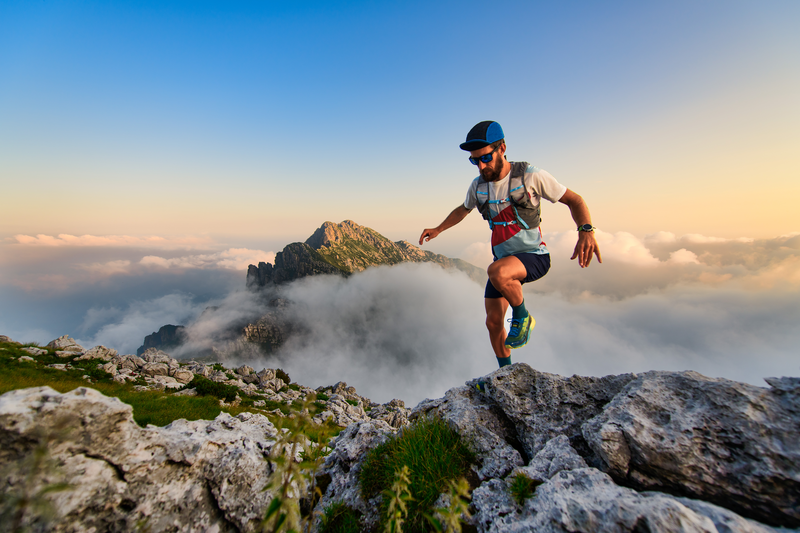
94% of researchers rate our articles as excellent or good
Learn more about the work of our research integrity team to safeguard the quality of each article we publish.
Find out more
EDITORIAL article
Front. Phys. , 13 August 2024
Sec. Biophysics
Volume 12 - 2024 | https://doi.org/10.3389/fphy.2024.1474263
This article is part of the Research Topic Biophysics of Muscle Contraction View all 6 articles
Editorial on the Research Topic
Biophysics of muscle contraction
Muscle biophysics is in some measure already a mature science. The sliding-filament theory, originally presented in the 1950s, remains the bedrock of our understanding of sarcomere force generation. The role of intracellular calcium as a principal regulator of contraction, through its interactions with the troponin-tropomyosin subsystem, is now textbook material. Experimental characterisations of muscle function, covering all length scales from single molecules to the whole organ (whole muscle or whole heart), have shaped our understanding of this multifaceted system. Over decades, experiments have informed theoretical biophysical models and vice versa, providing complementary insights into the intricacies of the contraction machinery and its physiological context.
Nonetheless, the field continues to evolve actively. Recent experiments have revealed novel fundamental regulatory mechanisms (e.g., the tension-dependent myosin “off” state) that remain to be fully characterised. New application perspectives are also emerging. Current ambitions include harnessing machine-learning-based analyses integrating experimental and clinical data to customise computational cardiac models to individual patients, hence guiding clinical diagnoses and interventions. The aim of this Research Topic was to sample some of the current research, fundamental or applied, in this diverse field. Inevitably, we could only expect to present a very partial snapshot. Nevertheless, even this succinct Research Topic conveys a flavour of present and future challenges.
Fundamental aspects of basic contraction regulation remain to be clarified. The perspective article by Chalovich revisits the conventional paradigm that calcium-mediated thin-filament activation results simply from the steric hindrance of actin-myosin interaction by tropomyosin. By using an energetics argument, it considers an alternative scenario involving a more nuanced three-way interdependence between calcium, actin, and myosin-ATP. Grasping the finer details of muscle regulation ultimately opens possibilities for understanding and treating specific mutation-based pathologies.
Thin-filament regulation by calcium is conventionally characterised empirically by a Hill-type sigmoidal dependence of force on intracellular calcium in the isometric steady state. However, as Dowrick et al. note in their review article, this characterisation is ill-adapted to dynamic situations. A definitive and more complete characterisation, taking into account all relevant calcium-influenced mechanisms, remains to be developed and is likely to involve a combination of multiple measurements and biophysical modelling.
Elucidating the detailed interdependence of strain, metabolites, and force generation remains a significant experimental challenge. The study by Musgrave et al. exemplifies the use of computational modelling to assess the relative ability of multiple hypothetical biophysical scenarios to reproduce observed behaviour. By comparing the effects of various combinations of coupled strain-dependence scenarios and metabolite conditions (ATP and Pi) on the dynamic modulus of cardiac muscle, they identify a direct regulatory effect by these metabolites on cross-bridge detachment rates. The resulting model opens the way to a more comprehensive integration of modelling within the broader physiological context.
An important challenge of biophysical modelling is to quantitatively describe the interface between interacting physiological subsystems. Kappaun et al. present a phenomenological model coupling skeletal muscle contraction with neuronal stimulation that accounts for the so-called latency period. This minimal model, comprising a small number of muscle motor units, provides an intuitive framework for further modelling the role of latency in controlling force generation in the macroscopic muscle system, and hence for investigating potential pathways leading to pathological deficiencies in contraction dynamics.
An ultimate goal of biophysical modelling is arguably to provide practical tools for aiding and guiding clinical practice. The review article by Rodero et al. on the clinical translation of cardiac biomechanical models surveys current state-of-the-art techniques, emphasising their potential while highlighting significant hurdles. A major challenge is to balance, on the one hand, the model complexity necessary to emulate the real physiology system with, on the other hand, available computational resources and the required accuracy, reliability, and validation of parameter calibrations.
To conclude, this brief Research Topic presents a concise cross-section through the ongoing research in muscle biophysics. Despite the breadth and richness of knowledge acquired to date in the field, there remains considerable scope for further development. Even as many fundamental effects are firmly established, their detailed quantitative description and the experimental validation of biophysical models remain as challenges to achieving a systematic holistic understanding of the overall physiology. Nonetheless, the development of novel analytical and computational tools and techniques continues to open new possibilities for refinement.
AL: Conceptualization, Writing–original draft, Writing–review and editing.
The author(s) declare that no financial support was received for the research, authorship, and/or publication of this article.
The author declares that the research was conducted in the absence of any commercial or financial relationships that could be construed as a potential conflict of interest.
All claims expressed in this article are solely those of the authors and do not necessarily represent those of their affiliated organizations, or those of the publisher, the editors and the reviewers. Any product that may be evaluated in this article, or claim that may be made by its manufacturer, is not guaranteed or endorsed by the publisher.
Keywords: editorial, biophysics, muscle contraction, biomechanics, biophysical modeling, computational modeling
Citation: Lewalle A (2024) Editorial: Biophysics of muscle contraction. Front. Phys. 12:1474263. doi: 10.3389/fphy.2024.1474263
Received: 01 August 2024; Accepted: 05 August 2024;
Published: 13 August 2024.
Edited and reviewed by:
Ralf Metzler, University of Potsdam, GermanyCopyright © 2024 Lewalle. This is an open-access article distributed under the terms of the Creative Commons Attribution License (CC BY). The use, distribution or reproduction in other forums is permitted, provided the original author(s) and the copyright owner(s) are credited and that the original publication in this journal is cited, in accordance with accepted academic practice. No use, distribution or reproduction is permitted which does not comply with these terms.
*Correspondence: Alexandre Lewalle, YS5sZXdhbGxlQGltcGVyaWFsLmFjLnVr
Disclaimer: All claims expressed in this article are solely those of the authors and do not necessarily represent those of their affiliated organizations, or those of the publisher, the editors and the reviewers. Any product that may be evaluated in this article or claim that may be made by its manufacturer is not guaranteed or endorsed by the publisher.
Research integrity at Frontiers
Learn more about the work of our research integrity team to safeguard the quality of each article we publish.