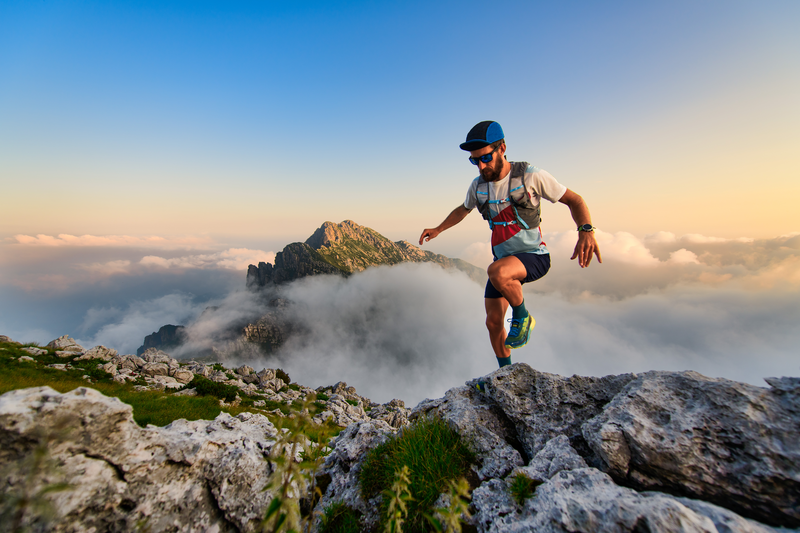
95% of researchers rate our articles as excellent or good
Learn more about the work of our research integrity team to safeguard the quality of each article we publish.
Find out more
EDITORIAL article
Front. Phys. , 20 June 2024
Sec. Medical Physics and Imaging
Volume 12 - 2024 | https://doi.org/10.3389/fphy.2024.1439081
This article is part of the Research Topic Multidisciplinary Approaches to The FLASH Radiotherapy View all 12 articles
Editorial on the Research Topic
Multidisciplinary approaches to the FLASH radiotherapy
Radiotherapy (RT) is extensively used in cancer treatment, although its toxicity often limits the treatment of radioresistant tumors. In this context, it has been recently shown, that irradiation at ultra-high dose rate (UHDR) (mean dose rate ≥40 Gy/s, with specific beam characteristics), called “FLASH-RT” may significantly reduce radiation-induced toxicity on normal tissues, while keeping similar antitumor effect as conventional RT [1]. This so called “FLASH effect” has been demonstrated in vivo on different animal models and various tumor types, using different radiations types (electrons, protons, carbon ions, and photons [2]) and pulse structures.
While these rapidly accumulating results indicate bright prospects, the clinical translation is still in its early phase, due to different challenges. First, several technological issues must be addressed to design new stable radiation sources capable of delivering beams with fluences orders of magnitude higher than those of conventional RT, and with a reliable real time beam monitoring system. This also implies the need of new dosimetric protocols, since most of the active dosimeters used for conventional beams do not respond accurately to UHDR and ultra high dose-per-pulse (UHDP) [3, 4]. Accurate dosimetry is not only needed for clinical implementation, but also for more robust and reproducible pre-clinical experiments [5]. The second challenge is understanding the biological mechanism underlying the FLASH effect, to explain the differential response of cancer vs. normal tissues. Several hypotheses have been considered, involving the whole cascade from the early radiation chemistry events to the classical radiation-induced molecular and cellular mechanisms and tissue recovery processes, also including a role for (epi)-genetics, stem cells or the immune system. While many results support different hypotheses, no compelling evidence exists that can yet confirm any of them.
The full clinical exploitation and optimization of UHDR beams and FLASH-RT requires a multidisciplinary approach. Figure 1 illustrates a possible scheme of such an effort, involving multiple interconnected research areas, from the technology of the beam production and characterization to the final effects on cell and tissues, through the dose distribution and molecular-subcellular dynamics. In this landscape the determinants of the FLASH effect can be identified by providing quantitative relationships between the irradiation parameters, tissue descriptors and radiobiological effects. With these motivations, we selected and collected, in this Frontiers Research Topic 11 contributions covering various aspects of these areas. Among these, Di Martino et al. report the dosimetric characterization of a dedicated UHDP electron linear accelerator (linac Electron Flash (EF) with triode-gun) with the capability of flexibly and independently varying all the beam parameters over a wide range, also allowing the implementation of radiobiological experiments in vitro and in vivo. This study completes some previous ones from the same authors [6, 7] providing a full description of the EF beams’ potential. The real time beam monitoring needs of these new linacs and related issues are addressed by Vojnovic et al. reporting the design of a beam charge integrating transformer achieving a high sensitivity with respect to standard UHDP beam monitoring systems. On the same topic, Medina et al. tested silicon-based sensors on UHDP electron beams and the possibility of using them as beam monitoring systems in FLASH regime by verifying their linear response with dose-per-pulse up to over 10 Gy. A major challenge in the case of protons is the realization of conformal treatments exploiting the spread out of Bragg peak. This topic is detailed in Horst et al., describing a perfect in vivo FLASH target station exploiting two different setups for range modulation. Recently, it was also proposed that the spatial fractionation of the beam on the micro-milli scale (amongst which mini-beam irradiation) might result in effects similar to FLASH-RT. Pensavalle et al. designed, realized and dosimetrically characterized the first mini-beam and mini-beam/FLASH beams for electrons, by modifying the EF beam optics with tungsten templates. This apparatus can be used for experiments exploring possible synergies between minibeam and FLASH effects in a clinical perspective.
Figure 1. Exemplary scheme of the multi-disciplinary efforts involved in the study and exploitation of the FLASH effect. Following the arrow flux from top left of the scheme: 1. dedicated accelerators are needed to generate beams with suitable and tunable parameters (dose rate, dose per pulse, pulse dynamics, linear energy transfer (LET), etc.) to explore the domain of the FLASH irradiation regime; 2. a strong dosimetry effort is needed to characterize the beams and the dose released to the cells in culture and tissues, either in vitro or in vivo; 3. subsequently, a number of different indicators of the radiobiological effects (e.g., ROS, cell survival, cognitive impairment) can be measured; 4. the descriptors of the radiobiological effects, as well as the descriptors of cell/tissues and the parameters of irradiation may be passed to a database for in silico elaboration based on simulations and machine learning algorithms to analyze data and extract the FLASH modifying factor; 5. Overall this multi-disciplinary elaboration will clarify the molecular mechanisms, allow a quantitative prediction of the effect on different tissues, and give indication for the treatment planning in a clinical final context.
Downstream of the irradiating beam, a vast amount of experimental evidence of the FLASH effect is accumulating. A systematic organization of the literature is difficult, since data are taken in very different conditions and use a multitude of different irradiation conditions and radiobiological “end points”. Del Debbio et al. report a systematic review of the in vitro experiments on electron-FLASH-RT presenting them in relation to the different hypotheses on the radiobiological mechanisms.
The in silico approaches are powerful tools in complement to experiments, to investigate the response of cancer vs normal tissues. Most of the modeling efforts concentrate on the chemical stages of radiation damage, considered as the most sensitive to spatio-temporal features of dose delivery, using e.g., reaction diffusion based models. The simulations by Baikalov et al. see a negligible role of the inter-track interactions in the parameters range where the FLASH effect is observed with electrons, confirming what was observed with protons [8] and carbon ions [9], indicating that effects might occur on larger time scales. To expand simulation time scales, Abolfath et al. use coarse models of tissues with different connectivity and porosity representing normal and cancer tissues, and show different inter-track effects, arguing this as a possible source of the differential effect of FLASH-RT. With a different approach Battestini et al. explored the connection between the chemical stages and the DNA damage through a multiscale extension of the generalized stochastic microdosimetric model [10] integrated with a chemical network [11], reproducing the experimental trend of the in vitro experiments in terms of dose, dose rate and LET dependence of the effect onset. Overall, we envision that combining Monte Carlo with multi-scale molecular dynamics simulations [12] would amplify the predictive power of in silico approaches.
The clinical perspectives for FLASH-RT are potentially huge, and their investigation is just at the beginning. Ursino et al. illustrate a clinical scenario of the FLASH effect, supported by pre-clinical in vivo studies, and focusing on possible future applications of low and very high energy electron (VHEE) beams. The potential of VHEE is also explored by Muscato et al. in intracranial lesions using a small number of mono-energetic fields and assuming an active-scanning-like beam delivery strategy, compared with conventional x-ray intensity modulated radiation therapy(IMRT) and proton therapy, both considering and not considering a possible FLASH sparing effect.
This Research Topic is a good representation of the state-of-the-art of research towards both the understanding of the mechanisms and the clinical translation of the FLASH effect: much has been done recently both in the field of the production and monitoring/measuring of UHDP beams. These results are the first step to proceed towards a deeper knowledge of the phenomenon and towards its optimal clinical implementation.
FDM: Conceptualization, Writing–original draft, Writing–review and editing. ES: Conceptualization, Writing–original draft, Writing–review and editing. VP: Writing–review and editing. PM-G: Writing–review and editing. FR: Writing–review and editing. AD: Writing–review and editing. VT: Conceptualization, Visualization, Writing–original draft, Writing–review and editing.
The author(s) declare that financial support was received for the research, authorship, and/or publication of this article. The authors acknowledge the support of Next Generation-EU (Piano Nazionale di Ripresa e Resilienza (PNRR), Missione 4, Componente 2, Ecosistemi dell’Innovazione) through the project Tuscany Health Ecosystem (THE-Spoke 1, grant ECS_00000017); European Union - NextGenera-tionEU PNRR - M4C2-l1.3 Project PE 00000019 “HEAL ITALIA”, INFN CSN5 through the FRIDA Call and the MIRO project.
The authors declare that the research was conducted in the absence of any commercial or financial relationships that could be construed as a potential conflict of interest.
The author(s) declared that they were an editorial board member of Frontiers, at the time of submission. This had no impact on the peer review process and the final decision.
All claims expressed in this article are solely those of the authors and do not necessarily represent those of their affiliated organizations, or those of the publisher, the editors and the reviewers. Any product that may be evaluated in this article, or claim that may be made by its manufacturer, is not guaranteed or endorsed by the publisher.
1. Montay-Gruel P, Petersson K, Jaccard M, Boivin T, Germond JF, Petit B, et al. Irradiation in a flash: unique sparing of memory in mice after whole brain irradiation with dose rates above 100 Gy/s. Radiother Oncol (2017) 124:365–9. doi:10.1016/j.radonc.2017.05.003
2. Vozenin MC, Bourhis J, Durante M. Towards clinical translation of FLASH radiotherapy. Nat Rev Clin Oncol (2022) 19:791–803. doi:10.1038/s41571-022-00697-z
3. Di Martino F, Del Sarto D, Bisogni MG, Capaccioli S, Galante F, Gasperini A, et al. A new solution for UHDP and UHDR (Flash) measurements: theory and conceptual design of ALLS chamber. Phys Med (2022) 102:9–18. doi:10.1016/j.ejmp.2022.08.010
4. Gómez F, Gonzalez-Castaño DM, Gómez Fernández N, Pardo-Montero J, Schüller A, Gasparini A, et al. J Paz-Martín Development of an ultra-thin parallel plate ionization chamber for dosimetry in FLASH radiotherapy. Med Phys (2022) 49:4705–471. doi:10.1002/mp.15668
5. Romano F, Bailat C, Jorge PG, Lerch MLF, Darafsheh A. Ultra-high dose rate dosimetry: challenges and opportunities for FLASH radiation therapy. Med Phys (2022) 49:4912–32. doi:10.1002/mp.15649
6. Felici G, Barca P, Barone S, Bortoli E, Borgheresi R, De Stefano S, et al. Transforming an IORT linac into a FLASH research machine: procedure and dosimetric characterization. Front Phys (2020) 8:374. doi:10.3389/fphy.2020.00374
7. Di Martino F, Barca P, Barone S, Bortoli E, Borgheresi R, De Stefano S, et al. FLASH radiotherapy with electrons: issues related to the production, monitoring, and dosimetric characterization of the beam. Front Phys (2020) 8:570697. doi:10.3389/fphy.2020.570697
8. Ramos-Méndez J, Domínguez-Kondo N, Schuemann J, McNamara A, Moreno-Barbosa E, Faddegon B. LET-dependent intertrack yields in proton irradiation at ultra-high dose rates relevant for FLASH therapy. Rad Res (2020) 194:351–62. doi:10.1667/RADE-20-00084.1
9. Weber UA, Scifoni E, Durante M. FLASH radiotherapy with carbon ion beams. Med Phys (2022) 49:1974–92. doi:10.1002/mp.15135
10. Cordoni F, Missiaggia M, Attili A, Welford SM, Scifoni E, La Tessa C. Generalized stochastic microdosimetric model: the main formulation. Phys Rev E (2021) 103:012412. doi:10.1103/PhysRevE.103.012412
11. Labarbe R, Hotoiu L, Barbier J, Favaudon V. A physicochemical model of reaction kinetics supports peroxyl radical recombination as the main determinant of the FLASH effect. Rad Oncol (2020) 153:303–10. doi:10.1016/j.radonc.2020.06.001
Keywords: radiotherapy, dosimetry, radiobiology, radiobiological modeling, FLASH radiotherapy, ultra high dose rate irradiation, ultra high dose-per-pulse, clinical translation
Citation: Di Martino F, Scifoni E, Patera V, Montay-Gruel P, Romano F, Darafsheh A and Tozzini V (2024) Editorial: Multidisciplinary approaches to the FLASH radiotherapy. Front. Phys. 12:1439081. doi: 10.3389/fphy.2024.1439081
Received: 27 May 2024; Accepted: 30 May 2024;
Published: 20 June 2024.
Edited and reviewed by:
Federico Giove, Centro Fermi—Museo storico della fisica e Centro studi e ricerche Enrico Fermi, ItalyCopyright © 2024 Di Martino, Scifoni, Patera, Montay-Gruel, Romano, Darafsheh and Tozzini. This is an open-access article distributed under the terms of the Creative Commons Attribution License (CC BY). The use, distribution or reproduction in other forums is permitted, provided the original author(s) and the copyright owner(s) are credited and that the original publication in this journal is cited, in accordance with accepted academic practice. No use, distribution or reproduction is permitted which does not comply with these terms.
*Correspondence: Fabio Di Martino, Zi5kaW1hcnRpbm9AYW8tcGlzYS50b3NjYW5hLml0; Emanuele Scifoni, ZW1hbnVlbGUuc2NpZm9uaUB0aXBmYS5pbmZuLml0
Disclaimer: All claims expressed in this article are solely those of the authors and do not necessarily represent those of their affiliated organizations, or those of the publisher, the editors and the reviewers. Any product that may be evaluated in this article or claim that may be made by its manufacturer is not guaranteed or endorsed by the publisher.
Research integrity at Frontiers
Learn more about the work of our research integrity team to safeguard the quality of each article we publish.