- 1National Key Laboratory of Science and Technology on Vacuum Electronics, Beijing Vacuum Electronics Research Institute, Beijing, China
- 2State Key Laboratory of Advanced Communication System and Networks, School of Electronics, Institute of Quantum Electronics, Peking University, Beijing, China
In order to get a better tradeoff between the long lifetime and high performances of cesium beam atomic clocks, we proposed a design scheme of compact optically pumped cesium beam tube (OPCBT) based on hexapole magnetic focusing (HMF) system for the confinement of cesium. A practical optically pumped cesium beam tube without HMF system has been implemented and tested with high-stability laser by modulation transfer spectroscopy (MTS), and a signal-to-noise ratio (SNR) of 18,000 in 1 Hz bandwidth and Allan deviation of
Introduction
Continuous atomic beams help to reduce the long-term frequency drift of atomic clocks [1–3]. As a reproduction device of the “definition of seconds”, cesium beam atomic clocks have a wide range of applications, for example, in time keeping, high-speed communication, navigation and fundamental research [4, 5]. As a core device, the cesium beam tube which provides a high vacuum environment for the generation of atomic beams and the interaction of radiation fields with atoms is the most important part affecting the performance and lifetime of cesium beam atomic clocks. For large primary cesium clocks in the laboratory, high performance and long life can be achieved together with big size [6–8]. For example, the resonance width of 62 Hz was obtained in NIST-7, served as the primary time and frequency standard in 1990s, with a Ramsey cavity length of 1.55 m [9–11]. And lifetime can be prolonged through increasing the use of more cesium in the Cs sources or timely supplement of Cs.
However, the length of Ramsey cavity in compact cesium clocks is less than 0.2 m, and the line width is generally around 350–800 Hz [12–14]. Therefore, increasing the atomic beam flux and thus improving the SNR is the most common way to improve the frequency stability of the Cs beam clocks. Unfortunately, the increase in cesium consumption shortens the lifetime of the Cs beam tubes [14]. For example, the compact magnetic state-selected cesium atomic clocks have a 10-years lifetime with the Standard Long-Life Cs Beam Tubes and a 5-years lifetime with the high-performance tubes whose consumption of atoms are large caused by high Cs oven temperature of about 130°C. Compact optically pumped cesium clocks with high atomic utilization have a lifetime of more than 10 years while ensuring high performance. To a certain extent, there has been a better tradeoff between performance and lifetime through optimization. However, the ultra-high-performance cesium clocks together with longer lifetime are expected in some application scenarios such as time keeping [15]. On the other hand, there is also the demand for cesium clocks with ultra-long lifetime of more than 20 years without performance degradation in satellites.
In this paper, commercial OPCBT are shown and tested with the high-stability laser by MTS. The obtained performances are the SNR of 18,000 in 1 Hz bandwidth and the Allan deviation of
Experiment on optically pumped cesium beam tube without hexapole magnetic focusing
One type of OPCBT without HMF has been manufactured in BVERI and over 200 tubes have been delivered to our customers and used in Cs beam clocks. The Cs beam tube is composed of Cs oven, Ramsey cavity, magnetic coil, magnetic shield, fluorescence detector, micro ion pump and vacuum shell. The size of the tube is 395 mm × 107 mm × 90 mm. The Cs oven has 200 micro-pores with a depth-to-diameter ratio (DDR) of 60 for the atom emission with a typical working temperature of 100°C. The distance between the two arms of Ramsey cavity is 165.8 mm, and the fluorescence collection efficiency was up to 80% in the reflective fluorescence detector.
The Cs atoms from the heated oven have the ground states of |F = 3> and |F = 4>, and are then prepared to |F = 3 > by the pumping laser in the region between the oven and the microwave cavity. Those Cs atoms with the state of |F = 3, mF = 0 > will have a transition chance to the state of |F = 4, mF = 0 > through the interaction with the microwave field in the Ramsey cavity. Subsequently, atoms populated on the state of |F = 4, mF = 0 > are detected by the laser probe. The fluorescence excited by the atoms will be converted to a current by the photodetector as frequency offset signal of the Cs beam clocks.
The overall configuration and diagram of the compact optically pumped cesium beam atomic clock based on the tube are shown in Figure 1. In order to reduce the laser frequency noise [18], a high-stability narrow-linewidth 852 nm laser by MTS was used in the experiments. The laser frequency for detection was locked to |F = 4>→|F’ = 5 > cycling transition, and the pumping laser was obtained by frequency shifting 251 MHz via an acousto-optic modulator (AOM), the corresponding energy level is shown in Figure 2A. The intensities of the detection laser and pumping laser are 0.7 mW/cm2 and 3.3 mW/cm2, respectively.
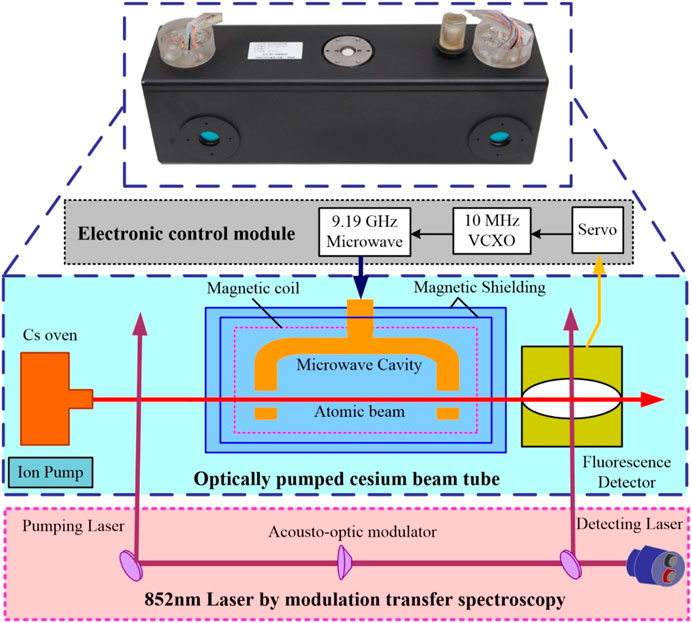
FIGURE 1. Overall configuration of the compact optically pumped cesium beam atomic clock. The cyan box is the diagram of the practical OPCBT made in BVERI, and the photograph is shown as above. The pink box is the 852 nm laser by MTS. The gray box is the electronic control module.
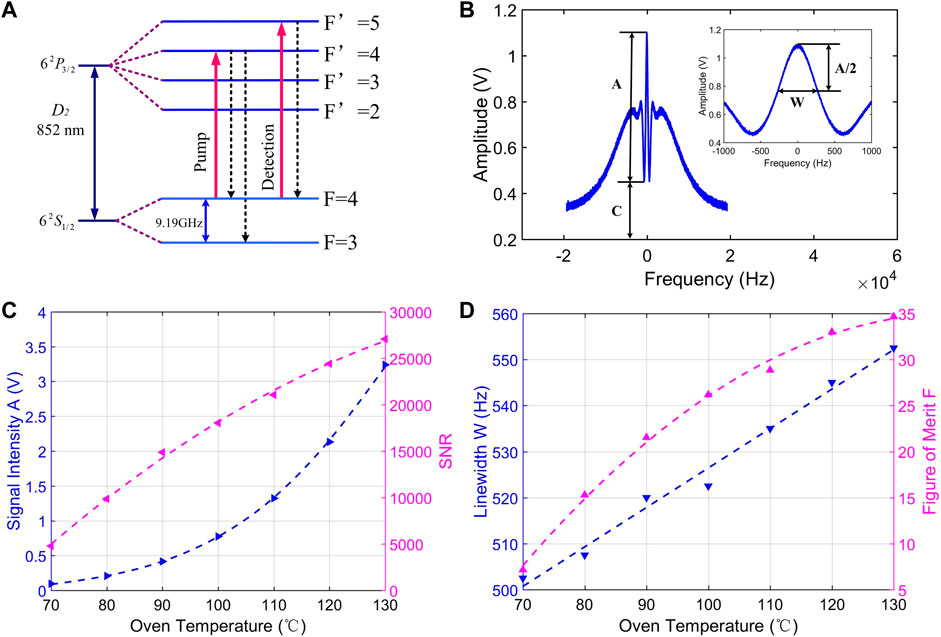
FIGURE 2. Energy levels and experimental results of OPCBT without HMF. (A) Relevant energy levels of cesium, |F = 4>→|F’ = 5> is cycling transition. (B) Ramsey fringes of the microwave clock transition, |F = 3, mF = 0>→ |F = 4, mF = 0>. (C) Measured signal intensity A (blue triangles) and SNR (purple triangles) in 1 Hz bandwidth at the Cs oven temperature of 70–130°C. (D) Measured linewidth W (blue triangles) and Figure of merit F (purple triangles) at the Cs oven temperature of 70–130°C.
The microwave signal applied to the Ramsey cavity comes from the frequency synthesis of a 10 MHz voltage controlled crystal oscillator (VCXO). Detailed design of the microwave source was descripted in [19]. The Ramsey pattern will be detected when the microwave frequency is tuned around 9192631770 Hz corresponding to the transition of ground state |F = 3, mF = 0 > to |F = 4, mF = 0>, as shown in Figure 2B. Linewidth, SNR and figure of merit are the most important indicators of cesium beam tubes, and are also the main factors that determine the frequency stability of cesium beam atomic clocks. The linewidth W and signal intensity A can be directly obtained from Figure 2B. Our cesium beam clocks operate at a microwave modulation frequency of 137 Hz, so the noise in 1 Hz bandwidth at 137 Hz of the cesium beam tubes will be measured by a fast Fourier transform (FFT) spectrum analyzer (Stanford Research Systems, SR770). The SNR is obtained by dividing the signal intensity A by noise N. A SNR of 18,000 in 1 Hz bandwidth was obtained when the Cs oven was operated at the typical temperature of 100°C. It is important to note that the microwave input to the cesium beam tubes is set to a spot frequency of 9192631770 Hz when measuring noise. The figure of merit F that characterizes the comprehensive performance of the tubes is defined by [1].
Where A is signal intensity, C is the valley or minimum voltage measured adjacent to the peak, as shown in Figure 2B,
As the Cs oven temperature rises from 70 to 130°C, the signal intensity rises exponentially. At the same time, the increase of the oven temperature means a large consumption of cesium and a significant reduction in the lifetime of Cs beam tubes. However, the growth of the SNR in 1 Hz bandwidth gradually slowed down and is shown in Figure 2C. This may be caused by useless atoms that are not absorbed by graphite getters. The linewidth of the central fringe increases linearly with the increase of the oven temperature, while the figure of merit F increase gradually, as shown in Figure 2D. It can be seen that constantly increased temperature does not keep the performance rising, but makes the life drop rapidly.
After modulating and demodulating the Ramsey fringes, an error signal is produced to lock the frequency of the 10 MHz VCXO. The output of the clock is also derived from the 10 MHz VCXO. Comparing with the 10 MHz sine output of a Hydrogen maser (VREMYA-CH, VCH-1003M, option L) by a high-performance phase noise and Allan deviation test set (Microsemi, 5120A), the Allan deviation of the compact optically pumped cesium beam atomic clock based on the tube without HMF is measured to be
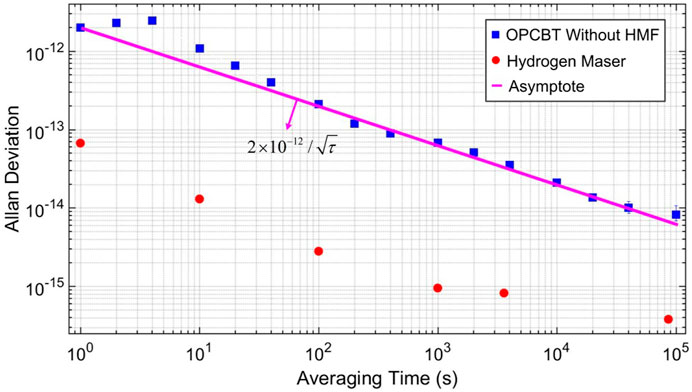
FIGURE 3. Allan deviation of the compact Cs beam clock based on the OPCBT without HMF (blue squares). The purple-solid line is the asymptote fitted with
According to the 8 g of cesium loaded in the oven and the typical working temperature of 100°C, the lifetime of the tubes without HMF is estimated to be more than 10 years. Although this has not been fully verified, some tubes have been running continuously for 9 years and show stable operation without any degradation of the performances.
Design of hexapole magnetic focusing for optically pumped cesium beam tubes
As is known, the cesium atomic beam emitted from the micro-hole array of oven is divergent, and most of the atoms are not utilized efficiently, and some even become the source of noise. Therefore, based on the above test results, reducing the oven temperature and increasing the atomic utilization rate is critical for improving the performance and lifetime. On the basis of the practical OPCBTs, a new tube that would realize a high flux of cesium atomic beam at low oven temperature has been designed. Figure 4 shows the overall approach, where two main measures are used to achieve the goals of “the low temperature operation of the Cs oven” and “the generation of high atomic beam flux".
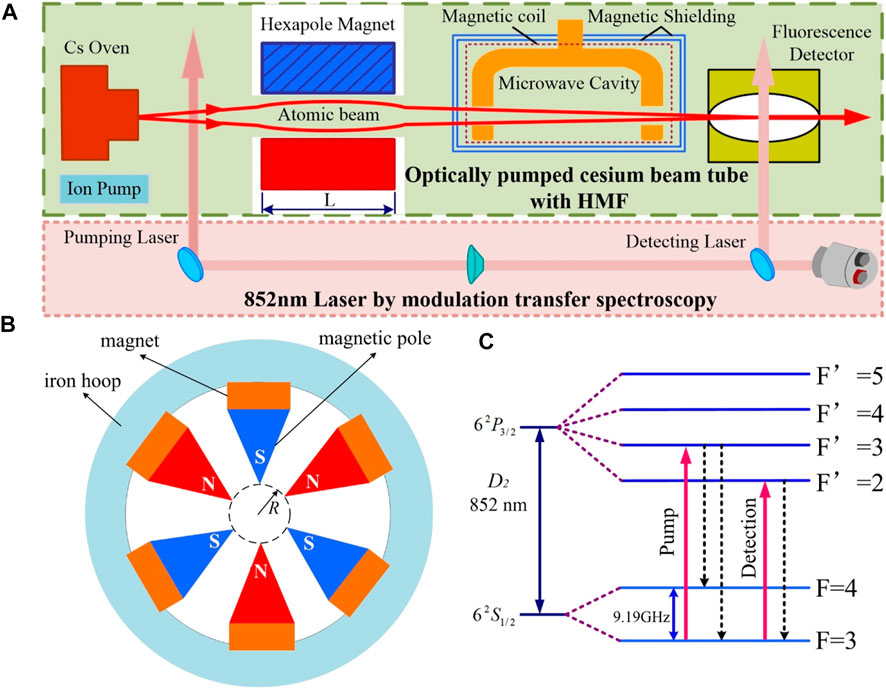
FIGURE 4. Energy levels and overall approach of OPCBT based on HMF. (A) The green box is the diagram of OPCBT with HMF system, L is the length of the hexa-pole magnets. The pink box is the 852 nm laser by MTS. (B) The cross-section of the HMF system, R is the bore radius of the hexa-pole magnets. (C) Relevant energy levels of cesium, |F = 3>→|F’ = 2> is cycling transition.
First, the DDR of the micro-pores for emitting atoms is increased from 60 to 100, which will reduce the divergence angle of the atomic beam and improve the atomic utilization rate. Limited by the magnetic field space inside the dipole magnetic state-selected cesium beam tube, the shape of the emission cross-section of the atomic beam is usually designed to be rectangular to form a sheet beam. In fact, although the shape of the emission cross-section is only limited by the laser beam diameter and the cutoff waveguide size of the microwave cavity in the OPCBT, the rectangular section is still used in the first generation of products considering the versatility of the cesium oven. In this protocol, a cross-section of emitted Cs atomic beam is nearly a square.
Second, a HMF system was added between the pumping area and the microwave cavity, as shown in Figure 4A. The HMF can confine the |F = 4 > atoms of large emission angles, which is similar to that a convex lens converges light. From the 1960s to the 1980s, the HMF system was studied to achieve the separation of cesium atoms with different energy states in the magnetic state-selected cesium beam clock [20–22], but was not ultimately chosen in compact cesium clocks. However, the HMF plays a new role of focusing the |F = 4 > atoms with a large divergence angle into the microwave cavity in this scheme. The cross-section of the HMF system is shown in Figure 4B.
It is important to note that only the cesium atoms of |F = 4 > state can be focused by the HMF, while those of |F = 3 > state are divergent. Therefore, in order to prepare the cesium atoms to |F = 4 > state, the pumping laser frequency will be locked to |F = 3>→|F’ = 3 > transition. In the microwave cavity region, a small magnetic field of about 0.06 G is applied to separate the state of |F = 4, mF = 0>. The clock transition |F = 4, mF = 0>→|F = 3, mF = 0 > will occurs under the excitation of the microwave. To obtain as much atomic fluorescence as possible, the detecting laser frequency will be locked to |F = 3>→|F’ = 2 > cycling transition, as shown in Figure 4C. It is worth noting that the locked frequency of the pumping and detecting lasers with 852 nm used here are different from the conventional optically pumped cesium beam frequency standards.
The beam flux of those Cs atoms with the state of |mF = 0> in the detecting region determines the signal intensity and the maximum SNR of OPCBT. The atomic beam flux in the detecting region of the OPCBT without HMF at temperatures from 40 to 130°C are calculated and shown together with the measured signal intensity of the OPCBT without HMF at temperatures from 70 to 130°C in Figure 5. It can be seen that the trend of signal intensity with oven temperature is consistent with that of atomic beam flux. Therefore, calculating the atomic beam flux in the detecting region can intuitively reflect the improvement effect of HMF on the OPCBT. We have developed a dedicated code to simulate the atomic trajectories and optimize various parameters such as the magnetic induction B at the pole cone end, the length L and the bore radius R of the hexa-pole magnets. Taking the maximum number of atoms that can reach the detecting region per second as the goal, the influence of various parameters was analyzed. Simulation model, magnet structure, magnetic field distributions, atomic trajectories, and parameter optimization processes were described in detail in our other paper [16]. Briefly, on the basis of fully considering the space size inside the existing Cs beam tube, a set of optimized parameters, L = 20mm, R = 3.2mm, B = 2.6T, was obtained. The materials of the magnets are Sm2Co17, and the materials of the magnetic poles are DT8A iron.
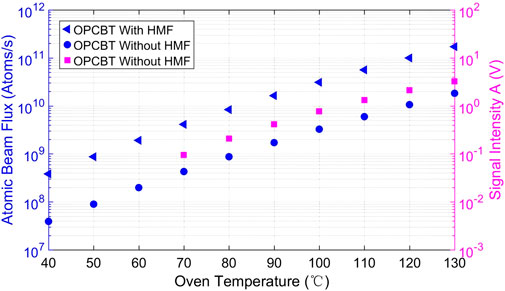
FIGURE 5. The calculated atomic beam flux of |mF = 0> in OPCBT with HMF (blue triangles) and OPCBT without HMF (blue circles). The measured signal intensity of OPCBT without HMF (purple squares).
The calculated atomic beam flux in the detecting region of the OPCBT with HMF at temperatures from 40 to 130°C are also shown in Figure 5. According to the calculation results, the same beam flux can be achieved at about 70°C in the new design, compared with 100°C in the current tube without HMF. Combined with the test results of Figure 2, the line width is expected to be narrowed to about 500 Hz, and the life expectancy will reach 20 years without changing the amount of cesium loaded in the oven. At the same operating temperature of 100°C, the signal intensity of the new tube with HMF will be about 9.5 times higher than that of the first generation tube. And when the laser by MTS is used for pumping and detecting on the new tube, the expected Allan deviation of the new compact clock will reach
In order to directly measure the increase in atomic utilization, an experiment is designed with a HMF system outside the vacuum shell of a conventional slightly longer OPCBT. And the test experiments will be carried out after the assembled tube is finished soon.
Discussion
Although the HMF has brought about an improvement in the utilization of atoms, its strong magnetic field may destroy the uniformity of the C field located in the microwave cavity region, which is used to separate the |F = 4, mF = 0 > magnetic sublevels. The OPCBT shown in Figure 2 has installed two layers of permalloy magnetic shields around the microwave cavity, but it can only ensure the uniformity of the C field when the ambient magnetic field is not greater than 2 Gauss. Combining the feasible scheme for shielding strong magnetic fields in the dipole magnetic state-selected cesium beam tube, a magnetic shield using industrial pure iron is designed to reduce the leakage of the hexapole magnetic field. In practice, other surrounding materials may interfere with the effect of shielding, which requires attention in experiments.
Scattered light entering the microwave cavity will cause frequency shift, which has always been a challenge for the optically pumped cesium beam atomic clocks [23]. The HMF system separates the laser pumping region and the microwave cavity, which can reduce the light frequency shift to a certain extent. The specific value of light frequency shift will be evaluated in the follow-up experimental.
In addition, calculations show that atoms with an emission angle of less than 3.95° will be detected, compared to those with a much smaller angle of 0.92° without the hexapole magnetic system. A significant increase in atomic utilization means fewer stray atoms, which will help reduce the noise of the Ramsey pattern.
If necessary, increasing the oven temperature to 130°C can achieve the expected frequency stability of
Conclusion
In summary, we first implement a commercial optically pumped cesium beam tube without HMF. Tested with the high-stability laser by MTS, the SNR of 18,000 in 1 Hz bandwidth were obtained at the typical operating temperature of 100 C. As the oven temperature rises from 70 to 130°C, the variation laws of the signal amplitude, the SNR in 1 Hz bandwidth, the linewidth W and the figure of merit F are tested and analyzed. It can be seen that constantly increasing the temperature does not keep the performance rising, but makes the life drop rapidly. By comparing with a Hydrogen maser, the Allan deviation of the compact optically pumped cesium beam atomic clock based on this type of tubes is measured to be
Then we proposed a design scheme of OPCBT based on HMF. A dedicated code is programed to simulate the atomic trajectories. Calculations show that the atomic utilization of OPCBT with HMF can be increased by about 9.5 times. This tube can be operated in ultra-long life or ultra-high-performance mode. When the oven temperature is operated at about 70°C, the life expectancy can reach more than 20 years with an Allan deviation of
HMF-based OPCBT and MTS-based high-stability laser can greatly improve the performance and lifetime of cesium clocks. The performance of the new compact optically pumped cesium beam atomic clock will be close to that of Hydrogen maser while maintaining a small volume, which will promote the fields such as metrology, timekeeping and space.
Data availability statement
The original contributions presented in the study are included in the article/Supplementary Material, further inquiries can be directed to the corresponding author.
Author contributions
HC: Conceptualization, Methodology,Validation, Formal Analysis, Writing—Original Draft; YY: Data Curation, Software,Visualization, Validation JC: Conceptualization, Writing—Review and Editing JF: Conceptualization, Writing—Review and Editing, Project Administration, Resources, Supervision.
Acknowledgments
We express our most sincere wishes on the occasion of Professor Prof. Yiqiu Wang’s 90th birthday. The authors would like to thank Prof. Wang for his selfless help in the development of the optically pumped cesium beam tube. As early as the 1980s, Prof. Wang carried out theoretical and experimental research on the optically pumped cesium beam frequency standard, which laid a solid foundation for our later work. HC sincerely thanks Prof. Wang for many useful discussions on the realization of the optically pumped cesium beam tube based on hexapole magnetic focusing. In order to answer our questions in a timely manner, he often worked late into the night, and we sometimes even received emails from him at 2 a.m. Prof. Wang’s rigorous attitude to scientific investigation and diligence in his work motivate us to keep moving forward.
Conflict of interest
The authors declare that the research was conducted in the absence of any commercial or financial relationships that could be construed as a potential conflict of interest.
Publisher’s note
All claims expressed in this article are solely those of the authors and do not necessarily represent those of their affiliated organizations, or those of the publisher, the editors and the reviewers. Any product that may be evaluated in this article, or claim that may be made by its manufacturer, is not guaranteed or endorsed by the publisher.
References
1. Vanier J, Audoin C. The quantum Physics of atomic frequency standards. Bristol: Adam Hilger (1989).
2. Audoin C, Dimarcq N, Giodano V, Viennet J. Physical origin of the frequency shifts in cesium beam frequency standards: Related environmental sensitivity. IEEE Trans Ultrason Ferroelectr Freq Control (1992) 39:412–21. doi:10.1109/58.143175
3. Audoin C, Vanier J. Atomic frequency standards and clocks. J Phys E (1976) 9:697–720. doi:10.1088/0022-3735/9/9/001
4. Vanier J, Audoin C. The classical caesium beam frequency standard: Fifty years later. Metrologia (2005) 42:S31. doi:10.1088/0026-1394/42/3/s05
5. Marlow BLS, Scherer DR. A review of commercial and emerging atomic frequency standards. IEEE Trans Ultrason Ferroelectr Freq Control (2021) 68:2007–22. doi:10.1109/tuffc.2021.3049713
6. Becker G. Recent progress in primary Cs beam frequency standards at the PTB. IEEE Trans Instrum Meas (1976) 25:458–65. doi:10.1109/tim.1976.6312264
7. DeMarchi A, Shirley J, Glaze DJ, Drullinger RE. A new cavity configuration for cesium beam primary frequency standards. IEEE Trans Instrum Meas (1988) 37:185–90. doi:10.1109/19.6049
8. Drullinger RE, Glaze DJ, Lowe L, Shirley JH. The NIST optically pumped cesium frequency standard. IEEE Trans Instrum Meas (1991) 40:162–4. doi:10.1109/tim.1990.1032906
9. Drullinger RE, Lowe JP, Glaze DJ, Shirley JH. NIST-7, the new US primary frequency standard. Proc IEEE Int Freq Control Symp (1993) 71.
10. Drullinger RE, Shirley JH, Lowe JP, Glaze DJ. Error analysis of the NIST optically pumped primary frequency standard. IEEE Trans Instrum Meas (1993) 42:453–6. doi:10.1109/19.278603
11. Lee WD, Shirley JH, Lowe JP, Drullinger RE. The accuracy evaluation of NIST-7. IEEE Trans Instrum Meas (1995) 44:120–3. doi:10.1109/19.377788
12. Huang LY, Chen J, Wang J, Zhang DX. Influence of rod tuner position on resonance frequency of Ramsey cavity in compact cesium atom clock. In: International Conference on Microwave and Millimeter Wave Technology (ICMMT) (2012). p. 1.
13. Mueller LF, Dull DB, Kusters JA. A new high-performance cesium beam tube compensated for Ramsey pulling. In: Proceedings of the 45th Annual Symposium on Frequency Control (1991). p. 554.
14. Hyatt RC, Mueller LF, Osterdock TN. A high-performance beam tube for cesium beam frequency standards. Hewlett Packard J (1973) 14:14.
15. Qu LL, Li B, Gao Z. Research on the relation between frequency stability and timekeeping clock allocation. In: 12th IEEE Int. Conf. Electron. Meas. Instrum. (ICEMI) (2015). p. 888.
16. Yan YF, Chen HJ, Pan D, Liu TY, Chen JB, Feng JJ. Enhancing the signal-to-noise ratio in optically pumped cesium beam tubes using a hexapole magnetic system. In: 2021 Joint Conference of the European Frequency and Time Forum and IEEE International Frequency Control Symposium (EFTF-IFCS) (2021). p. 1.
17. Liu TY, Pan D, Chen JB. Optical pumped cesium atomic clock with multi-pole magnet. In: 2020 Joint Conference of the IEEE International Frequency Control Symposium and International Symposium on Applications of Ferroelectrics (IFCS-ISAF) (2020). p. 1.
18. Shang HS, Zhang TY, Miao JX, Shi TT, Pan D, Zhao XW, et al. Laser with 10–13 short-term instability for compact optically pumped cesium beam atomic clock. Opt Express (2020) 28:6868. doi:10.1364/oe.381147
19. Cao Y, Zhao X, Xie W, Wei Q, Yang L, Chen H, Zhang S. A merchandized optically pumped cesium atomic clock. In: Proceedings of Joint European Frequency and Time Forum and International Frequency Control Symposium (2017). p. 618–21.
20. Emons DA, Rogers PJ. New cesium beam tube utilizing hexapole/double-dipole optics. In: 33rd Annual Symposium on Frequency Control (1979). p. 490.
21. Dorenwendt K. Development and performance of the PTB's CS1 primary clock. Proc IEEE (1986) 74:137–40. doi:10.1109/proc.1986.13421
22. Bauch A, Dorenwendt K, Fischer B, Heindorff T, Müller EK, Schröder R. CS2: The PTB's new primary clock. IEEE Trans Instrum Meas (1987) 36:613–6. doi:10.1109/tim.1987.6312751
Keywords: compact atomic clock, optically pumped, cesium beam tube, hexapole magnetic focusing, long lifetime, signal-to-noise ratio
Citation: Chen H, Yan Y, Chen J and Feng J (2022) Design of optically pumped cesium beam tube with hexapole magnetic system for longer lifetime and better SNR. Front. Phys. 10:956719. doi: 10.3389/fphy.2022.956719
Received: 30 May 2022; Accepted: 29 July 2022;
Published: 24 August 2022.
Edited by:
Hendrick L. Bethlem, VU Amsterdam, NetherlandsReviewed by:
Bin Jian, National Research Council Canada (NRC-CNRC), CanadaBen Edward Sauer, Imperial College London, United Kingdom
Copyright © 2022 Chen, Yan, Chen and Feng. This is an open-access article distributed under the terms of the Creative Commons Attribution License (CC BY). The use, distribution or reproduction in other forums is permitted, provided the original author(s) and the copyright owner(s) are credited and that the original publication in this journal is cited, in accordance with accepted academic practice. No use, distribution or reproduction is permitted which does not comply with these terms.
*Correspondence: Jinjun Feng, fengjinjun@tsinghua.org.cn