- 1National and Local Joint Engineering Research Center of Space Optoelectronics Technology, Changchun University of Science and Technology, Changchun, China
- 2College of Optoelectronic Engineering, Changchun University of Science and Technology, Changchun, China
To improve the bit error rate (BER) of underwater wireless laser communication and increase communication speed, a duplex underwater wireless laser communication system is designed. The system uses both blue and green lasers to transmit signals simultaneously, and adopts an improved modulation and demodulation algorithm to achieve separate modulation of the two sets of signals. A duplex underwater wireless laser communication system builds based on 440 and 550 nm lasers. On the basis of analyzing the absorption and scattering characteristics of seawater in laser communication, the influence of turbulence on communication efficiency is discussed. Improved the traditional duplex modulation algorithm and conducted communication testing at a depth of 2.0 m underwater. The experimental results show that when the communication distance increases from 5 to 50 m, the BER of the unoptimized duplex mode increases from 2.34 × 10−7 to 3.5 × 10−5. After adopting the optimization algorithm, the BER increases from 2.81 × 10−7 to 1.18 × 10−6, and the signal attenuation has been significantly suppressed. The duplex communication structure and algorithm can effectively reduce the impact of communication distance on bit BER.
1 Introduction
The ocean contains abundant biological and mineral resources, and about 70% of the Earth’s surface is covered by the ocean. The development and utilization of marine resources is a trend. The application of information and communication technology to ocean development and utilization is inevitable. Studying underwater communication for the ocean is one of the important directions for future development. Underwater wireless laser communication technology is a communication technology with important application value. Underwater wireless optical communication (UWOC) is an emerging communication technology [1]. It usually uses the blue green light band (450–570 nm) as an information carrier to achieve information transmission underwater [2]. Due to the characteristics of large bandwidth, high speed, and high security, UWOC has become a substitute communication technology for traditional underwater acoustic communication [3].
In 1960, Gilbert et al. [4] experimentally tested the low attenuation of blue-green light in water. In 1963, Duntley [5] tested the attenuation characteristics of light in various water bodies, verifying that the 450–550 nm blue-green band was a weak attenuation band in seawater, providing important design ideas for UWOC. In 1976, Karp et al. [6] used lasers to achieve bidirectional communication between satellites and underwater submarines, and designed corresponding communication models [7]. In 1991, Puschell J. J. [8] used duplex communication between aircraft and underwater submarines, and the laser used in the experiment was a blue green laser. In 1992, Snow et al. [9] completed underwater wireless laser communication of 5 m at a communication rate of 50 Mbps. In 2008, Hanson et al. [10] used the Monte Carlo method to analyze the optical communication characteristics of different water bodies. It achieves 1 Gbps/m underwater communication, and its 532 nm laser operates in an externally modulated mode. In 2012, John F. Muth et al. [11] designed an underwater wireless laser communication system with dual end transceiver. In 2017, Ho C. M. [12] achieved 10 Gbps transmission rate over a length of 10 m using a 405 nm blue laser and a 16 Quadrature-Amplitude-Modulation Orthogonal-Frequency-Division- Multiplexing modulation module. In 2019, Hong et al. [13] implemented 18.09 Gbps/m underwater optical communications using Discrete Multi Tone (DMT) modulation and shaping algorithms. In 2019, Wang J. [17] achieved indoor underwater optical communication of 100 m/500 Mbps with a transmission power of only 7.25 mW. Chi Nan et al. [14] utilized hardware equalization to achieve underwater transmission at a rate of 3.075 Gbps. Zhou Y [15] achieved 15.7 Gbps underwater visible light communication using GaN based LEDs and 64 Quadrature Amplitude Modulation technology. In 2019, Wang et al. [16] achieved underwater optical communication of 100 m/500 Mbps using a 520 nm laser and Non-Return-Zero On-Off-Keyed modulation in tap water.
In summary, underwater communication systems are developing towards multi wavelength, long-distance, and high-speed directions. In order to further improve the efficiency and stability of underwater wireless laser communication systems, we design a duplex laser communication system. By placing narrowband filters in front of the receiving window at different wavelengths, the signal-to-noise ratio of the sensing module is improved, thereby suppressing mutual interference.
2 System design
The Duplex underwater wireless laser communication system is shown in Figure 1. The system includes two parts: reception and transmission. The Transmission module includes Personal Computer (PC), modulation system, communication data database, modulation power supply, blue laser (at 440 nm), green laser (at 550 nm), and lens group. The Receiving module includes buncher, Administrative Distance (AD), Avalanche Photo Diode (APD), demodulator, PC, and display unit. The system modulates communication signals on both green and blue lasers simultaneously, and the receiving end obtains a duplex communication signal.
Multiple forms of encoding and modulation can be performed on the original source electrical signals. The system performs pre equalization processing on underwater fading channels. It drives the light source to emit a modulation signal. The photodetector in the receiving end receives the corresponding optical signal. After signal amplification, filtering, and other processing, the system can complete the collection and analysis of dual wavelength signals. Due to the fact that in real underwater application scenarios, the receiving and transmitting ends may be dynamic and cannot always maintain a good optimal state, which seriously affects the communication performance of the system. Therefore, the receiving end can design acquisition, pointing, and tracking modules, namely, APT modules, with the aim of maintaining the optical link alignment between the receiving and transmitting ends as much as possible. Corresponding optical components such as lenses and filters, it can be configured at both ends of the light source and detector to further optimize and improve the transmission performance of the beam.
3 Seawater characteristics analysis
3.1 Absorption and scattering
The underwater communication environment is complex, but it can be mainly divided into two categories: dissolved organic matter and suspended particles. The optical properties of these components are mainly manifested as absorption and scattering properties, corresponding to absorption coefficient and scattering coefficient. Therefore, attention coefficient can be expressed as:
Among them, α(λ) represents the absorption coefficient, and β(λ) represents the scattering coefficient, both in units of m−1. The two parameters exhibit different absorption and scattering effects on different water bodies. When light waves propagate in water, absorption refers to the conversion of photon energy into thermal or biological energy of substances in the water and their disappearance. Scattering refers to the phenomenon where photons interact with substances in seawater, causing the direction of photon transmission to randomly change without loss of energy. Therefore, scattering changes the energy distribution of the light field.
Seawater refers to water without any impurities, mainly composed of a mixture of dissolved salt and pure water. Therefore, the absorption effect mainly comes from water molecules and dissolved salts, including NaCl, MgCl2, CaCl2, etc. The absorption characteristics of pure seawater and pure water are very similar, with the lowest coefficient of absorption approximately in the 400–500 nm wavelength range, namely, the blue-green light band, while the absorption coefficients of other wavelengths are relatively high. This characteristic basically determines that blue-green light is the main wavelength band for underwater wireless optical communication.
Assuming the length of water passing through is l, the incident light power is Pi, the absorbed light power is Pα, and the scattering angle θ corresponds to the scattered light power Pθ. The absorption coefficient and scattering coefficient are:
When the water environment for communication is known, the testing of absorption coefficient and scattering coefficient can be completed through calibration methods.
The scattering phenomenon is the result of the water molecules combined action and suspended particles in seawater on light, and is also related to factors such as temperature, pressure, and salinity. To the complex size and distribution of different particles, it is difficult to find a unified scattering model. If the scattering effect generated by particle size is analyzed, it can be divided into Rayleigh scattering and Mie scattering. Rayleigh scattering is a common scattering phenomenon, and it refers to the scattering of incident light by particles with a diameter much smaller than the wavelength of light (less than one tenth of the wavelength), and the intensity of the scattered light is inversely proportional to the fourth power of the wavelength of light, that is, the shorter the wavelength, the stronger the scattering effect. The scattering of seawater mainly comes from Mie-Scattering, and it refers to the scattering phenomenon that occurs when the diameter of particles is equivalent to the radiation wavelength. The scattering intensity of Mie scattering is proportional to the square of frequency, and Forward scatter is stronger than backward scattering, with obvious directionality, while Rayleigh scattering is almost symmetrically distributed. Mie-Scattering can be simply understood as a random collision between photons and suspended particles that change the direction of transmission, and typically involves multiple collisions.
3.2 Turbulent interference
There are similarities between water and the atmospheric environment. Ocean turbulence in water is the chaotic movement of water. Turbulence refers to the flow where the velocity and direction of motion at any point in a water body change in an disordered manner, and there is a strong mixing phenomenon between different layers of fluid. The random changes in parameters such as temperature, salinity, and pressure of water result in significant fluctuations in the refractive index of the water body. The phase and intensity of underwater transmitted optical signals will fluctuate randomly and cause beam wanders, resulting in scintillation at the receiving end. Its phenomenon will reduce the transmission performance of laser communication systems. The turbulence intensity can be expressed as
Among them, L represents the characteristic length of water flow, v represents the velocity of water flow, and k represents the kinematic/eddy viscosity coefficient of water flow, expressed in m2/s. When Re > 2700, the water exhibits a significant turbulence phenomenon. The fading intensity caused by turbulence can be expressed as the scintillation index, with
Among them, I (l, L, λ) is the instantaneous intensity at the position vector (l, L), l is the previous position coordinate (x, y), L is the link distance, and E (I) represents the expected value.
In fluid mechanics, a dimensionless parameter characterizing the relative importance of momentum exchange and heat exchange in fluid flow. It indicates the relationship between the temperature boundary layer and the flow boundary layer, reflecting the influence of fluid physical properties on the convective heat transfer process, which can be defined as:
Among them, k represents the kinematic viscosity and χ represents the diffusion rate, expressed in m2s−1. The range of Prandtl numbers depends on the possibility of turbulence in the given medium. Each wavenumber can appear within the inertial or viscous range of its energy spectrum, or within the convective or diffusive range of its scalar spectrum.
The underwater environment also varies with changes in vertical depth, resulting in changes in optical properties. When the final system is applied to communication in seawater, the top layer is called the Euphoto zone, which is about 200 m deep in ocean water and can generate a large amount of solar radiation, such as phytoplankton. Therefore, the absorption coefficient of light in this area is relatively high. The middle layer is called the weak light zone, where there is insufficient sunlight, resulting in fewer algae and plants. The bottom layer is called the opaque zone, where sunlight is extremely scarce. The salinity, temperature, and other parameters of each layer vary, changes in the refractive index of the medium. Therefore, separate link modeling is required. This layering is meaningful for the weight matching of absorption and scattering parameters in the modeling process of underwater wireless laser communication.
3.3 Improved duplex modulation algorithm
Based on the impact of underwater environment on laser communication, the traditional laser modulation function is optimized and improved. In single wavelength laser communication, the echo waveform can be represented as
Among them, p(t) is the initial time-domain waveform, and Pc(t) is the impact response.
When using duplex communication, the hardware part uses bandpass filters to filter the corresponding wavelengths. At the same time, the processing algorithm performs differential calculations on the two sets of echo data. When the time difference between the emission laser of two wavelengths is Δ T, the phase difference is ΔÅ. During duplex modulation, the correction parameters are
By calculating the correction parameters, the amplitude ratio of two echo intensities can be solved. Meanwhile, the time difference between the two times is determined by the pre-set phase difference. This can achieve feedback control of the modulation state and suppress mutual crosstalk by correcting parameters.
4 Experiments
4.1 Experimental system
The system consists of a transmitter and a receiver. The emitting laser adopts dual lasers with center wavelengths of 440 and 550 nm, respectively. Each laser has a luminous power of approximately 200 mW. The detector adopts the APD detector of S8664-1010. Choose FPGA as the data acquisition module to achieve high-speed data acquisition. The modulator is used to modulate the signal of a duplex laser. The system is shown in Figure 2.
During laser communication, servo control used to achieve alignment between two modules. Due to the use of duplex communication, it is necessary to first determine which laser to communicate with during the alignment process, and then complete the alignment of laser communication. The reception process adopts time-sharing communication. There is a filter in front of the corresponding receiving window that is used to suppress other stray light.
4.2 Duplex signal sampling
Blue laser and green laser are used to emit laser signals respectively, and then APD is used to obtain the two optical signals. To distinguish the response results of laser signals, single laser pulses of 1 and 3 μs were used, respectively. The test results are shown in Figure 3.
The amplitude of the signal after filtering and amplification is about 400 mV, and the operating frequency is 25 MHz. When using duplex communication, it is first necessary to filter and denoise the two laser signals. The original signal contains a lot of noise, and using bandpass filtering can filter out high-frequency noise and low-frequency noise separately. The laser signals of green and blue light can be modulated using different pulse periods and phases, which facilitates data separation during later processing. As shown in Figure 3A, the input signal pulse width of the blue light signal source is 1 us, and the signal pulse width of the receiving end is 3.3 µs When modulating the amplitude strength of the input signal, the carrier signal is used to generate the envelope of communication data. It can be seen that the ripple noise of the blue response curve has been significantly filtered out. The square wave shape is obvious and can well reflect the peak and phase relationship of the input signal. As shown in Figure 3B, the input signal pulse width of the green light signal source is 3.0 µs, and the signal pulse width of the receiving end is 5.4 µs. When modulating the amplitude strength of the input signal, the carrier signal is used to generate the envelope of communication data. The energy analysis of signals can calculate the degree of energy drift during the modulation process. Comparing the two sets of test signals, it can be seen that using the filtering processing of this system can obtain better data waveform curves.
4.3 BER analysis of duplex communication
At a depth of 2.0 m, a duplex laser communication system was tested using single pass and dual pass tests, respectively. Direct communication and signal modulation communication methods were used during dual pass operation. The test results of BER with different communication distances are shown in Figure 4.
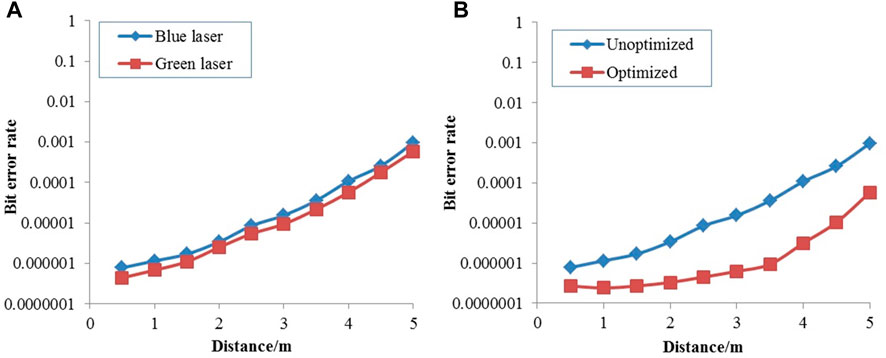
FIGURE 4. Response waveform of duplex signal. (A) Bit error rate of single laser (B) before and after optimization of dual laser.
As shown in Figure 4A, blue laser and green laser completed a 2.0 m deep underwater wireless laser communication test separately. When the communication distance increases from 5 to 50 m, the BER of blue laser increases from 1.54 × 10−7 to 9.57 × 10−4, and the BER of green laser increases from 1.03 × 10−7 to 5.88 × 10−5. The communication efficiency of the two monochromatic lasers is similar, and when the communication distance is the same, the BER does not differ significantly. As shown in Figure 4B, using duplex mode, compare whether to choose the optimization algorithm in this article. When the communication distance increases from 5 to 50 m, the bit error rate of the unoptimized duplex mode increases from 1.63 × 10−7 to 9.24 × 10−5, which is basically similar to the communication BER of monochromatic laser. After using the algorithm optimization in this article, the bit error rate increased from 2.81 × 10−7 to 1.18 × 10−6. In contrast, after optimization, the growth rate of the system’s bit error rate significantly decreases, and the trend of bit error rate variation with respect to distance weakens. It can be seen that using the duplex modulation optimization design in this article has good results.
5 Conclusion
A duplex underwater wireless laser communication system is proposed. The system uses a 440 nm green laser and a 550 nm blue laser to communicate together. On the basis of analyzing the characteristics of seawater, a modulation and demodulation algorithm for suppressing seawater interference is proposed. The experiments were conducted on monochromatic laser communication and duplex communication, respectively. After optimization, the growth rate of the system’s bit error rate significantly decreases, and the trend of bit error rate variation with respect to distance weakens. It can be seen that using the duplex modulation optimization design in this article has good results. This design can slow down the increase in bit error rate and has better application prospects in the field of underwater wireless laser communication.
Data availability statement
The original contributions presented in the study are included in the article/Supplementary Material, further inquiries can be directed to the corresponding authors.
Author contributions
BD proposed this idea and wrote this paper. ST provided theoretical guidance and analysis. PZ completed the experiment. YD participated in experimental data analysis and paper revision. All authors contributed to the article and approved the submitted version.
Funding
This work was supported in part by Natural Science Foundation of Jilin Province (YDZJ202301ZYTS394) and Science and Technology Research Project of Jilin Provincial Department of Education (JJKH20230814KJ).
Conflict of interest
The authors declare that the research was conducted in the absence of any commercial or financial relationships that could be construed as a potential conflict of interest.
Publisher’s note
All claims expressed in this article are solely those of the authors and do not necessarily represent those of their affiliated organizations, or those of the publisher, the editors and the reviewers. Any product that may be evaluated in this article, or claim that may be made by its manufacturer, is not guaranteed or endorsed by the publisher.
References
1. Zhou H, Zhang M, Wang X, Ren X. Design and implementation of more than 50m real-time underwater wireless optical communication system. J Lightwave Tech (2022) 40(12):3654–68. doi:10.1109/jlt.2022.3153177
2. Al-Zhrani S, Bedaiwi NM, El-Ramli IF, Barasheed AZ, Abduldaiem A, Al-Hadeethi Y, et al. Underwater optical communications: A brief overview and recent developments. Engineered Sci (2021) 16:146–86. doi:10.30919/es8d574
3. Khan IU, Iqbal B, Songzou L. Full-duplex underwater optical communication systems: A review//2021 international bhurban conference on applied Sciences and technologies (ibcast). IEEE (2021) 886–93.
4. Gilbert A, Stoner TR, Jernigan JL. Underwater experiments on the polarization, coherence, and scattering properties of a pulsed blue-green laser. Proc Underwater Photo Opt (1966) I.
6. Karp S. Optical communications between underwater and above surface (satellite) terminals. IEEE Trans Commun (2003) 24(1):66–81. doi:10.1109/tcom.1976.1093200
7. Khalil RA, Babar MI, Saeed N, Jan T, Cho HS. Effect of link misalignment in the optical-internet of underwater things. Electronics-Switz (2020) 9(4):646. doi:10.3390/electronics9040646
8. Puschell JJ, Giannaris RJ, Stotts L. The autonomous data optical relay experiment: First two way laser communication between an aircraft and submarine. In: proceedings of the Telesystems Conference; 19-20 May 1992; Washington (1992).
9. Snow JB, Flatley JP, Freeman DE, Landry MA, Lindstrom CE, Longacre JR, et al. Underwater propagation of high-data-rate laser communications pulses. In: Proceedings of SPIE - The International Society for Optical Engineering; 31 December 1992; San Diego, CA (1992). p. 1750.
10. Hanson F, Radic S. High bandwidth underwater optical communication. Appl Opt (2008) 47(2):277. doi:10.1364/ao.47.000277
11. Simpson JA, Hughes BL, Muth JF. Smart transmitters and receivers for underwater free-space optical communication. IEEE J Selected Areas Commun (2012) 30(5):964–74. doi:10.1109/jsac.2012.120611
12. Ho CM, Lu CK, Lu HH, Huang S, Cheng M, Yang Z, et al. A 10m/10Gbps underwater wireless laser transmission system; 19-23 March 2017; Los Angeles (2017). proceedings Opt Fiber Commun Conf Exhibition
13. Hong X, Fei C, Zhang G, Du J, He S. Discrete multitone transmission for underwater optical wireless communication system using probabilistic constellation shaping to approach channel capacity limit. Opt Lett (2019) 44(3):558–61. doi:10.1364/ol.44.000558
14. Nan C, Yuanyuan W, Yiguang W, Xingxing H, Xiaoyuan L. Ultra-high-speed single red–green–blue light-emitting diode-based visible light communication system utilizing advanced modulation formats. Chin Opt Lett (2014) 12(001):22–5. doi:10.3788/COL201412.010605
15. Zhou Y, Zhu X, Hu F, Shi J, Wang F, Zou P, et al. Common-anode LED on a Si substrate for beyond 15 Gbit/s underwater visible light communication. Photon Res (2019) 7(9):1019. doi:10.1364/prj.7.001019
Keywords: underwater wireless laser communication, duplex mode communication, modulation algorithm, bit error rate, optimization model
Citation: Dong B, Tong S, Zhang P and Du Y (2023) Research on duplex underwater wireless laser communication system. Front. Phys. 11:1225869. doi: 10.3389/fphy.2023.1225869
Received: 20 May 2023; Accepted: 03 July 2023;
Published: 14 July 2023.
Edited by:
Zhenxu Bai, Hebei University of Technology, ChinaReviewed by:
Zhenzhen Song, Nanjing University of Information Science and Technology, ChinaXiangqian Quan, Chinese Academy of Sciences (CAS), China
Copyright © 2023 Dong, Tong, Zhang and Du. This is an open-access article distributed under the terms of the Creative Commons Attribution License (CC BY). The use, distribution or reproduction in other forums is permitted, provided the original author(s) and the copyright owner(s) are credited and that the original publication in this journal is cited, in accordance with accepted academic practice. No use, distribution or reproduction is permitted which does not comply with these terms.
*Correspondence: Shoufeng Tong, tsf1998@sina.com; Peng Zhang, zp@cust.edu.cn