- 1The 53th Research Institute of China Electronics Technology Corporation, Jinzhou, China
- 2National Key Laboratory of Electromagnetic Space Security, Tianjin, China
In this paper, we demonstrated an efficient degenerate middle infrared (mid-IR) ZGP-OPO that is pumped by an electro-optically Q-switched diode-pumped Tm:YAP laser. The Tm:YAP laser, operating at a repetition rate of 1 kHz, produced a peak pulse energy of 9.66 mJ at 1.94 μm and a minimum pulse width of 25 ns. Utilizing the Tm:YAP laser as the pump source, a peak average output power of 3.14 W at 3.88 μm was achieved in the degenerate ZGP-OPO with an incident Tm power of 9.4 W, resulting in a slope efficiency of 62.7% and an optical conversion efficiency of 33.4%. The minimum pulse width was measured at 21 ns, leading to a peak power of 149.5 kW. Additionally, the beam quality factor of the degenerate mid-IR ZGP-OPO was evaluated to be approximately 2.7 at the maximum output power level.
1 Introduction
Solid-state lasers operating in the mid-infrared (mid-IR) spectral range hold significant appeal for various applications, including remote sensing, spectroscopy, medical procedures, environmental monitoring, national defense, and more. Optical parametric oscillators (OPO) present an exceptional method for producing high-performance mid-IR laser radiation [1–3]. Among the myriad of OPO materials, ZnGeP2 (ZGP) crystal stands out due to its remarkable attributes, such as a large nonlinear coefficient, high thermal conductivity, and broad transmission range, thereby enabling high nonlinear conversion efficiency in the mid-IR spectral range [4–8]. The room-temperature high-performance Ho lasers operating at around 2.1 μm [9, 10] were good pump source for generation of mid-IR laser radiation. With Ho pumping, mid-IR ZGP-OPO has demonstrated the capability to achieve output powers in the hundreds of watts with high optical conversion efficiency [11, 12].
In addition to Ho lasers, Tm lasers operating at 1.9 μm serve as efficient pump sources for mid-IR ZGP-OPO. In 2003, a mid-IR ZGP-OPO, pumped by an acousto-optically (AO) Q-switched Tm:YAP laser at a repetition rate of 10 kHz, was reported to achieve a slope efficiency of 40%, an average output power of 3 W, and a pulse width of less than 50 ns [13]. Subsequently, in 2008, a pulse energy of 1 mJ at a repetition rate of 100 Hz was obtained in a mid-IR ZGP-OPO driven by an electro-optically (EO) Q-switched Tm:YAG laser at 2.02 μm [14]. In the same year, under the pumping of pulsed Tm fibers at 2 μm, the ZGP-OPO delivered 20 ns mid-IR pulses and 658 mW average output power at a repetition rate of 30 kHz [15]. In 2014, as a peak power of 27.9 kW was achieved in a mid-IR ZGP-OPO pumped by a Tm fiber laser with a repetition rate of 4 kHz [16]. Subsequently, in 2018, the utilization of a passively Q-switched Tm:YAP laser at 1.94 μm as the pump source resulted in an average output power of 2.3 W and an optical conversion efficiency of 58% in mid-IR ZGP-OPO at a repetition rate of 6.2 kHz [17]. Most recently, in 2023, an efficient mid-IR ZGP-OPO, pumped by an AO Q-switched Tm:YLF laser at 1908 nm, was demonstrated to achieve an average output power of 17.8 W and a pulse width of 197 ns at a repetition rate of 15 kHz [18].
While the Tm lasers can efficiently pump mid-IR ZGP-OPO systems, the wider pulse widths or lower pulse energies often lead to reduced peak power levels, limiting their suitability for certain technical applications. In contrast, electro-optic (EO) Q-switches offer rapid switching speeds and high extinction ratios, enabling the generation of narrower pulse widths and higher peak powers. In this study, we report, to the best of our knowledge, the first utilization of an EO Q-switched Tm:YAP laser as the pump source for a degenerate mid-IR ZGP-OPO. For the pump laser source, a maximum pulse energy of 9.66 mJ at 1.94 μm and a minimum pulse width of 25 ns was obtained with a repletion rate of 1 kHz. Under Tm:YAP pumping, a maximum average output power of 3.14 W at 3.88 μm was realized in the degenerate ZGP-OPO configuration, with an incident Tm power of 9.4 W, resulting in a slope efficiency of 62.7% and an optical conversion efficiency of 33.4%. Notably, the system achieved a minimum pulse width of 21 ns in the mid-IR ZGP-OPO, corresponding to a peak power of 149.5 kW. Additionally, the beam quality factor (M2) of the degenerate mid-IR ZGP-OPO was measured to be approximately 2.7 at the maximum output power level.
2 Experimental setup
The schematic diagram of the degenerate mid-IR ZGP-OPO setup, driven by an electro-optically Q-switched Tm:YAP laser, is illustrated in Figure 1. The Tm:YAP laser utilized a fiber-coupled LD (nLight Corp.) with a maximum output power of 80 W, featuring a core diameter and numerical aperture of 200 μm and 0.22, respectively. The LD’s central wavelength at maximum output was 795 nm. The pump beam was evenly split into two beams, constituting a dual-end-pumping configuration. By employing a 1:4 telescope comprising two lenses, the pump spot diameter on the Tm:YAP crystal was focused to approximately 800 μm. The Tm:YAP crystal (Dientech Corp.), with dimensions of 2 × 6 × 12 mm³ (length), was cut along its b-axis and had a Tm ion doping concentration of 3.5 at%. Both end faces of the Tm:YAP crystal were coated with antireflection coatings for 795 nm and 1.94 μm. A water-cooled copper heatsink, equipped with a 0.05-mm-thick indium foil, was employed to mount the Tm:YAP crystal. The operating temperature of the heatsink was rigorously controlled at approximately 15°C.
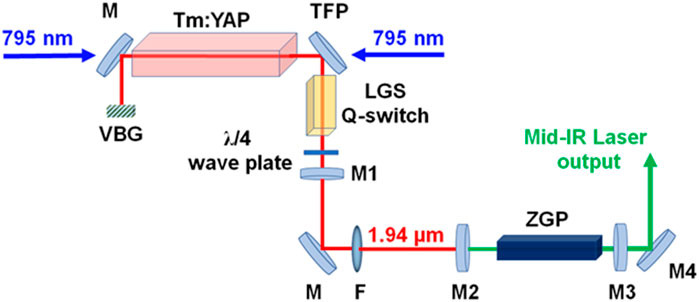
Figure 1. The schematic of experimental setup of degenerate mid-IR ZGP-OPO pumped by an electro-optically Q-switched Tm:YAP laser.
The Tm:YAP laser cavity comprised several components, including a volume Bragg grating (VBG), (OptiGrate Corp.), a flat 45° dichromatic mirror M, a 45° thin-film polarizer (TFP), and an output coupler M1. The VBG exhibited a diffraction efficiency of over 99% at 1940.2 nm. Mirror M was coated to achieve high transmission at 795 nm and high reflectivity at 1.94 μm. The TFP had high reflectivity for s-polarized 1.94-μm light, high transmission for p-polarized 1.94-μm light, and high transmission for 795 nm. The output coupler M1 was a plano-concave mirror with a radius of curvature of 200 mm and an output transmittance of 15%. To alter the polarization of the oscillating beam, an uncoated quarter-wave plate was integrated into the setup. A z-cut uncoated LGS Q-switch (Dientech Corp.) with a length of 48 mm was utilized, with an electro-driver (homemade) featuring a rise time of 10 ns driving the Q-switch. The quarter-wave voltage applied to the Q-switch was set at 2500 V. The EO Q-switch operated in the pulse-on mode. The physical length of the cavity was approximately 90 mm.
A type I ZGP crystal (Dientech Corp.) measuring 6 × 6 × 20 mm³ in length was employed as the optical parametric conversion medium, with a cutting angle of 61° to the c-axis, yielding a degenerate wavelength of 3.88 μm when pumped at 1.94 μm. Both end faces of the ZGP crystal were anti-reflection coated for 1.94 μm and 3.88 μm wavelengths. The ZGP crystal was mounted in a copper heatsink. The Tm pump beam was focused onto the ZGP crystal using a lens F with a focal length of 100 mm, resulting in a spot diameter of 1.2 mm. A simple linear cavity composed of two flat mirrors, M2 and M3, was utilized. Mirror M2 served as the input mirror with high transmission for 1.94 μm and high reflectivity for 3.88 μm. The output mirror M3 had a transmittance of 50% at 3.88 μm and high transmission for 1.94 μm. The physical length of the OPO cavity was set at 100 mm to ensure good beam quality. A flat 45° dichromatic mirror, M4, was employed to separate residual pump and mid-IR laser beams, featuring high reflectivity at 3.88 μm and high transmission at 1.94 μm.
3 Experimental results
A Coherent PM 30 power meter was utilized to measure the power during the experiment. Initially, the continuous wave (CW) output power of the Tm:YAP laser was recorded, as depicted in Figure 2A. Upon switching off the electro-driver and removing the quarter-wave plate, a peak output power of 16.2 W was obtained with an incident LD power of 61.5 W, resulting in a slope efficiency of 36.1% and an optical conversion efficiency of 26.3%. Subsequently, upon switching on the electro-driver and inserting the quarter-wave plate, the output characteristics of the EO Q-switched Tm:YAP laser were investigated, as illustrated in Figure 2A. At a repetition rate of 2 kHz, a maximum average output power of 10.6 W was achieved under the same pump power, corresponding to a slope efficiency of 23.4% and an optical conversion efficiency of 17.2%. In another case, at a repetition rate of 1 kHz, both the maximum average output power and slope efficiency decreased to 9.66 W and 21.3%, respectively. The thermal effect of Tm:YAP crystal can be analyzed by a thermal analysis model [19, 20]. Dual end-pumping architecture balanced the thermal distribution in Tm:YAP crystal under strong pumping conditions, which was beneficial for high-performance output of EO Q-switched Tm:YAP laser. Compared with reported works on the diode-pumped Tm:YAP lasers, the slope efficiency is not high. Un-coated quarter-wave plate and LGS Q-switch were used in this experiment, leading to relatively high insert loss. The output performance of Tm:YAP laser could be increased by employing AR-coated quarter-wave plate and LGS Q-switch. Moreover, the optimal cavity length and output transmittance could improve the output power and slope efficiency.
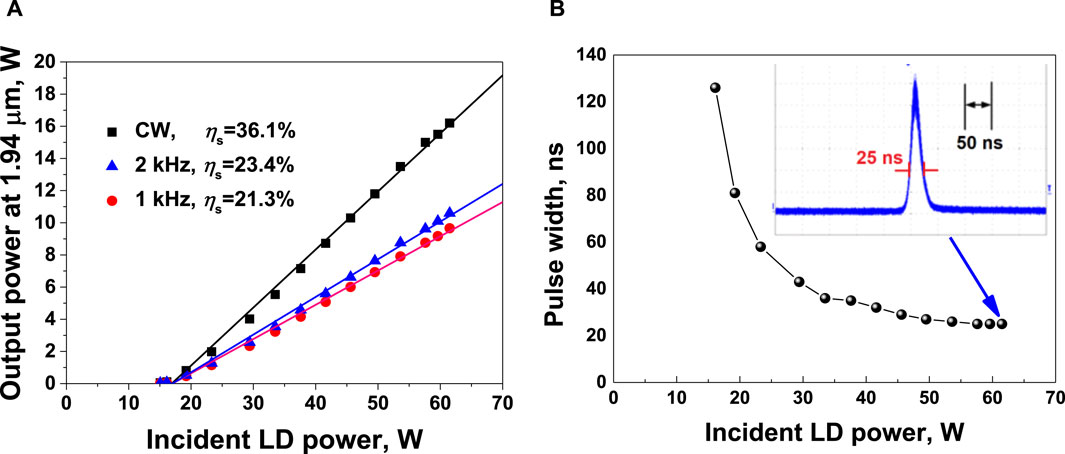
Figure 2. The output characteristics of EO Q-switched Tm:YAP laser, (A) powers (B) pulse widths at repletion rate of 1 kHz.
To measure the pulse width of the EO Q-switched Tm:YAP laser, a high-speed InGaAs photodiode (EOT, ET-5000) connected to an oscilloscope (Lecroy, T3DSO2354A) was utilized. At a repetition rate of 1 kHz, as the incident LD power increased from 16.1 W to 61.5 W, the pulse width decreased from 86 ns to 25 ns. The pulse profile corresponding to the minimum pulse width is displayed in the inset of Figure 2B. By calculating the peak power, it can be observed that it increased from 0.7 kW to 386.4 kW.
The output power of the degenerate mid-IR ZGP-OPO is displayed in Figure 3A. The threshold pump power was approximately 4.77 W, and a maximum average output power of 3.14 W was achieved with an incident Tm power of 9.4 W, resulting in a slope efficiency of 62.7% and an optical conversion efficiency of 33.4%, respectively. With a period of 1 hour, the power stability of degenerate mid-IR ZGP-OPO was approximately 2.3%. To measure the laser pulse of the degenerate mid-IR ZGP-OPO, a HgCdTe detector (Vigo, PVM-10.6) connecting with an oscilloscope (Tektronix, DPO5204B) was employed. At the maximum output level, a minimum pulse width of 21 ns was obtained, corresponding to a calculated peak power of 149.5 kW. The pulse-to-pulse stability of degenerate mid-IR ZGP-OPO was about 10%. Additionally, a monochromator (WDG30P) was utilized to record the degenerate spectrum, as depicted in Figure 3B. A broad oscillating spectrum with a full width at half maximum linewidth of approximately 140 nm was observed. The central wavelength was measured to be around 3,886 nm, indicating the degenerate operation of the mid-IR ZGP-OPO.
To measure the beam mode and beam quality of the mid-IR beam, a lens with a focal length of 100 mm was utilized. The beam radii along the beam propagation direction were measured using the 90/10 knife-edge method, as illustrated in Figure 4. The beam parameters were fitted based on the propagation equation of a Gaussian beam. The calculated M2-factor was approximately 2.7. In addition, a picture of far-field beam spot was taken by a camera (Ophir-spiricon Pyrocam IV), which was inserted in Figure 4. The TEM00 beam propagation was verified.
4 Conclusion
In conclusion, we successfully demonstrated an efficient degenerate mid-IR ZGP-OPO pumped by an electro-optically Q-switched diode-pumped Tm:YAP laser. Our experiment yielded remarkable results, including a maximum pulse energy of 9.66 mJ at 1.94 μm and a minimum pulse width of 25 ns at a repetition rate of 1 kHz. Moreover, under Tm:YAP pumping, we achieved a maximum average output power of 3.14 W at 3.88 μm in the degenerate ZGP-OPO. This result corresponds to a slope efficiency of 62.7% and an optical conversion efficiency of 33.4% with an incident Tm power of 9.4 W. Additionally, we observed a minimum pulse width of 21 ns, resulting in a peak power of 149.5 kW. Furthermore, the M2-factor of the degenerate mid-IR ZGP-OPO was measured to be approximately 2.7 at maximum output power. Limiting by Tm pump level and possibility of coating on the ZGP crystal, more high average output power was not achieved. We believe that more high output power of degenerate mid-IR ZGP-OPO could be reached with higher pump power and larger pump spot diameter. In addition, a longer cavity length would be better for improving the b M2-factor. These results indicate that the degenerate mid-IR ZGP-OPO, pumped by an electro-optically Q-switched diode-pumped Tm:YAP laser, is a promising candidate for high-peak-power all solid-state laser sources in the mid-IR spectral region.
Data availability statement
The original contributions presented in the study are included in the article/Supplementary Material, further inquiries can be directed to the corresponding author.
Author contributions
WD: Writing–review and editing, Writing–original draft, Project administration, Conceptualization. YD: Writing–original draft, Writing–review and editing, Formal Analysis, Data curation. TL: Writing–review and editing, Supervision, Investigation, Conceptualization. YuZ: Writing–review and editing, Supervision, Investigation, Formal Analysis. JG: Writing–review and editing, Investigation, Data curation, Conceptualization. CZ: Writing–review and editing, Validation, Project administration, Formal Analysis.
Funding
The author(s) declare that no financial support was received for the research, authorship, and/or publication of this article.
Conflict of interest
The authors declare that the research was conducted in the absence of any commercial or financial relationships that could be construed as a potential conflict of interest.
The handling editor XD declared a past Authorship with author YD.
Publisher’s note
All claims expressed in this article are solely those of the authors and do not necessarily represent those of their affiliated organizations, or those of the publisher, the editors and the reviewers. Any product that may be evaluated in this article, or claim that may be made by its manufacturer, is not guaranteed or endorsed by the publisher.
References
1. Xing T, Wang L, Hu S, Cheng T, Wu X, Jiang H. Widely tunable and narrow-bandwidth pulsed mid-IR PPMgLN-OPO by self-seeding dual etalon-coupled cavities. Opt Express (2017) 25(25):31810–5. doi:10.1364/oe.25.031810
2. Fu Q, Xu L, Liang S, Shardlow PC, Shepherd DP, Alam S-U, et al. High-average-power picosecond mid-infrared OP-GaAs OPO. Opt Express (2020) 28(4):5741–8. doi:10.1364/oe.380189
3. Yang K, Liu G, Li C, Yao B, Yao J, Chen Y, et al. Research on performance improvement technology of a Baga4Se7 mid-infrared optical parametric oscillator. Opt Lett (2020) 45(23):6418–21. doi:10.1364/ol.403759
4. Budni PA, Pomeranz LA, Lemons ML, Miller CA, Mosto JR, Chicklis EP. Efficient mid-infrared laser using 19-µm-pumped Ho:YAG and ZnGeP_2 optical parametric oscillators. J Opt Soc America B (2000) 17(5):723–8. doi:10.1364/josab.17.000723
5. Ganikhanov F, Caughey T, Vodopyanov KL. Narrow-linewidth middle-infrared ZnGeP_2 optical parametric oscillator. J Opt Soc America B (2001) 18(6):818–22. doi:10.1364/josab.18.000818
6. Duan X, Li L, Shen Y, Yao B, Wang Y. Efficient middle-infrared ZGP-OPO pumped by a Q-switched Ho:LuAG laser with the orthogonally polarized pump recycling scheme. Appl Opt (2018) 57(27):8102–7. doi:10.1364/ao.57.008102
7. Piotrowski M, Medina MA, Schellhorn M, Spindler G, Hildenbrand-Dhollande A. Effects of pump pulse energy and repetition rate on beam quality in a high-power mid-infrared ZnGeP2 OPO. Opt Express (2021) 29(2):2577–86. doi:10.1364/oe.414074
8. Alessandro Medina M, Piotrowski M, Schellhorn M, Wagner FR, Berrou A, Hildenbrand-Dhollande A. Beam quality and efficiency of ns-pulsed high-power mid-IR ZGP OPOs compared in linear and non-planar ring resonators. Opt Express (2021) 29(14):21727–37. doi:10.1364/oe.430717
9. Ji E, Shen Y, Nie M, Xing F, Liu Q. Spectra- and temperature-dependent dynamics of directly end-pumped holmium lasers. Appl Phys B (2017) 123:129. doi:10.1007/s00340-017-6707-x
10. Ji E, Liu Q, Shen Y, Nie M, Xing F. Generation of watt-level 2.06- $\mu$ m polarized light from diode wing-pumped Ho:YLF laser. IEEE Photon Technol Lett (2017) 29(19):1695–8. doi:10.1109/lpt.2017.2743745
11. Zhao B-R, Yao B-Q, Qian C-P, Liu G-Y, Chen Y, Wang R-X, et al. 231 W dual-end-pumped Ho:YAG MOPA system and its application to a mid-infrared ZGP OPO. Opt Lett (2018) 43(24):5989–92. doi:10.1364/ol.43.005989
12. Liu G, Mi S, Yang K, Wei D, Li J, Yao B, et al. 161 W middle infrared ZnGeP2 MOPA system pumped by 300 W-class Ho:YAG MOPA system. Opt Lett (2021) 46(1):82–5. doi:10.1364/ol.413755
13. Pomeranz LA, Ketteridge PA, Budni PA, Ezzo KM, Rines DM, Chicklis EP. Tm:YAlO3 laser pumped ZGP mid-IR source. In: J Zayhowski, editor. Advanced solid-state Photonics, Vol. 83. OSA Trends in Optics and Photonics Optica Publishing Group (2003). paper 142.
14. Eichhorn M, Hirth A. Electro-optically Q-switched Tm:YAG laser pumped ZGP optical-parametric oscillator. In: Conference on lasers and electro-optics/quantum electronics and laser science conference and photonic applications systems technologies, OSA technical digest (CD). Optical Society of America (2008). paper CTuII3.
15. Creeden D, Ketteridge PA, Budni PA, Setzler SD, Young YE, McCarthy JC, et al. Mid-infrared ZnGeP2 parametric oscillator directly pumped by a pulsed 2 μm Tm-doped fiber laser. Opt Lett (2008) 33(4):315–7. doi:10.1364/ol.33.000315
16. Gebhardt M, Gaida C, Kadwani P, Sincore A, Gehlich N, Jeon C, et al. High peak-power mid-infrared ZnGeP_2 optical parametric oscillator pumped by a Tm:fiber master oscillator power amplifier system. Opt Lett (2014) 39(5):1212–5. doi:10.1364/ol.39.001212
17. Cole B, Goldberg L, Chinn S, Pomeranz LA, Zawilski KT, Schunemann PG, et al. Compact and efficient mid-IR OPO source pumped by a passively Q-switched Tm:YAP laser. Opt Lett (2018) 43(5):1099–102. doi:10.1364/ol.43.001099
18. Wei X, Zhang S, Liu Z, Ye X, Yin W, Ren H, et al. High-power mid-infrared ZGP optical parametric oscillator directly pumped by pulsed Tm:YLF laser at 1908 nm. Opt Laser Tech (2023) 161:109135. doi:10.1016/j.optlastec.2023.109135
19. Shen Y, Gong M, Fu X. Beam quality improvement by population-dynamic-coupled combined guiding effect in end-pumped Nd:YVO4 laser oscillator. Appl Phys B (2018) 124:85. doi:10.1007/s00340-018-6954-5
Keywords: middle infrared lasers, Tm:YAP, EO Q-switched, degenerate OPO, ZGP
Citation: Dai W, Ding Y, Liu T, Zhao Y, Gao J and Zhao C (2024) Efficient degenerate middle infrared ZGP-OPO pumped by an electro-optically Q-switched Tm:YAP laser. Front. Phys. 12:1390115. doi: 10.3389/fphy.2024.1390115
Received: 22 February 2024; Accepted: 01 April 2024;
Published: 19 April 2024.
Edited by:
Xiaoming Duan, Harbin Institute of Technology, ChinaReviewed by:
Jintao Fan, Tianjin University, ChinaLei Zuotao, Harbin Institute of Technology, China
Yijie Shen, Nanyang Technological University, Singapore
Copyright © 2024 Dai, Ding, Liu, Zhao, Gao and Zhao. This is an open-access article distributed under the terms of the Creative Commons Attribution License (CC BY). The use, distribution or reproduction in other forums is permitted, provided the original author(s) and the copyright owner(s) are credited and that the original publication in this journal is cited, in accordance with accepted academic practice. No use, distribution or reproduction is permitted which does not comply with these terms.
*Correspondence: Yu Ding, ding_yu123@163.com