- 1Kamioka Observatory, Institute for Cosmic Ray Research, University of Tokyo, Kamioka, Gifu, Japan
- 2Kavli Institute for the Physics and Mathematics of the Universe (WPI), The University of Tokyo Institutes for Advanced Study, University of Tokyo, Kashiwa, Chiba, Japan
The Hyper-Kamiokande (Hyper-K) project is the world’s leading international neutrino and nucleon decay experiment comprising a next-generation underground water Cherenkov detector and upgraded Japan Proton Accelerator Research Complex (J-PARC) neutrino beam. It will provide an enormous potential to discover the leptonic charge-parity violation, to investigate the Grand Unified Theory by proton decay exploration, and to determine the neutrino mass ordering. Further, Hyper-K will significantly enhance the capability to observe solar neutrinos and neutrinos from other astronomical sources in comparison with its predecessors. After the budget approval in January 2020, the Hyper-K project officially began, and the operation is expected to start in 2027. The excavations of a 2.0 km long access tunnel and the tunnels for the detector facility have been completed. In October 2022 it was started the excavation of one of the world’s largest underground caverns to suite the HK detector. This article discusses the status and the prospects of the Hyper-K detector construction.
1 Introduction
The Hyper-Kamiokande (Hyper-K) project Abe et al. [1] is the world-leading international scientific research project hosted by the university of Tokyo and High Energy Accelerator Research Organization (KEK) consisting of a next-generation underground water Cherenkov detector and upgraded Japan Proton Accelerator Research Complex (J-PARC) neutrino beam. The Hyper-K international collaboration consists of about 560 researchers from 101 institutes in 21 countries (at the time of writing this article). The detector technology has been developed based upon the successful Super-K experiment Fukuda et al. [2] and feasibility studies have been completed by international groups. The Hyper-K operation plans to start in 2027. The supplementary budget for FY2019, including the first-year construction budget for Hyper-K project, was approved by the Japanese Diet on January 2020 and the Hyper-K project was officially started.
The Hyper-K detector will be built in one of the world’s largest underground caverns, which is being excavated ∼600 m underground beneath the Kamioka Mine in Kamioka-cho, Hida City, Gifu Prefecture. Figure 1 shows an overview of the Hyper-K underground facility and a schematic view of the area around the detector. The overburden at the top of the main cavern is 592m and 650 m at the center of the detector. The detector, 71 m high and 68 m diameter, will be filled with 0.260 million metric tons of ultra pure water, corresponding to an order of magnitude larger fiducial mass (0.188 million metric tons) than Super-K. It will be equipped with newly developed high-sensitivity photosensors, and it will use a high-intensity neutrino beam produced by the upgraded J-PARC accelerator facility. It will provide an enormous potential to discover leptonic charge-parity (CP) violation by observing neutrino and anti-neutrino particles from J-PARC, to investigate the Grand Unified Theory by exploring proton decay, and to determine the neutrino mass ordering by observing atmospheric neutrinos and combining beam data. Hyper-K will also have far better capabilities to observe solar neutrinos and neutrinos from other astronomical sources than those of predecessor experiments based on water.
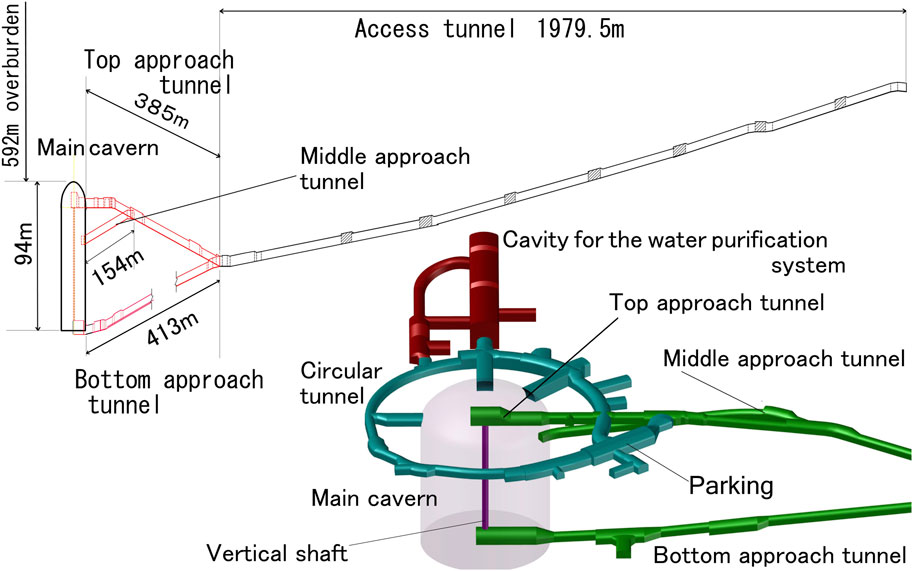
Figure 1. The Hyper-K underground facility overview (top) and the schematic view of the detector area (bottom).
Hyper-K will have capability to measure the magnitude of the CP violation with high precision, which could explain the baryon asymmetry in the Universe. By 10 years operation with predicted systematic error improvement, Hyper-K is expected to cover the 60% of the parameter space with a 5σ significance or better. More than 8σ significance will be expected if δCP = −π/2 as suggested by T2K Abe et al. [3]. The observation of the decay of the proton in the channel p → e+π0 is a strong signature favored by many Grand Unified Theory models, with a prediction close to the current limit of the proton decay life time. Hyper-K, thanks to the new high sensitive photomultiplier tubes (PMTs), will be able to strongly reduce the background induced by atmospheric neutrinos. This will help a lot the p → e+π0 search that is expected to be almost “background-free” (particularly in the free-proton enhanced signal region). Hyper-K is the only proposed experiment with the potential to go beyond and explore proton lifetimes larger than of 1 × 1035 years. Supernova burst neutrinos, once observed by Kamiokande, and undiscovered supernova relic neutrinos are also interesting targets to explore the history of elements and the Universe.
2 Construction overview
The Hyper-K underground facility consists of the main cavern, in which Hyper-K detector will be built, a cavity for the water purification system, tunnels, and a few small sub-cavities. The tunnel between the tunnel portal and the Hyper-K detector area is called the “access tunnel” which is approximately 2.0 km long with ∼6% downward slope. The access tunnel is split into three branches, called the “approach tunnels”, to allow to access the main cavern at diverse levels of the barrel section: the top, the middle, and the bottom section. Another “circular tunnel” surrounds the main cavern at the top level of the barrel section, and it is connected to additional cavities (e.g., the cavity for the water purification system) and to other smaller sub-cavities for auxiliaries systems.
All components of the Hyper-K underground facility are newly excavated. The first access tunnel excavation work started in 2021, while the excavations of the approach tunnels and sub-cavities were conducted subsequently. At the time of writing this article, all tunnels, sub-cavities and the cavity for the water purification system have been completed, while the excavation of the main cavern is still in progress. The following sections describe the details of the excavation work and their current status.
3 The tunnel excavation
3.1 Construction yard
The tunnel excavation started on 6 May 2021, immediately after the preparation of a construction yard in front of the portal of the access tunnel. The large excavation project required many specialized facilities, such as temporary storage for the excavated rock, a concrete plant, and a wastewater treatment facility for the water employed for the excavation. The construction yard and all the above facilities were prepared and installed in a ∼10,000 m2 area in approximately 6 months, including a breaktime due to snowfall.
For improve excavation safety, the yard preparation included the switchover of the prefectural road in front of the portal to separate construction traffic from civilian traffic. A new, temporary prefectural road was built so that the construction road could pass under that road without any interference.
3.2 Access tunnel
The access tunnel was first excavated; it is approximately 2.0 km long and connects the tunnel portal to the Hyper-K underground facility area. The end of the tunnel is approximately 100 m lower than the portal. The tunnel cross-section is 5 m wide and 5 m high, these dimensions were determined by considering sizes of required construction vehicles and facilities such as ventilation pipes. Widening sections are prepared every 200 m and employed for passing-each-other of vehicles.
The excavation was completed in only 9 months using long-hole blasting, which can cover a length of 4 m in a single blast. Initially, the schedule was delayed due to poor bedrock conditions, but as the bedrock conditions improved, the excavation speed was greatly improved. The speed reached a maximum of 15 m/day in the later part of the excavation, and the 1979.5 m excavation was completed as scheduled by February 2022.
3.3 Approach tunnel
The excavation of the approach tunnels, which connect the access tunnel to the main cavern, was the next step in the construction process. The approach tunnel splits into two tunnels at first; the “top approach tunnel” and “bottom approach tunnel”, which are connected to the top and the bottom, respectively, of the main cavern, each with a 10% slope. Thereafter, the “middle approach tunnel” branches from the top approach tunnel and connects to the middle level of the main cavern. The lengths of the top, bottom, and middle approach tunnels are 385 m, 413 m, and 154 m, respectively. From the first branch point of the approach tunnel, the excavation was implemented by two teams to expedite the process. The excavation started in March 2022 and ended in August 2022.
3.4 Circular tunnel and the sub-cavities
The circular tunnel surrounds the main cavern with a lap of 445 m. The width and height of the tunnel differ by area. The area with the highest width is the southeast area for parking as shown in the bottom of Figure 1. The length of that area is approximately 40 m, with a cross-section that is 12.5 m wide and 7.3 m high. The numbers were determined based on actual use in the Super-K experiment and expected number of vehicles in the Hyper-K experiment. In addition to its use for housing the experimental facilities, the circular tunnel was used to verify the condition of the bedrock around the main cavern in detail. During the excavation, collection of Measurements While Drilling data (logging the information related to drilling blast holes) and detailed observations of the tunnel surface were carried out.
The excavation of the circular tunnel started by branching from the top approach tunnel. In approximately 4 months, the excavation of the circular tunnel, including the sub-cavities arranged along with the circular tunnel, was completed as scheduled.
3.5 Cavity for the water purification system
Among the cavities attached to the circular tunnel, the largest is the cavity for the water purification system. The cavity is 101 m long, 17 m high, and 16 m wide. The excavation started from the work tunnel to the top of this large cavity. The main body was excavated in parallel with the dome excavation (see Figure 2), and completed May 2023.
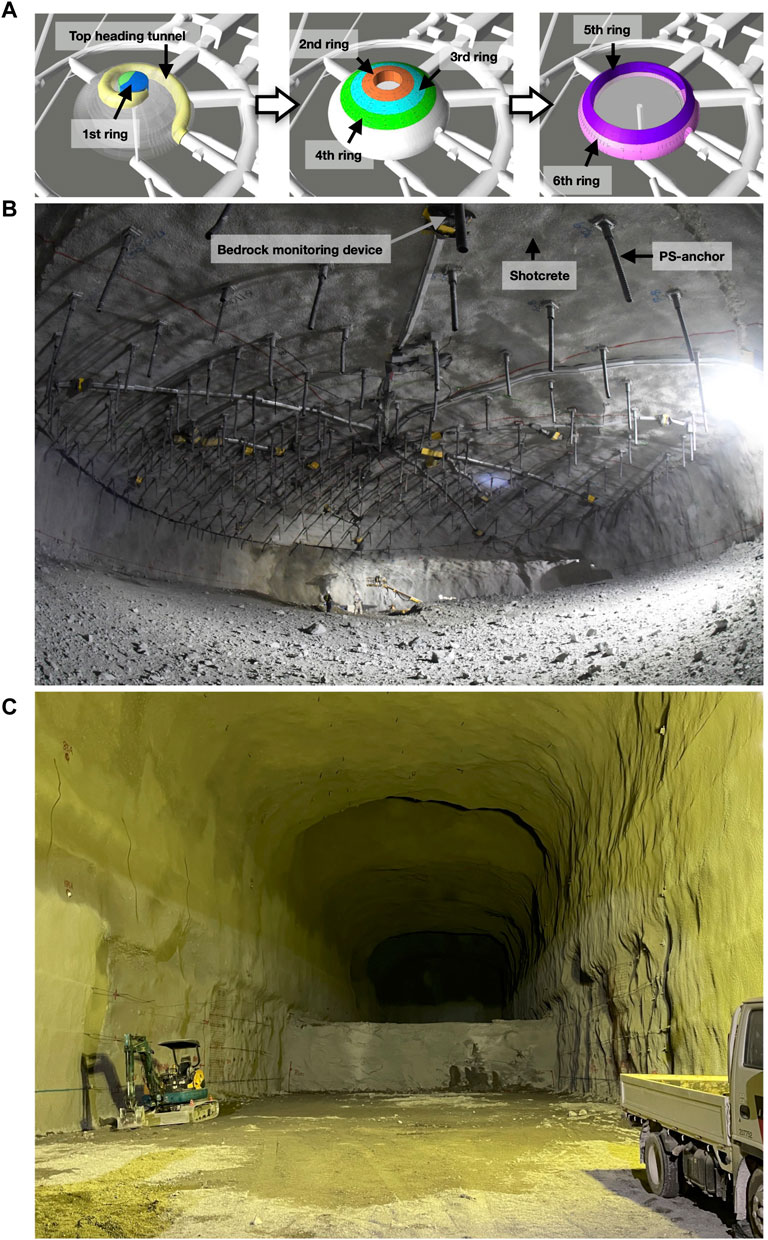
Figure 2. (A) Schematic of the excavation step of the dome section, (B) picture of the dome section as of the third ring excavation completed, early April 2023, (C) picture of the cavity for the water purification system as of early May 2023.
4 Cavern excavation
The Hyper-K main cavern consists of a rooftop portion, which is called a “dome section,” and a cylindrical “barrel section” under the dome section. The main cavern of Hyper-K is approximately 94 m high (the dome section is 21 m high, and the barrel section is 73 m high) with a diameter of 69 m1. The total excavation volume of the main cavern is approximately 330,000 m3.
Various geological investigation such as bore-hole drilling, laboratory rock test using drilled rock cores, seismic exploration in the adit, is-situ tests, measurement of initial state of underground stress, were carried out prior to the excavation of the Hyper-K main cavern Abe et al. [1]. The bedrock surrounding the main cavern is mainly composed of Hida gneisses (fine-grained paragneisses accompanied by coarse-grained migmatitic granitoids so-called Inishi migmatite). From the in-situ measurements and rock tests, a typical value of Young’s modulus and the Geological Strength Index Hoek [4] of the bedrock were evaluated to be 40 GPa and 73, respectively. Based on the results of the geological survey, we conducted cavern-stability analyses, including a three-dimensional elasto-plastic finite difference model analysis considering multi excavation steps, and designed the rock support, e,g., selection of rock-supporting materials, layout of the rock supports, and their specifications. The selected rock-supporting materials are shotcrete (thickness: ∼30 cm), rock bolts (length: 4–7 m), and pre-stressed anchors so-called “PS-anchors” (length: 8–22 m with applying pre-stress of 300–600 kN). The area density, length, and applied pre-stress (for the PS-anchors) of the rock support vary with the expected depth of the plastic zone and the geological conditions.
Since the Hyper-K main cavern is an underground cavern excavation of unprecedented scale worldwide, an “information-oriented design and excavation” method was employed. This method optimizes the rock support design and the excavation plan based on the latest geological information obtained as the excavation progresses and the data from the bedrock monitoring devices, e.g., bedrock displacement gauges (multiple stage extensometers) and PS-anchors load cells.
The main cavern excavation has started in October 2022. The main cavern is excavated step by step from the dome section through the bottom of the barrel section. For the dome section, a first pilot tunnel (called the “top heading tunnel”) was excavated to approach the dome zenith part. The dome section is being excavated with six excavation steps, called “rings”. The first ring is the dome zenith part with a diameter of 16 m, and the second to the sixth rings are excavated outward in an annular shape, as shown in Figure 2A. Notably, the diameter of the third ring is 40 m (see Figure 2B) which is the same as the diameter of the Super-K detector tank. At the time of writing this article, the excavation work for the fourth ring is underway. After the completion of the dome section, expected by end of 2023, the excavation of the barrel section will commence. The 73 m high barrel section is divided into 19 excavation steps in the vertical direction called “benches”; each one of these steps is 3–4 m deep. A vertical shaft with a diameter of 3.4 m was excavated with “raise boring” method through the entire barrel section from the top to the bottom and connected to the bottom approach tunnel as shown in Figure 1. The vertical shaft is used to transport the excavated rock of the barrel section to the bottom of the main cavern to be finally transported to the above ground via the bottom approach tunnel. This allows us to expedite the excavation by avoiding interference between the excavation work and the transportation of the excavated rocks. Furthermore, the excavation time for the barrel section is roughly identical to that for the dome section, despite that the excavation volume of the barrel section is 5.6 times higher than the dome section.
5 Summary and prospect
The excavation work for the Hyper-K underground facility, the first step in constructing the Hyper-K detector, is in progress. The tunnel, sub-cavity, and the cavity for the water purification system excavations have been completed, and the excavations of the Hyper-K main cavern is underway. The Hyper-K main cavern excavation is expected to be completed in 2024. The Hyper-K detector lining construction, PMT installation, and ultrapure water filling will be implemented subsequently. The Hyper-K collaboration aims to start the operation of the Hyper-K detector in the Japanese fiscal year of 2027.
Data availability statement
The original contributions presented in the study are included in the article/Supplementary Material, further inquiries can be directed to the corresponding author.
Author contributions
KA: Writing–original draft, H-KT: Writing–original draft.
Funding
The author(s) declare that no financial support was received for the research, authorship, and/or publication of this article.
Conflict of interest
The authors declare that the research was conducted in the absence of any commercial or financial relationships that could be construed as a potential conflict of interest.
Publisher’s note
All claims expressed in this article are solely those of the authors and do not necessarily represent those of their affiliated organizations, or those of the publisher, the editors and the reviewers. Any product that may be evaluated in this article, or claim that may be made by its manufacturer, is not guaranteed or endorsed by the publisher.
Footnotes
1A 50 cm thick lining concrete is placed after the cavern excavation.
References
1. Abe K, Aihara H, Aimi A, Akutsu R, Andreopoulos C, et al. Hyper-kamiokande design report (2018). arXiv , 1805.04163 Available at: https://arxiv.org/abs/1805.04163 (Accessed January 29, 2024).
2. Fukuda S, Hayakawa T, Ichihara E, Ishitsuka M, Itow Y, Kajita T, et al. The super-kamiokande detector. Nucl Instr Methods Phys (2003) A501:418–62. doi:10.1016/S0168-9002(03)00425-X
3. Abe K, Akutsu R, Ali A, Alt C, Andreopoulos C, Anthony L, et al. Constraint on the matter-antimatter symmetry-violating phase in neutrino oscillations. Nature (2020) 580:339–44. doi:10.1038/s41586-020-2177-0
Keywords: Hyper Kamiokande, super kamiokande, water cherenkov detector, underground, construction, excavation
Citation: Abe K and Tanaka H-K (2024) Hyper-Kamiokande construction status and prospects. Front. Phys. 12:1378254. doi: 10.3389/fphy.2024.1378254
Received: 29 January 2024; Accepted: 26 February 2024;
Published: 26 March 2024.
Edited by:
Aldo Ianni, Gran Sasso National Laboratory (INFN), ItalyReviewed by:
Paolo Lombardi, National Institute of Nuclear Physics of Milano, ItalyCopyright © 2024 Abe and Tanaka. This is an open-access article distributed under the terms of the Creative Commons Attribution License (CC BY). The use, distribution or reproduction in other forums is permitted, provided the original author(s) and the copyright owner(s) are credited and that the original publication in this journal is cited, in accordance with accepted academic practice. No use, distribution or reproduction is permitted which does not comply with these terms.
*Correspondence: Ko Abe, abe@km.icrr.u-tokyo.ac.jp; Hide-Kazu Tanaka, thide@km.icrr.u-tokyo.ac.jp