- 1School of Electronic Information, Hunan First Normal University, Changsha, China
- 2School of Electrical and Data Engineering, Tech Lab, University of Technology Sydney, Sydney, NSW, Australia
- 3School of Information and Communication Engineering, Beijing University of Posts and Telecommunications, Beijing, China
The metasurface hybridized with vanadium dioxide (VO2) can be dynamically tuned, which has attracted enormous attention in recent years and orbital angular momentum (OAM) multiplexing based on metasurfaces has shown promising prospects in terahertz communications. However, existing research on VO2 metasurface focuses on the metallic metasurface. The dielectric VO2 metasurface used for OAM multiplexing is rarely reported to the present. This paper proposed a terahertz reconfigurable dielectric metasurface hybridized with VO2 for two-dimensional multichannel multiplexing combing with spatial and frequency domains. The metasurface works in both reflection and transmission modes and simultaneously the polarization control and operating frequency band regulation can be realized by switching the VO2 from the metallic state to the insulator state. For the reflective or transmissive metasurface, when 4×M-channel (M is a positive integer) off-axis plane waves are incident on the metasurface, the co-polarization reflected or cross-polarization transmitted waves are transformed into 4×M-channel orthogonal on-axis beams with topological or frequency orthogonality. A metasurface composed of 14 × 14 unit cells is designed for verification. The simulated result shows that two-dimensional 12-channel multiplexing combing with OAM and frequency by the designed metasurface can be realized on the reflection and transmission modes in two different frequency bands. The proposed metasurface has great potential in terahertz communications.
Introduction
The metasurface [1], as the two-dimensional metamaterial, can effectively manipulate the phase, amplitude, and polarization of electromagnetic (EM) waves and considerable effort has been devoted to investigating the metasurface. The metasurface hybridized with dynamically tunable materials, such as graphene [2], liquid crystals [3], and PIN diode [4], is called reconfigurable metasurface [5]. Compared with the fixed metasurface without active materials, EM properties of the reconfigurable metasurface can be dynamically tuned by controlling the active devices, which has attracted enormous attention in recent years. The phase-change material vanadium dioxide (VO2) offers excellent switching behavior from insulator state to metallic state around 68°C driven by temperature, electric fields, and laser pumping in the terahertz region [6, 7]. Therefore, it is an efficient method to incorporate VO2 into the metasurface to realize the terahertz reconfigurable metasurface. Besides, according to the primary category of materials constituting metasurface, metasurfaces can be divided into metallic and dielectric metasurfaces. The dielectric metasurface has advantages of low Ohmic loss, low cost, ultrahigh transmission efficiency, etc., compared with metallic metasurface [8]. But the existing research on VO2 reconfigurable metasurface has been focused on the metallic metasurface. Therefore, the reconfigurable dielectric metasurface hybridized with VO2 is worthy of being further studied.
In general, five physical domains, including time, polarization, frequency, quadrature, and space, can be used for beam multiplexing to improve the data rate and capacity of communication systems [9]. The vortex beam carrying orbital angular momentum (OAM) is characterized by the doughnut-shaped intensity profile and helical phase front. Besides, OAM beams with various topological charges are orthogonal with each other and are available for spatial multiplexing [10]. Therefore, generating the OAM beam [11–15], achieving OAM multiplexing [13, 16–23], and further realizing two-dimensional or multi-dimensional multiplexing combined with OAM and other four physical domains by metasurface [24–28], have shown promising prospects in high-speed and huge-capacity communication systems, especially in terahertz communications. However, existing VO2 reconfigurable metasurfaces are mainly concentrated on achieving active manipulation of the transmission coefficient [29], reflection coefficient [30], planar-chiral response [31], and Mie resonant [32], or realizing dynamic polarization converter [33, 34], metalens [35], absorber [36, 37], beam splitter [38, 39], OAM generator [40], and meta-holography [41]. The VO2 metasurface used for OAM multiplexing [42] is rarely reported to the present.
A terahertz reconfigurable dielectric metasurface embedded with VO2 for two-dimensional multichannel multiplexing combing with spatial and frequency domains is presented in this paper. The metasurface can simultaneously realize reflection-transmission mode switching and polarization regulation and the working frequency is controlled by easily tuning the state of VO2 without physically changing the structure. The unit cell of the metasurface comprises a top silicon elliptical pillar and a silicon-VO2 substrate. When the circularly polarized wave is perpendicular to the unit cell with VO2 in the metallic and insulator states respectively, the simulation result shows that both the 3 dB bandwidth of the co-polarization reflection coefficient and the cross-polarization transmission coefficient is about 0.1 THz. Operating frequency bands are about 0.18–0.28 THz and 0.25–0.35 THz respectively. The metasurface is designed based on the method of two-dimensional multichannel multiplexing combing with spatial and frequency domains [27] and the Pancharatnam Berry (PB) phase (geometric phase) principle [43, 44]. The simulated results show that the designed metasurface can work in both reflection and transmission modes with the working frequency range of 0.18–0.28 THz and 0.25–0.35 THz respectively. By switching the state of VO2, 12-channel orthogonal on-axis co-polarization reflected beams and cross-polarization transmitted beams with topological or frequency orthogonality are generated, when 12-channel off-axis plane waves are incident onto the dielectric metasurface. That is, two-dimensional multichannel multiplexing combing spatial and frequency domains can be achieved in both reflection and transmission mode by the proposed metasurface. The proposed metasurface has great potential in terahertz communications.
Operating principle
Figure 1 shows the schematic diagram for two-dimensional multichannel multiplexing combing spatial and frequency domains by the terahertz reconfigurable dielectric metasurface which can work in both reflection and transmission modes by tuning the VO2.
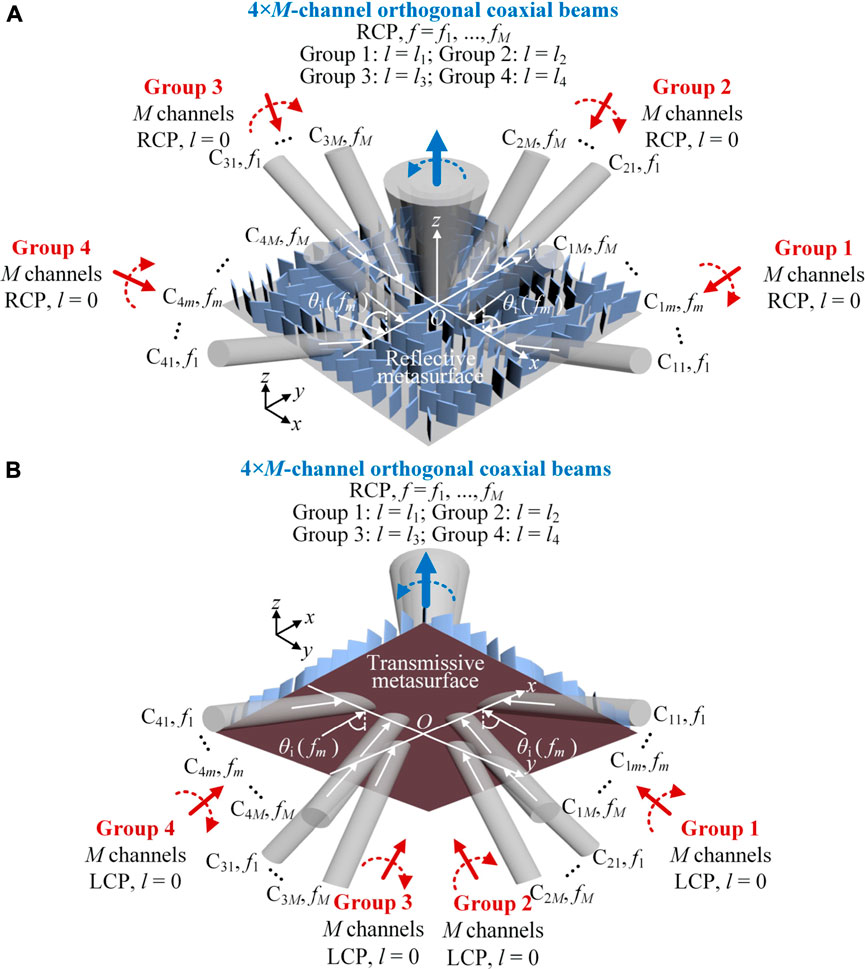
FIGURE 1. The schematic diagram for two-dimensional multichannel multiplexing combing with spatial and frequency domains is achieved by the reconfigurable dielectric metasurface which can work in both reflection and transmission modes (A) For reflective metasurface, 4×M-channel off-axis RCP plane waves with the frequency fm and angle of incidence θi (fm) (m = 1, … , M) are incident on the metasurface from the negative z-axis along negative x and y directions, and positive x and y directions respectively, RCP reflected waves are transformed into 4×M-channel orthogonal on-axis OAM beams with different topological charges (l = l1, l2, l3, and l4) or frequencies (f = f1 to fM) (B) Similarly, for the transmissive metasurface, 4×M-channel off-axis LCP plane waves are incident on the transmissive metasurface from the positive z-axis along with negative x and y directions, and positive x and y directions respectively, RCP transmitted waves are transformed into 4×M-channel orthogonal on-axis OAM beams with topological or frequency orthogonality.
As shown in Figure 1A, the designed metasurface works in the reflection mode. For the group 1 (channels C11 to C1M), M-channel right-handed circularly polarized (RCP) plane wave with frequency fm and angle of incidence θi (fm) (m = 1, … , M) obliquely illuminate the reflective metasurface from the negative z-axis along the -x direction, in the direction perpendicular to the metasurface, the co-polarization reflected waves (RCP reflected wave) are transformed into on-axis OAM beams with l1. Because frequencies of channels C11 to C1M are different, the generated M-channel on-axis OAM beams are orthogonal with each other. In the same way, for the group 2 (channels C21 to C2M), the group 3 (channels C31 to C3M), and the group 4 (channels C41 to C4M), the RCP plane wave with frequency fm and the angle of incidence θi (fm) obliquely illuminate on the reflective metasurface from the negative z-axis along negative y, positive x, and positive y directions respectively, in the direction perpendicular to the metasurface, RCP reflected waves are transformed into M-channel orthogonal on-axis OAM beams with l2, l3, and l4 respectively. Besides, the topological charges of these four groups of OAM beams are different from each other. Thus, it can be seen that 4×M-channel orthogonal on-axis beams with topological or frequency orthogonality are realized, and two-dimensional multichannel multiplexing combing with spatial and frequency domains by the reflective metasurface is achieved.
As shown in Figure 1B, the designed metasurface works in the transmission mode. Similarly, for the group 1 to group 4, 4×M-channel left-handed circularly polarized (LCP) plane waves with frequency fm and angle of incidence θi (fm) (m = 1, … , M) obliquely illuminate on the transmissive metasurface from the positive z-axis along negative x and y directions, as well as positive x and y directions respectively, in the direction perpendicular to the metasurface, the cross-polarization transmitted waves (RCP transmitted wave) are transformed into 4×M-channel orthogonal on-axis OAM beams with topological or frequency orthogonality. Thus, two-dimensional multichannel multiplexing combing with spatial and frequency domains by the transmissive metasurface can be realized.
First, to generate the OAM beam with topological charge l, the phase profile φl of the metasurface should satisfy Eq. 1:
where (x, y) represents the arbitrary coordinate position on the metasurface and l represents the topological charge. It is worth noting that the topological charge of the plane wave is 0.
Then, according to the generalized laws of reflection and refraction [45], set the phase-gradient metasurface as the interface between the media one and 2. When the EM wave is incident on the metasurface from media one along the negative z-axis, the incidence angle αi and reflected angle αr should satisfy Eq. 2, and when the EM wave is incident on the metasurface from media two along the positive z-axis, the incidence angle αi and refracted angle αt should satisfy Eq. 3:
where ni and nt are refractive indices of the media. (dφd/dx) and (dφd/dy) are constant gradients of phase discontinuity along the metasurface. If ni = 1, nt = 1, and αi = 0°, the phase profile φd of the phase-gradient metasurface can be expressed as Eq. 4:
where D = M · P is the super unit cell period along the phase gradient direction, P is the period of the unit cell, and M is the number of units constituting the super unit cell. αr and αt can be expressed as Eqs. 5,6:
where f is the incident wave frequency and C represents the EM wave velocity in the free space. αr and αt are related to f.
Therefore, according to Eqs. 1,4, when a plane wave with f is vertically incident on a reflective or transmissive metasurface, to generate four-channel off-axis beams deflected along ± x and ± y directions with αr or αt and different topological charges, the transfer function t of the metasurface should satisfy Eq. 7:
Next, according to Eq. 7, if four-channel off-axis plane beams are incident on the reflective metasurface from the negative z-direction or on the transmissive metasurface from the positive z-direction with f and the angle of incidence θi (f), the phase profile ϕ of the metasurface can be expressed as Eq. 8 [25, 44]. It is worth noting that for the reflective and transmissive metasurfaces, θi should satisfy Eqs. 5,6 respectively.
Therefore, if the designed metasurface satisfies Eq. 8, the two-dimensional multichannel multiplexing can be achieved by this metasurface working in reflection or transmission mode.
Then, according to the PB phase principle (geometric phase principle) [43], when the unit cell rotates φrot clockwise, for the reflective unit cell, there is a phase shift (PB phase) which is as twice the rotation angle 2φrot between the RCP reflective wave and RCP wave incident from above the unit cell. For the transmissive unit cell, there is a PB phase 2φrot between the RCP transmitted wave and LCP wave incident from the back of the unit cell. Therefore, the 0–2π phase shift can be covered by rotating the unit cell from 0 to π and the proposed metasurface can be designed by the geometric phase principle. The rotation angle profile of the metasurface can be expressed as follows:
Moreover, in the THz region, according to the Drude model, the permittivity ε of VO2 varying with angular frequency ω can be expressed as follows [37, 41]:
where ε∞ = 12 is the high frequency permittivity,
In summary, the required metasurface working in both reflection and transmission modes for two-dimensional multichannel multiplexing can be designed by Eq. 8, PB phase principle, and VO2.
Unit cell
Figure 2 depicts the unit cell of the proposed reconfigurable dielectric metasurface. As shown in Figures 2A,B, the unit cell comprises top silicon (ε = 11.9) elliptical pillar and Si-VO2 substrate. It is worth noting that to distinguish the Si pillar and the Si substrate, the different colors are used. The period of the unit cell is p = 550 μm, the height of the Si pillar is tp = 900 μm, the thickness of the Si-VO2 substrate is ts = 5 μm and tv = 0.2 μm, and the minor and major axes of the ellipse are a = 54 μm and b = 520 μm respectively. The angle between the major axis and positive x-axis is φrot. As shown in Figures 2C,D, the unit cell can work in reflection and transmission modes by switching the VO2 from the metallic state to the insulator state.
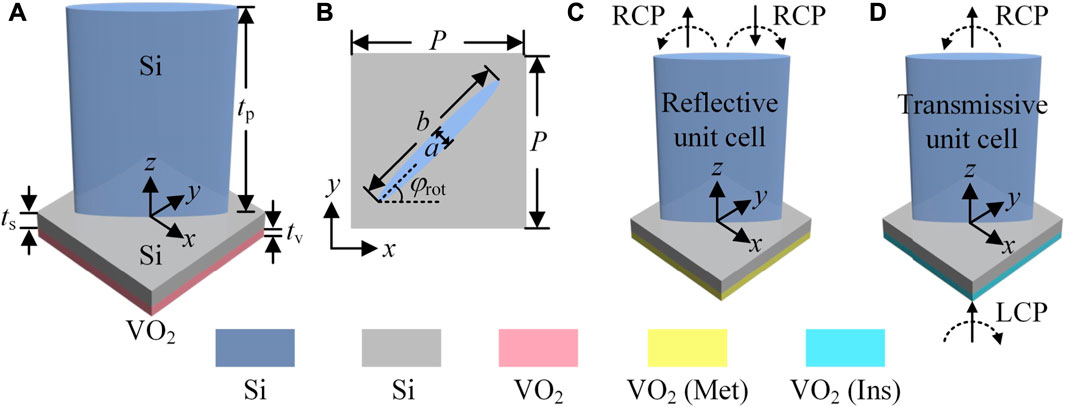
FIGURE 2. Schematic diagram of the unit cell composed of the top Si elliptical pillar and Si-VO2 substrate (A) Stereoscopic view (B) Vertical view (C) Reflective unit cell (D) Transmissive unit cell. p = 550 μm, tp = 900 μm, ts = 5 μm, tv = 0.2 μm, a = 54 μm, and b = 520 μm.
CST Microwave Studio is applied to investigate the unit cell numerically. Periodic boundaries and Floquet ports are employed along with the x-y and z directions. For the reflective and transmissive unit cell with VO2 in metallic and insulator state, RCP and LCP waves are normally incident onto the unit cell along -z and +z directions, respectively. VO2 is modeled by the Drude model by Eqs. 10,11. Besides, φrot changes from 0° to 180°. Figure 3 shows the simulated phase and amplitude of the co-polarization reflection coefficient RRR and the cross-polarization transmission coefficient TRL versus frequency with φrot = 0°, 30°, 65°, 105°, and 155°, respectively (Si substrate is hidden in the schematic diagram of the unit cell). The maximum amplitude of RRR and TRL are about 0.87 and 0.98. Therefore, the 3 dB bandwidth of the RRR and TRL are about 0.1 THz, and the operating frequency band is about 0.18–0.28 THz (The relative bandwidth is about 43.5%) and 0.25–0.35 THz (The relative bandwidth is about 36.7%) respectively. Besides, the phase shift is near-parallel and can cover 0–2π by rotating the unit cell from 0 to π in the operating frequency band. It is worth noting that based on the PB principle, the required phase shift can also be satisfied under the oblique incident wave.
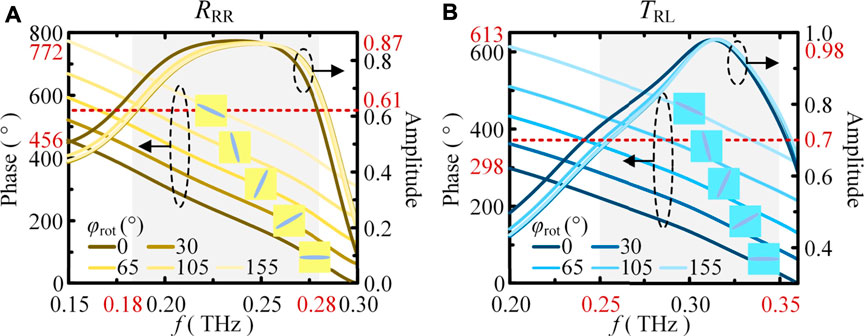
FIGURE 3. The simulated phase and amplitude versus frequency of the unit cell with φrot = 0°, 30°, 65°, 105°, and 155° respectively (A) The co-polarization reflection coefficient RRR with the RCP wave normally incident along the negative z-direction (B) The cross-polarization transmission coefficient TRL with the LCP wave normally incident along the positive z-direction.
Metasurface
A reconfigurable dielectric metasurface consist of 14 × 14 unit cells with M = 5, l1 = 0, l2 = +2, l3 = -2, and l4 = +4 is designed for verification. The phase profile ϕ (x, y) and rotation angle profile φrot (x, y) of the designed metasurface can be obtained by Equations 8, 9, respectively. The phase profile and structure diagram are shown in Figure 4.
When the metasurface works in the reflection mode with VO2 in the metallic state, take f1 = 0.18 THz, f2 = 0.23 THz, and f3 = 0.28 THz for example, the corresponding angle of incidences are θi (f1) ≈ 37.5°, θi (f2) ≈ 28.5°, and θi (f3) ≈ 23.1° respectively, calculated based on Eq. 5. While the metasurface works in the transmissive mode with VO2 in the insulator state, take f1 = 0.25 THz, f2 = 0.3 THz, and f3 = 0.35 THz with θi (f1) ≈ 26°, θi (f2) ≈ 21.4°, and θi (f3) ≈ 18.3° respectively for examples, obtained based on Eq. 6. According to Figure 1, the schematic diagram for two-dimensional 12-channel multiplexing combing with OAM and frequency realized by the designed metasurface (Si substrate is hidden) is shown in Figure 5.
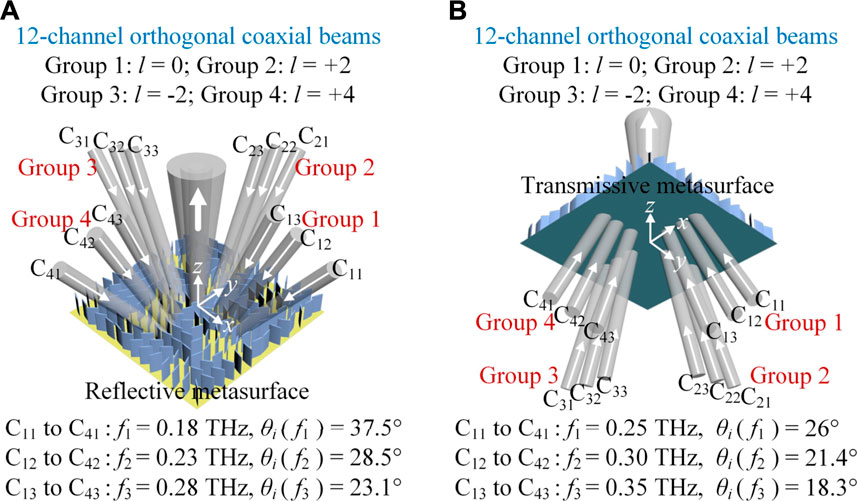
FIGURE 5. The schematic diagram for two-dimensional 12-channel multiplexing combing with OAM and frequency realized by the designed metasurface (A) Reflective metasurface (B) Transmissive metasurface.
When the designed metasurface works in the reflection mode, simulated far-field patterns of the RCP reflected wave are shown in Figure 6. As shown in Figure 6A, for the channel C11, the off-axis RCP plane wave is incident on the metasurface with f1 and θi (f1) along the negative x-axis, and an RCP reflected beam with a solid intensity profile and unchanged phase front is generated in the direction perpendicular to the metasurface. Therefore, the incident wave is transformed into a beam with f1 and l1 = 0. Similarly, for channel C12 or C13, the incident wave is transformed into a beam with f2 or f3 and l1 = 0. Therefore, the generated 3-channel on-axis beams of group 1 are orthogonal with each other. As shown in Figure 6B, For group 2, when the incident RCP plane wave with fm and θi (fm) (m = 1, 2, and 3) obliquely illuminates on the metasurface along the negative y-direction, an RCP reflected beam is generated in the direction perpendicular to the metasurface. Besides, the intensity profile is doughnut-shaped and the phase front changes 4π clockwise observing along the +z direction. Therefore, incident waves are transformed into 3-channel orthogonal on-axis beams with different frequencies (f1, f2, and f3) and l2 = +2 respectively. In the same way, for group 3 or group 4, when off-axis incident RCP plane waves with fm and θi (fm) illuminate on the metasurface along + x or + y directions respectively, in the direction perpendicular to the metasurface, RCP reflected waves are transformed into 3-channel orthogonal on-axis OAM beams with topological charge l3 = -2 or l4 = +4 (The intensity profile is doughnut-shaped and the phase front changes -4π or +8π clockwise along the +z direction). Besides, the topological charges of the four groups of beams are different from each other. Therefore, the 12-channel orthogonal on-axis beams with topological or frequency orthogonality can be achieved, and two-dimensional 12-channel multiplexing combing with spatial and frequency domains by the reflective metasurface can be realized. It is worth noting that there are strong sidelobes in the far field patterns resulting from the angle-multiplexed metasurface design principle [42], which will affect the overall efficiency of the metasurface. In addition, according to the far field pattern, the maximum intensity of the generated beam is available. Take group 1 for example, the maximum value is about -4.26, 2.8, and 3.41 dB respectively.
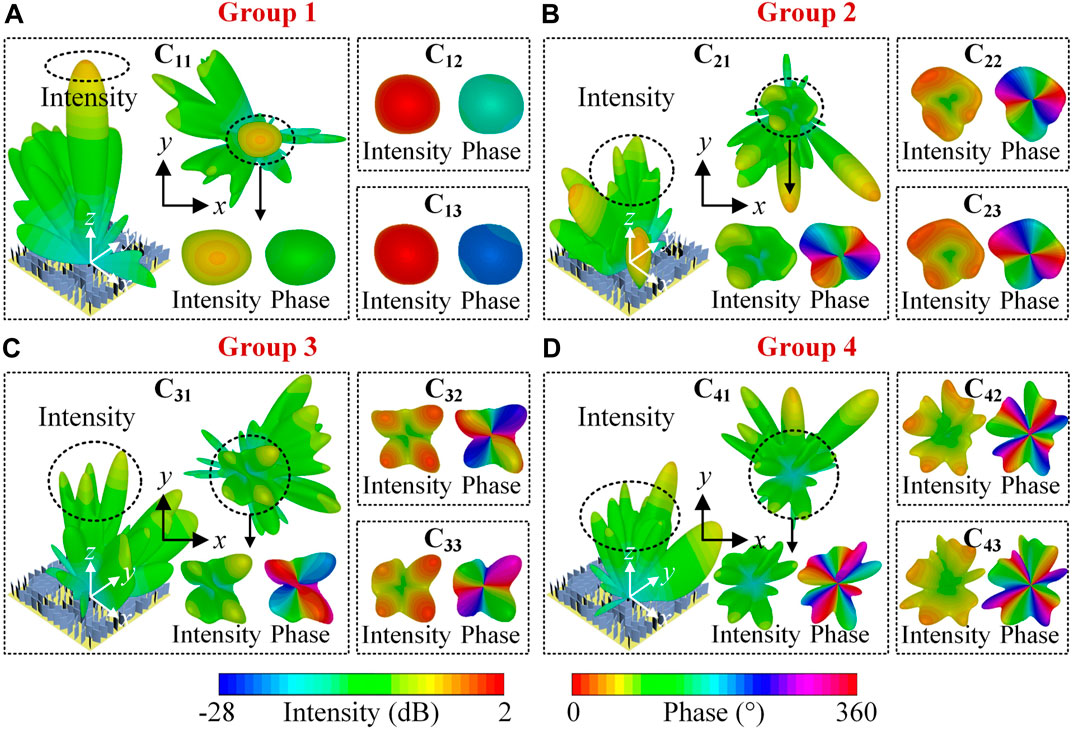
FIGURE 6. Simulated far field patterns of the RCP reflected wave. RCP reflected waves are transformed into 3-channel orthogonal on-axis beams with different frequencies (f1 = 0.18 THz, f2 = 0.23 THz, and f3 = 0.28 THz) and the same topological charge (A) l1 = 0 (B) l2 = +2 (C) l3 = -2 (D) l4 = +4. Therefore, 12-channel orthogonal on-axis beams with topological or frequency orthogonality can be achieved.
When the designed metasurface works in the transmission mode, simulated far-field patterns of the RCP transmitted wave are shown in Figure 7. For group 1 to group 4, when 12-channel LCP plane waves with fn and θi (fn) obliquely illuminate on the metasurface from the positive z-axis along negative x and y directions, and positive x and y directions respectively, RCP transmitted wave are transformed into 12-channel orthogonal on-axis beams with topological orthogonality (l1 = 0, l2 = +2, l3 = -2, and l4 = +4) or frequency orthogonality (f1 = 0.25 THz, f2 = 0.3 THz, and f3 = 0.35 THz). Therefore, two-dimensional 12-channel multiplexing combing with spatial and frequency domains by the transmissive metasurface can be realized. Similarly, take group 1 for example, the maximum intensity of the generated beam is about 2.56, 7.01, and 5.47 dB respectively.
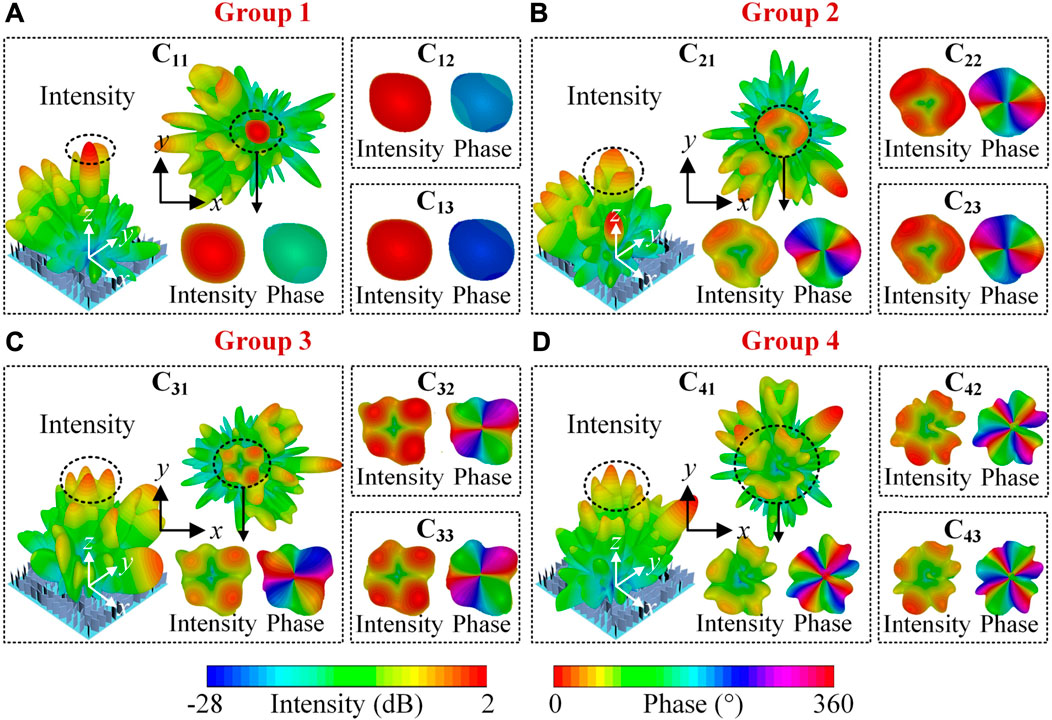
FIGURE 7. Simulated far field patterns of the RCP transmitted wave. RCP transmitted waves are transformed into 3-channel orthogonal on-axis beams with different frequencies (f1 = 0.25 THz, f2 = 0.3 THz, and f3 = 0.35 THz) and the same topological charge (A) l1 = 0 (B) l2 = +2 (C) l3 = -2 (D) l4 = +4. Therefore, the 12-channel orthogonal on-axis beams with topological or frequency orthogonality can be achieved.
In addition, the phase gradient method [46] can be used to measure the purity of the generated OAM mode based on the simulated far field phase. For the reflective metasurface, take channel C23 for example. The far field phase of the RCP reflected wave is sampled at a pitch angle of 5° and an interval of 1° in the spherical coordinate system. For the transmissive metasurface, take channel C43 for example. The far field phase of the RCP transmitted wave is sampled at a pitch angle of 10° and an interval of 0.5°. Table 1 shows the calculated variances σ2 for different OAM modes. It can be seen that for channel C23, when the topological charge l is set as +2 during calculation, the variance is the smallest and the purity is the highest. Therefore, the topological charge of the generated OAM beam is +2. Similarly, for channel C43, when l = +4, the variance is the smallest and the purity is the highest. Therefore, the topological charge of the generated OAM beam is +4.
Based on the above results, two-dimensional 12-channel multiplexing combing with spatial and frequency domains by the reconfigurable dielectric metasurface is realized. Besides, the metasurface works in both reflection mode and transmission mode with the operating frequency band switching from 0.18–0.28 THz to 0.25–0.35 THz by tuning the VO2.
Conclusion
A terahertz reconfigurable dielectric metasurface embedded with VO2 for two-dimensional multichannel multiplexing combing with spatial and frequency domains is presented in this paper. The metasurface works in both reflection mode and transmission mode by switching the VO2 from the metallic state to the insulator state. For reflective metasurface, 4×M-channel off-axis RCP plane wave with fm and angle of incidence θi (fm) (m = 1, … , M) illuminate on the metasurface from the negative z-axis along with negative x and y directions, and positive x and y directions respectively, in the direction perpendicular to the metasurface, RCP reflected waves are transformed into 4×M-channel orthogonal on-axis beams with topological orthogonality (l = l1, l2, l3, and l4) or frequency orthogonality (f = f1 to fM). For transmissive metasurface, 4×M-channel off-axis LCP plane waves are incident on the metasurface from the positive z-axis along with negative x and y directions, and positive x and y directions respectively, in the direction perpendicular to the metasurface, RCP transmitted waves are transformed into 4×M-channel orthogonal on-axis beams with topological or frequency orthogonality. A metasurface composed of 14 × 14 unit cells with l1 = 0, l2 = +2, l3 = -2, and l4 = +4 is designed for verification. The metasurface works in both reflection and transmission modes with the operating frequency band switching from 0.18–0.28 THz to 0.25–0.35 THz by tuning the state of VO2. When the metasurface works in the reflection or transmissive mode, take f1 = 0.18 THz, f2 = 0.23 THz, and f3 = 0.28 THz, or f1 = 0.25 THz, f2 = 0.3 THz, and f3 = 0.35 THz for examples. The simulation results show that two-dimensional 12-channel multiplexing combing with spatial and frequency domains by the metasurface is realized. The proposed metasurface has great potential in terahertz communications.
Data availability statement
The original contributions presented in the study are included in the article/supplementary material, further inquiries can be directed to the corresponding authors.
Author contributions
LW, YY, and LD conceived the work and suggested the outline of the article. LW, FG, ST, ZT, XZ, and JL carried out investigations and wrote the article.
Funding
This research was funded in part by the Natural Science Foundation of Hunan Province, grant number 2022JJ40114 and in part by the Research Foundation of Education Bureau of Hunan Province, grant number 21C0831.
Conflict of interest
The authors declare that the research was conducted in the absence of any commercial or financial relationships that could be construed as a potential conflict of interest.
Publisher’s note
All claims expressed in this article are solely those of the authors and do not necessarily represent those of their affiliated organizations, or those of the publisher, the editors and the reviewers. Any product that may be evaluated in this article, or claim that may be made by its manufacturer, is not guaranteed or endorsed by the publisher.
References
1. Bukhari SS, Vardaxoglou J, Whittow W. A metasurfaces review: Definitions and applications. Appl Sci (Basel) (2019) 9:2727. doi:10.3390/app9132727
2. Zhang LL, Li P, Song XW. Tunable wide-angle multi-band mid-infrared linear-to-linear polarization converter based on a graphene metasurface. Chin Phys B (2021) 30:127803. doi:10.1088/1674-1056/ac0cdd
3. Wu JB, Shen Z, Ge SJ, Chen BW, Shen ZX, Wang TF, et al. Liquid crystal programmable metasurface for terahertz beam steering. Appl Phys Lett (2020) 116:131104. doi:10.1063/1.5144858
4. Li Y, Lin J, Guo HJ, Sun WJ, Xiao SY, Zhou L. A tunable metasurface with switchable functionalities: From perfect transparency to perfect absorption. Adv Opt Mater (2020) 8:1901548. doi:10.1002/adom.201901548
5. Tsilipakos O, Tasolamprou AC, Pitilakis A, Liu F, Wang XC, Mirmoosa MS, et al. Toward intelligent metasurfaces: The progress from globally tunable metasurfaces to software‐defined metasurfaces with an embedded network of controllers. Adv Opt Mater (2020) 8:2000783. doi:10.1002/adom.202000783
6. Follan TG, Fali A, White ST, Matson JR, Liu S, Aghamiri NA, et al. Reconfigurable infrared hyperbolic metasurfaces using phase change materials. Nat Commun (2018) 9:4371–7. doi:10.1038/s41467-018-06858-y
7. Liu MK, Hwang HY, Tao H, Strikwerda AC, Fan K, Keiser GR, et al. Terahertz-field-induced insulator-to-metal transition in vanadium dioxide metamaterial. Nature (2012) 487:345–8. doi:10.1038/nature11231
8. Li CL, Yu P, Huang YJ, Zhou Q, Wu J, Li Z, et al. Dielectric metasurfaces: From wavefront shaping to quantum platforms. Prog Surf Sci (2020) 95:100584. doi:10.1016/j.progsurf.2020.100584
9. Winzer PJ. Spatial multiplexing in fiber optics: The 10x scaling of metro/core capacities. Bell Labs Tech J (2014) 19:22–30. doi:10.15325/BLTJ.2014.2347431
10. Allen L, Beijersbergen MW, Spreeuw RJC, Woerdman JP. Orbital angular momentum of light and the transformation of Laguerre-Gaussian laser modes. Phys Rev A (Coll Park) (1992) 45:8185–9. doi:10.1103/PhysRevA.45.8185
11. He JN, Wan ML, Zhang XP, Yuan SQ, Zhang LF, Wang JQ. Generating ultraviolet perfect vortex beams using a high-efficiency broadband dielectric metasurface. Opt Express (2022) 30:4806–16. doi:10.1364/OE.451218
12. Fu B, Yu SX, Kou N, Ding Z, Zhang ZP. Design of cylindrical conformal transmitted metasurface for orbital angular momentum vortex wave generation. Chin Phys B (2022) 31:040703. doi:10.1088/1674-1056/ac3a65
13. Zhang K, Wang YX, Burokur SN, Wu Q. Generating dual-polarized vortex beam by detour phase: From phase gradient metasurfaces to metagratings. IEEE Trans Microw Theor Tech (2021) 70:200–9. doi:10.1109/TMTT.2021.3075251
14. Wang Y, Wang H, Su RF, Li SH, Tu XC, Wu JB, et al. Flexible bilayer terahertz metasurface for the manipulation of orbital angular momentum states. Opt Express (2021) 29:33445–55. doi:10.1364/OE.439370
15. Wang YX, Zhang K, Yuan YY, Ding XM, Yang GH, Fu JH, et al. Generation of high-efficiency vortex beam carrying OAM mode based on miniaturized element frequency selective surfaces. IEEE Trans Magn (2019) 55:1–4. doi:10.1109/TMAG.2019.2919715
16. Ou K, Li GH, Li TX, Yang H, Yu FL, Chen J, et al. High efficiency focusing vortex generation and detection with polarization-insensitive dielectric metasurfaces. Nanoscale (2018) 10:19154–61. doi:10.1039/C8NR07480A
17. Jin ZW, Janoschka D, Deng JH, Ge L, Dreher P, Frank B, et al. Phyllotaxis-inspired nanosieves with multiplexed orbital angular momentum. ELight (2021) 1:5–11. doi:10.1186/s43593-021-00005-9
18. Ha YL, Guo YH, Pu MB, Li X, Ma XL, Zhang ZJ, et al. Monolithic‐Integrated multiplexed devices based on metasurface‐driven guided waves. Adv Theor Simul (2021) 4:2000239. doi:10.1002/adts.202000239
19. Liu MZ, Huo PC, Zhu WQ, Zhang C, Zhang S, Song MW, et al. Broadband generation of perfect Poincaré beams via dielectric spin-multiplexed metasurface. Nat Commun (2021) 12:2230–9. doi:10.1038/s41467-021-22462-z
20. Mai QL, Wang CF, Wang XR, Cheng SH, Cheng ML, He YL, et al. Metasurface based optical orbital angular momentum multiplexing for 100 GHz radio over fiber communication. J Lightwave Technol (2021) 39:6159–66. doi:10.1109/JLT.2021.3097176
21. Meng XS, Wu JJ, Wu ZS, Yang L, Huang L, Li X, et al. Erratum: “Generation of multiple beams carrying different orbital angular momentum modes based on anisotropic holographic metasurfaces in the radio-frequency domain” [appl. Phys. Lett. 114, 093504 (2019)]. Appl Phys Lett (2019) 114:139902. doi:10.1063/1.5096976
22. Feng Q, Kong XD, Shan MM, Lin YF, Li L, Cui TJ. Multi-orbital-angular-momentum-mode vortex wave multiplexing and demultiplexing with shared-aperture reflective metasurfaces. Phys Rev Appl (2022) 17:034017. doi:10.1103/PhysRevApplied.17.034017
23. Yuan YY, Zhang K, Ratni B, Song QH, Ding XM, Wu Q, et al. Independent phase modulation for quadruplex polarization channels enabled by chirality-assisted geometric-phase metasurfaces. Nat Commun (2020) 11:4186–9. doi:10.1038/s41467-020-17773-6
24. Zheng CL, Wang GC, Li J, Li JT, Wang SL, Zhao HL, et al. All‐dielectric metasurface for manipulating the superpositions of orbital angular momentum via spin‐decoupling. Adv Opt Mater (2021) 9:2002007. doi:10.1002/adom.202002007
25. Li SQ, Li XY, Zhang L, Wang GX, Zhang LX, Liu ML, et al. Efficient optical angular momentum manipulation for compact multiplexing and demultiplexing using a dielectric metasurface. Adv Opt Mater (2020) 8:1901666. doi:10.1002/adom.201901666
26. Chen SQ, Xie ZQ, Ye HP, Wang XR, Guo ZH, He YL, et al. Cylindrical vector beam multiplexer/demultiplexer using off-axis polarization control. Light Sci Appl (2021) 10:222–9. doi:10.1038/s41377-021-00667-7
27. Wang L, Yang Y, Deng L, Hong WJ, Zhang C, Li SF. Terahertz angle‐multiplexed metasurface for multi‐dimensional multiplexing of spatial and frequency domains. Adv Theor Simul (2020) 3:2000115. doi:10.1002/adts.202000115
28. Jiang ZH, Kang L, Hong W, Werner DH. Highly efficient broadband multiplexed millimeter-wave vortices from metasurface-enabled transmit-arrays of subwavelength thickness. Phys Rev Appl (2018) 9:064009. doi:10.1103/PhysRevApplied.9.064009
29. Hu YZ, Tong MY, Xu ZJ, Cheng XG, Jiang T. Spatiotemporal terahertz metasurfaces for ultrafast all‐optical switching with electric‐triggered bistability. Laser Photon Rev (2021) 15:2000456. doi:10.1002/lpor.202000456
30. Kim Y, Wu PC, Sokhoyan R, Mauser K, Glaudell R, Shirmanesh GK, et al. Phase modulation with electrically tunable vanadium dioxide phase-change metasurfaces. Nano Lett (2019) 19:3961–8. doi:10.1021/acs.nanolett.9b01246
31. Urade Y, Fukawa K, Miyamaru F, Okimura K, Nakanishi T, Nakata Y. Dynamic inversion of planar-chiral response of terahertz metasurface based on critical transition of checkerboard structures. Nanophotonics (2022) 11:2057–64. doi:10.1515/nanoph-2021-0671
32. Tripathi A, Kruk S, John J, Zhang Z, Nguyen HS, Berguiga L, et al. Tunable Mie-resonant dielectric metasurfaces based on VO2 phase-transition materials. ACS Photon (2021) 8:1206–13. doi:10.1021/acsphotonics.1c00124
33. Yan DX, Feng QY, Yuan ZW, Meng M, Li XJ, Qiu GH, et al. Wideband switchable dual-functional terahertz polarization converter based on vanadium dioxide-assisted metasurface. Chin Phys B (2022) 31:014211. doi:10.1088/1674-1056/ac05a7
34. Shu FZ, Wang JN, Peng RW, Xiong B, Fan RH, Gao YJ, et al. Electrically driven tunable broadband polarization states via active metasurfaces based on joule‐heat‐induced phase transition of vanadium dioxide. Laser Photon Rev (2021) 15:2100155. doi:10.1002/lpor.202100155
35. Kou W, Shi WQ, Zhang YX, Yang ZQ, Chen T, Gu JQ, et al. Terahertz switchable focusing planar lens with a nanoscale vanadium dioxide integrated metasurface. IEEE Trans Terahertz Sci Technol (2021) 12:13–22. doi:10.1109/TTHZ.2021.3105576
36. Wu GZ, Jiao XF, Wang YD, Zhao ZP, Wang YB, Liu JG. Ultra-wideband tunable metamaterial perfect absorber based on vanadium dioxide. Opt Express (2021) 29:2703–11. doi:10.1364/OE.416227
37. Liu WW, Song ZY. Terahertz absorption modulator with largely tunable bandwidth and intensity. Carbon (2021) 174:617–24. doi:10.1016/j.carbon.2020.12.001
38. Kang L, Wu YH, Werner DH. Active terahertz spin Hall effect in vanadium dioxide metasurfaces. Opt Express (2021) 29:8816–23. doi:10.1364/OE.421283
39. Li J, Li JT, Zhang YT, Li JN, Yang Y, Zhao HL, et al. All-optical switchable terahertz spin-photonic devices based on vanadium dioxide integrated metasurfaces. Opt Commun (2020) 460:124986. doi:10.1016/j.optcom.2019.124986
40. Chen L, Zhao L, Hao Y, Liu WY, Wu Y, Wei ZC, et al. Metasurface spiral focusing generators with tunable orbital angular momentum based on slab silicon nitride waveguide and vanadium dioxide (VO2). Nanomaterials (2020) 10:1864. doi:10.3390/nano10091864
41. Liu XB, Wang Q, Zhang XQ, Li H, Xu Q, Xu YH, et al. Thermally dependent dynamic meta‐holography using a vanadium dioxide integrated metasurface. Adv Opt Mater (2019) 7:1900175. doi:10.1002/adom.201900175
42. Wang L, Yang Y, Deng L, Hong WJ, Zhang C, Li SF. Vanadium dioxide embedded frequency reconfigurable metasurface for multi-dimensional multiplexing of terahertz communication. J Phys D Appl Phys (2021) 54:255003. doi:10.1088/1361-6463/abf166
43. Ding XM, Monticone F, Zhang K, Zhang L, Gao DL, Burokur SN, et al. Ultrathin Pancharatnam–Berry metasurface with maximal cross‐polarization efficiency. Adv Mater (2015) 27:1195–200. doi:10.1002/adma.201405047
44. Tan H, Deng J, Zhao RZ, Wu X, Li GX, Huang LL, et al. A free‐space orbital angular momentum multiplexing communication system based on a metasurface. Laser Photon Rev (2019) 13:1800278. doi:10.1002/lpor.201800278
45. Yu NF, Genevet P, Kats MA, Aieta F, Tetienne JP, Capasso F, et al. Light propagation with phase discontinuities: Generalized laws of reflection and refraction. Science (2011) 334:333–7. doi:10.1126/science.1210713
Keywords: terahertz, dielectric metasurface, vanadium dioxide, multiplexing, orbital angular momentum
Citation: Wang L, Yang Y, Gao F, Teng S, Tan Z-G, Zhang X, Lou J and Deng L (2022) Terahertz reconfigurable dielectric metasurface hybridized with vanadium dioxide for two-dimensional multichannel multiplexing. Front. Phys. 10:992037. doi: 10.3389/fphy.2022.992037
Received: 12 July 2022; Accepted: 16 August 2022;
Published: 06 September 2022.
Edited by:
Lin Chen, University of Shanghai for Science and Technology, ChinaReviewed by:
Dexian Yan, China Jiliang University, ChinaHuawei Liang, Shenzhen University, China
Fei Fan, Nankai University, China
Kuang Zhang, Harbin Institute of Technology, China
Guoxing Zheng, Wuhan University, China
Copyright © 2022 Wang, Yang, Gao, Teng, Tan, Zhang, Lou and Deng. This is an open-access article distributed under the terms of the Creative Commons Attribution License (CC BY). The use, distribution or reproduction in other forums is permitted, provided the original author(s) and the copyright owner(s) are credited and that the original publication in this journal is cited, in accordance with accepted academic practice. No use, distribution or reproduction is permitted which does not comply with these terms.
*Correspondence: Ling Wang, d2FuZ2xpbmdAaG5mbnUuZWR1LmNu; Li Deng, ZGVuZ2xAYnVwdC5lZHUuY24=