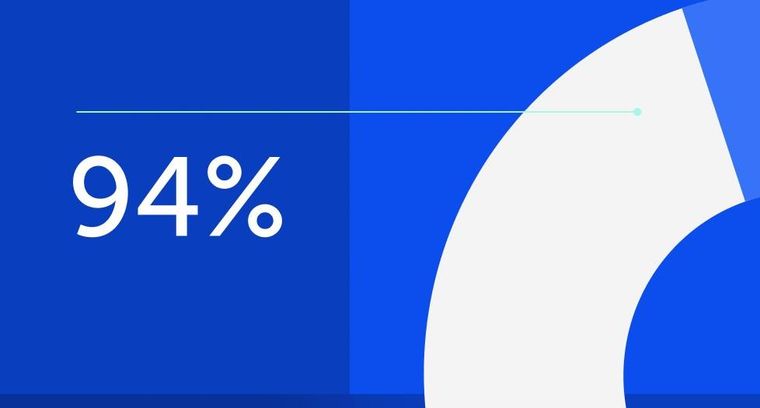
94% of researchers rate our articles as excellent or good
Learn more about the work of our research integrity team to safeguard the quality of each article we publish.
Find out more
ORIGINAL RESEARCH article
Front. Phys., 09 June 2022
Sec. Radiation Detectors and Imaging
Volume 10 - 2022 | https://doi.org/10.3389/fphy.2022.888078
This article is part of the Research TopicNovel Ideas for Accelerators, Particle Detection and Data Challenges at Future CollidersView all 17 articles
The Future Circular Collider (FCC) Integrated Project foresees, in a first stage, a high-luminosity high-energy electron-positron collider, serving as Higgs, top and electroweak factory, and, in a second stage, an energy frontier hadron collider, with a centre-of-mass energy of at least 100 TeV. This programme well matches the highest priority future requests issued by the 2020 Update of the European Strategy for Particle Physics. In 2021, with the support of the CERN Council, a five-year FCC Feasibility Study was launched. In this article, we present the FCC integrated project and the preparations for the FCC Feasibility Study.
The Future Circular Collider (FCC) shall be located in the Lake Geneva basin and linked to the existing CERN facilities [4]. The FCC “integrated programme” is inspired by the successful past Large Electron Positron collider (LEP) and Large Hadron Collider (LHC) projects at CERN. It represents a comprehensive long-term programme maximising physics opportunities. A similar project is under study in China [25, 32].
The first stage of the FCC integrated project is an e+e− collider, called FCC-ee, which would serve as Higgs factory, electroweak and top factory at highest luminosities, and run at four different centre-of-mass energies, namely on the Z pole, at the WW threshold, at the ZH production peak, and at the
The technical schedule of the FCC integrated project foresees the start of FCC tunnel construction around the year 2030 — or three years after a possible project approval —, the first e+e− collisions at the FCC-ee during the early 2040s, and the first FCC-hh hadron collisions by 2065–70 — see Figure 1. In this way, the FCC integrated project would allow for a seamless continuation of High Energy Physics (HEP) after the completion of the High Luminosity LHC (HL-LHC) physics programme.
FIGURE 1. Technical schedule of the FCC integrated project with year 1 equal to 2021 (a similar schedule was presented in Ref. [3]).
A comprehensive Conceptual Design Report (CDR) for the FCC was published in 2019 [26–28], describing the physics cases, the design of the lepton and hadron colliders, and the underpinning technologies and infrastructures. According to this design, the FCC-ee is the most sustainable of all the proposed Higgs and electroweak factory proposals, in that it implies the lowest energy consumption for a given value of total integrated luminosity [3], over the collision energy range from 90 to 365 GeV.
FCC-ee research and development (R&D) focuses on further improving the overall efficiency, on obtaining the measurement precision required, and on achieving the target performance in terms of beam current and luminosity.
Key FCC-ee R&D items for improved energy efficiency include high-efficiency continuous wave (CW) radiofequency (RF) power sources (klystrons and/or solid state), high-Q SC cavities for the 400–800 MHz range, and possible applications of high-temperature superconductor (HTS) magnets. For ultra high precision centre-of-mass energy measurements, the R&D should cover state-of-art and beyond in terms of spin-polarisation simulations and measurements (inv. Compton, beamstrahlung, etc.). Finally, for high luminosity, high current operation, FCC-ee requires a next generation beam stabilization/feedback system to suppress instabilities arising over a few turns, a robust low-impedance collimation scheme, and a machine tuning system based on artificial intelligence.
Since PETRA, TRISTAN and LEP-2, superconducting RF systems are the underpinning technology for modern circular lepton colliders. The FCC-ee baseline foresees the use of single-cell 400 MHz Nb/Cu cavities for high-current low-voltage beam operation at the Z production energy, four-cell 400 MHz Nb/Cu cavities at the W and H (ZH) energies, and a complement of five-cell bulk Nb 800 MHz cavities at 2 K for low-current high-voltage
Aside from the various RF systems, another major component of the FCC-ee is the regular arc, covering almost 80 km. The arc cells must be cost effective, reliable and easily maintainable. Therefore, as part of the FCC R&D programme it is planned to build a complete arc half-cell mock up including girder, vacuum system with antechamber and pumps, dipole, quadrupole and sextupole magnets, beam-position monitors, cooling and alignment systems, and technical infrastructure interfaces, by the year 2025.
Constructing some of the magnets for the FCC-ee final focus or arcs with advanced high-temperature superconductor (HTS) technology could lower energy consumption and increase operational flexibility. The focus of this HTS R&D will not be on reaching extremely high field, but on operating lower-field SC magnets at temperatures between 40 and 77 K.
As experience at previous and present colliders has taught us, adequate beam diagnostics is essential for reaching or exceeding design performance. For this reason, the FCC-ee R&D programme foresees the prototyping of key beam diagnostics, like bunch-by-bunch longitudinal charge-density monitors, ultra-low emittance measurements, beam-loss and beamstrahlung monitors, real time monitoring of the collision offsets, a polarimeter for each beam able to measure the 3D polarization vector as well as the beam energy, and fast luminometers.
Highly precise centre-of-mass energy calibration at c.m. energies of 91 GeV (Z pole) and 160 GeV (WW threshold), a cornerstone of the precision physics programme of the FCC-ee, relies on using resonant depolarisation of wiggler-pre-polarised pilot bunches [5]. The operation with polarised pilot bunches requires constant and high precision monitoring of the residual 3-D spin-polarization of the colliding bunches, which—if nonzero—would affect the physics measurements.
Concerning the FCC-ee pre-injector, the CDR design foresaw a pre-booster synchrotron. At present, this choice is under scrutiny. As an alternative, and possibly new baseline, it is proposed to extend the energy of the injection linac to 10–20 GeV, for direct injection into the full-energy booster. The S-band linac could be based on state-of-the-art technology as employed for the FERMI upgrade at the ELETTRA synchrotron radiation facility.
It is also envisaged to design, construct and then test with beam a novel positron source plus capture linac, and measure the achievable positron yield, at the PSI SwissFEL facility, with a primary electron energy that can be varied from 0.4 to 6 GeV.
The injection energy for the full-energy booster is defined by the field quality of its low-field magnets. Magnet development and prototyping of booster dipole magnets, along with field measurements, should guide the choice of the injection energy.
The SuperKEKB collider, presently being commissioned [19], features many of the key elements of FCC-ee: double ring, large crossing angle, low vertical IP beta function
In view of the SuperKEKB experience, studies of vertical emittance tuning is another important R&D frontier for FCC-ee. This includes simulating realistic beam measurements, constructing optics tuning knobs, especially for the final focus, and developing beam-based alignment procedures for the entire ring. Software development is an important component of this activity. Effects of beam-beam collisions and monitor resolution limits need to be considered, as should be the impact of machine errors and tuning on the dynamic aperture and on the achievable polarisation levels.
Beam studies relevant to FCC-ee—for example on optics correction, vertical emittance tuning, crab-waist collisions, or beam energy calibration—can, and will, also be conducted at INFN-LNF/DAFNE, DESY/PETRA III, and KIT/KARA [14].
The primary technology of the future circular hadron collider, FCC-hh, is the high-field magnets, Both high-field dipoles and quadrupoles [27] are required, or, possibly, combined-function magnets [12].
For constructing the accelerator magnets of the present LHC, the Tevatron, RHIC, and HERA, wires based on Nb-Ti superconductor were used. However, Nb-Ti magnets are limited to maximum fields of about 8 T, as being operated for the LHC. The HL-LHC will, for the first time in a collider, deploy some tens of dipole and quadrupole magnets with a peak field of 11–12 T, based on a new high-field magnet technology using a Nb3Sn superconductor. The Nb3Sn superconductor holds the promise to approximately double the magnetic field, from ∼8 T at the LHC, to 16 T for the FCC-hh.
Recently, several important milestones were accomplished in the development of high-field Nb3Sn magnets. At CERN, a block coil magnet, FRESCA2, with a 100 mm bore, achieved a world-record field of 14.6 T at 1.9 K [29]. In the US, a Nb3Sn cosine-theta accelerator dipole short-model demonstrator with 60 mm aperture [33], reached a similar field, of 14.5 T at 1.9 K [34].
Forces acting on the magnet coils greatly increase with the strength of the magnetic field, while, at the same time, most higher-field conductors, such as the brittle Nb3Sn, tend to be more sensitive to pressure. Therefore, force management becomes a key element in the design of future high-field magnets.
Beside the development of optimized magnet design concepts, such as “canted cosine-theta” dipoles [7], higher field can be facilitated by a higher-quality conductor. A Nb3Sn wire development programme was set up for the FCC [2]. For Nb–Ta–Zr alloys, it could be demonstrated that an internal oxidation of Zr leads to the refinement of Nb3Sn grains and, thereby, to an increase of the critical current density [6]. The phase evolution of Nb3Sn wire during heat treatment is equally under study, as part of the FCC conductor development programme in collaboration with TVEL, JASTEC, and KEK [13]. Advanced Nb3Sn wires with Artificial Pinning Centers (APCs) produced by two different teams reached the target critical current density for FCC, of 1500 A/mm2 at 16 T [1, 30], which is 50% higher than for the HL-LHC wires. The APCs allow for better performance; they decrease magnetization heat during field ramps, improve the magnet field quality at injection, and reduce the probability of flux jumps [31].
In addition to Nb3Sn wire, also high-temperature superconductors (HTS) are of interest, since they might allow for higher fields, operation at higher temperature, and, ultimately, perhaps even lower cost. In this context, the FCC conductor programme has been exploring the potential of REBCO coated conductors (CCs). In particular, the critical surfaces for the current density, Jc(T, B, θ), of coated conductors from six different manufacturers: American Superconductor Co. (US), Bruker HTS GmbH (Germany), Fujikura Ltd. (Japan), SuNAM Co. Ltd. (Korea), SuperOx ZAO (Russia) and SuperPower Inc. (US) have been studied [22].
Outside the accelerator field, HTS magnet technology could play an important role for fusion research. A number of companies are developing HTS magnets in view of fusion applications. One of these companies is Commonwealth Fusion Systems who in partnership with MIT’s Plasma Science and Fusion center are designing SPARC, a compact net fusion energy device [18]. Their magnets are based on second generation ReBCO (Rare-earth barium copper oxide) conductors. Recently they successfully demonstrated a coil with 20 T field [17]. An interesting view on HTS prospects is presented in a Snowmass 2020 Letter of Interest [16], according to which the actual material and process costs of HTS tapes are a small fraction of their current commercial value and that there is a historical link between manufactured volume and price [15].
The 2020 Update of the European Strategy for Particle Physics (ESPPU) [10] states that “An electron-positron Higgs factory is the highest-priority next collider. For the longer term, the European particle physics community has the ambition to operate a proton-proton collider at the highest achievable energy.” and “Europe, together with its international partners, should investigate the technical and financial feasibility of a future hadron collider at CERN with a centre-of-mass energy of at least 100 TeV and with an electron-positron Higgs and electroweak factory as a possible first stage. Such a feasibility study of the colliders and related infrastructure should be established as a global endeavour and be completed on the timescale of the next Strategy update.” Responding to this key request from the ESPPU, in the summer of 2021, the five-year Future Circular Collider Feasibility Study was launched [8, 9].
The 2019 FCC CDR describes the baseline FCC design with a circumference of 97.75 km, 12 surface sites, and two primary collision points. In 2021, a further design optimisation has resulted in an optimised placement of much lower risk, with a circumference of 91.2 km and only 8 surface sites, and which would be compatible with either 2 or 4 collision points. Consequently, adaptations of the CDR design and re-optimisation of the machine parameters are underway, taking into account not only the new placement, but also, for FCC-ee, the possibly larger number of interaction points, and the mitigation of complex “combined” effects, e.g. the interplay of transverse and longitudinal impedance with the beam-beam interaction. Figure 2 sketches the layouts and possible straight-section functions for both electron-positron and hadron collider.
FIGURE 2. Schematic layout of FCC-ee and its booster (A) and of FCC-hh (B) with a circumference of 91.2 km and strict four-fold superperiodicity.
Preliminary FCC-ee parameters for the cases of either two or four IPs are shown in Table 1, updated parameters for FCC-hh in Table 2. In the FCC-ee CDR [26], the operation at the Z and W assumed a 60°/60° phase advance per arc cell. The mitigation of the combined impedance and beam-beam effects requires a larger momentum compaction factor than in the CDR [23]. This has resulted in a “long” 90° cell, of twice the cell length used for the H and
TABLE 1. Preliminary key parameters of FCC-ee [20], as evolved from the CDR parameters, now with a shorter circumference of 91.2 km, and a new arc optics for Z and W running. Luminosity values are given per interaction point (IP), for scenarios with either 2 (left) or 4 IPs (right). Both the natural bunch lengths due to synchrotron radiation (SR) and their collision values including beamstrahlung (BS) are shown. The FCC-ee considers a combination of 400 MHz radiofrequency systems (at the first three energies, up to 2 × 2 GV) and 800 MHz (additional cavities for
In addition to the 4 baseline running modes on the Z pole, at the WW threshold, at the (Z) H production peak, and above the
The original contributions presented in the study are included in the article/Supplementary Material, further inquiries can be directed to the corresponding author.
MB is the FCC Study Leader, FZ his deputy. They have jointly managed the FCC conceptional design study, since 2014, and are also coordinating the new Feasibility Study. Together, they have defined the structure and contents of this article, and wrote the text.
This work was supported, in part, by the European Union’s Horizon 2020 Research and Innovation programme under grant no. 951754 (FCCIS).
The authors declare that the research was conducted in the absence of any commercial or financial relationships that could be construed as a potential conflict of interest.
All claims expressed in this article are solely those of the authors and do not necessarily represent those of their affiliated organizations, or those of the publisher, the editors and the reviewers. Any product that may be evaluated in this article, or claim that may be made by its manufacturer, is not guaranteed or endorsed by the publisher.
We extend our warm thanks to the global FCC collaboration for the results reported in this article. In particular, we wish to thank A. Ballarino, A. Blondel, M. Boscolo, G. Dissertori, M. Giovannozzi, J. Gutleber, K. Hanke, P. Janot, R. Kersevan, M. Koratzinos, R. Losito, V. Mertens, M. Migliorati, K. Oide, T. Raubenheimer, and D. Shatilov. Part of the contents of this manuscript has been presented, along with other similar proposals, at the European Physical Society Conference on High Energy Physics (EPS-HEP2021) [32].
1. Balachandran S, Tarantini C, Lee PJ, Kametani F, Su Y-F, Walker B, et al. Beneficial Influence of Hf and Zr Additions to Nb4at.%Ta on the Vortex Pinning of Nb3Sn with and without an O Source. Superconductor Sci Technology (2019) 32:044006.
2. Ballarino A, Hopkins SC, Bordini B, Richter D, Tommasini D, Bottura L, et al. The CERN FCC Conductor Development Program: A Worldwide Effort for the Future Generation of High-Field Magnets. IEEE Trans Appl Supercond (2019) 29:1–9. doi:10.1109/TASC.2019.2896469
3. Benedikt M, Blondel A, Janot P, Mangano M, Zimmermann F. Future Circular Colliders Succeeding the LHC. Nat Phys (2020) 16:402–7. doi:10.1038/s41567-020-0856-2
4. Benedikt M, Zimmermann F. The Physics and Technology of the Future Circular Collider. Nat Rev Phys (2019) 1:238–40. 3 p. doi:10.1038/s42254-019-0048-0
5. Blondel A, Janot P, Wenninger J, Abmann R, Aumon S, Azzuri P, et al. Polarization and Centre-of-Mass Energy Calibration at FCC-ee (2019). p. 107. arXiv 1909.12245. doi:10.48550/arXiv.1909.12245
6. Buta F, Bonura M, Matera D, Bovone G, Ballarino A, Hopkins SC, et al. Very High Upper Critical fields and Enhanced Critical Current Densities in Nb3Sn Superconductors Based on Nb-Ta-Zr Alloys and Internal Oxidation. J Phys Mater (2021) 4:025003. doi:10.1088/2515-7639/abe662
7. Caspi S, Borgnolutti F, Brouwer L, Cheng D, Dietderich D, Felice H, et al. Canted–Cosine–Theta Magnet (CCT)—a Concept for High Field Accelerator Magnets. IEEE Trans Appl superconductivity (2013) 24:1–4.
8. CERN Council . Main Deliverables and Timeline of the FCC Feasibility Study (2021). Restricted CERN Council - Two-Hundred-and-Third Session. CERN/SPC/1161
9. CERN Council . Organisational Structure of the FCC Feasibility Study (2021). Restricted CERN Council - Two-Hundred-and-Third Session. CERN/SPC/1155/Rev.2
10.European Strategy Group. 2020 Update of the European Strategy for Particle Physics (Brochure). CERN-ESU-015 (2020).
11. Faus-Golfe A, Valdivia Garcia M, Zimmermann F. The Challenge of Monochromatization, Direct s-Channel Higgs Production: e+e− → H. Eur Phys J Plus (2022) 137. doi:10.1140/epjp/s13360-021-02151-y
12. Giovannozzi M, Todesco E. Combined-function Optics for Circular High-Energy Hadron Colliders. Eur Phys J Plus (2022) 137. doi:10.1140/epjp/s13360-022-02583-0
13. Hopkins SC, Baskys A, Ballarino A, Lachmann J, Leineweber A. Phase Evolution during Heat Treatment of Nb3Sn Wires under Development for the FCC Study. IEEE Trans Appl Supercond (2021) 31:1–6. doi:10.1109/TASC.2021.3063675
14. Keintzel J, Benedikt M, Tomas R. Experimental Beam Tests for FCC-ee. PoS, Proc EPS-HEP2021 (2021) 6:877. doi:10.22323/1.398.0877
16. Matias V, Moeckly B. Price of 2G HTS Wire: Plenty of Room to Go Down. 2020 Snowmass Letter Of Interest. USA; CA (2020).
17.MIT. MIT News (2021). Available at: https://news.mit.edu/2021/MIT-CFS-major-advance-toward-fusion-energy-0908. (Accessed November 15, 2021).
18.MIT. SPARC (2021). Available at: https://www.psfc.mit.edu/sparc. (Accessed November 15, 2021).
19. Ohnishi Y. Status and Perspectives of the SuperKEKB Project. PoS, Proc EPS-HEP (2021) 2021:471–8. doi:10.1393/ncc/i2012-11156-2
21. Oide K, Aiba M, Aumon S, Benedikt M, Blondel A, Bogomyagkov A, et al. Design of beam optics for the future circular collider e+e− collider rings. Phys Rev Accel Beams (2016) 19:111005. doi:10.1103/PhysRevAccelBeams.19.111005
22. Senatore C, Barth C, Bonura M, Kulich M, Mondonico G. Field and Temperature Scaling of the Critical Current Density in Commercial REBCO Coated Conductors. Supercond Sci Technol (2015) 29:014002. doi:10.1088/0953-2048/29/1/014002
24. Syratchev I, Peauger F, Karpov I, Brunner O. A Superconducting Slotted Waveguide Elliptical Cavity for FCC-ee. Zenodo (2021) 031953. doi:10.5281/zenodo.5031953
25.The CEPC Study Group. CEPC Conceptual Design Report: Volume 1 - Accelerator (2018). arXiv 1809.00285.
28.The FCC Collaboration. FCC Physics Opportunities: Future Circular Collider Conceptual Design Report Volume 1. Eur Phys J C (2019) 79.
29. Willering G, Arnestad H, Bajko M, Bajas H, Bortot L, Bottura L, et al. Tests of the FRESCA2 100 Mm Bore Nb3Sn Block-Coil Magnet to a Record Field of 14.6 T. IEEE Trans Appl Supercond (2019) 29:1–6. doi:10.1109/TASC.2019.2907280
30. Xu X, Peng X, Rochester J, Sumption M, Tomsic M. Achievement of FCC Specification in Critical Current Density for Nb3Sn Superconductors with Artificial Pinning Centers (2019). arXiv preprint arXiv:1903.08121. doi:10.48550/arXiv.1903.08121
31. Xu X, Sumption M, Peng X, Collings EW. Refinement of Nb3Sn Grain Size by the Generation of ZrO2 Precipitates in Nb3Sn Wires. Appl Phys Lett (2014) 104:082602. doi:10.1063/1.4866865
32. Zimmermann F. Towards a Circular Higgs and Electroweak Factory. In: Proceedings of Science The European Physical Society Conference on High Energy Physics (EPS-HEP2021); 26–30 July 2021 (2022). Online conference, jointly organized by Universität Hamburg and the research center DESY; Copyright owned by the author(s) under the Creative Commons License CC BY-NC-ND 4.0.
33. Zlobin A, Barzi EZ, Carmichael JR, Chlachidze G, Dimarco J, Kashikhim VV, et al. First Measurements of Magnet Quench Performance and Field Quality of the 15 T Nb3Sn Dipole Demonstrator MDPCT1. In: 2019 North American Particle Accelerator Conf.(NA-PAC’19); September 2-6, 2019; Lansing, MI, USA. Geneva, Switzerland: JACoW (2019).
Keywords: hadron collider, lepton collider, future circular collider, European strategy for particle physics, Higgs factory, electroweak factory, top-quark factory, TeraZ factory
Citation: Benedikt M and Zimmermann F (2022) Future Circular Collider: Integrated Programme and Feasibility Study. Front. Phys. 10:888078. doi: 10.3389/fphy.2022.888078
Received: 02 March 2022; Accepted: 22 March 2022;
Published: 09 June 2022.
Edited by:
Alessandro Tricoli, Brookhaven National Laboratory (DOE), United StatesReviewed by:
Nadia Pastrone, Istituto Nazionale Fisica Nucleare, ItalyCopyright © 2022 Benedikt and Zimmermann. This is an open-access article distributed under the terms of the Creative Commons Attribution License (CC BY). The use, distribution or reproduction in other forums is permitted, provided the original author(s) and the copyright owner(s) are credited and that the original publication in this journal is cited, in accordance with accepted academic practice. No use, distribution or reproduction is permitted which does not comply with these terms.
*Correspondence: Michael Benedikt, bWljaGFlbC5iZW5lZGlrdEBjZXJuLmNo
Disclaimer: All claims expressed in this article are solely those of the authors and do not necessarily represent those of their affiliated organizations, or those of the publisher, the editors and the reviewers. Any product that may be evaluated in this article or claim that may be made by its manufacturer is not guaranteed or endorsed by the publisher.
Research integrity at Frontiers
Learn more about the work of our research integrity team to safeguard the quality of each article we publish.