- Department of Physiology, Otago School of Medical Sciences, University of Otago, Dunedin, New Zealand
A commentary on
Active transport of sodium as the source of electric current in the short-circuited isolated frog skin
by Ussing, H. H., and Zerahn, R. (1951). Acta Physiol. Scand. 23, 110–127.
Generally, a Commentary for the Frontiers in Physiology is aimed at a recent research article that has the potential to make a major impact on a particular scientific research field. However, where would the field of epithelial ion transport physiology be without the Ussing chamber? One has to ask that question in the 100th anniversary year of the birth of the great Danish physiologist Hans Henriksen Ussing and his famous “Little Chamber”; which is now over 60 years old.
The monumental works of Ussing (1911–2000), starting nearly 65 years ago, ushered in the “age of epithelial ion transport physiology,” which is still flourishing today in numerous epithelial physiology laboratories throughout the world. Indeed, Lindemann (2001) epitomized Ussing’s contribution to science in four simple words “…founder of epithelial transport….” Palmer and Andersen (2008) paid homage to Ussing by writing “…In a sense the field of epithelial polarity began in 1958 with the Koefoed-Johnsen and Ussing paper.”
The focus of this Commentary is to highlight the classic paper of Hans Ussing in which he used his now famous “Little Chamber” and the implementation of the fledgling short-circuit current technique to define the active transport of sodium as the source of electric current across the isolated frog skin (Ussing and Zerahn, 1951). This single paper introduced a new research direction that has led to our understanding of many cellular models of ion transport physiology in numerous epithelial tissues of the body. Notably, to quickly appreciate the impact of Ussing’s work, if one types in “Ussing” into PubMed over 2,975 citations are identified at the writing of this manuscript. There are undoubtedly hundreds, if not thousands; of other research papers in which the Ussing chamber technique has been used but not captured by scientific search indices. The reader is referred to recent reviews for the historical prospective of Ussing and technical details of the Ussing chamber short-circuit technique by Larsen (2002), Palmer and Andersen (2008), and Clarke (2009).
Prior to the 1951 paper, Ussing had established that the influx of Na+ transport across the frog skin was higher than the out flux of Na+ using double labeling with Na24 and Na22, respectively (Levi and Ussing, 1949). Ussing’s introduction of his Ussing chamber (Ussing and Zerahn, 1951) and implementation of the short-circuit current technique in which he mounted an isolated frog skin epithelium between two fluid-filled chambers (Figure 1, essentially the same design as used today; http://www.ussingchamber.com/) was the beginning of a new age of research. This allowed the first investigation of ion (Na+, in this case) transport physiology of an epithelium. Mounting the frog skin epithelium between the fluid-filled chambers allowed physical access to either side of the epithelial layer for testing modulators of ion transport. Indeed, Ussing and Zerahn used copper, adrenaline and a “neurohypophyseal extract” to examine the modulation of Na24 transport and the short-circuit current of the frog skin. Ussing and Zerahn, using the short-circuited technique, were able to short-circuit the tissues by driving (voltage clamping) any transepithelial current generated back to zero. This current was indicative of active transport determined by the net transport processes. Ultimately, Ussing and Zerahn demonstrated that the short-circuit current of the frog skin was essentially determined by the active transport of Na+ across the frog skin epithelium.
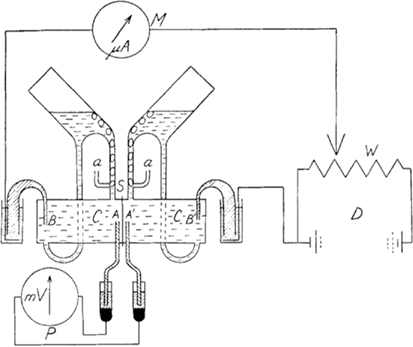
Figure 1. Diagram of the Ussing chamber apparatus. Abbreviations are: A and A′ are voltage-sensing electrodes; a are the air lines to aerate the frog skin; B and B′ are current-passing electrodes; C are the two half-chambers; S is where the skin is inserted between the half-chambers; D is the battery and W the potential divider that are used to adjust (by using current) the voltage across the skin to be equal to zero (i.e., short-circuited) as measured by P the potentiometer (voltmeter); any current-passing the skin while the skin is short-circuited is measured by M the microammeter and is the short-circuited current (μA), thus, active transport. The reproduction of Figure 1 from Ussing and Zerahn (1951) is with kind permission from John Wiley and Sons.
There are five important advances from Ussing and Zerahn’s (1951) paper. First, Ussing and Zerahn applied the short-circuit technique to an epithelial tissue preparation. Secondly, they describe the apparatus (the Ussing chamber) that enabled them to determine the simultaneous measurement of Na+ transport and electrical current through the frog skin. Third, Ussing and Zerahn bathed the frog skin with identical solutions (high NaCl Ringer’s solution), consequently, there would be no passive ion flow. Therefore, ions that are moved by active transport will continue and the observed short-circuit current will result from a net transport of those ions. Fourth, Ussing and Zerahn developed a hypothesis in which it is possible to calculate the electromotive force for Na+ and also the resistance to the Na+ current from the efflux of Na+ and the short-circuit current. Finally, they reported that the short-circuit current of the frog skin was essentially the net active transport of Na+ based on the Na24 experiments compared with the short-circuit current measurements. In other words, Na+ influx across the entire frog skin epithelium (from pond water to the blood) dominated the Na+ efflux with very little Na+ being transported from the blood to the pond water.
Implications of the 1951 Paper
The initial description of Na+ being the electrical basis of the short-circuit current led Ussing to propose the cellular model for Na+ absorption across the frog skin epithelium which was the subject of his classic 1958 paper (Koefoed-Johnsen and Ussing, 1958). Recently, Palmer and Andersen (2008) eloquently reviewed the 1958 paper.
Briefly, the essential feature of Ussing’s cellular model of Na+ absorption of epithelia is that the epithelial cell (a black box) is viewed as two membranes arranged in series separated by an intracellular compartment. The anatomy of epithelial cells is very different than muscle or nerve cells. We now know that epithelial cells are polarized, in that, the transport proteins that reside in the apical membrane are quite different from those of the basolateral membrane. These transport proteins function, in concert, to perform a specific transport task such as Na+ reabsorption by the collecting duct cell of the nephron, for example. Ussing’s initial work with the frog skin has led over two generation of scientists to understand this concept of “polarized” cells. Ussing and Koefoed-Johnsen predicted that Na+ moved across “the outer” surface (apical membrane) of the frog skin passively, however, that transport was highly selective. Therefore, Na+ moves into the cell down its concentration gradient. They suggested that the active transport of Na+ was located at the “inward facing membrane” (basolateral) of the epithelial cell. Additionally, Koefoed-Johnsen and Ussing wrote that a “…forced exchange of Na+ against K+…” (i.e., Na+/K+-ATPase) occurred across the basolateral membrane and there was a pathway for K+ to exit the cell across the basolateral membrane. Interestingly, Ussing (1947) had previously suggested that Na+ moved against its own concentration gradient and an electrical potential. The Na+/K+-ATPase maintains the low cellular concentration of Na+ and high extracellular Na+ concentration to help maintain the driving force for Na+ entry across the apical membrane (outer membrane of the frog skin).
Koefoed-Johnsen and Ussing’s cellular model of epithelial Na+ absorption has been repeatedly confirmed and extended in numerous epithelial tissues over the years. Tissues examined include amphibian and mammalian urinary bladder, distal colon, various segments of the mammalian distal nephron, respiratory epithelia, sweat ducts, and numerous cultured cell lines derived from various tight absorptive epithelia tissues.
Ultimately, Ussing’s (Koefoed-Johnsen and Ussing, 1958) suggestion of “…sodium movement through the outer border is passive, but highly selective…” was further strengthened by fluctuation analysis experiments by Lindemann and Van Driessche (1977) in which they proposed sodium- specific membrane channels blocked by amiloride in frog skin. However, the experimental evidence of amiloride-inhibitable epithelial Na+ channels was finally confirmed by patch-clamp studies by Hamilton and Eaton (1985, 1986) and Palmer and Frindt (1986). Over 35 years after Koefoed-Johnsen and Ussing published their model, molecular studies by Canessa et al. (1993, 1994) identified the genes for the epithelial Na+ channel, now simply known as ENaC.
Even in the molecular age of epithelial ion transport physiology, scientists still return to the Ussing chamber short-circuit current technique to demonstrate functional expression of channel proteins (Na+ absorption; Cl− and K+ secretion, for example) and electrogenic transporter proteins (i.e., Na+-solute co-transporters, Na+-amino acid co-transporters) and even electroneutral transporters (i.e., propionate absorption). The simplicity of the Ussing chamber short-circuit current technique lends itself to the investigation of many aspects of epithelial ion transport physiology.
Concluding Thoughts
It is difficult to imagine where the field of epithelial physiology would be in the twenty-first century had Ussing not decided to recreate the “pond water environment” of the frog with his fluid-filled chambers. We have gone from the “black box” of an epithelial cell of the 1950s to describing many intricate ion transport/cellular mechanisms and signaling pathways that modulate ion transport physiology of epithelia during the past 60 years. It is very exciting to dream about the future advances in epithelial transport physiology. Ussing left us his “Little Chamber” and with many thoughts about science including the following “…Be always prepared to reject or modify a hypothesis, if an experiment shows something unexpected.”
Acknowledgments
I thank Dr. Steven Condliffe and two anonymous reviewers for comments on this manuscript. This work was supported by a NZ Lottery Board grant and the Department of Physiology, University of Otago.
References
Canessa, C. M., Schild, L., Buell, G., Thorens, B., Gautschi, I., Horisberger, J. D., and Rossier, B. C. (1994). Amiloride-sensitive epithelial Na+ channel is made of three homologous subunits. Nature 367, 463–467.
Canessa, C. M., Horisberger, J. D., and Rossier, B. C. (1993). Epithelial sodium channel related to proteins involved in neurodegeneration. Nature 361, 467–470.
Clarke, L. L. (2009). A guide to Ussing chamber studies of mouse intestine. Am. J. Physiol. Gastrointest. Liver Physiol. 296, G1151–G1166.
Hamilton, K. L., and Eaton, D. C. (1985). Single recordings from amiloride-sensitive sodium channels. Am. J. Physiol. 249, C200–C207.
Hamilton, K. L., and Eaton, D. C. (1986). Regulation of single sodium channels in renal tissue: a role in sodium homeostasis. Fed. Proc. 45, 2713–2717.
Koefoed-Johnsen, V., and Ussing, H. H. (1958). The nature of the frog skin potential. Acta Physiol. Scand. 42, 298–308.
Larsen, E. H. (2002). Hans H. Ussing-scientific work: contemporary significance and perspectives. Biochim. Biophys. Acta 1566, 2–15.
Levi, H., and Ussing, H. H. (1949). Resting potential and ion movements in the frog skin. Nature 164, 928.
Lindemann, B., and Van Driessche, W. (1977). Sodium-specific membrane channels of frog skin pores: current fluctuations reveal high turnover. Science 195, 292–294.
Palmer, L. G., and Andersen, O. S. (2008). The two- membrane model of epithelial transport: Koefoed-Johnsen and Ussing (1958). J. Gen. Physiol. 132, 607–617.
Palmer, L. G., and Frindt, G. (1986). Amiloride-sensitive Na+ channels from the apical membrane of the rat cortical collecting tubule. Proc. Natl. Acad. Sci. U.S.A. 83, 2767–2770.
Ussing, H. H. (1947). Interpretation of the exchange of radio-sodium in isolated muscle. Nature 160, 262–263.
Citation: Hamilton KL (2011) Ussing’s “little chamber”: 60 years+ old and counting. Front. Physio. 2:6. doi: 10.3389/fphys.2011.00006
Received: 17 January 2011;
Accepted: 14 February 2011;
Published online: 03 March 2011.
Copyright: © 2011 Hamilton. This is an open-access article subject to an exclusive license agreement between the authors and Frontiers Media SA, which permits unrestricted use, distribution, and reproduction in any medium, provided the original authors and source are credited.
*Correspondence: kirk.hamilton@otago.ac.nz