, G. C., Guppy, M., Elliot, R. L., Hochachka, P. W., and Zapol, W. M. (1986). Hemoglobin concentrations and blood gas tensions of free-diving weddell seals. J. Appl. Physiol. 61, 1560–1569.
Richardson, M., de Bruijn, R., Holmberg, H., Björklund, G., Haughey, H., and Schagatay, E. (2005). Increase of hemoglobin concentration after maximal apneas in divers, skiers and untrained humans. Can. J. Appl. Physiol. 30, 276–281.
Richardson, M., de Bruijn, R., Pettersson, S., Reimers, J., and Schagatay, E. (2006). Correlation between spleen size and hematocrit during apnea in humans. Paper Presented at the Proceedings of the Undersea and Hyperbaric Medical Society/Divers Alert Network, Orlando, FL.
Ridgway, L., and McFarland, K. (2006). Apnea diving: long-term neurocognitive sequelae of repeated hypoxemia. Clin. Neuropsychol. 20, 160–176.
Schaefer, K. E., Allison, R. D., Dougherty, J. H. Jr., Carey, C. R., Walker, R., Yost, F., and Parker, D. (1968). Pulmonary and circulatory adjustments determining the limits of depths in breathhold diving. Science 162, 1020–1023.
Schagatay, E. (2009). Review: predicting performance in competitive apnoea diving. Part I: static apnoea. Diving Hyperb. Med. 39, 88–99.
Schagatay, E. (2010). Review: predicting performance in competitive apnea diving. Part II: dynamic apnea. Diving Hyperb. Med. 40, 11–22.
Schagatay, E. (2011). Review: predicting performance in competitive apnea diving. Part III: depth. Diving Hyperb. Med. 41, 216–228.
Schagatay, E., and Andersson, J. (1998). Diving response and apneic time in humans. Undersea Hyperb. Med. 25, 13–19.
Schagatay, E., Andersson, J. P. A., Hallén, M., and Pålsson, B. (2001). Selected contribution: role of spleen emptying in prolonging apneas in humans. J. Appl. Physiol. 90, 1623–1629.
Schagatay, E., Angelica, L.-S., and Erik, A. (2011). Underwater working times in two groups of traditional apnea divers in Asia: the Ama and the Bajau. Diving Hyperb. Med. 41, 27–30.
Schagatay, E., Haughey, H., and Reimers, J. (2005). Speed of spleen volume changes evoked by serial apneas. Eur. J. Appl. Physiol. 93, 447–452.
Schagatay, E., van Kampen, M., Emanuelsson, S., and Holm, B. (2000). Effects of physical and apnea training on apneic time and the diving response in humans. Eur. J. Appl. Physiol. 82, 161–169.
Stewart, I. B., and McKenzie, D. C. (2002). The human spleen during physiological stress. Sports Med. 32, 361–369.
Strømme, S. B., and Ingjer, F. (1978). Comparison of diving bradycardia and maximal aerobic power. Aviat. Space Environ. Med. 49, 1267–1270.
Keywords: spleen contraction, hematocrit, vital capacity, diving response, breath-hold, mammalian, diving capacity, training
Citation: Schagatay E, Richardson MX and Lodin-Sundström A (2012) Size matters: spleen and lung volumes predict performance in human apneic divers. Front. Physio. 3:173. doi: 10.3389/fphys.2012.00173
Received: 02 February 2012; Paper pending published: 02 March 2012;
Accepted: 11 May 2012; Published online: 18 June 2012.
Edited by:
Andreas Fahlman, Texas A&M University Corpus Christi, USAReviewed by:
John Fitz-Clarke, Dalhousie University, CanadaMatthew David White, Simon Fraser University, Canada
Copyright: © 2012 Schagatay, Richardson and Lodin-Sundström. This is an open-access article distributed under the terms of the Creative Commons Attribution Non Commercial License, which permits non-commercial use, distribution, and reproduction in other forums, provided the original authors and source are credited.
*Correspondence: Erika Schagatay, Department of Engineering and Sustainable Development, and Swedish Winter Sports Research Centre, Mid Sweden University, Akademigatan 1, 83125 Östersund, Sweden. e-mail: erika.schagatay@miun.se