- Research Institute for Electromagnetic Materials DENJIKEN, Tomiya, Japan
Magneto-optical Faraday devices, such as optical isolators, require a structure to apply a magnetic field to the incorporated magneto-optical material. The application of a magnetic field hinders the miniaturization and integration of these devices. Nanogranular films show Faraday rotation angles up to 40 times larger than Bi-YIG in the optical communication band (1,550 nm). Nanogranular films are submicron-to several-micrometer-thick and contribute to the miniaturization and integration of optical devices. Here, we introduce (Co-Pt)–(CaF2) nanogranular films exhibiting a magnetic-field-free magneto-optical Faraday effect, which are Co3Pt alloy that are hard magnets with residual magnetization. These nanogranular films exhibit the Faraday effect without requiring a magnetic field owing to their residual magnetization.
Introduction
The magneto-optical Faraday effect is used in optical communication devices such as optical isolators [1–3], and new devices are required for future highly information-oriented societies. Thin-film materials with Faraday effects are required for the miniaturization and integration of these devices, and nanogranular films have been shown to have a Faraday effect that is up to 40 times larger than that of Bi-YIG [4, 5] in the optical communication band wavelength [6].
Nanogranular films have a nanometer-sized structure in which magnetic metal nanogranules with diameters of several nanometers are dispersed in a dielectric matrix and exhibit unique functions based on the quantum effects on their structure [7–11]. It should be noted that the nanogranular film, which has a small amount of magnetic metal, becomes a transparent magnetic material with a large Faraday effect [12–14]. Furthermore, nanogranular films have practical advantages such as ease of production, good reproducibility, and good heat resistance [15, 16].
On the other hand, existing magneto-optical materials (thin films with high figure of merit [17]) do not have a large coercive force and require an external magnetic field to stabilize their magnetization state. Therefore, magneto-optical devices require a magnetic field generation mechanism, and hence, incorporating them to a device makes it difficult to miniaturize and simplify the device.
In nanogranular films, various combinations of granul and matrix materials are possible. We have reported that the refractive index of nanogranular films can be controlled independently of the Faraday rotation angle by selecting the matrix material [13]. The magnetic properties of the films may also be controlled by selecting the magnetic material of the nanogranules. Herein, we report the Faraday effect of (Co-Pt)–(CaF2) nanogranular films. Co-Pt [18–21] alloys have high coercivity and residual magnetization. CaF2 with high transmittances were selected as dielectric matrices. By adopting a granule with magnetic hysteresis, coercivity, and residual magnetization, the Faraday effect can be achieved without applying a magnetic field owing to the residual magnetization of the material.
Methods
Thin films were prepared using tandem deposition [22] with DC target facing sputtering and conventional RF-sputtering sources (Figure 1). DC-facing sputtering can reduce the plasma damage during film deposition. The films were 0.3- to 1.0-μm thick. Sputter deposition was performed on a 50 × 50 mm glass substrate (synthetic fused silica) at 300 °C in an argon atmosphere with a pressure of 1.3 Pa during deposition using 76 mm-diameter Co. disk with 5 × 5 mm chips as the metal target and a 76 mm diameter CaF2 powder compacted disk as the dielectric ceramic target. These films were annealed under vacuum at a predetermined temperature of 400–800°C for 1 h.
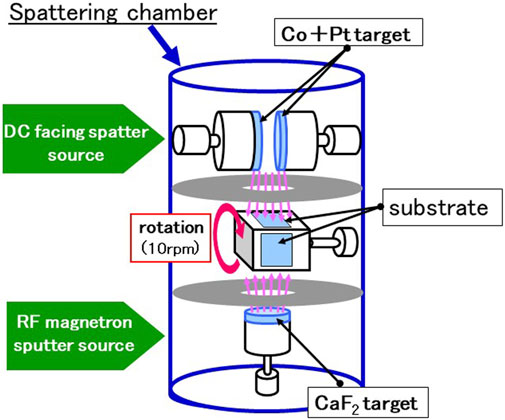
FIGURE 1. Schematic illustration of the tandem sputtering method with DC facing target sputtering and conventional RF-sputtering sources. (Co. + Pt) target was placed on the DC sputter source, and CaF2 the target was placed on the RF sputter source. The substrate holder rotates alternately towards the DC-sputtering and RF-sputtering sources.
The composition ratio of Co–Pt (granule) and CaF2 (matrix) was controlled by changing the electric power applied to each target. The chemical compositions of Co., Pt, Ca, and F in the thin films were analyzed using wavelength dispersion spectroscopy (WDS). X-ray diffraction (XRD) was used for the structural analysis of the film.
The magnetization curves were measured using a vibrating sample magnetometer (VSM). A spectrophotometer was used to measure the optical transmittance with a measurement waveband of 400–2000 nm. The Faraday rotation angle was measured in the wavelength range of 500–1700 nm using a BH-501F-SV1-DJK manufactured by NEOARC corporation. A magnetic field was applied from 0 to ±10 kOe perpendicular to the film surface of the samples to measure the magnetization curves.
All measurements were performed at room temperature.
Results and discussion
Figure 2A shows the Faraday loops of the annealed (Co-Pt)–(CaF2) (Co. + Pt = 23at. %) film at the wavelength of 1,550 nm. The Faraday loops of the annealed films exhibit magnetic hysteresis with coercivity and residual magnetizations, and exhibit a Faraday rotation angle of 0.36 deg./μm with no magnetic field at an annealing temperature of 600°C. As shown in the XRD results (Figure 2B), the Co3Pt ordered phase appears after annealing, and the films have a microstructure consisting of Co3Pt and CaF2. The diffraction peaks of Co3Pt and CaF2 sharpen with increasing annealing temperature, and at 800°C, peaks of the Co3Pt ordered phase were observed. The particle size of the Co3Pt granules in the film, determined from the width at half maximum of the XRD peaks using Scherrer equation [23], are as deposit state: 5.8 nm, T = 400°C: 6.2 nm, T = 600°C: 9.5 nm and T = 800°C: 39.8 nm, respectively. The ordering temperature of the Co3Pt alloy is approximately 600°C [19]. With an increase in the annealing temperature, Co3Pt granule growth and ordering occurred.
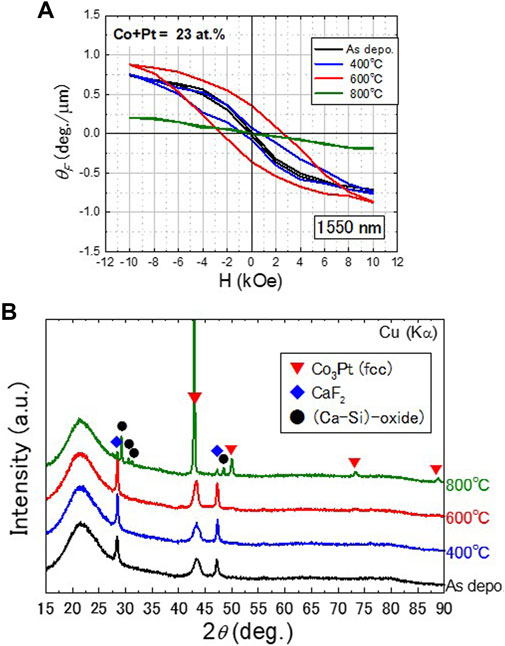
FIGURE 2. Faraday effect and structure of (Co0.8Pt0.2)23Ca23F54 (Co + Pt = 23 at%) nanogranular film. (A) Faraday loops at various annealing temperatures. (B) XRD patterns with different annealing temperatures.
The effect of annealing on the Faraday effect and coercivity of the (Co-Pt)–(CaF2) films are shown in Figure 3. The results for films with different amounts of Co. + Pt are shown (Co. + Pt = 13, 18, 23 at%). The Faraday rotation angles with a magnetic field (10 kOe) had negative values, and their absolute values increased as the amount of Co. + Pt increased (Figure 3A). The Faraday rotation angles without a magnetic field increased sharply at temperatures above 400°C, peaked at 600°C, and decreased at 800°C (Figure 3B). The annealing temperature dependence of the Faraday rotation angle without a magnetic field closely matched that of the coercivity (Hc) in Figure 3C. The Magnetic hysteresis curves of (Co-Pt)-(CaF2) films are shown in Figure 4. Hc was obtained from Figure 4. VSM measurements with a magnetic field applied parallel to the film surface. When the VSM is measured by applying a magnetic field perpendicular to the film surface, the magnetic loop is the same as that parallel to the film surface. Therefore, these films do not exhibit magnetic anisotropy.
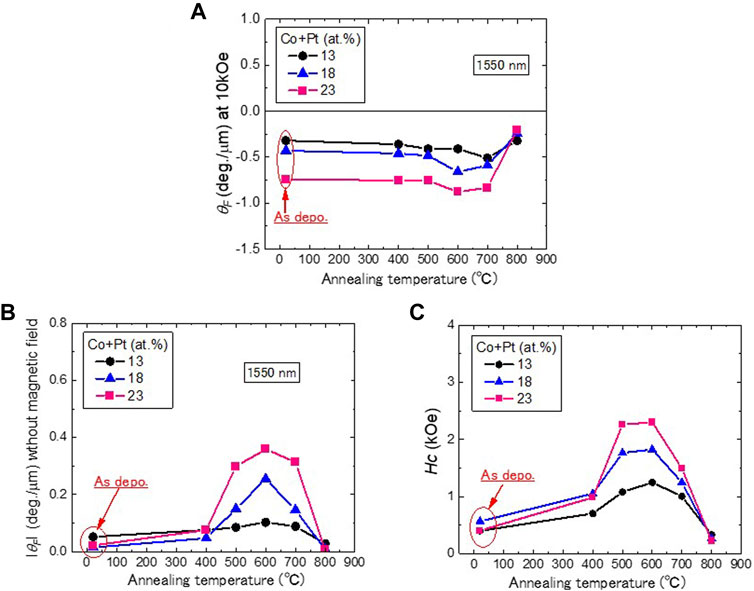
FIGURE 3. Annealing temperature dependence of the magnetic properties in (Co-Pt)-(CaF2) (Co. + Pt = 13,18 and 23 at%) nanogranular films. (A) Relationship between the Faraday rotation angle at 10 kOe and annealing temperature (1,550 nm). (B) Relationship between the Faraday rotation angle without a magnetic field and annealing temperature. (C) Annealing temperature dependence of coercivity (Hc).
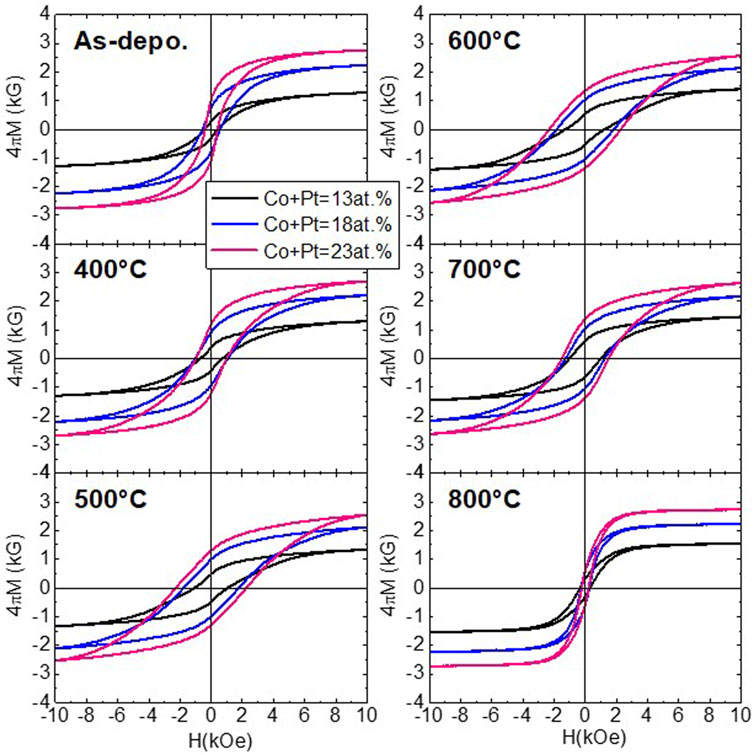
FIGURE 4. Magnetic hysteresis loops in (Co-Pt)-(CaF2) (Co. + Pt = 13,18 and 23 at%) nanogranular films as deposited state and annealed at 400, 500, 600, 700 and 800°C.
The effect of annealing on the transmittance of the (Co-Pt)-(CaF2) films is shown in Figure 5. The transmittance of the (Co-Pt)-(CaF2) film increased at all the measurement wavelengths after annealing, except at 800°C (Figure 5A). Reflectance was not changed by annealing but increased at 800°C.
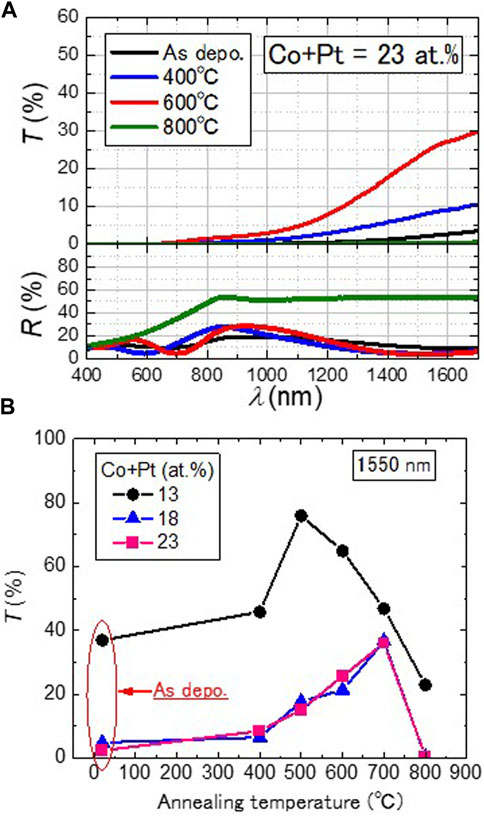
FIGURE 5. Effect of annealing on light transmittance (1 μm thickness). (A) Wavelength dependence of the transmittance of (Co-Pt)-(CaF2) (Co. + Pt = 13, 18 and 23) nanogranular films at various annealing temperatures. (B) Relationship between transmittance of (Co-Pt)-(CaF2) (Co. + Pt = 13, 18 and 23 at%) nanogranular films at 1,550 nm.
The transmittance of the (Co-Pt)-(CaF2) films was maximized at an annealing temperature of 500°C (Co. + Pt = 13 at%) and 700°C (Co. + Pt = 18, 23 at%), respectively (Figure 5B). The temperatures at which, the transmittance peaks, are in the temperature range where the Faraday rotation angle without a magnetic field increases, as shown in Figure 3B.
The results in Figures 3, 5 can be attributed to the changes in the microstructure of the films by annealing (see Figure 2B). With an increase in the annealing temperature, Co3Pt granules growth and ordering occurred. The increase in coercivity at approximately 600°C is due to the ordering of the Co3Pt alloy nanogranule. Therefore, the Faraday rotation angle without a magnetic field is exhibited by the residual magnetization of magnetic hysteresis with coercivity. The sharpening of the CaF2 peak indicated that the crystallinity of the CaF2 matrix was improved, resulting in an increase in transmittance [13]. However, their transmittance is still small (absorption coefficients are 0.3 μm−1 (Co. + Pt = 13 at%, 500°C annealed) and 1.0 μm−1 (Co. + Pt = 23 at%, 700°C annealed)). It is an issue to improve the transmittance.
At 800°C, the transmittance was greatly reduced and the reflectance increased (Figure 5). This behavior can be explained by the growth and binding of the Co3Pt nanogranule in the film (Figure 2B, the Co3Pt peak intensity is greatly increased). Furthermore, the Faraday rotation angle and coercivity decreased at 800°C. This decrease is due to the diffusion of atoms at the interface between the substrate material and film. As shown in Figure 2B, the (Ca-Si)- oxide peaks appear in the film annealed at 800°C. In this experiment, the temperature of 800°C exceeded the temperature limit of the substrate.
Conclusion
We proposed magneto-optic material for the Faraday effect without a magnetic field. By selecting a magnetic alloy (Co-Pt) with high coercivity and residual magnetization for the nanogranules in the films, films that exhibit the magnetic field-free Faraday effect can be realized by residual magnetization.
On the other hand, nanogranular films contain metal (alloy) particles, making light absorption unavoidable. For nanogranular films to be widely used in optical devices, it is important to reduce the loss by structural control, such as the improvement of crystallinity.
Data availability statement
The raw data supporting the conclusion of this article will be made available by the authors, without undue reservation.
Author contributions
The experiments were carried out by NK and TI. The data was discussed by NK, KI, and K-IA. All authors contributed to the writing and editing of the paper.
Funding
This work was supported by Japan Society for the Promotion of Science (19K21959, 20K03843, 20H02468, 20H02447) and Core Research for Evolutional Science and Technology (JPMJCR19T1).
Conflict of interest
The authors declare that the research was conducted in the absence of any commercial or financial relationships that could be construed as a potential conflict of interest.
Publisher’s note
All claims expressed in this article are solely those of the authors and do not necessarily represent those of their affiliated organizations, or those of the publisher, the editors and the reviewers. Any product that may be evaluated in this article, or claim that may be made by its manufacturer, is not guaranteed or endorsed by the publisher.
References
1. Fischer G. The Faraday optical isolator. J Opt Commun (1987) 8:18–21. doi:10.1515/joc.1987.8.1.18
2. Yadlowsky JM, Deliso ME, Da Silva LV. Optical fibers and amplifiers for WDM systems. Proc IEEE (1997) 85:1765–79. doi:10.1109/5.649655
3. Nabors DC, Farinas DA, Day T, Yang TS, Gustafson KE, Byer LR. Injection locking of a 13-W cw Nd:YAG ring laser. Opt Lett (1989) 14:1189–91. doi:10.1364/ol.14.001189
4. Wittekoek S, Robertson MJ, Popma AJT, Bongers FP. Faraday rotation and optical absorption of epitaxial films of Y3-xBixFe5O12. AIP Conf Proc (1972) 10:1418–22.
5. Takeuchi S, Ito I, Mikami I, Taniguchi S. Faraday rotation and optical absorption of a single crystal of bismuth substituted gadolinium iron garnet. J Appl Phys (1973) 44:4789–90. doi:10.1063/1.1662042
6. Kobayashi N, Ikeda K, Gu B, Takahashi S, Masumoto H, Maekawa S. Giant faraday rotation in metal-fluoride nanogranular films. Sci Rep (2018) 8:4978. doi:10.1038/s41598-018-23128-5
7. Ohnuma S, Fujimori H, Mitani S, Masumoto T. High-frequency magnetic properties in metal–nonmetal granular films (invited). J Appl Phys (1996) 79:5130. doi:10.1063/1.361531
8. Fujimori H, Ohnuma S, Kobayashi N, Masumoto T. Spintronics in metal-insulator nanogranular magnetic thin films. J Magn Magn Mater (2006) 304:32–5. doi:10.1016/j.jmmm.2006.02.005
9. Ohnuma S, Kobayashi N, Fujimori H, Masumoto T. Metal-insulator type nano-granular magnetic thin films -soft magnetic properties, TMR effect and their applications-. J Phys : Conf Ser (2009) 191:012020. doi:10.1088/1742-6596/191/1/012020
10. Kobayashi N, Masumoto H, Takahashi S, Maekawa S. Giant dielectric and magnetoelectric responses in insulating nanogranular films at room temperature. Nat Commun (2014) 5:4417. doi:10.1038/ncomms5417
11. Kobayashi N, Iwasa T, Ishida K, Masumoto H. Dielectric properties and magnetoelectric effects in FeCo-MgF insulating nanogranular films. J Appl Phys (2015) 117:014101. doi:10.1063/1.4905275
12. Kobayashi N, Masumoto H, Takahashi S, Maekawa S. Optically transparent ferromagnetic nanogranular films with tunable transmittance. Sci Rep (2016) 6:34227. doi:10.1038/srep34227
13. Kobayashi N, Ikeda K, Arai KI. Giant Faraday effect of FeCo-BaF and FeCo-SiN nanogranular films. IEEJ Trans FM (2021) 141(2):123–7. doi:10.1541/ieejfms.141.123
14. Ikeda K, Kobayashi N, Arai KI. Large Faraday effect in nanogranular films with a high refractive index matrix. Opt Mater Express (2022) 12(2):403. doi:10.1364/ome.447532
15. Kobayashi N, Ohnuma S, Masumoto T, Fujimori H. Effects of substrate temperature and heat treatment on GMR properties of Co–RE–O nano-granular films. Mater Trans JIM (1998) 39:679–83. doi:10.2320/matertrans1989.39.679
16. Kobayashi N, Ohnuma S, Fujimori H, Masumoto T. TMR and thermal stability improvement of FeCo-AlF nano-granular thin films. J Jpn Inst Met Mater (2012) 76:375–9. doi:10.2320/jinstmet.76.375
17. Block AD, Dulal P, Stadler BJH, Seaton NCA. Growth parameters of fully crystallized YIG Bi:YIG and Ce:YIG films with high faraday rotations. IEEE Photon J (2014) 6:1–8. doi:10.1109/jphot.2013.2293610
18. Carcia PF. Perpendicular magnetic anisotropy in Pd/Co and Pt/Co thin‐film layered structures. J Appl Phys (1988) 63:5066–73. doi:10.1063/1.340404
19. Treves D, Jacobs JT, Sawatzky E. Platinum‐cobalt films for digital magneto‐optic recording. J Appl Phys (1975) 46:2760–5. doi:10.1063/1.321953
20. Yamasaki M, Nawate M, Honda S, Kusuda T. Magnetic properties of sputtered Co-Pt alloy films. J Magn Soc Jpn (1991) 15:213–6. doi:10.3379/jmsjmag.15.213
21. Yamada Y, Suzuki T. Origin of large perpendicular anisotropy in Co3Pt alloy thin film. J Magn Soc Jpn (1999) 23:1855–60. doi:10.3379/jmsjmag.23.1855
22. Kobayashi N, Ohnuma S, Masumoto T, Fujimori H. Tunnel-type magnetoresistance in matel-nonmetal granular filmes prepared by tandem deposition method. J Magn Soc Jpn (1999) 23:76–8. doi:10.3379/jmsjmag.23.76
Keywords: magneto-optical effect, nanogranular, thin film, faraday effect, Co-Pt alloy, Calcium fluoride (CaF2)
Citation: Kobayashi N, Iwasa T, Ikeda K and Arai K-I (2022) Faraday effect in CoPt-CaF2 nanogranular films with hard magnetic property for magnetic-field-free faraday devices. Front. Phys. 10:940370. doi: 10.3389/fphy.2022.940370
Received: 10 May 2022; Accepted: 30 August 2022;
Published: 19 September 2022.
Edited by:
Takayuki Ishibashi, Nagaoka University of Technology, JapanCopyright © 2022 Kobayashi, Iwasa, Ikeda and Arai. This is an open-access article distributed under the terms of the Creative Commons Attribution License (CC BY). The use, distribution or reproduction in other forums is permitted, provided the original author(s) and the copyright owner(s) are credited and that the original publication in this journal is cited, in accordance with accepted academic practice. No use, distribution or reproduction is permitted which does not comply with these terms.
*Correspondence: Nobukiyo Kobayashi, bi5rb2JheWFzaGlAZGVuamlrZW4ubmUuanA=