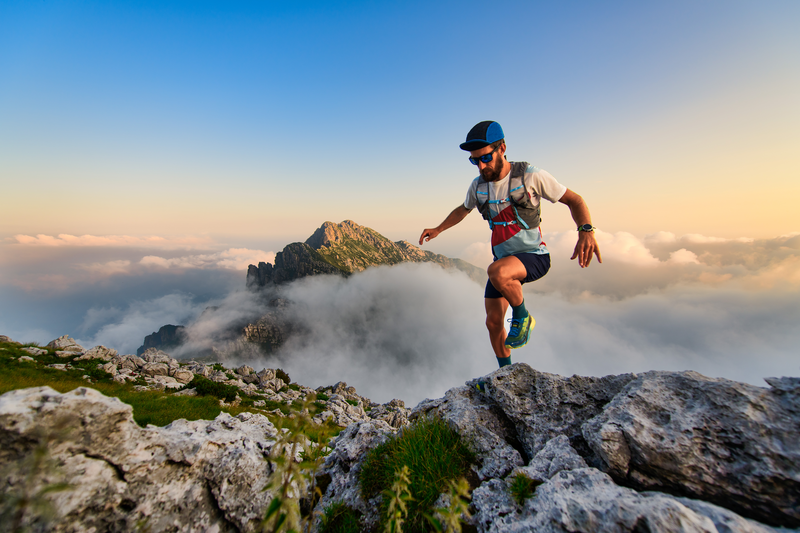
94% of researchers rate our articles as excellent or good
Learn more about the work of our research integrity team to safeguard the quality of each article we publish.
Find out more
ORIGINAL RESEARCH article
Front. Phys. , 03 January 2025
Sec. Optics and Photonics
Volume 12 - 2024 | https://doi.org/10.3389/fphy.2024.1486517
Femtosecond laser with pulse duration of 35 fs is used to introduce optical breakdown in water and ethanol. The initial dynamics of the laser-introduced break down is investigated with a time resolved shadow imaging method. The motion of the shock wave front at 600 ps, 4 ns, 12 ns, 16 ns, and 20 ns are observed. Experimental results indicate that under the same laser energy, the initial velocity of the shock wave generated in ethanol is slightly lower than that in water. This is mainly determined by liquid properties (viscosity coefficient, density, molecular weight, breakdown threshold). The evolution of the shock wave also indicates that the velocity decreases with time, and the decrease in ethanol is faster than that in water.
Laser induced breakdown (LIB) has been observed in various materials such as bulk glass, water and other aqueous medias. The mechanism involves plasma generation by multiphoton and cascade ionization, followed by shockwave releasing and propagation. The phenomena have been extensively investigated due to their wide applications in various areas, such as in microsurgery, material synthesis, photoacoustic applications, surface cleaning, and microfluidic operations [1–3].
The influence of pressure factors on spectral analysis of different substances has been studied [4, 5], making LIB suitable for spectral analysis of substance composition and properties [6, 7]. By generating plasma, atoms or molecules of a substance emit specific spectral signals, enabling component analysis and feature identification. Environmental application such as gas pollution monitoring and environmental monitoring has also been realized with such a scheme. By using laser induced plasma to excite gas molecules and generate specific radiation signals, the detection of gas composition and concentration in the environment can be achieved.
Dynamics of laser-induced breakdown in materials have been studied by using techniques such as high-speed imaging, time-resolved shadowgraph imaging, time-resolved digital holograms, pump-probe microscope, and time-resolved photoelastic imaging. Among which, the time resolved shadowgraph imaging is a technique used to observe ultrafast processes, which has been widely applied for probing the dynamics of laser-induced shock waves and cavitation bubbles. In this technique, the pump pulse causes the breakdown, while the probe pulse passes through the sample in a time delay, and illuminate the samples. The laser introduced break down causes perturbations in the refractive index, which act as refraction centers and are seen as dark areas on the camera. However, this technology also has some technical limitations and uncertainties. The method may be limited by the resolution of the imaging system, and the light source stability. Other factors in the experiment such as temperature, pressure, and gas composition, could also impact the results of shadowgraph imaging. Variations in these parameters may cause fluctuations or uncertainties in the imaging results.
The mechanism of LIB in liquid environments has been investigated intensively, and multiple factors are found to be affecting the behavior of the shockwave generation and propagation, such as laser parameters, hydrostatic pressure. Most works are based on experiment in water or aqueous media, but some other liquid materials should also be taken into consideration when inquiring the mechanism behind the phenomena.
In this work, a comparison is made between the laser introduced breakdown in water and ethanol. Ultrafast laser is utilized in the experiment, as well as the pump-probe method. The early growth dynamics of the shock wave are observed by a time resolved shadow imaging technique. Results indicate that under the same laser energy, the initial velocity of the shock wave generated in ethanol is slightly lower than that in water, and such velocity decreases faster than in water.
In the experiment, a Ti:sapphire femtosecond laser system (Spectra Physics, Solstice 35 fs, 1 kHz repetition rate at 800 nm) is used. Shadow imaging technology was used to detect the dynamics of laser shock waves. The experiment set up is plotted in Figure 1. The femtosecond laser is split into two laser beams through a beam splitter, the pump beam and the probe beam. The pump beam is used to cause the breakdown in the media, and the probe beam is used to illuminate the sample. A half waveplate and a Glan polarizer is used to adjust the pulse energy in the pump beam. A ×4 objective with a numerical aperture of 0.1 is used to focus the pump beam into a liquid chamber with size of 6 cm × 6 cm × 6 cm, and wall thickness of 2 mm. Plasma and shock waves are generated under the tight focused laser. The refractive index change caused by pressure waves causes reflection and scattering of the probe light, and this area appears as a shaded area on the CCD. The frequency of the probe light is doubled to the second harmonic through a BBO crystal. After passing through the target area, the probe light is collected and imaged on a CCD. For the optical path of the probe beam, several reflection mirror is introduced to cause time delays. As depicted in Figure 1, by tuning the positions of the reflection mirrors, different time delays can be introduced to the probe pulses. Consider that the pump and probe beams are from the same laser source, so there is not much technical challenge in synchronizing them. The time delay between the two beams can be easily calculated by measuring the distance in the reflecting mirrors. As the probe pulse reaches the target area at different time delays after the pump pulse, the shadow images of the shockwave at different stages can be captured.
In this article, we compared the optical breakdown of water and ethanol, and observed the characteristics of shock waves using different energies and delay times. The following are shadow imaging of plasma breakdown induced in pure water and analytical grade ethanol, respectively.
The time-resolved shadow imaging of laser introduced breakdown in water is shown in Figure 2A with a 35 fs pump pulse and an energy of 350 μJ. The pulse is incident from the right side, indicating that some optical filaments on the right side of the image converge to reach the focal point of the objective lens. This indicates that when the pump pulse converges towards the focus of the objective lens, higher energy will cause the formation of filaments, which are almost completed at the same time as the generation of plasma. The formation of plasma usually occurs in a very short period of time, such as a few picoseconds. In contrast, the propagation time scale of shock waves is relatively longer. This means that shock waves only begin to propagate after plasma formation.
Figure 2. A 350 μJ laser single pulse with a duration of 35 fs induces time-resolved shadow imaging of light breakdown in water through a NA = 0.1 objective lens, with the laser coming in from the right. (A) 600 ps, (B) 4 ns, (C) 12 ns, (D) 16 ns, (E) 20 ns. The scale is the same for all frames.
The focused laser introduces local multi-photon ionization of the solution, which generates a small plasma that extends along the beam channel of the pump pulse. Due to the very short formation time of plasma, it can concentrate a large amount of energy in a small area in a very short period of time, thereby generating high-intensity shock waves. The strong electric field in the local area causes the refractive index of the solution to decrease. Therefore, according to the refraction of light, areas with significant refractive index changes will undergo strong refraction and reflection, resulting in a dark shadow image. On the other hand, the nonlinear interaction between high intensity laser pulses and water can self phase modulate and generate supercontinuum spectra.
As shown in Figure 2B, the optical breakdown plasma corresponds to the accumulation of all micro-plasmas from the filament. Under the illumination of probe light, the center of the plasma will be darker, partially blocking the probe light through absorption or scattering. In Figure 2C, the energy of free electrons is transferred to the water molecule through collision, which in turn causes the breakdown of water. In our experiment, the power intensity level of the focused femtosecond laser pulses exceeds 1014 W/cm2. Under such power density, the temperature can be thousands of degrees, and the pressure can be several Gpa [8]. As shown in Figures 2C–E, the shock wave front diffuses outward and begins to expand over time.
Figure 3 records the variation trends of plasma shock waves with different energy levels at 600 ps, 4 ns, 12 ns, 16 ns, and 20 ns. The radius R of the shock wave is taken as the distance from the center of the plasma to the front of the shock wave. The shockwave front position can be told by referring to the edge of the shadow in the captured image. During the short generation time of supercontinuum and filamentation, large amount of energy is concentrated. The high temperature and high pressure environment causes extremely high speeds in the shock wave. OThe energy of the shock wave gradually dissipates as it propagate in liquids. About 65%–85% of the shock wave energy is dissipated by the surrounding liquid after propagating a few distance, until it ultimately decays into sound waves. Mathematical models have been built to describe such process [9]. Figure 4 shows the trend of shock wave dissipation when the pulse energy is 10 μJ. The change of velocity of the shock wave during the propagating process is not much different between high energy and low energy, as can be seen from Figure 5.
Figure 4. Evolution of the front radius and viscosity of shock wave generated in water (pulse energy 10 μJ).
Figure 5. Evolution of the front radius of optical breakdown shock waves with pulse energy of 100 μJ, 200 μJ and 300 μJ in water.
Physical parameters of liquids like viscosity, density are factors that could affect the shock wave behavior. In viscous fluids, the thickness of shock waves is greater than in inviscid fluids because viscosity causes energy dissipation, thereby slowing down the propagation speed of shock waves. The propagation of shock waves in high-density media will also experience more resistance, thereby reducing the intensity of shock waves. The optical breakdown in ethanol is experimentally investigated in comparison to verify such difference. The influence of the density on shock wave propagating can be described using the Rankine Hugoniot equation [10, 11]. Figure 6 shows Time resolved shadow imaging of light breakdown in analytical grade ethanol induced by a 350 μJ laser single pulse.
Figure 6. Time resolved shadow imaging of light breakdown in analytical grade ethanol induced by a 350 μJ laser pulse with a duration of 35 fs through a NA = 0.1 objective, with the laser incident from the right side. (A) 600 ps, (B) 4 ns, (C) 12 ns, (D) 16 ns, (E) 20 ns.
In Figure 6A, due to the short delay of the detection light, it can be seen that there are obvious black creases on the upper and lower exterior of the plasma, which are almost close to the outer side of the plasma. It can also be seen that there are many long filaments formed at the right incidence point and irregular plasma interactions near the center (Figures 6B–E).
Figures 7, 8 record the trend of changes in plasma shock waves with different energy levels. Under the same pulse energy, the initial velocity of the shock wave in ethanol is slightly smaller than that in water. That is because the viscosity coefficient of the solution, the absorption energy of molecular bonds, and the breakdown threshold of the solution can all affect the generation of the shock wave. From Figure 8, one can also tell that the velocity of the shock wave drops much faster compared with the case in water. This is because the viscosity of ethanol is higher than that of water, resulting in a faster decrease in shock wave velocity [12].
Figure 7. Changes in the radius of shock wave fronts at different energy and time periods in ethanol.
The initial dynamics of the laser-introduced break down in water and ethanol is investigated with a time resolved shadow imaging method. Ultrafast laser is utilized in the experiment, as well as the pump-probe method. Results indicate that under the same laser energy, the initial velocity of the shock wave generated in ethanol is slightly lower than that in water, and such velocity decreases faster than in water. The viscosity, density, and molecular weight quantitatively are the physical parameters of the liquid that affects the shock wave dynamics.
The raw data supporting the conclusions of this article will be made available by the authors, without undue reservation.
LS: Investigation, Writing–original draft. XQ: Methodology, Writing–original draft. RG: Formal Analysis, Writing–original draft. YJ: Data curation, Writing–original draft. CY: Validation, Writing–original draft. SL: Supervision, Writing–original draft.
The author(s) declare that no financial support was received for the research, authorship, and/or publication of this article.
The authors declare that the research was conducted in the absence of any commercial or financial relationships that could be construed as a potential conflict of interest.
All claims expressed in this article are solely those of the authors and do not necessarily represent those of their affiliated organizations, or those of the publisher, the editors and the reviewers. Any product that may be evaluated in this article, or claim that may be made by its manufacturer, is not guaranteed or endorsed by the publisher.
1. Zinnecker V, Madden S, Stokes-Griffin C, Compston P, Rode AV, Rapp L. Ultrashort pulse laser ablation of steel in ambient air. Opt & Laser Technology (2022/04/01/2022) 148:107757. doi:10.1016/j.optlastec.2021.107757
2. Smirnov NA, Kudryashov SI, Rudenko AA, Zayarny DA, Ionin AA. Pulsewidth and ambient medium effects during ultrashort-pulse laser ablation of silicon in air and water. Appl Surf Sci (2021/10/01/2021) 562:150243. doi:10.1016/j.apsusc.2021.150243
3. Zhang L, Liu J, Zhong Z, Jiang H, Gu H, Chen X, et al. Beam collapse and refractive index changes inside fused silica induced by loosely focused femtosecond laser. J Opt (2021/05/28 2021) 23(7):075402. doi:10.1088/2040-8986/abfc5b
4. Peng X, Chen M, Liu H. Optical emission spectrometric diagnosis of laser-induced plasma and shock front produced at moderate pressure. Chin Opt Lett (2023) 21(2):023001. doi:10.3788/col202321.023001
5. Hohreiter V, Hahn DW. Dual-pulse laser induced breakdown spectroscopy: time-resolved transmission and spectral measurements. Spectrochimica Acta B: At Spectrosc (2005/08/31/2005) 60(7):968–74. doi:10.1016/j.sab.2005.05.031
6. Zhang T-L, Wu S, Tang H-S, Wang K, Duan Y-X, Li H. Progress of chemometrics in laser-induced breakdown spectroscopy analysis. Chin J Anal Chem (2015/06/01/2015) 43(6):939–48. doi:10.1016/s1872-2040(15)60832-5
7. Michel APM. Review: applications of single-shot laser-induced breakdown spectroscopy. Spectrochimica Acta Part B: At Spectrosc (2010/03/01/2010) 65(3):185–91. doi:10.1016/j.sab.2010.01.006
8. Tian Y, Li Y, Wang L, Huang F, Lu Y, Guo J, et al. Laser-induced plasma in water at high pressures up to 40 MPa: a time-resolved study. Opt Express (2020) 28:18122–30. doi:10.1364/oe.391420
9. Min BB, Xiao C, Ming X, Ling Y, Hua SZ. The investigation of laser-induced plasma shock wave propagation in liquids. Acta Phys Sin (2004) 53(2):508–13. doi:10.7498/aps.53.508
10. Ma X, Kong D, Shi Y. Measurement and analysis of shock wave pressure in moving charge and stationary charge explosions. Sensors (2022) 22(17):6582. doi:10.3390/s22176582
11. Needham CE. The rankine-hugoniot relations. In: Blast waves. Shock wave and high pressure phenomena. Cham: Springer (2018). doi:10.1007/978-3-319-65382-2_3
Keywords: laser introduced breakdown, laser energy, shock wave velocity, time resolved shadow imaging method, shock wave front
Citation: Su L, Qiu X, Guo R, Jing Y, Yang C and Liu S (2025) Dynamics of ultrafast laser introduced breakdown in water and ethanol. Front. Phys. 12:1486517. doi: 10.3389/fphy.2024.1486517
Received: 26 August 2024; Accepted: 09 December 2024;
Published: 03 January 2025.
Edited by:
Gopala Krishna Podagatlapalli, Gandhi Institute of Technology and Management (GITAM), IndiaReviewed by:
Sunku Sreedhar, Madanapalle Institute of Technology and Science, Madanapalle, IndiaCopyright © 2025 Su, Qiu, Guo, Jing, Yang and Liu. This is an open-access article distributed under the terms of the Creative Commons Attribution License (CC BY). The use, distribution or reproduction in other forums is permitted, provided the original author(s) and the copyright owner(s) are credited and that the original publication in this journal is cited, in accordance with accepted academic practice. No use, distribution or reproduction is permitted which does not comply with these terms.
*Correspondence: Shuhui Liu, bHNoMjAwNjAzODhAMTYzLmNvbQ==
Disclaimer: All claims expressed in this article are solely those of the authors and do not necessarily represent those of their affiliated organizations, or those of the publisher, the editors and the reviewers. Any product that may be evaluated in this article or claim that may be made by its manufacturer is not guaranteed or endorsed by the publisher.
Research integrity at Frontiers
Learn more about the work of our research integrity team to safeguard the quality of each article we publish.