- 1 Division of Molecular Medicine, Cardiovascular Research Laboratories, Department of Anesthesiology, David Geffen School of Medicine at University of California Los Angeles, Los Angeles, CA, USA
- 2 Division of Molecular Medicine, Cardiovascular Research Laboratories, Department of Medicine/Cardiology, David Geffen School of Medicine at University of California Los Angeles, Los Angeles, CA, USA
- 3 Division of Cardiovascular Medicine, Oregon Health and Science University, Portland, OR, USA
Background/Objectives: Atrial fibrillation (AF) is the most common type of cardiac arrhythmia with patients dying frequently of stroke. In view of the unclear etiologies of AF and a potential role of oxidative stress, the present study examined cardiac reactive oxygen species production and NADPH oxidase (NOX) expression in AF patients. Methods and Results: Patients with AF were older than those without (58.8 ± 11.7 vs. 47.8 ± 19.2, p = 0.047). Whereas total production (determined by electron spin resonance) was similar in patients with and without AF, H2O2 production was more than doubled in AF patients (149.8 ± 26.28 vs. 66.9 ± 7.14 pmol/mg/min, p = 0.0055), which correlated well with a doubling in NOX isoform 4 (NOX4) expression. AF patients with co-existing hypertension had three-fold higher H2O2 production compared to those without (239.0 ± 125.1 vs. 83.6 ± 51.3 pmol/mg/min, p = 0.003). Treatment of HL-1 atrial cells with angiotensin II, a known modulator of atrial structural remodeling, resulted in upregulation of NOX4 and H2O2 production, further implicating a potential role of NOX4 in atrial remodeling. Conclusion: Our data represent the first implication that NOX4-derived H2O2 may play an important role in the etiologies of AF.
Introduction
Atrial fibrillation (AF) is the most common type of cardiac arrhythmia that is rapidly developing into an epidemic (Savelieva and Camm, 2008). It is associated with markedly increased risk of stroke, due to dislocation of thrombi that are most often originated from left atrial appendage (LAA). Although AF is clearly associated with aging, and cardiovascular conditions such as hypertension, mitral valve stenosis, and heart failure, molecular mechanisms underlying its etiology have remained elusive. Recent advances seem to suggest that AF is initiated by atrial structural and electrical remodeling, and that AF itself further augments these responses to perpetuate AF (Savelieva and Camm, 2008; Chou and Chen, 2009).
The known risk factors for AF have all been linked to oxidative stress, and inflammatory factors such as angiotensin II (Ang II) (Kannel et al., 1998; Nakashima et al., 2000; Kim et al., 2003, 2005). Ang II is a major vasoconstrictive hormone and its production is elevated in patients with hypertension. It is also involved in modulating cardiac hypertrophy and remodeling. Of note, hypertension is considered the most prevalent risk factor for AF. Atrial structural remodeling can be induced by Ang II, resulting in atrial fibrosis and possibly initiation of AF (Zografos and Katritsis, 2010; Tan and Zimetbaum, 2011). A meta-analysis of 7 clinical trials involving a total of 24,849 patients receiving ACEIs and/or ARBs for treatment of hypertension, ischemic heart disease, heart failure, or diabetes mellitus demonstrated that ACEIs/ARBs significantly reduces the risk of AF or its recurrence by 28% (Healey et al., 2005). Importantly, Ang II is an extremely potent activator of NADPH oxidase (NOX) in the vascular cells (Cai et al., 2003; Choi et al., 2008; Lassegue and Griendling, 2010). NOX-dependent reactive oxygen species (ROS) production leads to nitric oxide () inactivation and endothelial dysfunction, which underlie pathogenesis of vascular diseases such as hypertension and atherosclerosis (Cai and Harrison, 2000; Cai et al., 2003; Cai, 2005a,b). We hypothesize that NOX-derived ROS might be important in mediating Ang II-dependent development of AF. Some interesting data exist to show that hydrogen peroxide increases Kv1.5 current amplitude at voltages corresponding to the action potential repolarization phase and accelerate Kv1.5 channel opening. These changes can reduce the action potential duration, leading to a shortening of the atrial effective refractory period (Caouette et al., 2003)
To test this hypothesis in human AF, cardiac tissues from heart transplant patients were examined for ROS production and expression of NOX isoforms. Interestingly, we found a specific upregulation of NOX4 that was correlated with H2O2 overproduction in AF patients. None of the other NOX isoforms were however regulated in AF. AF patients with co-existing hypertension had more than 3-fold increase in H2O2 production compared to those without. In atrial HL-1 cells, Ang II treatment led to increased AT1 receptor expression, upregulation of NOX4 and increased H2O2 production. These human and cell culture data together implicate that there might be a novel role of NOX4-derived H2O2 in mediating Ang II-dependent AF development.
Materials and Methods
Patient Population
Left atrial appendage tissues isolated from randomly recruited patients undergoing cardiac transplant surgeries were subjected to electron spin resonance (ESR) determination of production, Amplex red determination of H2O2 production and RT-PCR analysis of NOX gene expression. The study was carried out in a double-blinded fashion. Clinical information was gathered upon completion of laboratory analyses. IRB approval was obtained for these studies.
Electron Spin Resonance Detection of Superoxide Radical
The specific spin trap CMH (0.5 mmol/L; Noxygen) solution was prepared freshly in nitrogen gas bubbled Krebs/HEPES buffer containing diethyldithiocarbamic acid (DETC; 5 μmol/l; Sigma) and deferoxamine (25 μmol/l; Sigma). The LAA homogenates (in 50 mmol/L Tris–HCl, pH 7.4, 0.1 mmol/L EDTA, 0.1 mmol/L EGTA, and 1% (v/v) of protease inhibitor cocktail from Sigma) were then mixed with the spin trap solution and loaded into glass capillary (Fisher Scientific) for analysis of production using ESR spectrometer (Bruker), following a similar procedure as we previously published for cultured endothelial cells (Chalupsky and Cai, 2005; Gao et al., 2009a,b) intact mouse aortas (Chalupsky and Cai, 2005; Oak and Cai, 2007; Gao et al., 2009a), and tissue homogenates (Lu et al., 2009). The ESR settings used were as follows: bio-field 3,410, field sweep 100G, microwave frequency 9.73 GHz, microwave power 13.26 mW, modulation amplitude 9.82G and 512 points of resolution.
Amplex-red Assay of Hydrogen Peroxide Production
The LAA lysates [in 20 mmol/L Tris–HCl, pH 7.4, 150 mmol/L NaCl, 1 mmol/L EGTA, 1 mmol/L EDTA, 1% (v/v) Triton X-100, 2.5 mmol/L sodium pyrophosphate, 1 mmol/L Na3VO4, 1 mmol/L β-glycerolphosphate, 1 mmol/L PMSF, 1% (v/v) of protease inhibitor cocktail from Sigma] were analyzed for H2O2 production using a fluorometric horseradish peroxidase assay (Amplex-Red assay; Molecular Probes). Fluorescence was measured (excitation 530 nm and emission 590 nm) after 1 h of incubation at 37°C in the dark against background fluorescence of buffer. Polyethylene glycol-conjugated catalase (500 U/ml, Sigma) inhibitable fraction reflects specific H2O2 signal. The rate of H2O2 production was presented as picomoles per milligram protein per minute after calculation, according to a standard curve generated using fresh H2O2 in reaction buffer.
RT-PCR Analysis of NOX mRNA Expression
Total RNA was extracted from the LAA using TRIzol (Invitrogen) according to the manufacturer’s instructions. Reverse transcription was performed in standard fashion with iScript cDNA synthesis Kit (Bio-Rad). The primer sequences used for PCR reactions are summarized in Table 1. The PCRs were carried out using an iCycler (Bio-Rad) with the protocol of [(95°C/2 min) (95°C/25 s, 57°C/5 s, 68°C 5 min) × 35 (72°C/10 min)]. The PCR mix contained GAPDH primers to generate GAPDH amplicon that served as an internal control.
HL-1 Cell Culture and Stimulation by Ang II
The HL-1 cells were obtained as generous gifts from Dr. William Claycomb from Louisiana State University that have been extensively characterized to maintain its differentiated cardiac phenotype using microscopic, genetic, immunohistochemical, electrophysiological, and pharmacological techniques (Claycomb et al., 1998; White et al., 2004). These beating cells were cultured following exact protocols as established by the Claycomb group and found to keep their phenotype during the 24 h exposure period to Ang II. The confluent cells were kept quiescent in media containing 0.01% FBS overnight and then stimulated with Ang II (100 nmol/L) for 24 h prior to analysis of NOX4 protein expression by Western blot (primary antibody from Abcam) and H2O2 production by Amplex Red assay. NOX4 mRNA expression from identically treated cells was determined by real-time RT-PCR.
Statistical Analysis
All data are presented as mean ± SEM. Differences in production, H2O2 production, and mRNA expression of different NOX isoforms between AF and non-AF groups were compared using t tests. ANCOVA were performed to examine the correlation between H2O2 and NOX4 mRNA expression in patients with and without AF. Statistical significance was set as p < 0.05.
Results
Patient Characteristics
Patient characteristics are summarized in Table 2. Eighteen patients with AF were older than those without (n = 17; p = 0.047). The gender distribution was not different. Both groups had similar incidence of co-existing cardiovascular conditions including hypertension, diabetes, and coronary artery disease. The percentages of patients receiving treatment of ACEI, AT1RB, calcium blocker or beta blocker were also similar between the two groups (Table 2). Of note, there was no significant difference in ejection fraction between the AF and non-AF groups. Whereas diastolic blood pressure, total cholesterol and triglyceride levels were not different in the AF vs. non-AF groups, systolic blood pressure (SBP) was higher in the AF patients (Table 2). ACEI-treated AF group had modestly higher SBP than those of non-AF group receiving ACEI (Table 3).
Reactive Oxygen Species Production
Among biologically relevant and abundant ROS, , and H2O2 appear to be most important in redox signaling. Whereas predominantly induces endothelial dysfunction by rapidly inactivating nitric oxide (), H2O2 influences different aspects of endothelial cell function via complex mechanisms. We hypothesize that and H2O2 also have differential roles in the regulation of cardiac functions. Both species were quantitatively and specifically determined by ESR and Amplex Red assay respectively. Whereas total production was not different between AF and non-AF groups (Figure 1A), we found that H2O2 production was more than doubled in AF patients compared to those without (149.8 ± 26.28 vs. 66.9 ± 7.14 pmol/mg/min, p = 0.0055, Figure 1B).
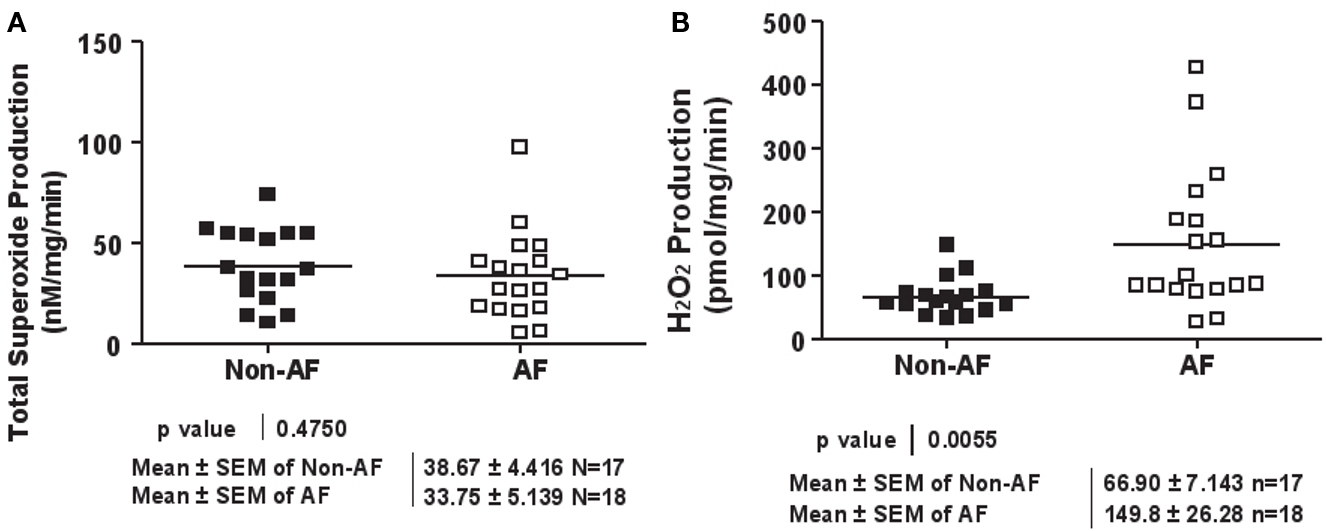
Figure 1. Superoxide and hydrogen peroxide production in patients with and without atrial fibrillation. (A) Total superoxide production. LAA were homogenated and subsequently analyzed for superoxide production using ESR. (B) Hydrogen peroxide production. LAA lysates were analyzed for hydrogen peroxide production using the Amplex Red assay.
H2O2 Production in AF Patients with Co-Existing Hypertension
Interestingly, AF patients with co-existing hypertension had approximately 3-fold higher H2O2 production compared to those without (239.0 ± 125.1 vs. 83.6 ± 51.3 pmol/mg/min, p = 0.003, Table 4). Older AF patients (>50) also had higher tissue levels of H2O2 compared to those younger AF patients (<50; 165 ± 118.8 vs. 95.0 ± 64.9 pmol/mg/min), although yet reaching statistical significance. In contrast, hypertension or age had no effect on H2O2 levels in patients without AF (Table 4).
Expression of Different NOX Isoforms and Its Relationship to H2O2
NOX4 is known to either produce H2O2 directly, or produce in subcellular organelles leaving membrane permeable H2O2 the sole detectable species (Martyn et al., 2006; Lambeth et al., 2007; Serrander et al., 2007; Chen et al., 2008; Block et al., 2009; Lassegue and Griendling, 2010). Other NOX isoforms however, have been shown to produce directly (Choi et al., 2008). Intriguingly, NOX4 mRNA expression was found significantly upregulated in AF patients (Figure 2A), which correlated well with a marked increase in H2O2 production (Figure 2B). The expression of NOX1 and NOX2 however, was not different in patients with and without AF (Figures 3A,B). NOX3 and NOX5 were not detectable in LAA. We did not find any correlation between ejection fractions and NOX4/H2O2 levels in both groups. This indicates that severity of heart failure is not linked to NOX4-H2O2 axis as AF does.
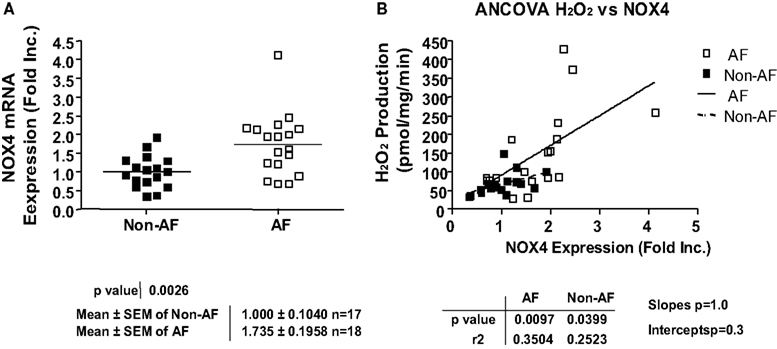
Figure 2. Upregulation of NOX4 expression and its correlation with hydrogen peroxide production in patients with atrial fibrillation. (A) NOX4 mRNA expression. NOX4 mRNA expression was determined by RT-PCR analysis and normalization to endogenous amplicon of GAPDH. (B) Correlation between NOX4 mRNA expression and hydrogen peroxide production.
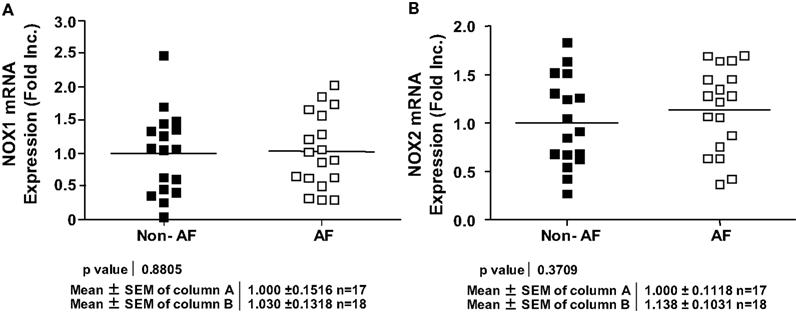
Figure 3. Expression of different NOX isoforms in patients with and without atrial fibrillation. (A) NOX1 mRNA expression. (B) NOX2 mRNA expression. NOX1 or NOX2 mRNA expression was determined by RT-PCR analysis and normalization to endogenous amplicon of GAPDH.
NOX4 Expression and H2O2 Production in Ang II-Stimulated HL-1 Atrial Cells
In additional experiments, we examined whether Ang II activates NOX4 in HL-1 atrial cells that have been well characterized to maintain a differentiated, adult cardiomyocyte and atrial cell-like phenotype. In our hand we have also been able to reproduce the culture condition and observe the beating HL-1 cells following the published Claycomb conditions. Of note, the Ang II receptor AT1 was abundantly expressed in these cells, and it was upregulated by Ang II (Figures 4A,C). Ang II stimulation resulted in upregulation of NOX4 protein expression and an associated increase in H2O2 production (Figures 4A,B,D). The NOX4 mRNA expression, determined by real-time RT-PCR, was also found upregulated by Ang II in HL-1 cells (Figure 4E).
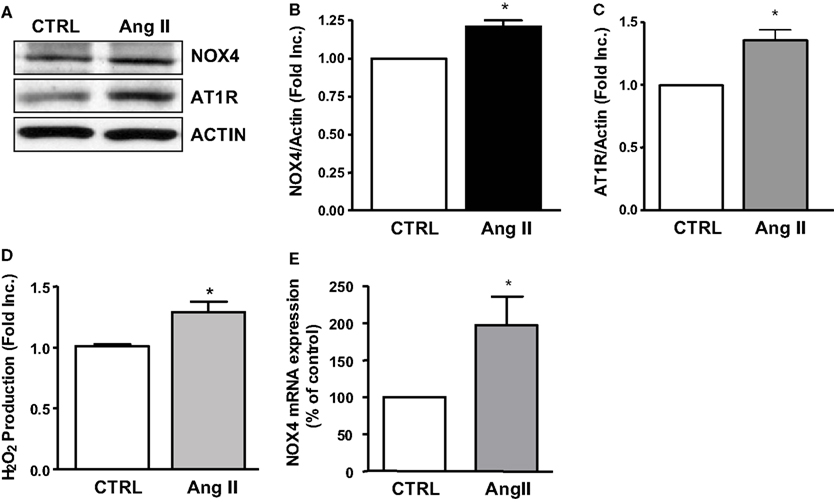
Figure 4. NOX4, AT1R expression and hydrogen peroxide production in angiotensin II treated HL-1 atrial cells. (A) Representative Western blots of NOX4 and AT1R expression in Ang II treated HL-1 cells. (B) Densitometic grouped data of NOX4 expression in Ang II treated HL-1 cells. (C) Densitometic grouped data of NOX4 expression in Ang II treated HL-1 cells. (D) Hydrogen peroxide production in Ang II treated HL-1 cells. (E) NOX4 mRNA expression in Ang II treated HL-1 cells. Data are represented as Mean ± SEM (N = 4, *p < 0.05 vs. CTRL).
Discussion
In the present study, we have discovered that NOX4-derived H2O2 production is markedly increased in the LAA tissues of AF patients. Hydrogen peroxide production in LAA was 3-fold higher in AF patients with co-existing hypertension than those without. Hypertension is an established risk factor for AF. Recent studies have indicated that H2O2 regulates electrical properties of isolated rabbit pulmonary vein and atrial cells (Lin et al., 2010). Together with the previously characterized role of H2O2 in myocardial fibrosis, these data suggest that NOX4-derived H2O2 may play an important role in the development of AF. Indeed, in additional studies utilizing HL-1 atrial cells, we found that Ang II stimulation led to NOX4 upregulation and increased H2O2 production, further implicating a potential role of NOX4-derived H2O2 in atrial structural remodeling that is considered an important initiating process of AF.
Aging and hypertension are the best-characterized, potential causal factors for AF. Of significant interest, we found that AF patients with co-existing hypertension had 3-folds higher H2O2 production compared to those without AF. AF patients that are older (>50) also had apparently higher H2O2 production. These observations may further suggest that augmented local cardiac production of H2O2 is involved in arrhythmogenesis, besides its proinflammatory and prothrombotic effects. On the other hand, AF may have made SBP control more difficult (ACEI-AF group had higher SBP than ACEI-non-AF group), which is likely related to the substantially higher H2O2 production in hypertensive AF patients. Although H2O2 can mediate compensatory relaxation under certain conditions such as in the apolipoprotein E deficient atherosclerotic mice (Landmesser et al., 2003), excessive H2O2 is involved in vascular remodeling which could in turn result in stiffer blood vessels to deteriorate hypertension.
We found that LAA production was not different in patients with and without AF. We also found that xanthine oxidase derived was not different between the two groups (data not shown). Xanthine oxidase has been previously shown to play an important role in cardiac production in patients with heart failure. However its role in AF patients has never been previously tested. It is interesting to speculate whereas xanthine oxidase and/or other NOX isoforms is more important for production in heart failure patients, NOX4-derived H2O2 production is more important in the condition of AF. Of course, this hypothesis needs to be further investigated using larger patient population. Potential regulations of ROS scavenging enzymes such as superoxide dismutases and glutathione peroxidases, under the environment of NOX4-dependent H2O2 overproduction, would also be interesting to be further investigated.
Our data revealed an innovative role of NOX4 in H2O2 production in AF patients. Previously NOX1 and NOX4 were found undetectable in RAA (Kim et al., 2005). In human LAA, however, we were able to detect abundant NOX1, NOX2, and NOX4 mRNA expression. Only NOX4 mRNA expression was upregulated by AF, which correlated well with overproduction of H2O2 in AF patients. Accumulating evidence have characterized the relatively newly recognized NOX4 and its ROS-producing activity in various cell types such as endothelial cells (Ago et al., 2004, 2005), vascular smooth muscle (Lassegue et al., 2001; Wingler et al., 2001; Touyz et al., 2002), and kidney cells (Shiose et al., 2001; Wingler et al., 2001; Chabrashvili et al., 2002). Functionally, NOX4 has been implicated in cell differentiation (Clempus et al., 2007; Ismail et al., 2009; Schroder et al., 2009; Xiao et al., 2009), cell cycle transition (Sturrock et al., 2007), transcriptional activation (Bonello et al., 2007), and myofibroblast activation and fibrogenic responses (Hecker et al., 2009). In the heart, NOX4 has been shown to be involved in hypertrophy induced cardiac apoptosis and mitochondrial dysfunction (Ago et al., 2010), and pressure overload-induced heart failure (Byrne et al., 2003; Kuroda et al., 2010). Our data add to this rapidly growing knowledge of NOX4 functions, demonstrating a potential role of NOX4 in enhancing H2O2 production in patients with AF, which might be involved in the pathogenesis of AF. Of note, recent studies have demonstrated an important role of myocardial fibrosis in AF (Everett and Olgin, 2007). Therefore, H2O2 may also contribute to AF via augmentation of myocardial fibrosis as recently reported (Morita et al., 2009).
HL-1 cardiomyocytes, derived from atrial cardiac muscle cells from the AT1 mouse atrial cardiomyocyte tumor lineage, maintains the differentiated adult cardiomyocyte phenotype (Claycomb et al., 1998). This cell has been proved to possess the organized sarcomere, ion channels, functional receptors, and intracellular signaling proteins so that it contracts spontaneously, divides, generates action potential, and responds to agonists (White et al., 2004). Therefore, HL-1 cardiomyocyte has been established as the useful tool to study the cellular and molecular mechanisms underlying cardiac pathophysiologies (Rodriguez-Sinovas et al., 2005; Brady et al., 2006; Barba et al., 2009). In particular, expression of renin–angiotensin system components and Ang II regulation of L-type calcium channel have been characterized in HL-1 cells (Tsai et al., 2007, 2008). Moreover, transcriptional profile regulated by rapid electrical stimulation in HL-1 cells was similar to those from human AF (Mace et al., 2009). Therefore, we employed HL-1 cells as the cellular model to investigate whether NOX4 is modulated by Ang II, which has been shown to modulate atrial remodeling and fibrillation. We found AT1R was expressed in HL-1 cells and further upregulated by Ang II treatment. Most importantly, exposure of HL-1 cells to Ang II resulted in increased NOX4 mRNA and protein expression, without affecting NOX1 and NOX2 mRNA levels. This was accompanied by a significant elevation in H2O2 production.
In summary, our data have uncovered a NOX4-mediated overproduction of H2O2 in patients with AF. AF patients with hypertension had markedly higher LAA H2O2 levels compared to those without AF. In addition, H2O2 has been recently reported to regulate electrical properties of pulmonary vein and atrial cells, where the initiation of ectopic beats is believed to occur to induce AF. It is also known to promote myocardial fibrosis. The modulator of atrial remodeling and fibrosis, Ang II, also significantly upregulated NOX4 expression and H2O2 production in atrial HL-1 cells. Taken together, these data suggest that NOX4 may play an important role in the pathogenesis of AF.
Conflict of Interest Statement
The authors declare that the research was conducted in the absence of any commercial or financial relationships that could be construed as a potential conflict of interest.
Acknowledgments
The authors’ work has been supported by National Heart, Lung and Blood Institute (NHLBI) Grant HL077440 (Hua Cai), HL081571 (Hua Cai), HL088975 (Hua Cai), HL101228 (Hua Cai, PP Ping, JN Weiss) and HL108701 (Hua Cai, DG Harrison).
References
Ago, T., Kitazono, T., Kuroda, J., Kumai, Y., Kamouchi, M., Ooboshi, H., Wakisaka, M., Kawahara, T., Rokutan, K., Ibayashi, S., and Iida, M. (2005). NAD(P)H oxidases in rat basilar arterial endothelial cells. Stroke 36, 1040–1046.
Ago, T., Kitazono, T., Ooboshi, H., Iyama, T., Han, Y. H., Takada, J., Wakisaka, M., Ibayashi, S., Utsumi, H., and Iida, M. (2004) Nox4 as the major catalytic component of an endothelial NAD(P)H oxidase. Circulation 109, 227–233.
Ago, T., Kuroda, J., Pain, J., Fu, C., Li, H., and Sadoshima, J. (2010). Upregulation of Nox4 by hypertrophic stimuli promotes apoptosis and mitochondrial dysfunction in cardiac myocytes. Circ. Res. 106, 1253–1264.
Barba, I., Chavarria, L., Ruiz-Meana, M., Mirabet, M., Agullo, E., and Garcia-Dorado, D. (2009). Effect of intracellular lipid droplets on cytosolic Ca2+ and cell death during ischaemia-reperfusion injury in cardiomyocytes. J. Physiol. (Lond.) 587, 1331–1341.
Block, K., Gorin, Y., and Abboud, H. E. (2009). Subcellular localization of Nox4 and regulation in diabetes. Proc. Natl. Acad. Sci. U.S.A. 106, 14385–14390.
Bonello, S., Zahringer, C., BelAiba, R. S., Djordjevic, T., Hess, J., Michiels, C., Kietzmann, T., and Gorlach, A. (2007). Reactive oxygen species activate the HIF-1alpha promoter via a functional NFkappaB site. Arterioscler. Thromb. Vasc. Biol. 27, 755–761.
Brady, N. R., Hamacher-Brady, A., and Gottlieb, R. A. (2006). Proapoptotic BCL-2 family members and mitochondrial dysfunction during ischemia/reperfusion injury, a study employing cardiac HL-1 cells and GFP biosensors. Biochim. Biophys. Acta 1757, 667–678.
Byrne, J. A., Grieve, D. J., Bendall, J. K., Li, J. M., Gove, C., Lambeth, J. D., Cave, A. C., and Shah, A. M. (2003). Contrasting roles of NADPH oxidase isoforms in pressure-overload versus angiotensin II-induced cardiac hypertrophy. Circ. Res. 93, 802–805.
Cai, H. (2005a). Hydrogen peroxide regulation of endothelial function: mechanisms, consequences and origins. Cardiovasc. Res. 68, 26–36.
Cai, H. (2005b). NAD(P)H oxidase-dependent self-propagation of hydrogen peroxide and vascular disease. Circ. Res. 96, 818–822.
Cai, H., Griendling, K. K., and Harrison, D. G. (2003). The vascular NAD(P)H oxidases as therapeutic targets in cardiovascular diseases. Trends Pharmacol. Sci. 24, 471–478.
Cai, H., and Harrison, D. G. (2000). Endothelial dysfunction in cardiovascular diseases: the role of oxidant stress. Circ. Res. 87, 840–844.
Caouette, D., Dongmo, C., Berube, J., Fournier, D., and Daleau, P. (2003). Hydrogen peroxide modulates the Kv1.5 channel expressed in a mammalian cell line. Naunyn Schmiedebergs Arch. Pharmacol. 368, 479–486.
Chabrashvili, T., Tojo, A., Onozato, M. L., Kitiyakara, C., Quinn, M. T., Fujita, T., Welch, W. J., and Wilcox, C. S. (2002). Expression and cellular localization of classic NADPH oxidase subunits in the spontaneously hypertensive rat kidney. Hypertension 39, 269–274.
Chalupsky, K., and Cai, H. (2005). Endothelial dihydrofolate reductase: critical for nitric oxide bioavailability and role in angiotensin II uncoupling of endothelial nitric oxide synthase. Proc. Natl. Acad. Sci. U.S.A. 102, 9056–9061.
Chen, K., Kirber, M. T., Xiao, H., Yang, Y., and Keaney, J. F. Jr. (2008). Regulation of ROS signal transduction by NADPH oxidase 4 localization. J. Cell Biol. 181, 1129–1139.
Choi, H., Leto, T. L., Hunyady, L., Catt, K. J., Bae, Y. S., and Rhee, S. G. (2008). Mechanism of angiotensin II-induced superoxide production in cells reconstituted with angiotensin type 1 receptor and the components of NADPH oxidase. J. Biol. Chem. 283, 255–267.
Chou, C. C., and Chen, P. S. (2009). New concepts in atrial fibrillation: neural mechanisms and calcium dynamics. Cardiol. Clin. 27, 35–33.
Claycomb, W. C., Lanson, N. A. Jr., Stallworth, B. S., Egeland, D. B., Delcarpio, J. B., Bahinski, A., and Izzo, N. J. Jr. (1998). HL-1 cells: a cardiac muscle cell line that contracts and retains phenotypic characteristics of the adult cardiomyocyte. Proc. Natl. Acad. Sci. U.S.A. 95, 2979–2984.
Clempus, R. E., Sorescu, D., Dikalova, A. E., Pounkova, L., Jo, P., Sorescu, G. P., Schmidt, H. H., Lassegue, B., and Griendling, K. K. (2007). Nox4 is required for maintenance of the differentiated vascular smooth muscle cell phenotype. Arterioscler. Thromb. Vasc. Biol. 27, 42–48.
Everett, T. H., and Olgin, J. E. (2007). Atrial fibrosis and the mechanisms of atrial fibrillation. Heart Rhythm 4, S24–S27.
Gao, L., Chalupsky, K., Stefani, E., and Cai, H. (2009a). Mechanistic insights into folic acid-dependent vascular protection: dihydrofolate reductase (DHFR)-mediated reduction in oxidant stress in endothelial cells and angiotensin II-infused mice. A Novel HPLC-based Fluorescent Assay for DHFR Activity. J. Mol. Cell. Cardiol. 47, 752–760.
Gao, L., Pung, Y. F., Zhang, J., Chen, P., Wang, T., Li, M., Meza, M., Toro, L., and Cai, H. (2009b). Sepiapterin reductase regulation of endothelial tetrahydrobiopterin and nitric oxide bioavailability. Am. J. Physiol. Heart Circ. Physiol. 297, H331–H339.
Healey, J. S., Baranchuk, A., Crystal, E., Morillo, C. A., Garfinkle, M., Yusuf, S., and Connolly, S. J. (2005). Prevention of atrial fibrillation with angiotensin-converting enzyme inhibitors and angiotensin receptor blockers: a meta-analysis. J. Am. Coll. Cardiol. 45, 1832–1839.
Hecker, L., Vittal, R., Jones, T., Jagirdar, R., Luckhardt, T. R., Horowitz, J. C., Pennathur, S., Martinez, F. J., and Thannickal, V. J. (2009). NADPH oxidase-4 mediates myofibroblast activation and fibrogenic responses to lung injury. Nat. Med. 15, 1077–1081.
Ismail, S., Sturrock, A., Wu, P., Cahill, B., Norman, K., Huecksteadt, T., Sanders, K., Kennedy, T., and Hoidal, J. (2009). NOX4 mediates hypoxia-induced proliferation of human pulmonary artery smooth muscle cells: the role of autocrine production of transforming growth factor-{beta}1 and insulin-like growth factor binding protein-3. Am. J. Physiol. Lung Cell Mol. Physiol. 296, L489–L499.
Kannel, W. B., Wolf, P. A., Benjamin, E. J., and Levy, D. (1998). Prevalence, incidence, prognosis, and predisposing conditions for atrial fibrillation: population-based estimates. Am. J. Cardiol. 82, 2N–9N.
Kim, Y. H., Lee, J. H., Lim do, S., Shim, W. J., Ro, Y. M., Park, G. H., Becker, K. G., Cho-Chung, Y. S., and Kim, M. K. (2003). Gene expression profiling of oxidative stress on atrial fibrillation in humans. Exp. Mol. Med. 35, 336–349.
Kim, Y. M., Guzik, T. J., Zhang, Y. H., Zhang, M. H., Kattach, H., Ratnatunga, C., Pillai, R., Channon, K. M., and Casadei, B. (2005). A myocardial Nox2 containing NAD(P)H oxidase contributes to oxidative stress in human atrial fibrillation. Circ. Res. 97, 629–636.
Kuroda, J., Ago, T., Matsushima, S., Zhai, P., Schneider, M. D., and Sadoshima, J. (2010). NADPH oxidase 4 (Nox4) is a major source of oxidative stress in the failing heart. Proc. Natl. Acad. Sci. U.S.A. 107, 15565–15570.
Lambeth, J. D., Kawahara, T., and Diebold, B. (2007). Regulation of Nox and Duox enzymatic activity and expression. Free Radic. Biol. Med. 43, 319–331.
Landmesser, U., Dikalov, S., Price, S. R., McCann, L., Fukai, T., Holland, S. M., Mitch, W. E., and Harrison, D. G. (2003). Oxidation of tetrahydrobiopterin leads to uncoupling of endothelial cell nitric oxide synthase in hypertension. J. Clin. Invest. 111, 1201–1209.
Lassegue, B., and Griendling, K. K. (2010). NADPH oxidases: functions and pathologies in the vasculature. Arterioscler. Thromb. Vasc. Biol. 30, 653–661.
Lassegue, B., Sorescu, D., Szocs, K., Yin, Q., Akers, M., Zhang, Y., Grant, S. L., Lambeth, J. D., and Griendling, K. K. (2001). Novel gp91(phox) homologues in vascular smooth muscle cells: nox1 mediates angiotensin II-induced superoxide formation and redox-sensitive signaling pathways. Circ. Res. 88, 888–894.
Lin, Y. K., Lin, F. Z., Chen, Y. C., Cheng, C. C., Lin, C. I., Chen, Y. J., and Chen, S. A. (2010). Oxidative stress on pulmonary vein and left atrium arrhythmogenesis. Circ. J. 74, 1547–1556.
Lu, G., Sun, H., Korge, P., Koehler, C. M., Weiss, J. N., and Wang, Y. (2009). Functional characterization of a mitochondrial Ser/Thr protein phosphatase in cell death regulation. Meth. Enzymol. 457, 255–273.
Mace, L. C., Yermalitskaya, L. V., Yi, Y., Yang, Z., Morgan, A. M., and Murray, K. T. (2009). Transcriptional remodeling of rapidly stimulated HL-1 atrial myocytes exhibits concordance with human atrial fibrillation. J. Mol. Cell. Cardiol. 47, 485–492.
Martyn, K. D., Frederick, L. M., von Loehneysen, K., Dinauer, M. C., and Knaus, U. G. (2006). Functional analysis of Nox4 reveals unique characteristics compared to other NADPH oxidases. Cell. Signal. 18, 69–82.
Morita, N., Sovari, A. A., Xie, Y., Fishbein, M. C., Mandel, W. J., Garfinkel, A., Lin, S. F., Chen, P. S., Xie, L. H., Chen, F., Qu, Z., Weiss, J. N., and Karagueuzian, H. S. (2009). Increased susceptibility of aged hearts to ventricular fibrillation during oxidative stress. Am. J. Physiol. Heart Circ. Physiol. 297, H1594–H1605.
Nakashima, H., Kumagai, K., Urata, H., Gondo, N., Ideishi, M., and Arakawa, K. (2000). Angiotensin II antagonist prevents electrical remodeling in atrial fibrillation. Circulation 101, 2612–2617.
Oak, J. H., and Cai, H. (2007). Attenuation of angiotensin II signaling recouples eNOS and inhibits nonendothelial NOX activity in diabetic mice. Diabetes 56, 118–126.
Rodriguez-Sinovas, A., Garcia-Dorado, D., Pina, P., Ruiz-Meana, M., and Soler-Soler, J. (2005). Effect of sarcolemmal rupture on myocardial electrical impedance during oxygen deprivation. Am. J. Physiol. Heart Circ. Physiol. 288, H1396–H1403.
Savelieva, I., and Camm, J. (2008). Update on atrial fibrillation: part I. Clin. Cardiol. 31, 55–62.
Schroder, K., Wandzioch, K., Helmcke, I., and Brandes, R. P. (2009). Nox4 acts as a switch between differentiation and proliferation in preadipocytes. Arterioscler. Thromb. Vasc. Biol. 29, 239–245.
Serrander, L., Cartier, L., Bedard, K., Banfi, B., Lardy, B., Plastre, O., Sienkiewicz, A., Forro, L., Schlegel, W., and Krause, K. H. (2007). NOX4 activity is determined by mRNA levels and reveals a unique pattern of ROS generation. Biochem. J. 406, 105–114.
Shiose, A., Kuroda, J., Tsuruya, K., Hirai, M., Hirakata, H., Naito, S., Hattori, M., Sakaki, Y., and Sumimoto, H. (2001). A novel superoxide-producing NAD(P)H oxidase in kidney. J. Biol. Chem. 276, 1417–1423.
Sturrock, A., Huecksteadt, T. P., Norman, K., Sanders, K., Murphy, T. M., Chitano, P., Wilson, K., Hoidal, J. R., and Kennedy, T. P. (2007). Nox4 mediates TGF-beta1-induced retinoblastoma protein phosphorylation, proliferation, and hypertrophy in human airway smooth muscle cells. Am. J. Physiol. Lung Cell Mol. Physiol. 292, L1543–L1555.
Tan, A. Y., and Zimetbaum, P. (2011). Atrial fibrillation and atrial fibrosis. J. Cardiovasc. Pharmacol. 57, 625–629.
Touyz, R. M., Chen, X., Tabet, F., Yao, G., He, G., Quinn, M. T., Pagano, P. J., and Schiffrin, E. L. (2002). Expression of a functionally active gp91phox-containing neutrophil-type NAD(P)H oxidase in smooth muscle cells from human resistance arteries: regulation by angiotensin II. Circ. Res. 90, 1205–1213.
Tsai, C. T., Lai, L. P., Hwang, J. J., Chen, W. P., Chiang, F. T., Hsu, K. L., Tseng, C. D., Tseng, Y. Z., and Lin, J. L. (2008). Renin-angiotensin system component expression in the HL-1 atrial cell line and in a pig model of atrial fibrillation. J. Hypertens. 26, 570–582.
Tsai, C. T., Wang, D. L., Chen, W. P., Hwang, J. J., Hsieh, C. S., Hsu, K. L., Tseng, C. D., Lai, L. P., Tseng, Y. Z., Chiang, F. T., and Lin, J. L. (2007). Angiotensin II increases expression of alpha1C subunit of L-type calcium channel through a reactive oxygen species and cAMP response element-binding protein-dependent pathway in HL-1 myocytes. Circ. Res. 100, 1476–1485.
White, S. M., Constantin, P. E., and Claycomb, W. C. (2004). Cardiac physiology at the cellular level: use of cultured HL-1 cardiomyocytes for studies of cardiac muscle cell structure and function. Am. J. Physiol. Heart Circ. Physiol. 286, H823–H829.
Wingler, K., Wunsch, S., Kreutz, R., Rothermund, L., Paul, M., and Schmidt, H. H. (2001). Upregulation of the vascular NAD(P)H-oxidase isoforms Nox1 and Nox4 by the renin-angiotensin system in vitro and in vivo. Free Radic. Biol. Med. 31, 1456–1464.
Xiao, Q., Luo, Z., Pepe, A. E., Margariti, A., Zeng, L., and Xu, Q. (2009). Embryonic stem cell differentiation into smooth muscle cells is mediated by Nox4-produced H2O2. Am. J. Physiol. Cell Physiol. 296, C711–C723.
Keywords: atrial fibrillation, hydrogen peroxide, NADPH oxidase, NOX4, hypertension, HL-1 cells, angiotensin II
Citation: Zhang J, Youn JY, Kim AY, Ramirez RJ, Gao L Ngo D, Chen P, Scovotti J, Mahajan A and Cai H (2012) NOX4-dependent hydrogen peroxide overproduction in human atrial fibrillation and HL-1 atrial cells: relationship to hypertension. Front. Physio. 3:140. doi: 10.3389/fphys.2012.00140
Received: 13 March 2012; Accepted: 26 April 2012;
Published online: 05 June 2012.
Edited by:
Jeannette Vasquez-Vivar, Medical College of Wisconsin, USAReviewed by:
Grant R. Drummond, Monash University, AustraliaFeng Chen, Georgia Health Sciences University, USA
Copyright: © 2012 Zhang, Youn, Kim, Ramirez, Gao, Ngo, Chen, Scovotti, Mahajan and Cai. This is an open-access article distributed under the terms of the Creative Commons Attribution Non Commercial License, which permits non-commercial use, distribution, and reproduction in other forums, provided the original authors and source are credited.
*Correspondence: Hua Cai, Division of Molecular Medicine, Cardiovascular Research Laboratories, Departments of Anesthesiology and Medicine, David Geffen School of Medicine at University of California Los Angeles, 650 Charles E. Young Drive, Los Angeles, CA 90095, USA. e-mail: hcai@mednet.ucla.edu