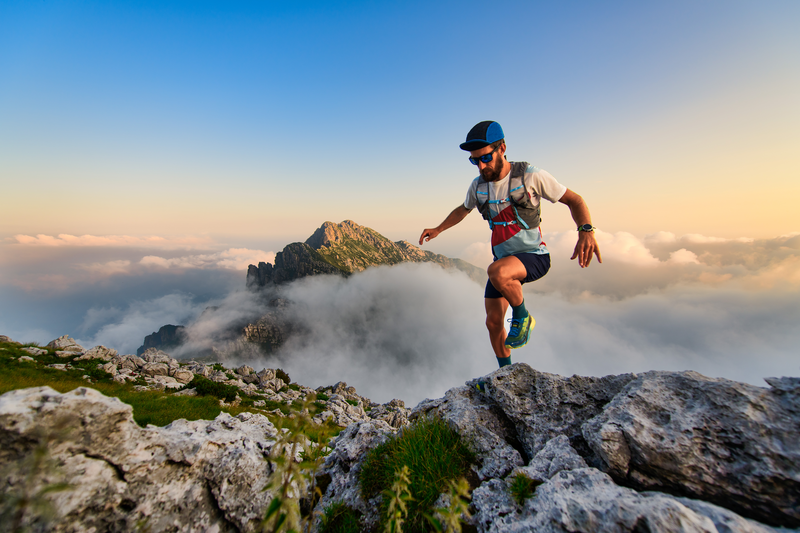
95% of researchers rate our articles as excellent or good
Learn more about the work of our research integrity team to safeguard the quality of each article we publish.
Find out more
EDITORIAL article
Front. Phys. , 04 July 2022
Sec. Medical Physics and Imaging
Volume 10 - 2022 | https://doi.org/10.3389/fphy.2022.950928
This article is part of the Research Topic Capturing biological complexity and heterogeneity using multidimensional MRI View all 9 articles
Editorial on the Research Topic
Capturing Biological Complexity and Heterogeneity Using Multidimensional MRI
In the context of medical imaging, the term “resolution” most often refers to the measure of the smallest object that can be resolved from the image, i.e., spatial resolution. The two most prominent modalities that offer comparable spatial resolution on the order of 1 mm3 in clinical applications, computed tomography (CT) and magnetic resonance imaging (MRI), provide the ability to noninvasively probe biological tissue in depth and in vivo. While important macroscopic information resides at the 1 mm length scale, such as white and gray matter discrimination or visible lesions identification, some tissue level and definitely cell-level processes remain elusive.
Despite its limited spatial resolution, MRI and more precisely nuclear magnetic resonance (NMR) is a spectroscopic modality, and therefore its spectral resolution is a second, more unique type of resolution that is leveraged in MRI applications. The idea to jointly encode more than a single MR parameter (e.g., relaxation, diffusion), or so-called dimension, was first proposed more than 4 decades ago [1], and was repeatedly shown to enhance the spectral resolution in a range of complex systems, from biological tissue [2, 3] to sedimentary rocks [4]. Spectral resolution in the current context should not be confused with the more common use of the term in Fourier transform NMR spectroscopy with multidimensional correlation of, for instance, 1H and 15N chemical shifts. In biological applications, relaxation-diffusion multidimensional MR provides information from compositionally- and microstructurally-diverse environments, thus offering a probe of tissue heterogeneity. More recently, the use of multidimensional MRI to capture sub-voxel information has been gaining attention, leading to increased specificity and sensitivity towards a range of biological states and diseases [5–9].
The goal of this Research Topic was to give a platform to introduce novel and cutting-edge methods that utilize MRI as a multidimensional tool, and to advance the field towards more refined, sensitive, and specific imaging. The papers here focused on taking advantage of untapped dimensions to increase the spectral resolution, and as a result, to increase the effective spatial resolution by mapping sub-voxel components that describe physical and chemical properties of the different microenvironments in complex and heterogeneous biological materials.
The first few papers in this Research Topic are focused on introducing new frameworks to process multidimensional diffusion data. Magdoom et al. explore a novel diffusion tensor distribution (DTD) paradigm exploiting its ability to probe features below MRI voxel sizes to study neural tissue. Results in ex vivo spinal cord tissue of macaque monkeys showed its ability to detect the boundaries of cortical layers in the higher moments of the mean diffusivity (MD) spectrum consistent with histology, revealed crossing and splaying fibres penetrating into the cortex, and skewed fiber diameter distributions in the white matter tracts. Different diffusion encoding properties span a new multidimensional parameter space allowing to detect hidden tissue properties. Song et al. present a new approach to estimate the full DTD (FDTD) without any constraints on the functional form of the distribution and/or the symmetries of the tensor components, based on data acquired with multi-shell single diffusion encoding data up to very high diffusion weighting (300 mT/m). They show their approach for data acquired on the Connectom scanner for one volunteer and two patients with diffuse gliomas. They introduce the use of a k-means algorithm to cluster the FDTD data, and demonstrate the potential utility for characterization of tissue types in gliomas. By incorporating a more realistic diffusion signal model, Boito et al. introduce the confinement tensor distribution (CTD) as an augmented approach of the previously suggested DTD for analyzing restricted and anisotropic diffusion in biological tissues. In their work, they consider a model that takes restriction into account, thus replacing the multi-exponential signal representation inherent to DTD. They introduce expanded theory, extensive numerical simulations, and then apply the CTD framework on a human brain dataset, providing new insights regarding the structural composition of complex media. Shifting the focus to exchange, Cai et al. propose an acquisition scheme to independently characterize restriction and exchange with various diffusion exchange spectroscopy (DEXSY) measurements. Results in a viable ex vivo mouse spinal cord tissue using a low-field static NMR system demonstrate the newly disentangled effects of restriction and exchange. Combining multiple diffusion encoding paradigms with exchange mechanisms opens a different multidimensional space, allowing to separate normally confounded properties.
The two next papers incorporate relaxation to the diffusion encoding scheme and introduce new processing pipelines. Narvaez et al. join together diffusion-relaxation correlation, time/frequency-dependent diffusion, and tensor-valued encoding into a common data acquisition and analysis framework. They leverage the inherent frequency dependence of the diffusion gradients waveforms to resolve a “massively multidimensional” diffusion-relaxation correlation dataset in ex vivo rat brain, with subsequent histological analyses and comparisons, and demonstrate unprecedented level of detail within the brain. Using a different approach and pulse sequence, Benjamini et al. propose applying an adaptive nonlocal multispectral filter denoising procedure to increase the effective signal-to-noise ratio of multidimensional MRI data, achieving significant reduction in data requirement and subsequent acquisition acceleration. They demonstrate this approach by reprocessing data from a recent study that identified imaging biomarkers of subtle axonal injury pathology in ex vivo human Corpus Callosum samples using diffusion-relaxation MRI. The obtained results show improved accuracy of the reconstructed injury MRI biomarker maps, while reducing the required data amount.
With a departure from the central nervous system, Lasič et al. present a novel prediction framework for time-dependent diffusion effects using b-tensor encoding. Experimental data from ex vivo mouse and pig hearts and the resulting strong and predictable variation of MD time-dependent diffusion paves the way for future in vivo cardiac experiments. This is a great illustration of how multidimensional MRI–here different shapes of the b-tensor in combination with time-dependence–captures otherwise not accessible properties of biological tissue. Lastly, Afzali et al. provide a review focusing on applications of tensor valued encoding, a prominent diffusion MRI technique, in the brain and in several organs in the body including the heart, breast, kidney and prostate, to infer tissue microstructural information that is not accessible using traditional diffusion MRI techniques. The application of this technique to various diseases and conditions is described. The review also provides a brief description of the different diffusion encoding schemes and technical considerations, with an exhaustive list of corresponding literature.
In conclusion, this Research Topic illustrates the incredible versatility and untapped potential of MR in gleaning information from heterogeneous biological systems. While it is becoming increasingly clear that no single MR contrast mechanism is able to grasp the full complexity of our biology, the concept of jointly encoding several MR contrasts and thus shedding light on different tissue properties simultaneously is especially enticing. We hope that the Research Topic of works will serve as a catalyst for more wide-spread use of multidimensional MRI.
DB drafted the first version of the editorial. MB and JH revised the first draft and made contributions about papers they edited.
The authors declare that the research was conducted in the absence of any commercial or financial relationships that could be construed as a potential conflict of interest.
All claims expressed in this article are solely those of the authors and do not necessarily represent those of their affiliated organizations, or those of the publisher, the editors and the reviewers. Any product that may be evaluated in this article, or claim that may be made by its manufacturer, is not guaranteed or endorsed by the publisher.
1. Peemoeller H, Pintar MM. Two-dimensional Time-Evolution Approach for Resolving a Composite Free-Induction Decay. J Magn Reson (1969) (1980) 41:358–60. doi:10.1016/0022-2364(80)90085-2
2. English AE, Whittall KP, Joy MLG, Henkelman RM. Quantitative Two-Dimensional Time Correlation Relaxometry. Magn Reson Med (1991) 22:425–34. doi:10.1002/mrm.1910220250
3. Does MD, Gore JC. Compartmental Study ofT1 andT2 in Rat Brain and Trigeminal Nerve In Vivo. Magn Reson Med (2002) 47:274–83. doi:10.1002/mrm.10060
4. Hürlimann MD, Flaum M, Venkataramanan L, Flaum C, Freedman R, Hirasaki GJ. Diffusion-relaxation Distribution Functions of Sedimentary Rocks in Different Saturation States. Magn Reson Imaging (2003) 21:305–10. doi:10.1016/s0730-725x(03)00159-0
5. Slator PJ, Hutter J, Palombo M, Jackson LH, Ho A, Panagiotaki E, et al. Combined Diffusion-Relaxometry MRI to Identify Dysfunction in the Human Placenta. Magn Reson Med (2019) 82:95–106. doi:10.1002/mrm.27733
6. Benjamini D, Hutchinson EB, Komlosh ME, Comrie CJ, Schwerin SC, Zhang G, et al. Direct and Specific Assessment of Axonal Injury and Spinal Cord Microenvironments Using Diffusion Correlation Imaging. NeuroImage (2020) 221:117195. doi:10.1016/j.neuroimage.2020.117195
7. de Almeida Martins JP, Tax CMW, Reymbaut A, Szczepankiewicz F, Chamberland M, Jones DK, et al. Computing and Visualising Intra-voxel Orientation-specific Relaxation–Diffusion Features in the Human Brain. Hum Brain Mapp (2021) 42:310–28. doi:10.1002/hbm.25224
8. Slator PJ, Palombo M, Miller KL, Westin C, Laun F, Kim D, et al. Combined Diffusion-Relaxometry Microstructure Imaging: Current Status and Future Prospects. Magn Reson Med (2021) 86(6):2987–3011. doi:10.1002/mrm.28963
Keywords: multidimensional, MRI, diffusion, relaxation, medical imaging, microstructure, exchange
Citation: Bouhrara M, Hutter J and Benjamini D (2022) Editorial: Capturing Biological Complexity and Heterogeneity Using Multidimensional MRI. Front. Phys. 10:950928. doi: 10.3389/fphy.2022.950928
Received: 23 May 2022; Accepted: 13 June 2022;
Published: 04 July 2022.
Edited by:
Ewald Moser, Medical University of Vienna, AustriaReviewed by:
Daniel Topgaard, Lund University, SwedenCopyright © 2022 Bouhrara, Hutter and Benjamini. This is an open-access article distributed under the terms of the Creative Commons Attribution License (CC BY). The use, distribution or reproduction in other forums is permitted, provided the original author(s) and the copyright owner(s) are credited and that the original publication in this journal is cited, in accordance with accepted academic practice. No use, distribution or reproduction is permitted which does not comply with these terms.
*Correspondence: Dan Benjamini, ZGFuLmJlbmphbWluaUBuaWguZ292
Disclaimer: All claims expressed in this article are solely those of the authors and do not necessarily represent those of their affiliated organizations, or those of the publisher, the editors and the reviewers. Any product that may be evaluated in this article or claim that may be made by its manufacturer is not guaranteed or endorsed by the publisher.
Research integrity at Frontiers
Learn more about the work of our research integrity team to safeguard the quality of each article we publish.