- 1SEECS Photonics Research Group, Islamabad, Pakistan
- 2Electrical Engineering Department, HITEC University Taxila, Taxila, Pakistan
- 3Electrical Engineering Department, Mirpur University of Science and Technology, Mirpur, Pakistan
- 4Electrical Engineering Department, University of Azad Jammu and Kashmir, Muzaffarabad, Pakistan
- 5SEECS, National University of Science and Technology, Islamabad, Pakistan
- 6Department of Physics and Astronomy, Uppsala University, Uppsala, Sweden
- 7Optiwave Systems Inc., Ottawa, ON, Canada
- 8Department of Computer Science, College of Computer and Information Sciences, King Saud University, Riyadh, Saudi Arabia
The safety of SCUBA divers remains at high risk in deep-sea owing to multiple factors such as dangerous surrounding, rely upon technical equipment necessary for life support, decreased underwater navigation, and communication infrastructure. Gradual decrease and increase in water temperature and pressure corresponding to depth are among the most common problems that cause most of the fatalities in deep-sea diving. Therefore, different gadgets for accurate measurement of vital parameters, reliable navigation, and seamless communication are of prime importance. In this paper, we propose an all-optical technique for local and remote monitoring of underwater temperature and pressure for deep-sea SCUBA divers based on fiber Bragg grating (FBG) sensors and underwater optical communication-single mode fiber (UWOC-SMF) integrated transmission system. The proposed technique is implemented using two FBG temperature and pressure sensors fixed over diver’s suit and UWOC-SMF integrated transmission system for simultaneous local and remote monitoring of underwater temperature and pressure. Remote monitoring of underwater temperature and pressure is achieved at ship station through a remotely operated underwater vehicle (ROV) and UWOC-SMF integrated transmission system by means of shifts in the original Bragg wavelengths of sensors due to temperature and pressure variations. The performance of the sensors is analyzed for pressure and temperature in the range of 0 to 6.4 MPa (
1 Introduction
In the latest technological era, humans have generally explored nearly all of Earth’s continental surface except Antarctica, conquered space, and contemplated the possibility of multiple universes [1]. However, they lack the same accomplishment in the underwater domain when the oceans cover almost 71% of Earth’s surface and yet this area is almost unexplored [1, 2]. Over the past few decades, the humans have attempted to explore the universe under oceans to exploit the hidden resources for utilization of mankind but only 5% of the oceans have been explored so far [3]. Now, more than ever before in human history, tools and technology are increasingly providing oceanographers with opportunities to explore the deep-sea. However, underwater exploration faces many challenges that hinder the humans to achieve the required goals because the underwater environment is not a natural habitat for humans [4].
SCUBA diving, acronym for “Self Contained Underwater Breathing Apparatus,” is a form of underwater diving where divers use a portable breathing suit equipped with various devices that help them to explore the underwater world [5]. SCUBA diving may be done recreationally or professionally. The professional activities are related to works such as repairing jobs (e.g., oil and gas pipelines, port infrastructure, cable jointing, etc.), scientific research (e.g., sample collection, underwater archaeology, marine biology, coral reef conservation, environmental monitoring, etc.), and public safety missions (e.g., military operations, rescue missions, etc.) while the recreational diving involves any venture whose only purpose is recreation and enjoyment including underwater archaeological tourism [6, 7]. However, deep-sea diving poses several challenges, primarily due to the extreme environment of the oceans. Main challenges are temperature, pressure, visibility, aquatic sicknesses, isolation, and technical equipment related issues such as air supply, Nitrogen narcosis, telecommunication, and navigation [7]. Overall, deep-sea diving requires specialized training, equipment, and careful planning to ensure divers’ safety and mission’s success but the design and development of accurate and reliable sensors, navigation devices, and communication systems are readily identified as main topics to be addressed [4].
The percentage of divers’ fatalities specifically due to underwater pressure and temperatures can vary depending on several factors, including the diving environment, diver’s experience, equipment, and adherence to safety protocols. However, these factors are among the key contributors to diving accidents. Therefore, the local and remote monitoring of underwater pressure and temperature is crucial for deep-sea divers for several reasons. For example, pressure sensors help the divers to monitor the depth, ensuring they do not descend to dangerous levels where they could experience nitrogen narcosis, barotrauma, and other physiological effects [8]. Similarly, temperature sensors provide information about water temperature, which is important for selecting the right exposure protection to prevent the hypothermia [8]. Therefore, the research on the design and development of efficient pressure and temperature monitoring system for sea diving is significant, enabling the diving activities safe and productive.
1.1 Related work
Underwater temperature and pressure sensors have been extensively studied over the past few decades. For instance, Liu et al. [9] demonstrated an optical sensor based on two cascaded external Fabry-Perot interferometers (EFPIs) and a fiber Bragg grating (FBG) for monitoring temperature, depth, and salinity in the deep-sea. The proposed sensor can measure pressure, salinity, and temperature in the range of 0–20 MPa (
Different methods were discussed to solve the problem of overlap among reflected spectra to enhance the accuracy of peak wavelength detection.
In order to further elaborate the literature survey, we have shown the main contributions of past studies in Table 1. We report a technique for measurement of underwater temperature and pressure for deep-sea divers based on two FBG sensors and UWOC-SMF integrated transmission system through numerical simulation. The FBG sensors are fixed over diver’s suit and UWOC-SMF integrated transmission system is used to transmit the sensors’ signals to respective FBG interrogators (FBGIs) installed at diver and ship station for simultaneous local and remote measurement of underwater temperature and pressure, respectively. Performance of the sensors is analyzed for temperature and pressure in the range of 0–6.4 MPa and 40 to −2°C, respectively corresponding to different depths. The results show that a temperature sensitivity of 4.3 p.m./°C and a pressure sensitivity of 30.5 p.m./MPa are achieved.
Based upon above argument, the main achievements of this study are as under.
1. Monitoring of underwater temperature and pressure for deep-sea divers based on FBG sensors and UWOC-SMF integrated transmission system.
2. The FBG sensors are fixed over diver’s suit and UWOC-SMF integrated transmission system is used to transmit the sensors’ signals to respective FBGIs installed at diver and ship station for simultaneous local and remote monitoring of underwater temperature and pressure, respectively.
3. The performance of the FBG sensors is analyzed for underwater temperature and pressure in the range of 0–7 MPa and 40 to −2°C, respectively corresponding to different depths.
4. A temperature sensitivity of 4.3 p.m./oC and a pressure sensitivity of 30.5 p.m./MPa are achieved.
In this work, Optiwave Inc.’s OptiSystem (version 21) was used to design the sensor architecture and transmission system and analyze the overall performance [19]. The structure of the paper is as follows. Section 2 provides a theoretical overview, Section 3 describes the proposed setup, Section 4 discusses the results, and Section 5 concludes the paper.
1.2 The application plan of the proposed study
Despite advances in robotic technology, diving remains the dominant method for many underwater applications as discussed earlier [20]. Diving is not only considered for completing many complex tasks in deep-sea, but is also a popular form of leisure activity. To improve divers’ safety and enable new operational scenarios, a robust and reliable sensing, communication, and navigation system will be of great help. Many diving expeditions are usually monitored remotely by an operator on a ship station who observes the divers’ trajectory and various important parameters related to that particular dive [20]. Operators can be very helpful provided they have the ability to simultaneously (I) sense various safety related parameters such as underwater temperature, depth, oxygen tank pressure, etc., (II) track the divers’ location and movement, and (III) seamlessly communicate with the divers. Such applications will greatly improve diver safety.
An application scenario of the proposed work as envisioned by the experts has been shown in Figure 1. It may be observed that the underwater network consists of various kinds of sensor nodes, relays, underwater vehicles, divers, and ship stations interconnected with each other through optical wirless, RF, and fiber optic links. Diver safety can be further increased by using ROVs to monitor participants during diving expeditions. Monitoring the divers from a ship station allows operators to monitor underwater activity in real time and ensure that divers are safe and following required safety protocols. Specifically, the underwater temperature and pressure is measured using FBG sensors installed at the diving suit and locally interrogated by the diver enabling him to get awareness about these parameters. Moreover, these parameters can be remotely monitored at the same time at the ship station for data logging or to alert the diver about dangerous zones in deep-sea.
2 Theoretical background
2.1 Sensing fundamental
Figure 2 shows a block diagram of a Bragg grating fabricated within the core of an optical fiber and its response to an applied optical signal from a broadband light source, such as white light source (WLS) shown in the inset of Figure 2. It can be seen that
The grating period of an FBG sensor can be changed due to physical disturbances such as stress, strain, and temperature [21]. The change in Bragg wavelength is directly interrelated to the change in strain
Where
2.2 Line-of-sight underwater channel model
The line-of-sight (LOS) underwater channel between diver and ROV is modelled considering the underwater optical signal transmission between two telescopes that are on a line of sight for different types of waterbodies. UWOC is highly susceptible to the negative effects of absorption
The values of
Where
3 Proposed setup
The proposed setup has been depicted in Figure 3. Let us assume that the diver’s suit is equipped with two FBG sensors and a part of UWOC-SMF integrated transmission system. The broadband optical signal in visible range from a WLS operating at 532 nm whose spectral plot is shown in Figure 4, is split into two parts using a 50:50 power splitter (PS).
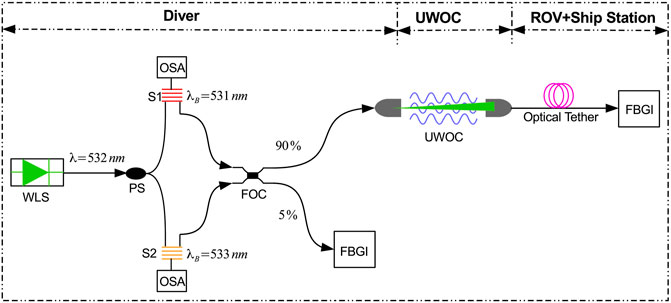
Figure 3. Block diagram of the proposed setup, WLS: White light source, PS: Power splitter, S: FBG sensor, FOC: Fused optical coupler, UWOC: Underwater optical communication channel, FBGI: Fiber Bragg grating interrogator, OSA: Optical spectrum analyzer.
Both of the parts are applied to the sensing ports of the FBGs as shown in block diagram. The original Bragg wavelengths of FBG temperature sensor (S1) and pressure sensor (S2) are 531 nm and 533 nm, respectively. As the diver descends or ascends in the deep-sea of an average depth of 0.7 km, the underwater temperature and pressure vary corresponding to different depths achieved by the diver resulting in a shift in original Bragg wavelengths of the sensors. This scenario is emulated in software by sweeping the temperature and pressure in the range of 40 to −2°C and 0–7 MPa, respectively. It is pertinent to mention here that the reason of selecting the average depth of 0.7 km in this work is that maximum depth that can be achieved for saturation diving is around 1 km [25]. Moreover, the underwater temperature varies in the range of 40 to −2°C [26] while the pressure increases by a factor of 0.101 MPa for every 10 m as per recommendation of National Oceanic and Atmospheric Administration (NOAA) [27]. The effect of pressure on the wavelength shift of the S2 is much more compared to the variation due to the temperature. Typically, there might be an overlap between both effects on the wavelength shift of the sensors that causes ambiguity. However, using calibration tables and proper choice of the center wavelength of sensors, it is possible to synthesize the effect when both temperature and pressure produce any wavelength shift and avoid ambiguities. However, the measurement resolution can be affected. Moreover, it is crucial to choose the FBGs’ center wavelengths to avoid ambiguity by dedicating an FBG for temperature monitoring with operating wavelength range away from the other FBG used for the pressure. Due to the small range of wavelength shift of S1, the resolution on the S2 could be limited to 0.7 MPa to allow synthesizing the temperature variation such that a combined temperature and pressure can be simulated and sensed. This process can be designed using the sensitivity (slope) parameter of the sensors. The reflected optical signals from sensors at shifted Bragg wavelengths corresponding to the swept values of the temperature and pressure in the range of 40 to −2°C and 0–7 MPa, respectively are combined using 5:95 cross coupler (x-coupler) which is basically a fused optical coupler (FOC).
For simultaneous local and remote monitoring of temperature and pressure at diver and ship station respectively, an integrated transmission system based on UWOC-SMF hybrid link and two FBGIs is designed as shown in Figure 3. Optical signal from 5% port of the FOC is given to a FBGI for local demodulation of sensors and monitoring of the temperature and pressure by the diver. Similarly, optical signal from 95% port of the x-coupler is given as input to transmitter telescope and then transmitted over 50 m long LOS UWOC channel towards receiver telescope installed at ROV. It is necessary to mention here that we have considered pure sea in this work which is characterized by
The important simulation parameters used in this work are shown in Table 2.
4 Results and discussion
Figures 5A,B show the plots between wavelength shifts and underwater temperature and pressure, respectively. These plots are obtained using OSAs connected at the reflection ports of S1 and S2 before x-coupler when underwater temperature and pressure are swept in software in the range of 40 to −2°C and 0–7 MPa, respectively and corresponding shifts in reflected wavelengths of sensors are obtained. It is evident that a temperature sensitivity of 4.3 p.m./oC and a pressure sensitivity of 30.5 p.m./MPa is achieved which is comparable to sensitivity values obtained in various experimental works as mentioned in Table 1.
Figure 6 shows the reflected and transmitted spectra of S1 and S2 at shifted wavelengths for different underwater temperature and pressure values when underwater temperature and pressure are swept in the range of 40 to −2°C and 0–7 MPa, respectively. Plots (a) and (c) are obtained using OSAs connected at the reflection ports of S1 and S2 before x-coupler while plots (b) and (d) are obtained using OSAs connected at the transmission ports of S1 and S2 when underwater temperature and pressure are swept in software in the range of 40 to −2°C and 0–7 MPa, respectively.
Figures 7A–C show the traces of reflected spectra of S1 and S2 at shifted wavelengths corresponding to three different values of temperature and pressure for local monitoring of underwater temperature and pressure by diver. These plots are obtained using FBGI connected at the 5% port of x-coupler as shown in Figure 3. Similarly, Figures 7D–F show the traces of reflected spectra of S1 and S2 at shifted wavelengths corresponding to three different values of temperature and pressure for remote monitoring of underwater temperature and pressure by an operator on-board at ship station. These plots are also obtained using FBGI at the ship station as shown in Figure 3. It is evident that the reflected spectra of S1 and S2 are attenuated due to adverse effects of UWOC-SMF hybrid link such as absorption, scattering, and attenuation losses. However, the reflected spectra of S1 and S2 after signal transmission over UWOC-SMF hybrid link have sufficient power margin required for proper demodulation of sensors at FBGI as shown in Figure 7.
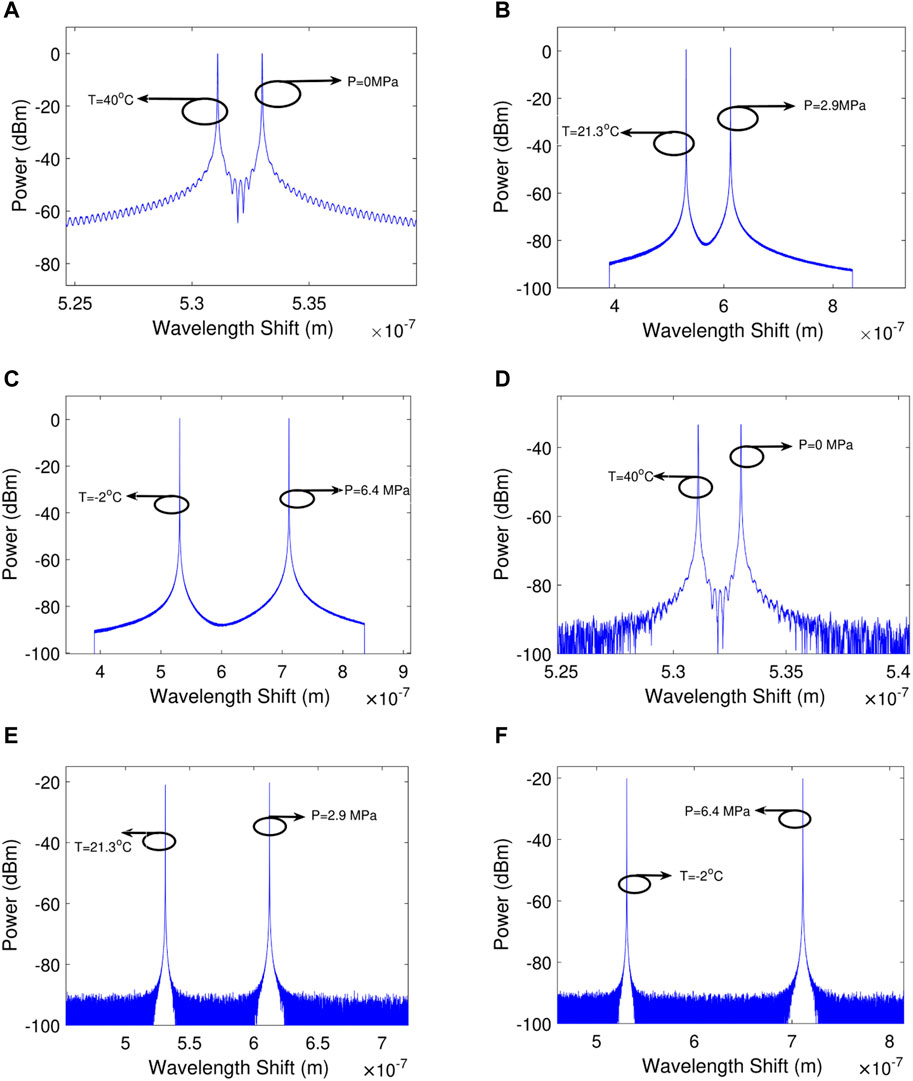
Figure 7. (A), (B), and (C) Spectral traces of S1 and S2 for local monitoring of underwater temperature and pressure (D), (E), and (F) Spectral traces of S1 and S2 for remote monitoring of underwater temperature and pressure. These traces are obtained for three different values of temperature and pressure.
5 Conclusion
Local and remote monitoring of underwater temperature and pressure for deep-sea SCUBA divers based on fiber Bragg grating sensors and underwater optical communication and single-mode fiber integrated transmission system is proposed and implemented using numerical simulations. Temperature and pressure sensors are fixed over diver’s suit. The sensors’ signals are transmitted using underwater optical communication and single-mode fiber based hybrid link towards respective interrogators for simultaneous local and remote monitoring of underwater temperature and pressure. The performance of the sensors is evaluated for temperature and pressure in the range of 0–7 MPa and 40 to −2°C, respectively corresponding to different depths achieved by the diver. The results show that temperature sensitivity of 4.3 p.m./oC and a pressure sensitivity of 30.5 p.m./MPa are achieved.
Data availability statement
The original contributions presented in the study are included in the article/Supplementary Material, further inquiries can be directed to the corresponding author.
Author contributions
JM: Conceptualization, Investigation, Project administration, Supervision, Writing–original draft. FK: Software, Writing–original draft, Writing–review and editing. US: Writing–original draft, Writing–review and editing. SG: Software, Writing–review and editing. IA: Funding acquisition, Writing–review and editing. AAt: Software, Writing–review and editing. AAl: Conceptualization, Writing–review and editing. AH: Writing–review and editing. BK: Formal Analysis, Software, Writing–review and editing.
Funding
The author(s) declare that financial support was received for the research, authorship, and/or publication of this article.
Acknowledgments
This work was supported by King Saud University, Riyadh, Saudi Arabia, through Researchers Supporting Project number (RSP2024R184).
Conflict of interest
Author AAt was employed by the Optiwave Systems Inc.
The remaining authors declare that the research was conducted in the absence of any commercial or financial relationships that could be construed as a potential conflict of interest.
Publisher’s note
All claims expressed in this article are solely those of the authors and do not necessarily represent those of their affiliated organizations, or those of the publisher, the editors and the reviewers. Any product that may be evaluated in this article, or claim that may be made by its manufacturer, is not guaranteed or endorsed by the publisher.
References
1. Islam KY, Ahmad I, Habibi D, Zahed MIA, Kamruzzaman J. Green underwater wireless communications using hybrid optical-acoustic technologies. IEEE Access (2021) 9:85109–23. doi:10.1109/access.2021.3088467
2. Zeng Z, Fu S, Zhang H, Dong Y, Cheng J. A survey of underwater optical wireless communications. IEEE Commun Surv tutorials (2016) 19(1):204–38. doi:10.1109/comst.2016.2618841
3. Ocean exploration. Technology. Available from: https://education.nationalgeographic.org/resource/ocean-exploration/
4. Nad D, Mandic F, Miskovic N. Using autonomous underwater vehicles for diver tracking and navigation aiding. J Mar Sci Eng (2020) 8(6):413–29. doi:10.3390/jmse8060413
5. Scuba diving. Available from: https://www.scubadiving.com/ (Accessed March 10 2024).
7. Cibis T, McEwan A, Sieber A, Eskofier B, Lippmann J, Friedl K, et al. Diving into research of biomedical engineering in scuba diving. IEEE Rev Biomed Eng (2017) 10:323–33. doi:10.1109/rbme.2017.2713300
8. Tetzlaff K, Friege L, Koch A, Heine L, Neubauer B, Struck N, et al. Effects of ambient cold and depth on lung function in humans after a single scuba dive. Eur J Appl Physiol (2001) 85:125–9. doi:10.1007/s004210100421
9. Liu Y, Jing Z, Liu Q, Li A, Peng W, Cheung Y, et al. All-silica fiber-optic temperature-depth-salinity sensor based on cascaded EFPIs and FBG for deep sea exploration. Opt Express (2021) 29(15):23953–66. doi:10.1364/oe.432943
10. Fu G, Wang K, Liu C, Su L, Fu X, Jin W, et al. Study on the sensing characteristics of Salinity-Temperature-Depth based on SNS-FBG cascade. Opt Commun (2024) 552:130092. doi:10.1016/j.optcom.2023.130092
11. Hsu C-Y, Chiang C-C, Hsieh T-S, Chen T-H, Chen Y-H. A study of strain measurement in cylindrical shells subjected to underwater shock loading using FBG sensors. Optik (2020) 217:164701. doi:10.1016/j.ijleo.2020.164701
12. Razali NF, Bakar MHA, Tamchek N, Yaacob MH, Latif AA, Zakaria K, et al. Fiber Bragg grating for pressure monitoring of full composite lightweight epoxy sleeve strengthening system for submarine pipeline. J Nat Gas Sci Eng (2015) 26:135–41. doi:10.1016/j.jngse.2015.06.020
13. Li P, Chen Y, Hu J, Zhang G, Cui B, Meng L, et al. Simultaneous measurement of salinity and temperature of seawater based on U-shaped tapered no-core fiber. Infrared Phys Technology (2023) 130:104617. doi:10.1016/j.infrared.2023.104617
14. Ahmad Z, Geisar P, Salih O, Rajbhandari S. Design of a visible light communication system for deep sea divers based on analogue frequency modulation. In: 10th IEEE international symposium on communication systems, networks and digital signal processing (CSNDSP) (2016). p. 1–5.
15. Hafizi ZM, Vorathin E. A temperature-compensated FBG pressure sensor for underwater pipeline monitoring. In: 8th IEEE international conference on photonics (ICP) (2020). p. 66–7.
16. Amos S, Prabhu R, Njuguna J. Theoretical design and analysis of a sensing system for high pressure and temperature measurement in subsea underwater applications. In: Ieee oceans (2017). p. 1–7.
17. Duraibabu DB, Leen G, Toal D, Newe T, Lewis E, Dooly G. Underwater depth and temperature sensing based on fiber optic technology for marine and fresh water applications. Sensors (2017) 17(6):1228–40. doi:10.3390/s17061228
18. Dehnaw AM, Manie YC, Du L-Y, Yao C-K, Li Y-L, Hayle ST, et al. Bidirectional free space optics communication for long-distance sensor system. J Lightwave Technol (2023) 41(18):5870–8. doi:10.1109/jlt.2023.3270864
19. OptiSystem. Available from: https://optiwave.com/products/optisystem/ (Accessed March 10 2024).
20. Anjangi P, Gibson A, Ignatius M, Pendharkar C, Kurian A, Low A, et al. Diver communication and localization system using underwater acoustics. In: IEEE global oceans. Singapore: Singapore-US Gulf Coast (2020). p. 1–8.
21. Presti DL, Massaroni C, D Abbraccio J, Massari L, Caponero M, Longo UG, et al. Wearable system based on flexible FBG for respiratory and cardiac monitoring. IEEE Sensors J (2019) 19(17):7391–8. doi:10.1109/jsen.2019.2916320
22. Abro ZA, Hong C, Zhang Y, Siddiqui MQ, Abbasi AMR, Abro Z, et al. Development of FBG pressure sensors using FDM technique for monitoring sleeping postures. Sensors Actuators A: Phys (2021) 331:112921. doi:10.1016/j.sna.2021.112921
23. Arnon S, Kedar D. Non-line-of-sight underwater optical wireless communication network. JOSA A (2009) 26(3):530–9. doi:10.1364/josaa.26.000530
25. Abraini JH, David HN, Vallee N, Risso J-J. Theoretical considerations on the ultimate depth that could be reached by saturation human divers. Med Gas Res (2016) 6(2):119–21. doi:10.4103/2045-9912.184722
26. NOAA’s recommendation for underwater temperature. Available from: https://oceanexplorer.noaa.gov/facts/temp-vary.html (Accessed March 10 2024).
27. NOAA’s recommendation for underwater pressure. Available from: https://oceanservice.noaa.gov/facts/pressure.html (Accessed March 10 2024).
Keywords: SCUBA diving, FBG sensors, underwater optical communication, line-of-sight, remotely operated underwater vehicle, ship station, hydrostatic pressure
Citation: Mirza J, Kanwal F, Salaria UA, Ghafoor S, Aziz I, Atieh A, Almogren A, Haq AU and Kanwal B (2024) Underwater temperature and pressure monitoring for deep-sea SCUBA divers using optical techniques. Front. Phys. 12:1417293. doi: 10.3389/fphy.2024.1417293
Received: 14 April 2024; Accepted: 08 July 2024;
Published: 29 July 2024.
Edited by:
Rajib Biswas, Tezpur University, IndiaReviewed by:
Muhammad Hafiz Abu Bakar, Putra Malaysia University, MalaysiaWei Ren, Xi’an University of Posts and Telecommunications, China
Copyright © 2024 Mirza, Kanwal, Salaria, Ghafoor, Aziz, Atieh, Almogren, Haq and Kanwal. This is an open-access article distributed under the terms of the Creative Commons Attribution License (CC BY). The use, distribution or reproduction in other forums is permitted, provided the original author(s) and the copyright owner(s) are credited and that the original publication in this journal is cited, in accordance with accepted academic practice. No use, distribution or reproduction is permitted which does not comply with these terms.
*Correspondence: Imran Aziz, imran.aziz@physics.uu.se