- 1Department of Electronic Engineering, Shanghai Jiao Tong University, Shanghai, China
- 2Shanghai Institute of Satellite Engineering, Shanghai, China
- 3Shanghai Academy of Spaceflight Technology, Shanghai, China
In today’s era of increasing energy constraints, harnessing the power of electromagnetic waves and converting them into directly usable energy has great potential in the field of renewable energy. This paper presents a highly efficient electromagnetic energy harvester with wide operation angles based on metasurface. The metasurface unit comprises four rotated copper rings plated on a Rogers RT5880 substrate. This unique design incorporates a 3.6 k
1 Introduction
In the modern technological era, the proficient manipulation of electromagnetic waves holds an ever-growing significance [1–3]. Specifically, the effective absorption and harvesting of electromagnetic energy have gained increasing importance. An energy harvester stands as a pivotal element in wireless power transmission or electromagnetic energy harvesting systems [4–6]. Its fundamental role is to capture direct or ambient electromagnetic wave energy and efficiently transform it into usable DC power [7, 8]. This technology holds immense potential for various renewable energy applications, especially in remote or hard-to-reach areas where a continuous power supply is crucial. Nonetheless, several technical challenges still need to be overcome. Notably, the polarization sensitivity, incident angle sensitivity, and conversion efficiency of current electromagnetic energy harvesters require further development. Addressing these limitations is crucial for the widespread adoption and practicality of electromagnetic energy harvesting systems.
Metamaterials or metasurfaces are artificially structured materials that exhibit extraordinary electromagnetic properties not readily available in conventional materials [9, 10]. [11] proposed that split-ring resonator (SRR)-based metamaterials can have equivalent energy harvesting. [12] demonstrated the viability of wideband ground-backed complementary SRR arrays with significant power conversion efficiency and bandwidth enhancement in comparison to the technology used in current electromagnetic energy harvesting systems. [13] reported a metamaterial with polarization-insensitive, wide-angle, and considerably high harvesting efficiency operating in triple bands. [14] proposed a metasurface device consisting of four identical omega ring resonators for achieving a harvesting efficiency of up to 93.1% under normal incidence. [15] proposed a metasurface harvester using a single band via to realize polarization-insensitive characteristics, which can achieve a maximum harvesting efficiency of up to 88% at 5.8 GHz. [13] demonstrated a tri-band, polarization-insensitive, and wide-angle metamaterial array for electromagnetic energy harvesting, achieving the highest harvesting efficiency of 87%, which is relatively lower than the efficiency reported in our work. Most recently, [16] reported a dual-band metasurface array capable of simultaneous electromagnetic energy harvesting and wireless power transfer. Despite the reasonable effort devoted to this field, there is still a high demand for a metasurface harvester with high efficiency, polarization insensitivity, and angle stability.
In this paper, we introduce a high-performance metasurface specifically designed for electromagnetic energy harvesting in the microwave regime. Utilizing a unique four-fold rotational configuration, this metasurface not only achieves almost perfect absorption but also effectively transfers the absorbed energy to resistive loads. These resistive loads serve as a simplified model for the input impedance of a rectifier circuit, which can be seamlessly attached to each cell of the metasurface. The key advantage of this design lies in its ability to maintain enhanced electromagnetic energy harvesting efficiency over a wide incident angle, regardless of the polarization states of the incident wave. This flexibility and efficiency make our metasurface a promising candidate for various energy harvesting applications, where reliable and continuous energy supply is crucial.
2 Design of the metasurface harvester
The metasurface harvester configuration under consideration is shown in Figure 1. Such a metasurface consists of a four-fold rotational copper ring plated on a thick Rogers RT5880 substrate having a permittivity of
The electromagnetic performance of the metasurface is numerically studied using the commercial software CST Microwave Studio. Unit cell boundaries are applied in the
3 Numerical results
Since the transmission is mostly blocked by the thick ground plane, the electromagnetic absorption can be directly calculated from the reflection coefficient, expressed by Eq. 1,
Figure 2 shows the simulation results of reflection, transmission, and absorption at normal incidence. Reflection minimization is achieved by adjusting the physical parameters of the metasurface to match its effective impedance to the effective impedance of free space. The simulation results show that the electromagnetic wave is almost completely absorbed near the frequency of 5.85 GHz. The harvesting efficiency of the meta-harvester is expressed by Eq. 2,
where
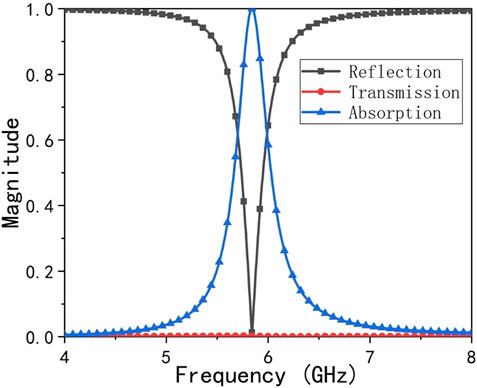
Figure 2. Simulated reflection, transmission, and absorption of the metasurface harvester at normal incidence.
The angle stability of different polarization angles under normal incidence is further analyzed. As shown in Figure 4, the metamaterial device exhibits stable energy harvesting characteristics despite the change in the polarization angle of the incident wave.Moreover, the absorption and harvesting efficiency performance of the metasurface harvester under oblique incidence are further analyzed. In Figure 5, we can observe that the absorption of the metasurface exhibits stable energy harvesting characteristics despite the change in the polarization angle. We can see that, for the case of TE incidence, the absorption curves are close to 100% below
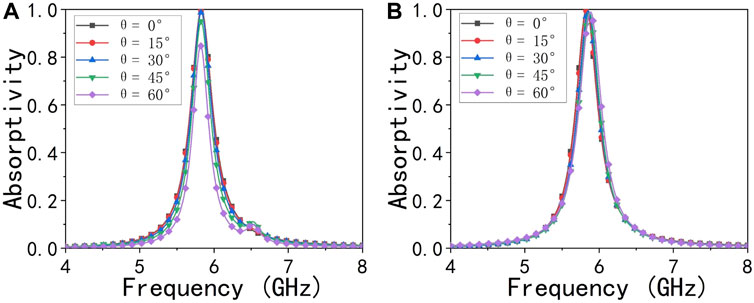
Figure 5. Absorption spectra of the metasurface harvester for oblique incidence waves, with the incident angle ranging from 0 to
The energy harvesting efficiency is studied under oblique incidence for both TE and TM polarizations, as shown in Figure 6. It is observed that with the oblique incident angle up to
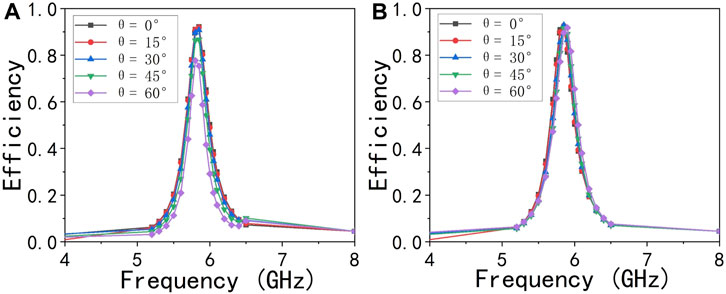
Figure 6. Harvest efficiency of the metasurface harvester for oblique incidence waves, with the incident angle ranging from 0 to
In order to explain the physical mechanism of perfect harvesting, the surface current of the unit cell was studied. We assume that the incident plane wave is a linearly polarized wave that is incident perpendicularly, and its electric component is along the
Table 1 shows the comparison of the proposed design with that in other reported works. As we can see, the proposed design in this paper shows the highest harvesting efficiency, along with the merits of polarization insensitivity and angle stability, which are highly demanded for practical applications. As a final remark, it is worth noting that, although the metasurface energy harvester in this paper is designed for operating at microwave frequencies, a similar concept could also be extended to THz or optical frequencies by exploring the design of metasurface absorbers [17, 19].
4 Conclusion
In conclusion, this paper has introduced a high-performance metasurface energy harvester tailored for electromagnetic energy harvesting. Leveraging the full absorption concept, we have designed a metasurface harvester that boasts a remarkable harvesting efficiency of up to 92.3% at a frequency of 5.85 GHz. This innovative design not only offers exceptional efficiency but also demonstrates polarization independence and a wide-angle incident frequency response, ensuring robust harvesting characteristics across various conditions. The metasurface harvester’s ability to perform efficiently, regardless of polarization or angle of incidence, makes it a versatile and reliable solution for electromagnetic energy harvesting, paving the way for advanced energy-harvesting technologies in the future. This design represents a significant step forward in the field of energy harvesting, opening up new possibilities for sustainable and efficient energy solutions.
Data availability statement
The raw data supporting the conclusions of this article will be made available by the authors, without undue reservation.
Author contributions
AC: writing–original draft. SX: writing–review and editing. YH: writing–review and editing. LZ: writing–review and editing. CL: writing–review and editing. ZC: writing–review and editing.
Funding
The author(s) declare that financial support was received for the research, authorship, and/or publication of this article. The Preresearch Project on Civil Aerospace Technologies funded by the China National Space Administration (grant no. D010105).
Conflict of interest
The authors declare that the research was conducted in the absence of any commercial or financial relationships that could be construed as a potential conflict of interest.
Publisher’s note
All claims expressed in this article are solely those of the authors and do not necessarily represent those of their affiliated organizations, or those of the publisher, the editors, and the reviewers. Any product that may be evaluated in this article, or claim that may be made by its manufacturer, is not guaranteed or endorsed by the publisher.
References
1. Shao L, Zhu W. Electrically reconfigurable microwave metasurfaces with active lumped elements: a mini review. Front Mater (2021) 8:689665. doi:10.3389/fmats.2021.689665
2. Wu X, Li Z, Zhang Z, Wang X, Si L, Zhu W. Mechanically reconfigurable folded reflectarray antenna for variable near-field focusing. IEEE Trans Antennas Propagation (2023) 71:10038–43. doi:10.1109/tap.2023.3323092
3. Li Q, Zhang J, Liu L, He C, Zhu W. Graphene-based optically transparent metasurface for microwave and terahertz cross-band stealth utilizing multiple stealth strategies. Carbon (2024) 219:118833. doi:10.1016/j.carbon.2024.118833
4. Nie MJ, Yang XX, Tan GN, Han B. A compact 2.45-ghz broadband rectenna using grounded coplanar waveguide. IEEE Antennas Wireless Propagation Lett (2015) 14:986–9. doi:10.1109/lawp.2015.2388789
5. Song C, Huang Y, Carter P, Zhou J, Yuan S, Xu Q, et al. A novel six-band dual cp rectenna using improved impedance matching technique for ambient rf energy harvesting. IEEE Trans Antennas Propagation (2016) 64:3160–71. doi:10.1109/tap.2016.2565697
6. Liu B, Zhu W, Gunapala SD, Stockman MI, Premaratne M. Open resonator electric spaser. ACS Nano (2017) 11:12573–82. doi:10.1021/acsnano.7b06735
7. Xu X, Wu Q, Pang Y, Cao Y, Fang Y, Huang G, et al. Multifunctional metamaterials for energy harvesting and vibration control. Adv Funct Mater (2022) 32:2107896. doi:10.1002/adfm.202107896
8. Chen Z, Guo B, Yang Y, Cheng C. Metamaterials-based enhanced energy harvesting: a review. Physica B: Condensed Matter (2014) 438:1–8. doi:10.1016/j.physb.2013.12.040
9. Li J, Jin R, Geng J, Liang X, Wang K, Premaratne M, et al. Design of a broadband metasurface luneburg lens for full-angle operation. IEEE Trans Antennas Propagation (2019) 67:2442–51. doi:10.1109/tap.2018.2889006
10. Akram MR, Bai X, Jin R, Vandenbosch GAE, Premaratne M, Zhu W. Photon spin hall effect-based ultra-thin transmissive metasurface for efficient generation of oam waves. IEEE Trans Antennas Propagation (2019) 67:4650–8. doi:10.1109/tap.2019.2905777
11. Ramahi OM, Almoneef TS, AlShareef M, Boybay MS. Metamaterial particles for electromagnetic energy harvesting. Appl Phys Lett (2012) 101:173903. doi:10.1063/1.4764054
12. Alavikia B, Almoneef TS, Ramahi OM. Complementary split ring resonator arrays for electromagnetic energy harvesting. Appl Phys Lett (2015) 107:033902. doi:10.1063/1.4927238
13. Zhong HT, Yang XX, Tan C, Yu K. Triple-band polarization-insensitive and wide-angle metamaterial array for electromagnetic energy harvesting. Appl Phys Lett (2016) 109:253904. doi:10.1063/1.4973282
14. Shang S, Yang S, Shan M, Liu J, Cao H. High performance metamaterial device with enhanced electromagnetic energy harvesting efficiency. AIP Adv (2017) 7:105204. doi:10.1063/1.5002165
15. Yu F, Yang X, Zhong H, Chu C, Gao S. Polarization-insensitive wide-angle-reception metasurface with simplified structure for harvesting electromagnetic energy. Appl Phys Lett (2018) 113:123903. doi:10.1063/1.5046927
16. Wei Y, Jing H, Deng H, Song C, Duan J, Wang J, et al. A dual-band, polarization-insensitive, wide-angle metasurface array for electromagnetic energy harvesting and wireless power transfer. Results Phys (2023) 46:106261. doi:10.1016/j.rinp.2023.106261
17. Huang TY, Tseng CW, Yeh TT, Yeh TT, Luo CW, Akalin T, et al. Experimental realization of ultrathin, double-sided metamaterial perfect absorber at terahertz gap through stochastic design process. Sci Rep (2015) 5:18605. doi:10.1038/srep18605
18. Hsiao HH, Liu AY. Ultrasensitive and broadband optical toroidal modes in all-dielectric nanostructures. Laser Photon Rev (2022) 16:2100404. doi:10.1002/lpor.202100404
Keywords: metasurface, energy harvester, high-efficiency, wide-angle, absorption
Citation: Cao A, Xu S, Huang Y, Zhang L, Liu C and Chen Z (2024) High-efficiency and wide-angle metasurface electromagnetic energy harvester. Front. Phys. 12:1423036. doi: 10.3389/fphy.2024.1423036
Received: 25 April 2024; Accepted: 01 July 2024;
Published: 13 August 2024.
Edited by:
Jin Zhang, Aalto University, FinlandReviewed by:
Hsin Yu Yao, National Chung Cheng University, TaiwanJun Ding, East China Normal University, China
Copyright © 2024 Cao, Xu, Huang, Zhang, Liu and Chen. This is an open-access article distributed under the terms of the Creative Commons Attribution License (CC BY). The use, distribution or reproduction in other forums is permitted, provided the original author(s) and the copyright owner(s) are credited and that the original publication in this journal is cited, in accordance with accepted academic practice. No use, distribution or reproduction is permitted which does not comply with these terms.
*Correspondence: Zhansheng Chen, zhansheng.chen@ieee.com