- 1 Department of Anthropology, University of North Carolina at Chapel Hill, Chapel Hill, NC, USA
- 2 Carolina Population Center, University of North Carolina at Chapel Hill, Chapel Hill, NC, USA
- 3 Department of Anthropology, Emory University, Atlanta, GA, USA
- 4 Departments of Pharmacology and Medicine, University of Virginia Health System, Charlottesville, VA, USA
While the activation of the infant hypothalamic–pituitary–gonadal axis and the existence of a postnatal gonadotropin surge were first documented in the early 1970s, study of the longitudinal development of gonadal hormones in infancy, and the potential physiological and behavioral correlates of this development, have been hampered by reliance on infrequent serum sampling. The present study reports the validation of a non-invasive method for repeated assessment of steroid hormones in infant fecal samples. Fecal samples were collected in and excised from cotton diaper liners and extracted using methanol. Extracts were analyzed for estradiol using a diluted assay modification. Method validity was supported by a steroid recovery rate of at least 80%, a sensitivity of 0.35 pg/ml, and inter- and intra-assay coefficients of variations of less than 10 and 20%, respectively. Variation in estradiol concentration was assessed across (1) sample type (scraped vs. cut from diaper liner), (2) time of day (morning vs. afternoon/evening samples), (3) time interval between samples, and (4) time-to-assay (1 day vs. 489 days after collection). Of these characteristics, only the time interval between samples within an individual was significantly associated with estradiol concentration. This is the first report of human infant fecal estradiol levels. The results support fecal recovery as a novel and powerful non-invasive tool for longitudinal studies of human infants, expanding research opportunities for investigating development of sex-specific behaviors in infancy, and the potential effects of endocrine disruptors on development.
Introduction
Growing concerns about the potentially endocrine-disrupting effects of environmental estrogens in general (Kelce and Wilson, 1997; Aksglaede et al., 2006; Vandenberg et al., 2009), and soy formulas in particular (Irvine et al., 1998; Setchell et al., 1998; Sharpe et al., 2002), together with increasing awareness of the consequences of pre- and postnatal exposures for lifelong health (Barker, 1995; Gluckman et al., 1999; Persson et al., 1999), highlight the importance of understanding hypothalamic–pituitary–gonadal (HPG) axis development during infancy. The importance of estrogens in the postnatal development of the body, brain, and behavior (Arnold and Gorski, 1984; Mann et al., 1993; Alexander et al., 2009) raises specific concerns about the role of exogenous estrogens for long-term reproductive function and sociosexual behavior (Mann and Fraser, 1996; Sharpe, 2003).
Present knowledge about the infant HPG axis is limited. The existence of a postnatal gonadotropin surge and the postnatal production of estradiol were first documented in the mid-1970s (Forest et al., 1974; Winter et al., 1975). According to these early reports, serum levels of estradiol were low after the first month of life, dropping below the level of detection of standard immunoassays. A coherent model of postnatal developmental trends in estradiol production has been challenging to devise due to the substantial heterogeneity in estradiol levels in infancy previously reported (Andersson et al., 1998; Quigley, 2002; Chellakooty et al., 2003; Lee, 2003). The paucity of data on the developmental trajectory of estradiol is due in large part to the ethical and methodological difficulties inherent in repeated serum sampling in healthy infants. The evidence to date of postnatal sex steroid levels is derived predominantly from studies of the gonadal contents of autopsied infants (Bidlingmaier et al., 1987; Forest and Ducharme, 1993), small clinical samples (Forest et al., 1974; Winter et al., 1976; Main et al., 2000; Ibanez et al., 2002), cross-sectional assessment of infants within a limited age range (Schmidt and Schwarz, 2000; Chellakooty et al., 2003) and infrequent longitudinal serum assessment (Andersson et al., 1998). Consequently, existing data can only describe the range of variability in hormonal levels and cannot address developmental trends or the meaning of this variability for individual behavioral development.
The present study aims to validate a novel non-invasive approach based on the analysis of fecal material to provide an alternative tool for assessing estradiol levels during infancy, thereby expanding the options for increasing our understanding of endogenous estrogen production and its biobehavioral correlates during the critical period of postnatal development. Previously developed salivary (Ellison, 1988; Ellison and Lipson, 1999; Shirtcliff et al., 2000) and bloodspot assays (Worthman and Stallings, 1997; Shirtcliff et al., 2000), considered minimally invasive for sample collection in children and adults, present both technical and practical problems for longitudinal studies of young infants. Provocation of sufficient saliva quantities to assay has relied on the use of cotton swabs, known to interfere with quantifiable steroid levels (Shirtcliff et al., 2001), or specialized collection devices (Alexander et al., 2009). Bloodspot collection, while minimally invasive among adults, is not equally non-invasive among healthy infants, and few parents or infants would tolerate the frequent finger or heel pricks required in longitudinal studies.
Fecal steroid measurement has been extensively employed in field endocrinology of non-human primates (Wasser et al., 1988; Stavisky, 1995; Brockman and Whitten, 1996; Whitten et al., 1998a) and is particularly effective when non-invasive sampling collection is required (Whitten et al., 1998a). Animal studies comparing fecal and serum values have documented that fecal steroids yield values highly correlated to those of serum (Whitten and Russell, 1996). The advantages of fecal data include non-invasive sample collection that does not interfere with a subject’s behavior or endocrine state and the need for only small quantities of stool (usually less than 1 g; Whitten et al., 1998a). Despite the extensive validation of fecal steroid measures in the studies of non-human primates (Wasser et al., 1988; Whitten et al., 1998b; Ziegler et al., 2000; Ziegler and Sousa, 2002; Beehner et al., 2005), fecal hormonal concentrations have been only infrequently utilized in studies of adult humans (Martin et al., 1975; Goldin et al., 1982; Gorbach and Goldin, 1987; Sobolik et al., 1996; Lewis et al., 1997). These methods have not previously been applied to human infants.
Fecal steroids have several characteristics that make them particularly useful indicators of hormone production for studies among human infants. First, unlike in urine, another potential non-invasive measure, the primary estrogen metabolite in feces is estradiol, and the majority of estradiol excreted in the feces (98%) is unconjugated, providing an analytically useful estimate of free, bioactive concentrations in the body (Adlercreutz and Jarvenpaa, 1982). Second, unlike serum samples which can be influenced by the pulsatile nature of sex steroid production, fecal steroids provide a time-integrated measure over several hours to several days (Whitten et al., 1998a). Thus, fecal samples present the possibility for a non-invasively acquired substrate with which to identify time-integrated bioactive estrogen secretion among infants. This may increase the probability of capturing levels of estradiol above the detection limit of present commercial assays.
This investigation tested the feasibility of adapting methods developed for non-human primate field studies to document steroid hormone concentrations in human infant feces. These methods are particularly well-suited to the study of human infant feces, since the microradioimmunoassay techniques used in fecal analysis have the advantages of being highly specific for the measured hormone, reducing the potential effects of cross-reactivity, and having a high sensitivity to allow the quantification of even low steroid levels (Stavisky, 1995; Whitten et al., 1998a). The present study employed modifications of (1) an extraction method developed for non-human primate field samples (Stavisky, 1995) and (2) a microimmunoassay technique developed for use in bloodspot samples (Shirtcliff et al., 2000). The results of extraction and RIA protocols applied to the feces of male and female infants include assay performance characteristics in terms of specificity, sensitivity, reliability, precision, accuracy and linearity.
Materials and Methods
Sample Collection and Preparation
The study sample was comprised of 32 infants (15 male, 17 female), aged 7 days to 15 months. All infants were singletons, born after 37 gestational weeks at >2500 g, following uncomplicated pregnancies. No participating infants had known endocrine, metabolic or other pathologies, either at birth or during the course of the study. A requirement for study participation was that the infant still be dependent on diapers for toilet needs. All parental participants signed informed consent for the study according to procedures reviewed and approved by the Emory University institutional human subjects research ethics board.
Parents were asked to retain soiled diapers after each diaper change during a 24-h period. In the initial phase of methods development, three types of diapers were provided for collection, standard gel-based disposable diapers, an all-natural cotton diaper, and rice paper liners used inside cloth diapers, according to parental preference. We found no significant differences in measured estradiol or assay performance by diaper type and, consequently, we asked parents to retain all soiled diapers. The majority of parents used the provided all-natural cotton diapers and none used the rice paper liners. The diapers were placed in plastic storage bags and labeled for date and time of collection. Bagged diapers were stored in portable coolers equipped with ice packs frozen at −80°C. Within 24 h, the diapers were collected and transported to the Laboratory of Reproductive Ecology at Emory University for storage at −80° C. Samples were analyzed between 24 h and 12 months after collection.
Extraction Methods
In preparation for analysis, diapers were thawed overnight at 2–8°C. Only diapers that were properly chilled for collection with sufficient fecal matter uncontaminated by co-mingling with urine, assessed through visual inspection of the cotton liner and underlying absorbent material, were used for this study. Preliminary analysis identified that a 0.25-g fecal sample mass was sufficient, and these were either scraped, if accumulated on top of the diaper liner, or excised, when soaked into the diaper liner, using disposable razor blades. Excised or scraped samples were then placed in borosilicate glass tubes with 5 ml of methanol and vortexed for 10 min. Three milliliters of this extract was then pipetted into polypropylene tubes and centrifuged for 5 min (1500×g) to remove solid particles. After spinning, the liquid phase of the samples was decanted into 0.2 μm nylon microfilter centrifuge tubes (Centrex, Whatman Laboratory, Clifton, NJ, USA) and centrifuged for an additional 5 min (1500×g). Samples were transferred to labeled polypropylene tubes for storage at −80°C until analysis. A minimum of two samples was extracted from each diaper sample when possible.
Estradiol Assay
The present assay is a modification of methods previously developed for estradiol assessment from saliva and bloodspots (Shirtcliff et al., 2000), using the DSL double antibody RIA kit for estradiol (DSL-39100, DSL, Webster, Texas). In the present study, estradiol standards and controls provided in the DSL kit were diluted four-fold with working buffer, a 0.1% gelatin-phosphate-buffered saline (pH 7.4) made by adding 0.1 g of gelatin to 100 ml of Dulbecco buffer (Gibco/Invitrogen, Grand Island, NY, USA) and incubating for 4 h at 45°C. This resulted in standard concentrations of 0.0, 0.375, 1.25, 3.75, 12.5, and 37.5 pg/ml of estradiol. The first antiserum (rabbit-produced anti-E2) was diluted 1:4, the DSL 125I tracer was diluted 1:3, and diaper extracts were diluted 1:5 with the working buffer. Duplicate aliquots (250 μl) of the diluted standards, controls, and reconstituted samples were pipetted into labeled duplicate 12 × 75 polypropylene tubes. 100 μl of the diluted first antibody was pipetted into all tubes, except the non-specific binding (NSB) tube and total count (TC) tubes, and an equal amount of working buffer was added to NSB tubes. All tubes were vortexed and then incubated at room temperature for 4 h. Following incubation, 100 μl diluted 125I E2 tracer was added to all tubes, tubes were vortexed and incubated for another 18–22 h at 4°C. Following the overnight incubation, tubes were brought to room temperature and precipitating reagent (500 μl) was added to all tubes except the TC tubes. The tubes, with the exception of the TC tubes, were again vortexed, incubated at room temperature for 20 min and then centrifuged for 1 h (1500×g). Finally, the supernatant was decanted, blotted and counted for 5 min in a RIASTAR gamma counter (Packard, Downers Grove, IL, USA), fitted with RIASMART and Expert QC software. Results are calculated in pg/ml using log-linear regression.
RIA Validation
The RIA assay modification was validated for sensitivity, accuracy, precision, and parallelism for use with fecal samples. Assay sensitivity was determined by the quantity of unlabeled steroid required to inhibit binding of tracer, by an amount equal to two standard deviations below the mean in the absence of unlabeled hormone. The accuracy of the method was assessed by measuring the recovery of incremental known quantities of steroid to low diaper extract samples. Within-assay precision was assessed through the mean coefficient of variation (%CV) of replicate determinations of high and low serum controls, and high and low fecal extract controls within the same assay. Between-assay precision was measured by %CV of low and high serum controls and low, medium and high fecal controls run across multiple assays. Controls were included in each assay. These comprised a pool of low and high fecal extracts, two serum controls (low and high) provided with the kit, and two Lyphochek serum controls (low and high) purchased separately. Parallelism was assessed by serially diluting a high sample and comparing the slope of the expected dose to the percent bound to the slope of the standard curve for the RIA.
Investigation of Potential Confounders
As the present study aims to validate a novel method, a series of steps were taken to assess the potential influences of the protocol on the assay results. These potential influences include both technical and individual biological effects. Assessment of the effects of potential interference from the diaper matrix, storage conditions and time-to-assay were necessary as diapers have not been previously validated for specimen collection in this type of study. In addition, it was necessary to assess the influences of individuals’ biological differences on within- and between-sample variability resulting from time of day, interval between depositions, and other unknown individual-based sources of variation. Steroid hormones have been documented to vary within an individual fecal sample (Adlercreutz and Jarvenpaa, 1982; Millspaugh and Washburn, 2003), and the number of fecal samples varies between individual infants and changes with age (Nyhan, 1952; Lemoh and Brooke, 1979; Tham et al., 1996).
Statistical Analysis
SAS version 9.0 (SAS Institute, NC, USA) and STATA/SE version 9 (Stata Corporation, College Station) were used for all statistical analyses. For assessment of inter- and intra-sample variability in steroid fecal values, samples were corrected for fecal weight and are represented in pg/g. Methodological effects of sampling strategy were assessed using Student’s two-sample and matched-pair t-tests. Repeated measures mixed models were used to assess the effect of time of day and interval between samples on estradiol levels. Subject was included as a random effect in all repeated measures models to control for the within-subject correlation. Although the data were not normally distributed, results of non-parametric and parametric analysis did not differ due to the large sample sizes used in t-test and mixed model analyses. Parametric results are presented for ease of interpretation. Statistical significance was defined as p < 0.05.
Results
Assay Performance
A total sample of 1374 diapers was available for analysis. Assay validation results for the modified microassay are presented in Table 1.
The analytical sensitivity of the diluted assay was 0.35 pg/ml. In tests of accuracy, the percent recovery of estradiol averaged 89% over a 0.4–12 pg/ml range of concentrations (Table 2).
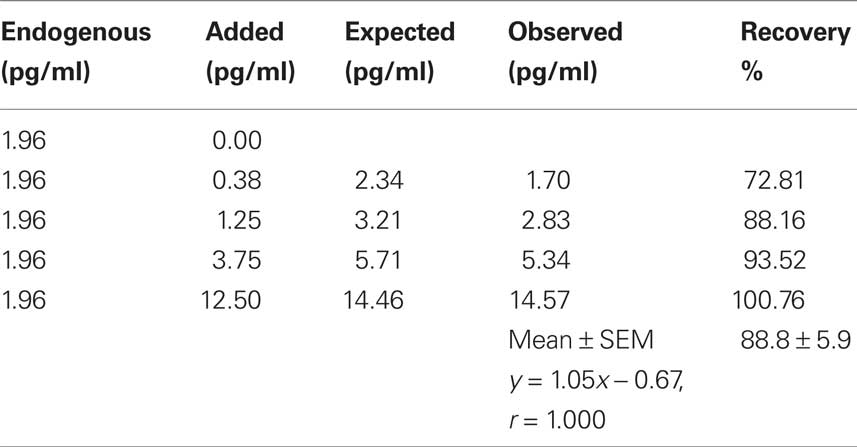
Table 2. Recovery of estradiol added to diaper extract with low endogenous estradiol using modified assay.
Serial dilution of extracts gave displacement curves that paralleled the standard curve over the range of 5–48 pg/ml, diluted 1:1 to 1:8 (Figure 1).
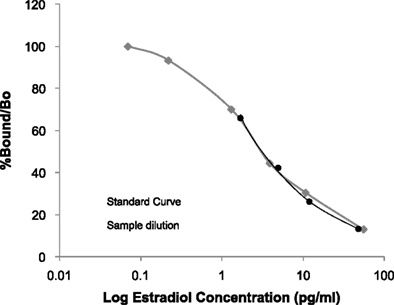
Figure 1. Serial dilution of a diaper extract to the standard curve of the modified estradiol assay.
Intra-assay coefficients of variation were 7.2–10.3% for high and low serum controls, respectively, and 2.2 and 5.8% for high and low fecal controls. Inter-assay CVs were 11 and 13% for high and low serum controls, respectively, and ranged from 9.9 to 18.5% for low, medium and high fecal controls.
Investigation of Potential Confounders
The diaper liner
In the first step, samples of clean cotton diaper liner from both the all-natural cotton diapers and standard gel-based disposable diapers were run through the extraction and assay procedures to test for the presence of any cross-reacting metabolite in the liner. There was no evidence of interference from the cotton liners in the measurement of fecal estradiol from the infant diaper samples. Cotton liners subjected to the extraction protocol and assayed following the diluted procedure yielded no detectable estradiol.
In a second test, clean samples of cotton liner (n = 25) were cut at sizes similar to those used in the sample collection process and weighed. Similarly sized liner samples (n = 25) were then cut from soiled diapers and the weight difference between the clean and soiled liners was assessed to address the proportion of the fecal/cotton sample that was accounted for by the cotton liner. As shown in Figure 2, the inclusion of the cotton liner in excised samples accounted for only a small proportion of the sample mass at the sizes used in the assay, between 16 and 25 cm2.
Additionally, in samples from diapers providing sufficient fecal quantities (n = 205), both a scraped sample and a liner sample were collected to test for any differences introduced by sample type. There were no significant differences in estradiol dose among the 205 paired-samples (paired t-test: t = 0.80, p = 0.42). The mean estradiol dose of liner samples was 0.51 pg/g (SD = 0.58), while the mean dose of scraped samples was 0.49 pg/g (SD = 0.47), indicating that the matrix had little effect on assessed estradiol.
Time-to-assay
The mean length of time between sample collection and assay was 103 days, with a range of 1–489 days. No significant association between time-to-assay and estradiol value was found in the sample (mixed model: β = 0.0003, p = 0.23). A subset of 31 samples were rerun at varying intervals and no significant correlation between estradiol dose and the length of time between assay runs was found (Spearman’s r = −0.17, p = 0.35).
Within-diaper variability
The homogeneity of fecal steroid distribution across an individual diaper was assessed in 352 duplicate samples of the same type (both liner or both scraped) collected from different portions of the diaper. Additionally, in a subsample of diapers (n = 4), multiple samples were extracted from the same diaper, with two adjacent samples collected as well as two samples collected from different parts of the diaper. Samples taken from different areas of the diaper had higher %CV than samples that were taken from more adjacent areas (Table 3).
The mean for duplicate samples from adjacent areas was 5.3%, while that from different areas was 16.2%. Pooling the samples reduced this variability, yielding a %CV of 6.4%. Despite this intra-diaper heterogeneity in %CV, differences in dose between the duplicate samples were not significant in matched-pair t-test analysis (t = 0.28, p = 0.78).
Between-sample variability
Potential diurnal variability in fecal estradiol was investigated by comparing morning to non-morning samples within individuals. Morning samples were considered as those collected between: (1) 0:00–11:59 am (n = 625), (2) 0:00–10:00 am (n = 393), chosen to correspond to a morning pattern of urinary cortisol secretion previously documented in this sample of infants, and (3) 5:00–9:00 am (n = 259), corresponding to the normal time range of awakening among this sample. Non-morning samples were those not included in the above definitions, n = 591, 769, and 907 respectively. Mean estradiol levels from the morning fecal samples did not differ from those at other times of the day, regardless of timeframe assessed, in either paired t-test analysis (unequal variances) or mixed model regression analysis (Table 4).
Time between samples
The number of daily fecal samples ranged from 0 to 5 before 3 months of age, and 0 to 3 at 6 months of age. Eight infants for whom a multiday series of diapers was available permitted an assessment of the effect of fecal deposition interval on estradiol levels. Mixed model regression analysis, controlling for age, the interaction between age and hours between diapers, and the random effect of individual, indicated that the hours between diaper samples had a modest, if significant, negative effect on estradiol level (β = −0.02, p = 0.003).
Discussion
This study is the first documentation that estradiol levels can be identified among infants aged 7 days to 15 months through a non-invasive fecal recovery method involving diapers as a collection device. The recovery rate of over 89%, a sensitivity of 0.35 pg/ml, and intra- and inter-assay coefficients of variation less than 10 and 20%, respectively, identify this approach as a useful counterpart to alternative methods based on serum and saliva. Moreover, the proposed extraction and assay procedures have the advantages over these alternative approaches of (1) having low sample volume requirements (≤0.25 g/sample), (2) providing adequate sensitivity to detect estradiol levels among both male and female infants (sensitivity = 0.35 pg/ml) across developmental ages, and (3) being well-suited for use in a longitudinal protocol (non-invasive, easy to collect, and well-tolerated by participants).
Fecal Assay Advantages
The assay procedures outlined here offer several advantages by comparison with commercially available assays. Compared to commercially available assays, this modified procedure has an increased sensitivity and a relatively high recovery rate for the range of 5–48 pg/ml over which parallelism was observed. This improved sensitivity likely facilitated the measurement of declining estradiol levels with age (Andersson et al., 1998), allowed the dilution of samples to reduce potential matrix effects and cross-reactivity of other metabolites, and reduced the cost of the assay for use in frequent longitudinal sampling by reducing the amount of reagents used per assay.
The fecal-based procedure reported here quantified estradiol in 97% of samples from both male and female infants, considerably higher than previous studies based on infant serum (Andersson et al., 1998; Chellakooty et al., 2003). It is likely that the greater proportion of samples above the detection limit highlights one of the main advantages of fecal sampling in infants: fecal levels are time-integrated. Unlike serum samples, fecal samples provide a composite, summary measure of estradiol production over several hours to a day (Whitten et al., 1998a). Although the precise timeframe involving steroid transit among human infants has yet to be described, gut transit time in general has been shown to range from 90 min, irrespective of feeding type, age or sex (Khin et al., 1999), to 17.42 h in 4-month-old formula-fed infants (Sievers et al., 1993). Observations tracking the appearance of undigested solid foods in the stool of infants in the present sample suggest that transit of solid foods may be as little as several hours (unpublished data).
Fecal vs. Serum Assay
The direct correlation between fecal and serum steroid levels was not measured in this study. This would have required repeated serum blood draws among healthy infants, an approach that was not included in the study protocol for ethical considerations. Further, there are currently no validated, published protocols for infant urinary, salivary, or bloodspot estradiol to compare with the present fecal results. However, two lines of evidence support the physiological association between fecal and serum estradiol levels. First, fecal levels of estradiol have been documented to closely parallel serum values in primates (Whitten and Russell, 1996), with co-occurrence among peaks and troughs in the longitudinal profile of fecal and serum hormone measurements in individual animals. Second, a comparison of fecal steroid levels between the study infants and previous studies of human adults reveals overlap (Goldin et al., 1982; Sobolik et al., 1996), a pattern that is also seen in infant serum when compared to adult serum (Forest and Ducharme, 1993; Andersson et al., 1998). These observations provide evidence that fecal hormonal levels reflect serum levels. Unlike serum analysis, fecal samples can be collected both frequently and over a long period of time. Future longitudinal analysis will allow the association between fecal levels of steroids and physiological measures to be assessed and provide physiological validation independent of serum measures.
Challenges Associated with the Fecal Analysis
Fecal Recovery
While the relatively elevated levels of sex steroids in human feces (Adlercreutz and Jarvenpaa, 1982) provide the methodological advantage of permitting analysis of infant estradiol levels throughout the ages measured here, the analysis of estradiol from infant diaper samples introduces challenges not seen in serum studies. First, there is the potential issue of cotton interference from the diapers used to collect the fecal sample. Any interference of cotton would be more likely at earlier ages among breastfed infants, whose stool is looser and more highly incorporated into the cotton liner. Previous examination of the effects of a cotton collection matrix on salivary levels of estradiol documented that cotton dental swabs used to collect saliva in infants and children can bias results (Shirtcliff et al., 2001).
The use of cotton diaper liners did not appear to affect the results in the current analysis. Estradiol values did not significantly differ between samples scraped from the feces on top of the liners and those excised from the cotton liner within the same diaper, suggesting that the cotton matrix did not interfere with steroid recovery. Further, unsoiled cotton samples run through the extraction and assay procedures documented no measurable estradiol levels, indicating that the cotton itself does not cross-react with the assay. These differences from past assessments may be due to the relatively higher levels of steroids present in feces compared to saliva, or to the use of methanol extraction to draw the steroids out of the cotton matrix, a step that was not taken in the salivary analysis (Shirtcliff et al., 2001).
The analysis of diaper-based samples raises the possibility for urine contamination of feces in diapers that contain both fluids. To minimize this potential for cross-contamination, diapers with an obvious mixture of urine and feces were not used in the present analysis. In diapers that contained both substances, care was taken when extracting samples to avoid fecal samples overtly mixed with urine. While it cannot be definitively excluded, it is unlikely that inadvertent urine contamination played a significant role in the variability evidenced between samples. This is because urine and feces differ in their main metabolite of estrogen, estrone vs. estradiol, respectively. A minimal fraction of urinary estradiol is in the unconjugated form measured by this assay (Adlercreutz and Martin, 1980). For a comparative perspective, Wasser et al. (1988) found that the addition of 1 ml of urine per gram of feces in ethanol-extracted fecal samples from baboons had no effect on calculated doses of estradiol. This experimental amount of urine is greater than that expected to occur physiologically.
A further potential confounder in the analysis of fecal steroids is that the amount of steroid hormone may be heterogeneous within the same fecal sample (Adlercreutz and Jarvenpaa, 1982; Millspaugh and Washburn, 2003). Comparison of the coefficients of variation between adjacent sampled areas and more divergent areas, ranging between 4.1–19.2%, suggested that this may be a source of variation in this study. To minimize this effect, duplicate samples from different areas of the same diaper were subsequently used in a pooled aliquot when the values varied. Overall analysis of the differences in estradiol dose between these duplicate samples showed no significant differences, indicating that specimen heterogeneity is unlikely to have a large effect on the sample level.
The comparative assessments focused attention on the need to attend to sample dispersion within and between diapers and to consider the possible effects of unequal inclusion of liner in the recovery from diaper to test tube. The latter effect was a less important consideration among large samples than small samples, where the liner content contributed a greater proportion to the sample. These assessments highlight the need to standardize by weight and/or size when extracting diaper samples.
Fecal Sampling and Protocol
Time of day had no significant effects on endocrine heterogeneity between samples. Serum studies have previously documented a diurnal pattern of estradiol secretion in adolescent girls and adult women (Norjavaara et al., 1996; Licinio et al., 1998), but fecal samples have less consistently shown a diurnal pattern (Beehner and Whitten, 2004), likely due to the time-integrated nature of the fecal sample. These results suggest that non-invasive fecal sampling is robust to different sampling strategies and can be employed without bias in long-term studies.
Time-to-assay did not contribute to variability in the results suggesting that the storage protocol as described is a robust one.
Conclusion and Future Work
This is the first report quantifying fecal recovery of estradiol among a sample of human infants. The present study extends previous investigations documenting the utility of fecal samples in the study of primate sex steroid levels (Whitten et al., 1998a). The validity of this novel, non-invasive method for assessing estradiol in human infants was supported by a steroid recovery rate of over 89%, a sensitivity of 0.35 pg/ml, and intra- and inter-assay coefficients of variations of less than 10 and 20%, respectively.
The use of fecal steroid assays may be a powerful tool in monitoring the development and activation of the HPG axis in early infancy among humans, yielding a method suitable for examining both inter- and intra-individual differences in hormonal concentrations. The ability to frequently sample hormonal levels presents the unique opportunity to explore both individual developmental changes and the potential associations between fecal steroid levels and behavioral measures, such as the development of toy preference or sex differences in cognitive testing (Moore and Johnson, 2008; Quinn and Liben, 2008; Alexander et al., 2009). Moreover, these methods provide tools to collect data bearing on the potential longitudinal associations between estrogen levels in infancy and long-term reproductive and behavioral development (Persson et al., 1999; Adair, 2001; Sharpe, 2003). The development of robust non-invasive methods is central to further investigation of the association between postnatal hormone production and the development of sex-specific biology and behavior.
Conflict of Interest Statement
The authors declare that the research was conducted in the absence of any commercial or financial relationships that could be construed as a potential conflict of interest.
Acknowledgments
This study was supported in part by the Woodruff Fund Award, Robert H. Woodruff Health Sciences Center, Emory University and the National Science Foundation Dissertation Improvement Grant (NSF BCS#0424307). We gratefully acknowledge the participating families and the technical and laboratory assistance provided by James Millette, Amy Cobden, Juliet Alla, and Betsy Russell. We also thank Paul Leslie and Bethany Turner for comments on the draft.
References
Adlercreutz, H., and Jarvenpaa, P. (1982). Assay of estrogens in human feces. J. Steroid Biochem. 17, 639–645.
Adlercreutz, H., and Martin, F. (1980). Biliary excretion and intestinal metabolism of progesterone and estrogens in man. J. Steroid Biochem. 13, 231–244.
Aksglaede, L., Juul, A., Leffers, H., Skakkebaek, N. E., and Andersson, A. M. (2006). The sensitivity of the child to sex steroids: possible impact of exogenous estrogens. Hum. Reprod. Update 12, 341–349.
Alexander, G. M., Wilcox, T., and Farmer, M. E. (2009). Hormone-behavior associations in early infancy. Horm. Behav. 56, 498–502.
Andersson, A. M., Toppari, J., Haavisto, A. M., Petersen, J. H., Simell, T., Simell, O., and Skakkebaek, N. E. (1998). Longitudinal reproductive hormone profiles in infants: peak of inhibin B levels in infant boys exceeds levels in adult men. J. Clin. Endocrinol. Metab. 83, 675–681.
Arnold, A. P., and Gorski, R. A. (1984). Gonadal steroid induction of structural sex differences in the central nervous system. Annu. Rev. Neurosci. 7, 413–442.
Beehner, J. C., Phillips-Conroy, J. E., and Whitten, P. L. (2005). Female testosterone, dominance rank, and aggression in an Ethiopian population of hybrid baboons. Am. J. Primatol. 67, 101–119.
Beehner, J. C., and Whitten, P. L. (2004). Modifications of a field method for fecal steroid analysis in baboons. Physiol. Behav. 82, 269–277.
Bidlingmaier, F., Strom, T. M., Dorr, H. G., Eisenmenger, W., and Knorr, D. (1987). Estrone and estradiol concentrations in human ovaries, testes, and adrenals during the first two years of life. J. Clin. Endocrinol. Metab. 65, 862–867.
Brockman, D. K., and Whitten, P. L. (1996). Reproduction in free-ranging Propithecus verreauxi: estrus and the relationship between multiple partner matings and fertilization. Am. J. Phys. Anthropol. 100, 57–69.
Chellakooty, M., Schmidt, I. M., Haavisto, A. M., Boisen, K. A., Damgaard, I. N., Mau, C., Petersen, J. H., Juul, A., Skakkebaek, N. E., and Main, K. M. (2003). Inhibin A, inhibin B, follicle-stimulating hormone, luteinizing hormone, estradiol, and sex hormone-binding globulin levels in 473 healthy infant girls. J. Clin. Endocrinol. Metab. 88, 3515–3520.
Ellison, P. T. (1988). Human salivary steroids: methodological considerations and applications in physical anthropology. Yearb. Phys. Anthropol. 31, 115–142.
Ellison, P. T., and Lipson, S. F. (1999). Salivary estradiol – a viable alternative? Fertil. Steril. 72, 951–952.
Forest, M., and Ducharme, J. (1993). “Gonadotropic and gonadal hormones,” in Pediatric Endocrinology: Physiology, Pathophysiology and Clinical Aspects, 2nd Edn., eds J. Bertrand, R. Rappaport, P. C. Sizonenko (Baltimore: Williams and Wilkins), 100–120.
Forest, M. G., Sizonenko, P. C., Cathiard, A. M., and Bertrand, J. (1974). Hypophyso-gonadal function in humans during the first year of life. 1. Evidence for testicular activity in early infancy. J. Clin. Invest. 53, 819–828.
Gluckman, P. D., Sizonenko, S. V., and Bassett, N. S. (1999). The transition from fetus to neonate–an endocrine perspective. Acta Paediatr Suppl 88, 7-11.
Goldin, B. R., Adlercreutz, H., Gorbach, S. L., Warram, J. H., Dwyer, J. T., Swenson, L., and Woods, M. N. (1982). Estrogen excretion patterns and plasma levels in vegetarian and omnivorous women. N. Engl. J. Med. 307, 1542–1547.
Gorbach, S. L., and Goldin, B. R. (1987). Diet and the excretion and enterohepatic cycling of estrogens. Prev. Med. 16, 525–531.
Ibanez, L., Valls, C., Cols, M., Ferrer, A., Marcos, M. V., and De Zegher, F. (2002). Hypersecretion of FSH in infant boys and girls born small for gestational age. J. Clin. Endocrinol. Metab. 87, 1986–1988.
Irvine, C. H., Fitzpatrick, M. G., and Alexander, S. L. (1998). Phytoestrogens in soy-based infant foods: concentrations, daily intake, and possible biological effects. Proc. Soc. Exp. Biol. Med. 217, 247–253.
Kelce, W. R., and Wilson, E. M. (1997). Environmental antiandrogens: developmental effects, molecular mechanisms, and clinical implications. J. Mol. Med. 75,198–207.
Khin, M., Bolin, T. D., Tin, O., Thein Win, N., Kyaw-Hla, S., and Thein Thein, M. (1999). Investigation of small-intestinal transit time in normal and malnourished children. J. Gastroenterol. 34, 675–679.
Lee, M. (2003). Reproductive hormones in infant girls – a harbinger of adult reproductive function? J. Clin. Endocrinol. Metab. 88, 3513–3514.
Lemoh, J. N., and Brooke, O. G. (1979). Frequency and weight of normal stools in infancy. Arch. Dis. Child 54, 719–720.
Lewis, S. J., Heaton, K. W., Oakey, R. E., and McGarrigle, H. H. (1997). Lower serum oestrogen concentrations associated with faster intestinal transit. Br. J. Cancer 76, 395–400.
Licinio, J., Negrao, A. B., Mantzoros, C., Kaklamani, V., Wong, M. L., Bongiorno, P. B., Mulla, A., Cearnal, L., Veldhuis, J. D., Flier, J. S., McCann, S. M., and Gold, P. W. (1998). Synchronicity of frequently sampled, 24-h concentrations of circulating leptin, luteinizing hormone, and estradiol in healthy women. Proc. Natl. Acad. Sci. U.S.A. 95, 2541–2546.
Main, K. M., Schmidt, I. M., and Skakkebaek, N. E. (2000). A possible role for reproductive hormones in newborn boys: progressive hypogonadism without the postnatal testosterone peak. J. Clin. Endocrinol. Metab. 85, 4905–4907.
Mann, D. R., Akinbami, M. A., Gould, K. G., Tanner, J. M., and Wallen, K. (1993). Neonatal treatment of male monkeys with a gonadotropin-releasing hormone agonist alters differentiation of central nervous system centers that regulate sexual and skeletal development. J. Clin. Endocrinol. Metab. 76, 1319–1324.
Mann, D. R., and Fraser, H. M. (1996). The neonatal period: a critical interval in male primate development. J. Endocrinol. 149, 191–197.
Martin, F., Peltonen, J., Laatikainen, T., Pulkkinen, M., and Adlercreutz, H. (1975). Excretion of progesterone metabolites and estriol in faeces from pregnant women during ampicillin administration. J. Steroid Biochem. 6, 1339–1346.
Millspaugh, J. J., and Washburn, B. E. (2003). Within-sample variation of fecal glucocorticoid measurements. Gen. Comp. Endocrinol. 132, 21–26.
Moore, D. S., and Johnson, S. P. (2008). Mental rotation in human infants: a sex difference. Psychol. Sci. 19, 1063–1066.
Norjavaara, E., Ankarberg, C., and Albertsson-Wikland, K. (1996). Diurnal rhythm of 17 beta-estradiol secretion throughout pubertal development in healthy girls: evaluation by a sensitive radioimmunoassay. J. Clin. Endocrinol. Metab. 81, 4095–4102.
Nyhan, W. L. (1952). Stool frequency of normal infants in the first week of life. Pediatrics 10, 414–425.
Persson, I., Ahlsson, F., Ewald, U., Tuvemo, T., Qingyuan, M., von Rosen, D., and Proos, L. (1999). Influence of perinatal factors on the onset of puberty in boys and girls: implications for interpretation of link with risk of long term diseases. Am. J. Epidemiol. 150, 747–755.
Quigley, C. A. (2002). Editorial: the postnatal gonadotropin and sex steroid surge-insights from the androgen insensitivity syndrome. J. Clin. Endocrinol. Metab. 87, 24–28.
Quinn, P. C., and Liben, L. S. (2008). A sex difference in mental rotation in young infants. Psychol. Sci. 19, 1067–1070.
Schmidt, H., and Schwarz, H. P. (2000). Serum concentrations of LH and FSH in the healthy newborn. Eur. J. Endocrinol. 143, 213–215.
Setchell, K. D., Zimmer-Nechemias, L., Cai, J., and Heubi, J. E. (1998). Isoflavone content of infant formulas and the metabolic fate of these phytoestrogens in early life. Am. J. Clin. Nutr. 68,1453S–1461S.
Sharpe, R. M., Martin, B., Morris, K., Greig, I., McKinnell, C., McNeilly, A. S., and Walker, M. (2002). Infant feeding with soy formula milk: effects on the testis and on blood testosterone levels in marmoset monkeys during the period of neonatal testicular activity. Hum. Reprod. 17,1692–1703.
Shirtcliff, E. A., Granger, D. A., Schwartz, E., and Curran, M. J. (2001). Use of salivary biomarkers in biobehavioral research: cotton-based sample collection methods can interfere with salivary immunoassay results. Psychoneuroendocrinology 26, 165–173.
Shirtcliff, E. A., Granger, D. A., Schwartz, E. B., Curran, M. J., Booth, A., and Overman, W. H. (2000). Assessing estradiol in biobehavioral studies using saliva and blood spots: simple radioimmunoassay protocols, reliability, and comparative validity. Horm. Behav. 38, 137–147.
Sievers, E., Oldigs, H. D., Schulz-Lell, G., and Schaub, J. (1993). Faecal excretion in infants. Eur. J. Pediatr. 152, 452–454.
Sobolik, K. D., Gremillion, K. J., Whitten, P. L., and Watson, P. J. (1996). Sex determination of prehistoric human paleofeces. Am. J. Phys. Anthropol. 101, 283–290.
Stavisky, R. C. (1995). Fecal steroid analysis of ovarian cycles in free-ranging baboons. Am. J. Primatol. 36, 285–297.
Tham, E. B., Nathan, R., Davidson, G. P., and Moore, D. J. (1996). Bowel habits of healthy Australian children aged 0–2 years. J. Paediatr. Child Health 32, 504–507.
Vandenberg, L. N., Maffini, M. V., Sonnenschein, C., Rubin, B. S., and Soto, A. M. (2009). Bisphenol-A and the great divide: a review of controversies in the field of endocrine disruption. Endocr. Rev. 30, 75–95.
Wasser, S. K., Risler, L., and Steiner, R. A. (1988). Excreted steroids in primate feces over the menstrual cycle and pregnancy. Biol. Reprod. 39, 862–872.
Whitten, P. L., Brockman, D. K., and Stavisky, R. C. (1998a). Recent advances in noninvasive techniques to monitor hormone-behavior interactions. Am. J. Phys. Anthropol. 27(Suppl.), 1–23.
Whitten, P. L., Stavisky, R., Aureli, F., and Russell, E. (1998b). Response of fecal cortisol to stress in captive chimpanzees (Pan troglodytes). Am. J. Primatol. 44, 57–69.
Whitten, P. L., and Russell, E. (1996). Information content of sexual swellings and fecal steroids in sooty mangabeys (Cercocebus torquatus atys). Am. J. Primatol. 44, 57–69.
Winter, J. S., Faiman, C., Hobson, W. C., Prasad, A. V., and Reyes, F. I. (1975). Pituitary-gonadal relations in infancy. I. Patterns of serum gonadotropin concentrations from birth to four years of age in man and chimpanzee. J. Clin. Endocrinol. Metab. 40, 545–551.
Winter, J. S., Hughes, I. A., Reyes, F. I., and Faiman, C. (1976). Pituitary-gonadal relations in infancy: 2. Patterns of serum gonadal steroid concentrations in man from birth to two years of age. J. Clin. Endocrinol. Metab. 42, 679–686.
Worthman, C. M., and Stallings, J. F. (1997). Hormone measures in finger-prick blood spot samples: new field methods for reproductive endocrinology. Am. J. Phys. Anthropol. 104, 1–21.
Ziegler, T., Hodges, K., Winkler, P., and Heistermann, M. (2000). Hormonal correlates of reproductive seasonality in wild female hanuman langurs (Presbytis entellus). Am. J. Primatol. 51, 119–134.
Keywords: estradiol, infant, fecal steroids, development, non-invasive methods, developmental endocrinology
Citation: Thompson AL, Whitten PL, Johnson ML and Lampl M (2010) Non-invasive methods for estradiol recovery from infant fecal samples. Front. Physiology 1:148. doi: 10.3389/fphys.2010.00148
Received: 09 June 2010;
Paper pending published: 30 July 2010;
Accepted: 07 October 2010;
Published online: 11 November 2010.
Edited by:
Leon Farhy, University of Virginia, USACopyright: © 2010 Thompson, Whitten, Johnson and Lampl. This is an open-access article subject to an exclusive license agreement between the authors and the Frontiers Research Foundation, which permits unrestricted use, distribution, and reproduction in any medium, provided the original authors and source are credited.
*Correspondence: Michael L. Johnson, Department of Pharmacology, University of Virginia Health System, Charlottesville, VA 22908-0735, USA. e-mail:bWxqOGVAdmlyZ2luaWEuZWR1