- 1Institute of Quantum Electronics, Peking University, Beijing, China
- 2Institute of Fundamental Experiment Education, Peking University, Beijing, China
The research of optically pumped cesium beam atomic clock (OPCB) at Peking University has lasted for decades. At present, the short-term frequency stability is
1 Introduction
The portable cesium beam atomic clock occupies an important position in the field of time and frequency [1]. Its excellent medium and long-term frequency stability can not be completely replaced by other atomic clocks. Cesium beam atomic clocks can be divided into two types: magnetic state-selection and optically pumped. Compact magnetic state-selection cesium beam atomic clocks have commercial products and have been widely used, such as 5071A [2] from the Microsemi, OSA3235B [3] from Oscilloquartz and LIP-Cs3000 [4] from China. The frequency stability of the magnetic state-selection cesium beam atomic clocks is approximately
Researches on OPCB have been carried out both in China and abroad. GPS3 [6] using commercial laser can reach
This paper introduces the improvement of the frequency stability of the portable OPCB in Peking University. It is divided into the following parts: in the Section 2, the principle of portable OPCB is introduced. Section 3 introduced the improvement of short-term and long-term frequency stability of portable OPCB. The last section is a conclusion.
2 Principle
The portable OPCB is a closed-loop feedback system, which can output the transition frequency of cesium atoms. It can be divided into three parts: cesium beam tube, optical part and circuits.
2.1 Cesium beam tube
The cesium beam tube part of the portable OPCB includes a vacuum tube, a cesium oven, a microwave cavity and two fluorescence collecting bowls (Figure 1). The vacuum tube provides an environment for realizing atomic transition. It is a cylindrical tube approximately 40 cm long and approximately 18 cm in diameter. After sealing, its interior is pumped to a vacuum of 10−7 Pa. In such a vacuum environment, the cesium atomic beam can hardly collide with stray gas. The cesium oven stores about 5 g cesium atoms. Under the heating effect of the heating wire, the cesium atoms are gasified into gas and ejected from the collimator on the cesium oven due to the saturated steam pressure to form a cesium atomic beam. At 100 °C, the flux of cesium atomic beam can reach 4 × 1013 atom/s. Under this beam flow, the cesium oven can continuously eject cesium atoms for 10 years, meeting the needs of long-term continuous operation and time-keeping. The microwave cavity is a waveguide cavity in the shape of two arms. When the cesium atomic beam passes through the openings of the two arms of the microwave cavity, it interacts with the microwave and realize the Ramsey double resonance [16]. The length of the microwave cavity is 16.7 cm, and the opening of the microwave cavity is about 12 mm2. The detuning of the microwave cavity can be controlled within 500 kHz. There are two fluorescence collecting bowls in the OPCB, which provides a place for the interaction between light and atoms. When the atomic beam passes through fluorescence collecting bowls, it interacts with a vertical laser beam and generates fluorescence. The fluorescence is reflected in the fluorescent bowl and is collected by photodetectors installed on fluorescent bowls. The fluorescence collection efficiency can reach 25%.
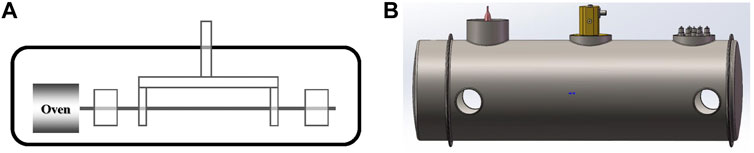
FIGURE 1. Cesium beam tube of the OPCB. (A) There is a cesium oven, a microwave cavity and two fluorescent collecting bowls, which are arranged in a straight line in a cesium beam tube. (B) A cesium beam tube of the portable OPCB in Peking university.
2.2 Optical bench
Compared with magnetic state-selection cesium beam atomic clock, the optical part is added to the portable OPCB. The optical part mainly includes a laser, an isolator, an acousto-optic modulator (AOM), a liquid crystal phase retarder and various optical lenses (Figure 2). The output wavelength of the laser is 852 nm, which corresponds to the transition of the D2 line of cesium atoms. Its maximum output power can reach 100 mW, which is enough to meet the requirements of laser pumping and laser detection. The function of the optical isolator is to prevent the laser beam from being fed back inside to the laser thereby affecting the operation of the laser and resulting in the instability of the laser frequency and power. The function of the AOM is to realize laser frequency shift. The pumping laser uses

FIGURE 2. Optical bench of the OPCB. (A) The pumping laser and the probing laser are generated by one laser. (B) A photo of the optical bench.
2.3 Circuits
The circuit system of the portable OPCB consists of three parts: microwave source, control-servo part and auxiliary circuits. The auxiliary circuits include power supply circuit, screen display part, frequency output and phase synchronization part, satellite calibration part and other interactive parts. The microwave source realizes the function of frequency multiplication of 10 MHz OCXO crystal oscillator signal to 9192 MHz microwave signal. The maximum output power of the microwave source is 5 dBm, and the obtained phase noise is − 45dBc@1Hz. The phase noise can reach − 120dBc@1MHz, and the frequency and power of the final microwave signal can be accurately tuned. The frequency adjustment accuracy can reach 1μHz, and the power adjustment accuracy can reach 0.01 dBm. The control-servo part realizes the precise control of the operating parameters in the OPCB, including maintaining the high-voltage for vacuum, controlling the cesium oven temperature, controlling the C-field current, laser driver, AOM and liquid crystal retarder driver circuits. The high-voltage can be maintained at 3500 V, the fluctuation range of the cesium oven temperature can be stabilized within 0.01°C for a long time, the fluctuation range of the C-field current can be controlled within 100 nA, and the fluctuation range of the laser current can be controlled within 10 μA. The control-servo part also includes OCXO closed-loop locking, active C-field intensity servo, active microwave power servo and active laser powers servo.
3 Improvement of frequency stability
The frequency stability of the OPCB includes two aspects: short-term frequency stability and long-term frequency stability. The short-term frequency stability mainly depends on the signal-to-noise ratio of the obtained Ramsey signal, while the long-term frequency stability mainly depends on the sensitivity of both the controlled system parameters and the associated frequency shift to the environment.
3.1 Short-term frequency stability
The short-term frequency stability of the portable OPCB is inversely proportional to the signal-to-noise ratio. The higher the signal-to-noise ratio of Ramsey signal, the better the short-term frequency stability:
3.1.1 Atomic beam
Adding a collimator to the cesium oven can improve the intensity of cesium atomic beam. There is a certain divergence angle after cesium atoms ejected from the cesium oven. If a collimator is not installed, the divergence angle of cesium atomic beam is large (Figure 3), and the number of effective cesium atoms that can actually pass through the two microwave cavities to the detection area is small. That means the signal-to-noise ratio of the beam reduced [17]:
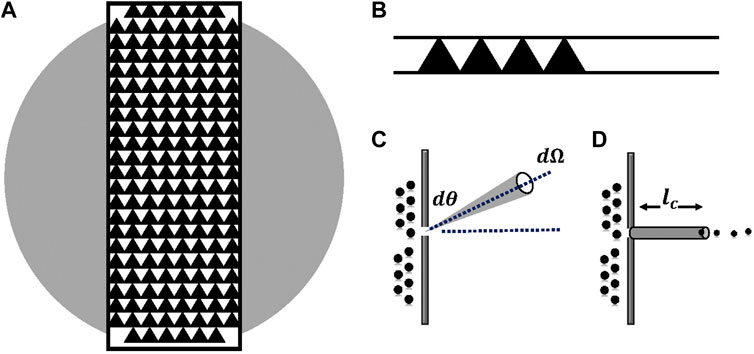
FIGURE 3. Cesium oven collimator of the OPCB. Front view: Cesium oven nozzle is rectangular, consists of many triangular thin tubes (0.003 mm2), with a total area of 12 mm2. (A) Zoom out (B) zoom in. Side view: (C) no collimator (D) collimator.
The length of the collimator of the cesium oven in our OPCB has been increased to 6mm, and the nozzle area of the collimator is about 12 mm2 (Figure 3). In this case, the divergence angle of the cesium atomic beam can be controlled to about 40 mrad. The actual cesium atomic beam flow from the collimator is 4 × 1013 atoms/s, and the actual effective cesium atomic beam flow in the detection area is:
It is approximately 109 atoms/s. Therefore, it can be concluded that the signal-to-noise ratio of the beam noise in the portable OPCB is approximately 30,000, and the short-term frequency stability that can be supported reaches
3.1.2 Laser stabilization
Using atomic beam spectrum to stabilize the laser frequency can reduce laser noise [18]. Compared with previous laser frequency stabilization method (saturated absorption spectrum frequency stabilization), beam spectrum frequency stabilization has the following advantages (Figure 4):
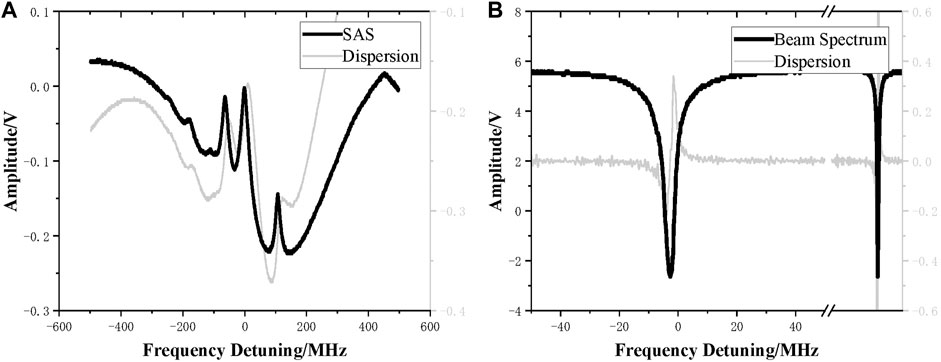
FIGURE 4. Laser stabilization spectroscopy of the OPCB. (A) Saturated absorption spectrum. (B) Atomic beam spectrum.
First, the intensity of the beam spectrum is greater than that of the saturated absorption spectrum. The frequency stabilization using saturated absorption spectrum needs a cesium cell in the optical path. The number of cesium atoms used in the saturated absorption spectrum in a cell is approximately 106 atoms, while the effective number of cesium atoms used in the frequency stabilization by atomic beam spectrum is approximately 109 atoms.
Second, the laser frequency obtained by beam spectrum can generate the maximum fluorescence signal. The beam spectrum has no Doppler background and no interference of cross absorption peaks. When using the saturable absorption spectrum, there will be a frequency shift of the transition peak due to the existence of the Doppler background and the larger cross-absorption peaks. At the same time, there will be an frequency error between the cesium atoms and the cesium atomic beam because the probing laser and the cesium atomic beam in detection area may not be completely vertical. Therefore, the laser frequency obtained by saturated absorption spectrum may not generate the maximum fluorescence signal.
Third, cesium cell is highly sensitive to the environment. The atomic beam is in the cesium atomic beam tube and the magnetic shielding layer of the tube effectively shields the influence of the external magnetic field. Meanwhile the cesium atoms in cell are greatly affected by the ambient temperature and there are more collisions between atoms and the cell, but this does not occur in a cesium atomic beam.
Fourth, the long-term reliability of a beam spectrum frequency stabilization is better. Cesium beam atomic clock needs long-term and continuous operation. So, maintaining long-term and reliable frequency stabilization of the laser is important. Compared with the saturated absorption spectrum, the beam spectrum has only three peaks. And it is easy to identify the largest
3.1.3 Cyclic transition
Using
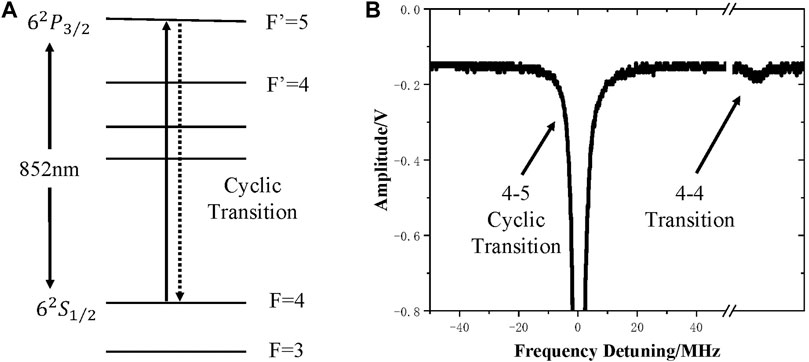
FIGURE 5. Cyclic transition of the OPCB. (A)
Through the above three improvements, under the conditions of the commercial DFB laser, the short-term frequency stability of the portable OPCB in our group has reached
3.2 Long-term frequency stability
In a portable OPCB, microwave power, C-field intensity and laser powers are the three most sensitive parameters to the environment. During the long-term operation of the OPCB, they need to be maintained by active servos otherwise the OPCB can not withstand the environmental fluctuations. For the portable OPCB, if the frequency stability needs to be less than 1 × 10−14 at 5 days, under the assumption of a linear drift,
and according to the calculation formula of Allan variance, we have:
The drift rate of the portable OPCB should be controlled in the range of 2.8 × 10−15 /day.
3.2.1 Microwave servo
We use the transition probability curve to servo the microwave power (Figure 6). The reason is that this curve is depended by the physical system and is a stable reference. Compared with the locking method through the detection of microwave power, this method can eliminate the influence of the microwave cavity, the microwave signal wiring and other parts. It directly control the microwave power actually interacting with atoms. In other words, the method decides the Rabi frequency of the interaction. During the operation of the OPCB, the microwave power is served once every 2 min. The microwave power is square wave modulated to obtain the error signal, which is then fed back to the microwave source. In normal operation, the microwave source only has an bad impact on the frequency stability of the OPCB after several thousands seconds averaging time. Therefore, the servo every 2 min can achieve a good long-term maintenance of the microwave power.
3.2.2 C-field servo
The intensity of the C-field directly affects the second-order Zeeman frequency shift in the OPCB and also affects the Rabi pulling frequency shift in the OPCB. During the long-term operation of the portable OPCB, the change of the external magnetic field will lead to the change of the internal magnetic field in the cesium beam tube, resulting in a frequency shift. We lock the C-field intensity according to the Zeeman frequency of the cesium atomic spectral line (Figure 7). The Zeeman frequency refers to the frequency difference between the mF = 0 and mF = +1 Ramsey signals. The magnitude of Zeeman frequency is directly related to the magnetic field intensity in the actual microwave cavity,
Since the frequency resolution of the Ramsey resonance line in the OPCB can reach 1 × 10−12, the locking of C-field intensity can reach 10−5. At this time, the control accuracy of second-order Zeeman effect can be within the range of 10−15. In order to reduce the impact of Rabi pulling on the atomic clock, we control the Zeeman frequency of cesium atomic clock at 60 kHz. In this case, the frequency uncertainty caused by Rabi pulling can be controlled and the impact of the magnetic field on stability is eliminated during long-term operation.
3.2.3 Laser power servo
The fluctuation of laser powers will bring about the light shift and the fluctuation of the Ramsey signal, which will affect the normal close-loop of the OPCB. We have measured the frequency shift caused by laser power fluctuation, and found that the frequency shift caused by laser powers are approximately 4 × 10−13 /mw. In the case of 10−2 /day drift in laser power, the frequency shift caused by laser power change can reach 4 × 10−15 /day, which exceeds the requirement of the portable OPCB. The laser power drift caused by the aging of the laser and the long-term aging of the optical devices can hardly be overcome, so it is necessary to servo the laser powers actively. We proposed a method of using fluorescence to lock the laser powers (Figure 8). Compared with locking the laser powers in optical path, the method has the following advantages: 1) Directly controlling the intensity of fluorescence inside the cesium beam tube, which is the direct causes of the light shift. 2) Controlling the Ramsey signal of the atomic clock to make the feedback system of the OPCB stable. The control of the laser powers makes the frequency drift caused by the laser powers shift in the order of 10−17, which meets the requirement.
After the above works, the long-term frequency stability of the portable OPCB in our group has been improved. The latest measurement results show that the long-term (5-day) frequency stability of the portable OPCB can exceed 7 × 10−15.
4 Conclusions and prospects
The frequency stability of the portable OPCB is shown in Figure 9, which respectively shows the research results over the years, from
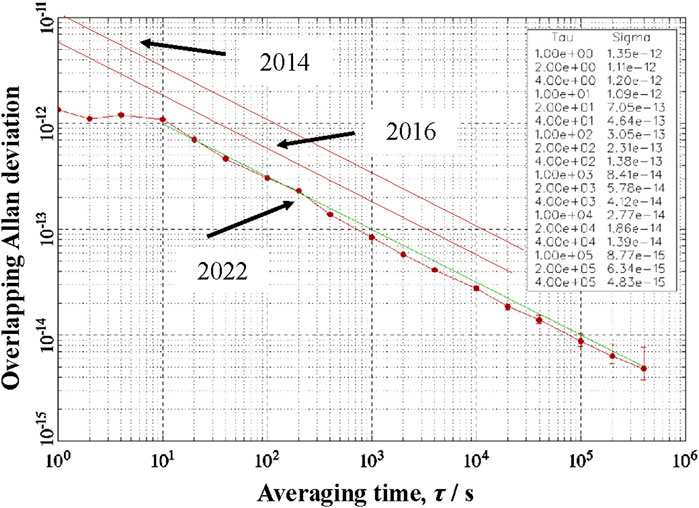
FIGURE 9. Overlapping Allan deviation of the OPCB. In 2014, the short-term stability reached
In the future, we will continue the research of the OPCB. One the one hand, we will continue to improve the frequency stability of the OPCB through the following methods: 1) Using a narrower linewidth laser. The frequency noise of the laser is one of the main noise sources in OPCBs [19] at present; 2) Using two lasers to prepare the atomic state [20]. The method can greatly increase the effective atomic beam flow and reduce the beam noise; 3) Using 2D-MOT to realize the cold cesium atomic beam [21]. The method can reduce the linewidth of the Ramsey signal. On the other hand, we will also explore the frequency stability performance of the portable OPCB in complex environments, such as in vehicle, satellite and others.
The portable OPCB is expected to move towards commercial production, make contributions to the time-frequency field in China and even the world, and provide important reference instruments for time-frequency service systems, satellite navigation and positioning systems and high-speed digital communication systems.
Author contributions
XQ, QW, and XC contributed to conception and design of the study. XH, SF, and XQ organized the database. XH performed the statistical analysis. XH, ZY, and JC wrote the first draft of the manuscript. All authors contributed to manuscript revision, read, and approved the submitted version.
Funding
This work is supported by the National Natural Science Foundation of China (Grants Nos.11920101004, 11334001, 61727819, 61475007), and the National Key Research and Development Program of China (Grant Nos. 2021YFA1400900, 2021YFA0718300).
Acknowledgments
The author would like to thank all the predecessors who have made efforts in the research project of portable OPCB, especially Yiqiu Wang, Donghai Yang, Jianwei Zhang and others.
Conflict of interest
The authors declare that the research was conducted in the absence of any commercial or financial relationships that could be construed as a potential conflict of interest.
Publisher’s note
All claims expressed in this article are solely those of the authors and do not necessarily represent those of their affiliated organizations, or those of the publisher, the editors and the reviewers. Any product that may be evaluated in this article, or claim that may be made by its manufacturer, is not guaranteed or endorsed by the publisher.
References
1. Vanier J, Audoin C. The classical caesium beam frequency standard: Fifty years later. Metrologia (2005) 42:S31–S42. doi:10.1088/0026-1394/42/3/s05 |
2.Microsemi. 5071a primary frequency standard. Aliso Viejo, California, United States: Microsemi (2018). Available from: https://www.microsemi.com/product-directory/cesium-frequency-references/4115-5071a-cesium-primary-frequency-standard (Accessed September 6, 2022).
3.Oscilloquartz. Osa 3235b cesium clock. Neuchâtel, Switzerland: Oscilloquartz (2022). Available from: https://www.oscilloquartz.com/en/products-and-services/cesium-clocks/osa-3230-series (Accessed September 6, 2022).
4. Chen J, Ma P, Wang J, Guo L, Ma Y, Cui J, et al. Progress in commercialization of compact magnetically selected cesium atomic clocks. J Astronautic Metrology Meas (2020) 40:12–6. doi:10.12060/j.issn.1000-7202.2020.03.03 |
5. Picqué J-L. Hyperfine optical pumping of a cesium atomic beam, and applications. Metrologia (1977) 13:115–9. doi:10.1088/0026-1394/13/3/006 |
6. Lutwak R, Emmons D, Garvey R, Vlitas P. Optically pumped cesium-beam frequency standard for gps-iii (2001). p. 15.
7. Lecomte S, Haldimann M, Ruffieux R, Berthoud P, Thomann P. Performance demonstration of a compact, single optical frequency cesium beam clock for space applications. In: 2007 IEEE International Frequency Control Symposium Joint with the 21st European Frequency and Time Forum. Geneva, Switzerland: IEEE (2007). p. 1127–31. doi:10.1109/FREQ.2007.4319254 |
8. Gurandell S, Hermann V, Barillet R, Cerez P, Theobald G, Audoin C, et al. Operation of a compact cesium beam tube: How to improve the stability towards the 10/sup -12//spl tau//sup -1/2/level. In: Proceedings of the 2002 IEEE International Frequency Control Symposium and PDA Exhibition (Cat. No.02CH37234). New Orleans, LA, USA: IEEE (2002). p. 480–3. doi:10.1109/FREQ.2002.1075931 |
9. Spaceon C. Ta1000 optically pumped cesium beam atomic clock (2022). Available from: http://www.elecspn.com/productdetail-1395.html (Accessed September 6, 2022).
10. Yang DH, Wang YQ. Preliminary results of an optically pumped cesium beam frequency standard at peking University. IEEE Trans Instrum Meas (1991) 40:1000–2. doi:10.1109/19.119781 |
11. Chen J, Wang F, Wang Y, Yang D. A new design of a diffused laser light optically pumped small cesium beam frequency standard. IEEE Trans Ultrason Ferroelectr Freq Control (2000) 47:457–60. doi:10.1109/58.827435 | |
12. Dong-Hai ZJW, Dong-Hai Y. High performance small optically pumped caesium beam frequency standard. Chin Phys Lett (2007) 24:1553–5. doi:10.1088/0256-307x/24/6/033 |
13. He X, Fang S, Yuan Z, Xie W, Chen N, Xiong Z, et al. Compact optically pumped cesium beam atomic clock with a 5-day frequency stability of 7 × 10 − 15. Appl Opt (2021) 60:10761–5. doi:10.1364/AO.443812 | |
14. He X, Wang Q, Xie W, Chen N, Xiong Z, Qi X, et al. A high performance and portable optically pumped cesium beam frequency standard. In: 2020 Joint Conference of the IEEE International Frequency Control Symposium and International Symposium on Applications of Ferroelectrics (IFCS-ISAF). Keystone, CO, USA: IEEE (2020). p. 1–4. doi:10.1109/IFCS-ISAF41089.2020.9234812 |
15. Xie W, Wang Q, He X, Chen N, Xiong Z, Fang S, et al. Frequency instability of a miniature optically pumped cesium-beam atomic frequency standard. Rev Scientific Instr (2020) 91:074705. doi:10.1063/5.0001749 | |
16. Ramsey NF. A molecular beam resonance method with separated oscillating fields. Phys Rev (1950) 78:695–9. doi:10.1103/PhysRev.78.695 |
17. Dimarcq N, Giordano V, Cerez P. Statistical properties of laser-induced fluorescence signals. Appl Phys B (1994) 59:135–45. doi:10.1007/BF01081164 |
18. Wang Q, Duan J, Qi X-H, Zhang Y, Chen X. Improvement of laser frequency stabilization for the optical pumping cesium beam standard. Chin Phys Lett (2015) 32:054206. doi:10.1088/0256-307x/32/5/054206 |
19. Dimarcq N, Giordano V, Cerez P, Theobald G. Analysis of the noise sources in an optically pumped cesium beam resonator. IEEE Trans Instrum Meas (1993) 42:115–20. doi:10.1109/19.278532 |
20. Avila G, Giordano V, Candelier V, de Clercq E, Theobald G, Cerez P. State selection in a cesium beam by laser-diode optical pumping. Phys Rev A (Coll Park) (1987) 36:3719–28. doi:10.1103/PhysRevA.36.3719 | |
21. Xie W, Wang Q, He X, Qi X, Chen X. Optically pumped cold cesium beam atomic clock based on two-dimensional magneto-optical trap. In: 2020 Joint Conference of the IEEE International Frequency Control Symposium and International Symposium on Applications of Ferroelectrics (IFCS-ISAF). Keystone, CO, USA: IEEE (2020). p. 1–4. doi:10.1109/IFCS-ISAF41089.2020.9234950 |
Keywords: cesium clock, optically pumped, stability, laser, frequency
Citation: He X, Yuan Z, Chen J, Fang S, Chen X, Wang Q and Qi X (2022) Improvement of the short- and long-term stability of high performance portable optically pumped cesium beam atomic clock. Front. Phys. 10:970030. doi: 10.3389/fphy.2022.970030
Received: 15 June 2022; Accepted: 16 August 2022;
Published: 15 September 2022.
Edited by:
José Paulo Santos, Universidade NOVA de Lisboa, PortugalReviewed by:
Xinye Xu, East China Normal University, ChinaMa Hongyang, Qingdao University of Technology, China
Copyright © 2022 He, Yuan, Chen, Fang, Chen, Wang and Qi. This is an open-access article distributed under the terms of the Creative Commons Attribution License (CC BY). The use, distribution or reproduction in other forums is permitted, provided the original author(s) and the copyright owner(s) are credited and that the original publication in this journal is cited, in accordance with accepted academic practice. No use, distribution or reproduction is permitted which does not comply with these terms.
*Correspondence: Xianghui Qi, xhqi@pku.edu.cn; Qing Wang, qw1988@pku.edu.cn