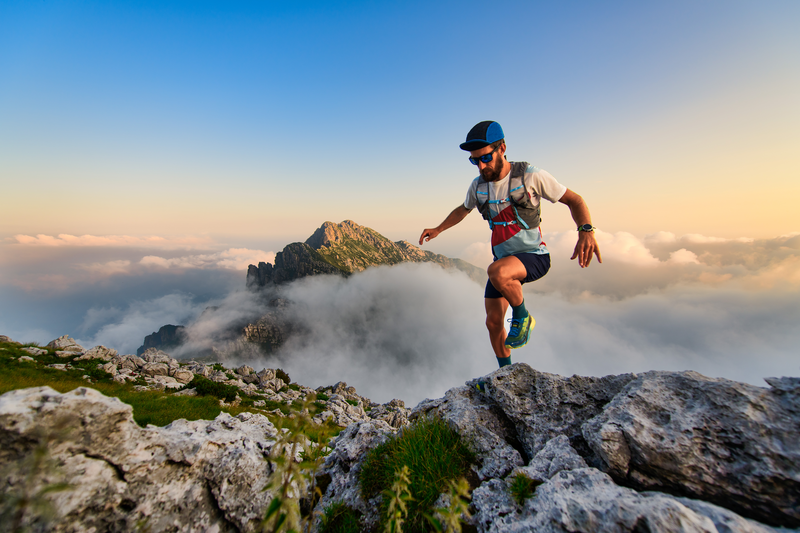
94% of researchers rate our articles as excellent or good
Learn more about the work of our research integrity team to safeguard the quality of each article we publish.
Find out more
REVIEW article
Front. Phys. , 12 May 2022
Sec. Optics and Photonics
Volume 10 - 2022 | https://doi.org/10.3389/fphy.2022.869537
This article is part of the Research Topic Application of Terahertz Frequency in Substance Detection and Recognition View all 9 articles
Recently, terahertz spectroscopy has received a lot of attention because of its unique properties such as biosafety, fingerprint spectrum, and good penetration. In this review, we focus on the research progress of terahertz spectroscopic techniques for the detection and recognition of substances. First, we describe the fundamentals of terahertz spectroscopy. Then, we outline the applications of terahertz spectroscopy in biomedicine, agriculture, food production, and security inspection. Subsequently, metamaterials, which have recently received extensive attention, are also investigated for the applications in terahertz spectroscopic detection and recognition of substances is illustrated. Finally, the development trend of terahertz spectroscopy for substance detection and recognition is also prospected.
The Terahertz (THz) band of the electromagnetic spectrum lies between microwave and far infrared, and is usually defined as electromagnetic radiation from 0.1 to 10 THz, corresponding to a wavelength range of 3 mm to 30 µm [1]. THz radiation possesses the following remarkable characteristics. 1) Fingerprint spectrum. The photon energy of a THz radiation is similar to the excitation energy of the rotational transitions in molecules, so information such as molecular vibration and rotation is contained in the terahertz spectroscopy. This is the fingerprint characteristic of terahertz spectroscopy, and it is also an important reason why it can be applied to substance detection and recognition. 2) Good Bio-safety. Compared to X-rays, THz waves have a very low photon energy of about 4 meV at 1 THz and are non-ionizing [2]. Therefore, THz waves do not cause ionization damage to samples such as biological tissues [3, 4]. 3) Coherent measurement. THz waves are usually generated by coherent laser pulses using nonlinear optical effects or dipole oscillations driven by coherent currents, so THz waves are coherent and can directly measure the amplitude and phase information of the electric field [2]. 4) High spatial and time resolution. Compared with microwaves and millimeter waves, THz waves have shorter wavelengths and can achieve higher resolution [5, 6]. THz pulses have good time resolution with pulse width on the sub-picosecond to picosecond time scale, which allows the analysis of transient changes in molecules, electrons, etc. [7, 8]. Thus, it is possible to use THz time-resolved spectroscopy in the detection and recognition of substances. 5) Good penetration. THz waves have good penetration and can penetrate general dielectric materials including plastics, clothing, and ceramics [9, 10]. This gives terahertz spectroscopy the potential to detect dangerous goods [11, 12]. Although THz waves are easily absorbed and lost by polar molecules such as water [13], the absorption spectroscopy at this time can also be used to evaluate the water content of the sample [14, 15].
THz radiation has so many properties that it has great potential for applications in biomedicine [3, 16–19], agriculture and food manufacturing [20–23], security inspection [24, 25], and many other fields [26–29], as shown in Figure 1. However, due to the limitation of THz wave generation and detection technologies, THz wave has not received much attention and research before 1990s, which is called “THz Gap” [2]. With the development of ultrafast optoelectronics and low-scale semiconductor technology, terahertz spectroscopy has made rapid progress, and related research and applications have focused more and more attention.
FIGURE 1. Terahertz spectroscopy and some typical applications [1, 22, 25, 29, 30]. Reproduced with permissions. © 2013 Chinese Physical Society and IOP Publishing Ltd. © 2017 IEEE. © 2020 Springer Science Business Media, LLC, part of Springer Nature. © 2021 The Japan Society of Applied Physics.
As aforementioned, we can conclude that terahertz spectroscopy can provide a comprehensive response to the optical characteristics of a sample in the detection and recognition of a substance. The current terahertz spectroscopic technologies can generally be divided into three types: THz Time-Domain Spectroscopy (THz-TDS) [2], time-resolved terahertz spectroscopy [31, 32] and THz emission spectroscopy based on the concept of laser THz emission microscopy [33]. The most commonly used technique is THz-TDS, which was first proposed in the 1980s [34, 35]. It mainly uses a broadband pulsed radiation source generated by the excitation of semiconductor materials using ultrashort laser pulses [36]. Based on the coherent measurement method, THz-TDS provides the variation of the THz electric amplitude with time after a THz pulse interacts with the sample. The frequency-domain amplitude and phase information of the THz response of the sample can also be calculated by performing a Fourier transform on the measured time domain results. Hence, electromagnetic parameters such as absorption coefficient, refractive index and dielectric constant of the sample can be easily extracted. Terahertz spectroscopy also has the advantages of wide bandwidth, large dynamic range, transient feature, and high sensitivity. Currently, the THz-TDS systems can achieve excellent test performances: a broad bandwidth of about 0.1–3 THz (some can even extend to 10 THz), a high peak signal-to-noise ratio greater than 100 dB, and a frequency resolution up to 10 GHz. Besides, the sampling time can be as low as a few seconds [37, 38].
Taking into account different samples, different detection standards, etc., the measurement modes of THz-TDS systems can usually be divided into transmission type, reflection type, attenuated total reflection type, etc. At present, the common THz-TDS experimental systems are mainly transmission type and reflection type. A typical THz-TDS experimental setup is shown in Figure 2 [1]. An ultrafast pulse is generated by a femtosecond laser, and then divided into pump beam and probe beam after passing through a beam splitter. The pump beam excites a THz emitter (e.g., a photoconductive antenna) to generate a THz time-domain pulse, which is collimated and focused on the sample by a parabolic mirror. Then, the THz pulse carrying the sample information is collimated and refocused onto the THz detector (e.g., electro-optical crystal). The probe beam collinear with the THz beam is used to gate the detector and measure the instantaneous THz electric field. A delay stage is used to adjust the time delay between the pump beam and probe beam and allows the THz temporal profile to be iteratively sampled. The time domain waveform of the THz pulse is obtained by scanning the time delay. Afterwards, the time domain waveform is amplified by a lock-in amplifier and processed by a computer. In most cases, reflection and transmission measurement modes differ only in that the former receives reflected pulses, while the latter receives transmitted pulses. Transmission spectroscopy is limited by the maximum dynamic range, especially when the sample absorbs THz radiation strongly, resulting in a lower penetration depth. In contrast, the reflection spectroscopy is determined by the signal phase and amplitude accuracy rather than the sample, the maximum absorption depends on the signal-to-noise ratio (SNR). Thus, reflection-type THz-TDS will be more applicable to absorbing materials or devices [39]. In addition, in most experiments, the experimental setup will be placed in a dry air or nitrogen purged box in order to avoid the absorption of THz waves by moisture in the air.
FIGURE 2. Schematic of a typical transmission-type THz-TDS experimental setup [1].
Our paper focuses on a review of the application of terahertz spectroscopy for the detection and recognition of substances, describes the fundamentals of terahertz spectroscopy, and provides an overview of the applications of terahertz spectroscopy in biomedicine, agriculture and food production, and security inspection. Subsequently, the applications of metamaterials in terahertz spectroscopic detection and recognition of substances are discussed. In addition, the development trend of terahertz spectroscopy applied to substances detection and recognition is also prospected.
As mentioned in the previous section, THz waves have fingerprint spectrum, so the terahertz spectroscopy of various biomolecules is characteristic and can be relied upon for the identification of various biomolecules. Additionally, the non-ionizing nature of THz radiation makes it possible to detect biological tissues non-invasively. THz waves are strongly absorbed by polar molecules such as water, while biomolecules have limited absorption, so the THz spectrum can be used to distinguish the water content of biological tissues to assess their status. This, coupled with the penetrating of THz and its relatively high resolution, suggests that terahertz spectroscopy has promising applications in the biomedical field [3].
As early as 2002, Ferguson B et al. used THz-TDS to record terahertz spectroscopy of the four nucleobases [adenine (A), cytosine (C), guanine (G) and thymine (T)] and the corresponding nucleosides that form the deoxyribonucleic acid (DNA) building blocks, as shown in Figure 3A,B [40]. As can be seen, the spectrum of each molecule consists of a series of resonances distributed between 1 and 3.5 THz. An absorption peak is observed at each resonance, and the characteristic change is related to the refractive index. In addition, due to the reduction of the bond length in the system at low temperatures, the position of the bands usually shifts towards high frequencies when cooling. Globus T et al. measured the spectroscopy of DNA samples in liquid phase and could distinguish the spectral patterns for native and denatured DNA [41]. Zhang W et al. measured the THz absorption fingerprints of DNA in microliter DNA solution [17], as shown in Figure 3C,D. Then, they clarified that the fingerprint characteristic comes from DNA locally cross-linked by hydrogen bond. In 2013, Tsurkan MV et al. studied the THz spectra of herring degradation DNA using a femtosecond laser and 2 THz spectroscopy methods based on Backward-Wave Oscillator (BWO) and a high Q cavity frequency synthesizer, and showed that herring DNA has characteristic absorption peaks at 306 GHz, 339 GHz, and 375 GHz, providing a method for more accurate analysis of DNA THz spectra [42]. In 2015, Tang M et al. applied terahertz spectroscopy to the detection of DNA mutations, and their method successfully performed label-free analysis of single-base mutations in DNA molecules [43]. Besides, terahertz spectroscopy can be used to detect amino acids [44–46], proteins [47–49], and other biomolecules with good results.
FIGURE 3. (A) Absorption coefficients and (B) refraction indices of the nucleobases A, C, G and T at 10 and 300 K [40]. Reproduced with permission. © 2002 IOP Publishing Ltd. Spectra of microliters of DNA solution (C) background, sample and noise floor and (D) spectra after exclusion of background effects [17]. Reproduced with permission. © 2013 AIP Publishing. (E) THz resonance peaks for DNA samples after baseline correction, genomic DNA from different cancer types (PC3, A431, A549, MCF-7, and SNU-1 cell lines) has the same resonance peak at 1.67 THz [50] (F) The degree of DNA methylation for each DNA sample. The magnitude of the resonance peak for each sample was quantified by the amount of genomic DNA methylation and compared with commercial DNA methylation quantification measurements (enzyme-linked immunosorbent assay like reaction method (ELISA)-like reaction method, Epigentek Group, Inc.) [50]. Reproduced under a Creative Commons Attribution 4.0 International License. © 2016 Springer Nature.
Carcinogenesis causes chemical and structural abnormalities in biomolecules, one of which is widely known to be abnormal methylation of DNA. In 2016, Cheon H et al. proposed that molecular resonances could be monitored using THz-TDS in aqueous solutions of genomic DNA from cancer cell lines [50]. The quantification of resonance signals led to the identification of cancer cell types with some degree of DNA methylation. It was shown that identical resonance peaks at 1.67 THz were found in genomic DNA from various cancer types (PC3, A431, A549, MCF-7, and SNU-1 cell lines), and the THz resonance peaks for DNA samples after baseline correction are shown in Figure 3E. The magnitude of the resonance peaks for each sample was quantified by the amount of genomic DNA methylation, the degree of DNA methylation for each DNA sample is shown in Figure 3F. This demonstrates that cancer cell DNA also has THz fingerprinting spectra and provides a way to diagnose cancer at the molecular level. Shortly after, Cheon H et al. applied this method to the detection of blood cancers [51], using THz-TDS to observe characteristic resonances of DNA in blood cancers at a frequency of about 1.7 THz, and used this to analyze their methylation levels. Subsequently, they applied THz radiation to the treatment of cancer [52].
Terahertz spectroscopy has been applied to human tissue scanning and cancer diagnosis since around 2000. Woodward RM et al. used the absorption characteristics of THz waves for polar molecules such as water [16], and they distinguished between carcinoma diseased tissue and normal tissue of human basal cell by terahertz spectroscopy. Compared to normal tissue, basal cell carcinoma shows positive THz contrast, while inflammatory and scar tissue shows negative THz contrast, whereby THz images can be further drawn, which can help to better diagnose diseases such as cancer. In 2006, Wallace V further studied this and explained how parameters related to reflected THz pulses provide information about the lateral spread of tumors [53]. In 2009, Ashworth PC et al. used terahertz spectroscopy for the identification of human breast cancer tissue and found cancerous samples with high refractive indices and absorption coefficients in the 0.15–2.0 THz range [54]. In 2014, Hou D et al. used terahertz spectroscopy to differentiate between normal and cancerous gastric tissues, with significantly different shapes and amplitudes between the absorption spectra of the two, and a clustering approach was used to achieve automatic identification of gastric cancer [55].
In 2014, Meng K et al. found that paraffin-embedded gliomas had higher refractive index, absorption coefficient and dielectric constant than normal brain tissue under the terahertz spectroscopy [56]. In 2019, Gavdush AA et al. observed differences in THz spectra of intact tissue and gliomas of grades I to IV, reflecting the potential of terahertz spectroscopy in the intraoperative label-free diagnosis of human brain gliomas. The experimental setup is shown in Figure 4A [57]. In 2021, they further proposed physical models describing the THz permittivity of healthy and pathological brain tissue, on the basis of the Double Debye (DD) and Double Overdamped (DO) oscillator models [19]. The DO model and summation rule were applied ex vivo to estimate water content in intact tissues and gliomas, and the observed results are in good agreement with previously reported data demonstrating that water is a major endogenous label for brain tumors in the THz range. Besides, terahertz spectroscopy is also used in the examination of human colon tissue [58, 59], oral tissue [60], pigmented skin nevi [61], liver tissue [62] and related cancers.
FIGURE 4. (A) Reflection-mode terahertz spectroscopy unit for measuring gelatin-embedded human brain gliomas, based on an off-axis gold-coated parabolic mirror and a reference window on top of which tissue samples are placed [57]. Reproduced under a Creative Commons Attribution 4.0 Unported License. © 2019 SPIE. (B) The process of acquiring THz signals from the corneal surface and corneal subsurface [78]. Reproduced under a Creative Commons Attribution 4.0 Unported License. © 2021 SPIE. After acquiring the waveform once at an incident angle of 30°, a series of reflected beams were consecutively acquired at different distances (multiple points with a spacing of 2 mm) away from the direct reflection.
Considering the differences between cancer patients, THz imaging technologies were employed to aid the diagnosis of cancer. For example, Bowman TC et al. in 2015 used THz pulse imaging to image and analyze heterogeneous breast cancer tissue [63]. They proposed a THz imaging method capable of distinguishing heterogeneous regions of breast tumors, and the results were compared and validated with standard histopathology images. In addition, THz imaging has also been applied to the diagnosis of colon cancer [64, 65], gastric cancer [66], skin cancer [67], liver cancer [30], etc. With the popularity of artificial intelligence in recent years, artificial intelligence techniques such as machine learning have also been used to diagnose cancer by combining with terahertz spectroscopy and imaging techniques [68–70]. Recently, Liu W et al. devised an automatic recognition strategy for THz pulse signals in breast invasive ductal carcinoma (IDC) based on wavelet entropy feature extraction and machine learning classifier [69]. Using principal component analysis method and machine learning classifier to automatically classify THz signals from breast IDC samples, the accuracy, sensitivity, and specificity of breast IDC recognition can reach 92.85%, 89.66%, and 96.67%, respectively.
In addition to diagnosing cancer, terahertz spectroscopy can also be used for the diagnosis of diseases such as diabetes. As early as the beginning of this century, researchers have used terahertz spectroscopy in dental care [71–74], for example in the detection of dental caries, where there is a higher attenuation of THz radiation in decayed enamel than that in healthy enamel. In 2010, Sy S et al. investigated in detail the correlation between THz properties of liver tissue, water content, structural changes, and cirrhosis, showing significant differences between the THz properties of normal and cirrhotic tissues [75]. Recently, Lykina AA et al. used terahertz spectroscopy to study plasma samples from diabetic and non-diabetic patients hoping to complete the assay nondestructively [76, 77]. The results showed that the normalized refractive index of particles from diabetic samples in the range of 0.2–1.4 THz increased by an average of 9–12% compared to particles from non-diabetic samples, and their terahertz spectroscopy were also spatially separated in principal component space. Detection of corneal edema can prevent corneal disease from reaching its end stage, and Ke L et al. assessed the degree of corneal edema by means of high-sensitivity THz broadband spectroscopy to detect corneal components at different depths. The process of THz signals acquired from the corneal surface and corneal subsurface is illustrated in Figure 4B [78].
Controlling the content of pesticide residues and harmful substances in food is an important measurement to ensure food safety. This is also an important application of terahertz spectroscopy in the agricultural field. As early as 2010, Hua Y et al. carried out research on the application of terahertz spectroscopy to detect the pesticide residues [79]. They used THz-TDS at frequencies of 0.5–1.5 THz for cyfluthrin n-hexane solutions in the concentration range of 1–20 mg/ml, and proposed THz-TDS plus partial least squares (PLS) and nonlinear regression methods of simple least-squares support vector machine (LS-SVM) is an effective tool for the quantitative analysis of cyfluthrin n-hexane solutions. Then, Hua Y et al. investigated four pesticides and three food powders as well as polyethylene using THz-TDS in the frequency range of 0.5–1.6 THz [20], and used PLS regression for the detection and analysis of imidacloprid in polyethylene and glutinous rice powder at different weight ratios. The results of the study showed that the four pesticides could be distinguished from each other by their unique absorption peaks, as well as from food powders that did not have this feature. In addition, differences in refractive index between pesticides and food powders can be observed. Imidacloprid can be recognized by its absorption fingerprints in mixtures of imidacloprid and polyethylene and imidacloprid and glutinous rice flour, as shown in Figure 5A,B. Through the linear relationship between the weight ratio of imidacloprid and the absorption coefficient, the authors predicted the weight ratio of imidacloprid in the above two mixtures of powders and achieved a relative error of less than 5%.
FIGURE 5. THz absorption spectra of different weight ratios of imidacloprid (A) in polyethylene and (B) in glutinous rice flour. The labels show the corresponding weight percentages of imidacloprid in these mixtures. The numbers in the graph represent the location of the peaks and the arrows indicate an overall increasing trend in the weight ratio of imidacloprid in the mixtures [20]. Reproduced with permission. © 2010 IEEE. (C) Absorption spectra of methomyl at 0.1–3 THz and (D) calibration curve at 1 THz [83]. Reproduced with permission. © 2015 Springer Science Business Media New York.
Subsequently, more and more researchers applied terahertz spectroscopy to detect pesticides, insecticides, and fungicides residues. Wang Q et al. conducted a study on nitrofen [80], and then measured the THz spectrum of thiabendazole at room temperature [81]. Maeng I et al. used terahertz spectroscopy to detect seven different pesticide residues in wheat flour [82]. Baek SH et al. used THz-TDS to detect methomyl (a carbamate insecticide) in food, and the characteristic absorption peaks of methomyl measured at room temperature in the frequency range of 0.1–3 THz were 1, 1.64, and 1.89 THz as shown in Figure 5C [83]. The three absorption peaks of methomyl are linearly proportional to their concentrations in high-density polyethylene. The peak at 1 THz was used as the fingerprint for detecting methomyl in rice and wheat flours. Figure 5D shows the calibration curve for methomyl which showed a regression coefficient >0.957 and a detection limit <3.74%. Accuracy and precision expressed as recovery and relative standard deviation (RSD, %) in interday repeatability were in the ranges 78.0–96.5 and 2.83–4.98%, respectively. Although THz-TDS can be used to rapidly detect methomyl in food, its sensitivity still needs to be improved. Qin B et al. applied THz-TDS combined with fast search and find of density peaks (CFSFDP) clustering to detect carbendazim residues [21]. Zhang H et al. combined terahertz spectroscopy with an improved PLS method for the detection of the harmful additives Auramine O in the medicinal herb Pollen Typhae [84]. Liu W et al. applied machine learning to terahertz spectroscopy to detect aflatoxin B1 in soybean oil, and obtained an accuracy rate of over 90% [85]. Recently, Ma Q et al. combined terahertz spectroscopy with a back-propagation neural network (BPNN) for the quantitative analysis of ternary pesticide mixtures in wheat flour [23], and obtained good results with correlation coefficients of 0.9913, 0.9948, and 0.9923 for the prediction sets, the corresponding root mean square errors of 0.0211%, 0.0176%, and 0.0191%, respectively.
The application of terahertz spectroscopy in the agricultural field is far more than the detection of pesticide residues and harmful substances, but also in the identification of agricultural products [86–88], the detection of soil [89–91] and the detection of genetically modified crops [92–94]. More brand new applications are being explored.
In 2003, Campbell MB et al. proposed that terahertz spectroscopy could be used to non-destructively detect explosives and other weapons of mass destruction [95], and demonstrated that a THz spectral database that includes biological warfare agent (BWA) simulants, chemical warfare agent (CWA) simulants, explosives, pharmaceuticals, dozens of potential hoax materials as well as several types of papers and plastics used in common containers that may hold threat agents. Chen Y et al. in 2004 used Fourier transform infrared spectroscopy (FTIR) and THz-TDS to measure 14 commonly used explosive samples [96], and the far-infrared spectra of the explosives 2, 3-dinitro toluene (2,4-DNT), 4-nitro toluene (4NT), and 2, 6-dinitro toluene (2,6-DNT) measured via FTIR and THz were in good agreement with their structure and vibrational frequencies obtained using density functional theory (DFT) [97, 98]. In 2005, they applied THz diffuse reflectance spectroscopy (DRS) to the detection of explosives [24]. The experimental results show that DRS technology has higher sensitivity and easier sample preparation than transmission spectroscopy. In 2006, they obtained the absorption spectrum of the explosive hexahydro-1,3,5-trinitro-1,3,5-triazine (RDX) from the reflectance spectrum [99], and were able to distinguish RDX from other materials. In 2007, they used 0.1–2.8 THz THz-TDS to study the absorption spectrum of 17 explosives [100]. Most of the explosives show characteristic absorption, which can be used as a basis to build a relevant database. Moreover, they also considered that explosives are usually hidden, so they studied the absorption spectrum of explosives under the cover material to prove that the THz system can be used to detect hidden explosives. The experimental results show that the transmission spectra of RDX and tetramethylene tetranitramine (HMX) samples covered with three different materials - plastic, cotton, and leather at 0.1–3 THz agree well with those of the samples alone, which indicates that the barrier materials are mostly transparent to the THz wave.
Considering that the sample may not be a single-component explosive but a mixture in the actual security inspection, based on the spectral data of known pure explosives components, Chen Y et al. proposed the THz spectral uncertainty analysis using micro genetic algorithm [101], by applying intelligent computing to the analysis and optimization of THz spectral combination. In this work, the mixture of benzoic acid, o-toluic acid and p-toluic acid were specifically analyzed. In 2015, Trofimov VA et al. proposed a method for the efficient analysis of explosives by pulsed terahertz spectroscopy using spectral dynamics [102]. In recent years, they have also developed an efficient tool to detect and identify substances in ternary explosive mixtures with similar spectral properties using broadband reflected THz signals [103].
In security applications, terahertz spectroscopy is used not only for the inspection of explosives, but also for the inspection of other contraband such as drugs [104, 105]. The THz images obtained after the analysis and processing of THz spectral information can also be used for the rapid detection of firearms and controlled knives [106–108]. As the application of terahertz spectroscopy technology for security screening technology continues to mature, more and more THz security inspection equipment began to put into practical application [25, 109].
As we have already mentioned, terahertz spectroscopy can be used for non-destructive testing. We have also described its applications in biomedicine, security inspection, etc. This property can also be used for the detection of other substances and materials. In 2008, Jackson JB et al. used terahertz spectroscopy for the nondestructive examination of frescoes [110]. Subsequently, Abraham E et al. applied terahertz spectroscopy to the inspection of art paintings [26], and in 2015, Koch Dandolo CL et al. applied it to the inspection of panel paintings beneath gilded finishes [111].
THz fingerprints are available for pigments, adhesives and substrates of artworks, however, researchers still lack a database of THz spectra of these substances and materials, which makes the application of terahertz spectroscopy for artwork identification still difficult, and many researchers have set out to determine the THz spectra of different pigments. Li CY et al. in 2017 performed terahertz spectroscopy on several traditional Chinese pigments including cinnabar, red lead, oyster shell white, realgar, malachite, orpiment and azurite [112]. In 2019, Kleist EM et al. provided insight into the molecular and intermolecular forces that give rise to spectroscopy absorption features of crystalline copper-containing historical pigments in solid-state density flooding theory simulations and reveal deviations from simple harmonic vibrational behavior that may complicate these spectra, while investigating terahertz spectroscopy of solid azurite, malachite, and verdigris [113]. In the same year, Squires AD et al. analyzed phthalocyanine pigments under terahertz spectroscopy [114], while the identification and differentiation of copper phthalocyanines’ α, β and
The aforementioned applications of terahertz spectroscopy are almost based on typical THz-TDS. Nevertheless, other types of terahertz spectroscopy have also been reported. In 2003, Kiwa T et al. used THz emission spectroscopy to check electrical faults in integrated circuits (IC), and the two-dimensional THz emission images of IC chips could be clearly observed [33]. In 2008, Dressel M et al. investigated the superconducting energy gap of superconductors using terahertz time-resolved spectroscopy [32]. They obtained terahertz spectroscopy of niobium and niobium alloys, magnesium diboride, and some organic and high-temperature superconductors to explore the inherent laws of superconductors.
Furthermore, terahertz spectroscopy can also be applied to building detection [28], energy power [116, 117], pharmaceuticals [118], food science [119], marine engineering [27], and other fields [120, 121].
In the previous section, we have talked about the great advantages of terahertz spectroscopy, nevertheless, the terahertz spectroscopy for substance detection also subjects to some limitations. The fact that the electromagnetic waves in the THz band have longer wavelengths compared to X-rays, for example. This means that their resolution and sensitivity is not high enough for detection of small samples and trace analysis when performing nondestructive testing. In order to solve these problems, researchers have carried out a lot of research.
One of the solutions is the application of THz metamaterials, which can enhance the interaction of the sample with THz wave, thereby improving the resolution and sensitivity of THz spectroscopic detection. Metamaterials are artificial structures composed of subwavelength unitary structures arranged and combined in a periodic or non-periodic form [122, 123]. In the design process, it is only necessary to design the parameters of its unit structure or change its spatial arrangement to realize desired extraordinary physical properties that cannot or are difficult to achieve with natural materials and traditional technologies, such as negative permittivity [124, 125], negative permeability [126, 127], negative refraction [128, 129], zero refraction [130], super absorption [131–133], super transparency [134, 135], etc. It is also possible to achieve arbitrary distribution of the medium parameters. In the application of THz spectroscopic detection, we make the resonance with THz waves appear as we need and enhance it by a rationally designed metamaterial unit structure. As early as 2004, Yen TJ et al. proposed a metamaterial that can be used for THz spectroscopic detection [136]. The use of metamaterials can greatly enhance the interaction between the small or even trace samples to be measured and the THz waves, thus making terahertz spectroscopy higher sensitivy for the detection of substances.
For the field of biomedicine, in 2016, Bui TS et al. used THz metamaterials as amplifiers to enhance the absorption signal of THz vibrations in the ultrathin adsorption layer of organic molecules, and on this basis, they detected protein macromolecules such as bovine serum albumin (BSA) [137]. Wang S et al. designed a polarization-insensitive THz metamaterial for the detection of BSA [138]. The detection setup is shown in Figure 6A, and the measured data are displayed in Figure 6B,C. The black curve is the biosensor without BSA molecules, the red curve is 0.75 mmol/L, the blue curve is 1.5 mmol/L, and the pink curve is 3 mmol/L BSA solution. The corresponding resonance frequencies are 1.463, 1.413, 1.3, and 1.188 THz, respectively. This indicates that there is a red shift in the resonant frequency with increasing BSA concentration, and higher concentrations of biological analytes result in a corresponding increase in effective dielectric constant. The change in resonant frequency is approximately linear with the BSA concentration, so the displacement of the resonance can be defined as a function of the change in BSA concentration. They obtained a maximum resonant frequency difference of 1.68 GHz with a minimum resolution of 17.7 μmol/L at full-angle range rotation. Another major challenge for the application of terahertz spectroscopy to biomedical detection is the strong absorption of THz waves in polar solvents such as water. In 2018, Tang M et al. overcame this difficulty by combining THz metamaterials with microfluidics to detect DNA oligonucleotides with base mutations [139]. Based on THz split resonant ring (SRR) metamaterials and microfluidics technology as shown in Figure 6D, Geng Z et al. detected alpha fetoprotein (AFP) and glutamine transferase isozyme II (GGT-II) as biomarkers for early hepatocellular carcinoma, and the detection results based on individual SRR gaps are shown in Figure 6E,F [140]. In 2019, Yan X et al. fabricated a THz metamaterial biosensor based on Fano resonance for the detection of cancer cells, with a theoretical sensitivity close to 455.7 GHz/RIU [141]. In 2020, Karmakar S et al. developed a stacked metasurface for solid matter, thin film, and chemical detection, where analytes can be detected between stacked metasurface that form Fano cavity [142]. Their study shows that broadside coupling can trigger much stronger near-field interactions and large-area interactions with substances leading to ultrasensitive sensing, capable of achieving >1 THz/RIU (= 1.76 × 105 nm/RIU) and a figure of merit of about 14.05 in the THz range. After the occurrence of COVID-19, Ahmadivand A et al. used THz metamaterials for the detection of SARS-CoV-2, and was able to detect SARS-CoV-2 stinger protein at femtomolar concentrations rapidly and accurately, which provided a more convenient and rapid method for the detection of COVID-19 [143]. Recently, Cui N et al. used THz metamaterial biosensors (see Figure 6G) to detect early cancer biomarkers carcinoembryonic antigen (CEA) [144] and breast cancer marker carbohydrate antigen 125 (CA125, results in Figure 6H–K) [145] both achieved good sensitivity. All of the above works indicate that the combination of metamaterials and terahertz spectroscopy has great potential in the future biomedical field.
FIGURE 6. (A) Experimental setup for THz spectral detection combined with metamaterials (B) THz transmission spectra of BSA solutions with different concentrations (C) Least squares fitting of experimental data of resonant frequencies to BSA concentrations [138]. Reproduced with permission. © 2016 IEEE. (D) THz metamaterials with integrated microfluidics for THz spectroscopic detection [140]. Reproduced under a Creative Commons Attribution 4.0 International License. © 2017 Springer Nature. (E) Transmission spectroscopy of liver cancer antibody AFP (1 μg/ml) and liver cancer serum antigen (0.02524 μg/ml) were detected using SRRs biosensors with a gap of 2 µm. The frequency shift of the transmission spectrum before and after injection of AFP antibody and serum antigen was 8.6 GHz [140] (F) The liver cancer biomarkers GGT-II antibody (1 μg/ml) and GGT-II antigen (5 μg/ml) were also detected by the same method. The transmission spectra were shifted by 18.7 GHz before and after injection of GGT-II antibody and antigen [140] (G) THz metamaterials for biomedical detection and the mechanism of reaction of their electric fields with analytes (H) Frequency shifts based on three dips for different concentrations of CA125 antigen and fixed concentrations (30 μg/ml) of CA125 antibody; transmission spectra of (I) U-bowtie triangle ring (BTR) Dip1 (J) S-BTR Dip1 and (K) S-BTR Dip2 at different concentrations of CA125 antigen [145]. Reproduced with permission. © 2021 American Chemical Society.
As for agriculture and food production, we mentioned in the previous section that the researchers used terahertz spectroscopy to detect carbendazim, however, the data processing was complicated and the sensitivity was low. In order to improve the detection sensitivity, they further combined metamaterials to detect carbendazim [146]. The results showed that, the designed metamaterial can detect carbendazim down to 5 mg/L. Tetracycline hydrochloride (TCH) is a residue of antibiotics which is widely used in animals, and excessive residues in food can cause adverse effects on human health. Qin J et al. designed a THz metamaterial for TCH detection, as shown in Figure 7A,B [147]. The THz transmission spectra of TCH deposited on metamaterials were detected at different concentrations and the results were shown in Figure 7C. It shows the transmission spectra of the metamaterial as the TCH concentration varies from 0.01 mg/L to 10,000 mg/L. When the TCH concentration is lower than 0.1 mg/L, there is no significant difference in the transmission amplitude. It can be found that, due to the addition of metamaterials, their method can successfully detect TCH as low as 0.1 mg/L, and its sensitivity is greatly improved compared to the direct use of THz-TDS. In 2017, Xu W et al. applied metamaterials to the detection of chlorpyrifos-methyl (CM) [148]. In this experiment, the THz metamaterial works in reflection mode as shown in Figure 7D, and 10 μL of CM solutions of different concentrations are dropped onto the THz metamaterial and repeated three times. The THz reflection spectra of CM solutions at concentrations of 0.2–1.0 mg/L as well as the frequency shift results are shown in Figure 7E,F. It can be found from Figure 7E that the resonant peaks regularly shift to lower frequencies, and the value of the frequency shift increases with the increase of the CM concentration. Therefore, the frequency shift value should be a function of CM concentration, as shown in Figure 7F. We can observe a good linear relationship between the CM concentration and the frequency shift of the reflectance curve. Consequently, the detection limit of chlorpyrifos reached 0.204 mg/L after loading the THz metamaterial. The emergence of the good results is mainly due to the significant localized electric field enhancement effect of the surface excitation of the THz metamaterial. Subsequently, Nie P et al. designed an all-dielectric broadband THz metamaterial absorber for the detection of chlorpyrifos [133], increasing the detection limit to 0.1 mg/L. Their design has an interaction efficiency of 99% at 1.33 THz, with bandwidth covering a center frequency of 600 GHz, and the sensitivity and stability can be maintained under different temperature, humidity and time conditions. Recently, Liu J used THz metamaterials for the determination of organochlorine pesticide [149], Li B et al. combined terahertz spectroscopy with metamaterials for low-concentration nolosin detection [150].
FIGURE 7. (A) THz metamaterial for the detection of TCH and (B) its Scanning Electron Microscope (SEM) images (C) THz transmission spectra of TCH deposited on metamaterial detected at different concentrations [147]. Reproduced with permission. © 2016 Elsevier Ltd. (D) THz metamaterials for the detection of CM (E) THz reflectance spectra and (F) Frequency shift results of CM at different concentrations (0.2–1.0 mg/L), and the blue curve is a linear fit to the data [148]. Reproduced with permission. © 2017 Elsevier Ltd.
In conclusion, we overview the advantages offered by terahertz spectroscopy in the field of detection and identification of substances, such as biosafety, identification properties, penetration, coherence, high resolution, etc. In addition, with the introduction of metamaterials, terahertz spectroscopy can further improve its resolution and sensitivity to carry out the detection of micro or even trace substances.
At present, whether in biomedicine, security inspection or artwork identification, researchers are more likely to perform spectroscopic detection of specific substances. To advance the application of terahertz spectroscopy, a more extensive THz spectrum database of substances needs to be established. This will require more comprehensive investigations on various materials via terahertz spectroscopy in the future to supplement and improve the relevant data.
Besides, the strong absorption of THz waves by polar molecules such as water in the air remains an obstacle to the application of terahertz spectroscopy, and hence dry air or nitrogen purging or experiments under vacuum conditions are performed to eliminate this effect. However, such conditions are usually unavailable in large-scale practical applications, and this also limit the fast detection of substances. In addition, although we also mentioned that THz waves are penetrating, their penetration depth is still limited, especially when the sample is hidden behind an obstacle containing water. In the future, it is necessary to further optimize the structure of metamaterials, develop more new technologies similar to microfluidic channels, and propose newer data processing algorithms to more accurately describe the characteristics of terahertz spectroscopy of substances. Additionally, it is also necessary to further reduce the cost of THz technology, increase the detection limit, and further improve the resolution and signal-to-noise ratio of instruments. Overall, terahertz spectroscopy technology has been showing more and more significant potential for application in the detection and recognition of substances.
XF and TJC: supervision, preparation, and revision of the manuscript. YL wrote the first draft of the manuscript. QC and YF contributed to the preparation of the manuscript. All authors approved the submitted version.
This work was supported in part by the National Key Research and Development Program of China (Grant Nos. 2018YFB1801505, 2017YFA0700201, 2017YFA0700202, and 2017YFA0700203), in part by the National Natural Science Foundation of China (Grant Nos. 61871127, 61735010, 61731010, 61890544, 61801117, 61722106, 61701107, 61701108, 61701246, and 61631007), and in part by the 111 Project (Grant Nos. 111-2-05).
The authors declare that the research was conducted in the absence of any commercial or financial relationships that could be construed as a potential conflict of interest.
All claims expressed in this article are solely those of the authors and do not necessarily represent those of their affiliated organizations, or those of the publisher, the editors and the reviewers. Any product that may be evaluated in this article, or claim that may be made by its manufacturer, is not guaranteed or endorsed by the publisher.
1. Ferguson B, Zhang X-C. Materials for Terahertz Science and Technology. Nat Mater (2002) 1:26–33. doi:10.1038/nmat708
2. Zhang X-C, Xu J. Terahertz Radiation. In: X-C Zhang, and J Xu, editors. Introduction to THz Wave Photonics. Boston, MA: Springer (2010). p. 1–26. doi:10.1007/978-1-4419-0978-7_1
3. Yang X, Zhao X, Yang K, Liu Y, Liu Y, Fu W, et al. Biomedical Applications of Terahertz Spectroscopy and Imaging. Trends Biotechnol (2016) 34:810–24. doi:10.1016/j.tibtech.2016.04.008
4. Ren A, Zahid A, Fan D, Yang X, Imran MA, Alomainy A, et al. State-of-the-art in Terahertz Sensing for Food and Water Security - A Comprehensive Review. Trends Food Sci Technol (2019) 85:241–51. doi:10.1016/j.tifs.2019.01.019
5. Baxter JB, Guglietta GW. Terahertz Spectroscopy. Anal Chem (2011) 83:4342–68. doi:10.1021/ac200907z
6. Hübers H-W, Eichholz R, Pavlov SG, Richter H. High Resolution Terahertz Spectroscopy with Quantum Cascade Lasers. J Infrared Milli Terahz Waves (2013) 34:325–41. doi:10.1007/s10762-013-9973-7
7. Pal SK, Peon J, Bagchi B, Zewail AH. Biological Water: Femtosecond Dynamics of Macromolecular Hydration. J Phys Chem B (2002) 106:12376–95. doi:10.1021/jp0213506
8. Němec H, Kužel P, Sundström V. Charge Transport in Nanostructured Materials for Solar Energy Conversion Studied by Time-Resolved Terahertz Spectroscopy. J Photochem Photobiol A: Chem (2010) 215:123–39. doi:10.1016/j.jphotochem.2010.08.006
9. Tao YH, Fitzgerald AJ, Wallace VP. Non-Contact, Non-destructive Testing in Various Industrial Sectors with Terahertz Technology. Sensors (2020) 20:712. doi:10.3390/s20030712
10. Nüßler D, Jonuscheit J. Terahertz Based Non-destructive Testing (NDT). Tm - Tech Mess (2021) 88:199–210. doi:10.1515/teme-2019-0100
11. Tribe WR, Newnham DA, Taday PF, Kemp MC. Hidden Object Detection: Security Applications of Terahertz Technology. In: RJ Hwu, editor. Terahertz And Gigahertz Electronics And Photonics III. Integrated Optoelectronic Devices 2004; 2004 Apr 8. San Jose, CA, USA. Bellingham: SPIE (2004). p. 168–76. doi:10.1117/12.543049
12. Damian V, Logofătu PC, Vasile T. 3D THz Hyperspectrum Applied in Security Check-In. In: M Vladescu, R Tamas, and I Cristea, editors. Advanced Topics In Optoelectronics, Microelectronics, and Nanotechnologies VIII. Advanced Topics in Optoelectronics, Microelectronics, and Nanotechnologies 2016; 2016 Dec 14; Constanta, Romania. Bellingham: SPIE (2016). p. 100100Y. doi:10.1117/12.2243276
13. Jin Q, Yiwen E, Williams K, Dai J, Zhang X-C. Observation of Broadband Terahertz Wave Generation from Liquid Water. Appl Phys Lett (2017) 111:071103. doi:10.1063/1.4990824
14. Borovkova M, Khodzitsky M, Demchenko P, Cherkasova O, Popov A, Meglinski I. Terahertz Time-Domain Spectroscopy for Non-invasive Assessment of Water Content in Biological Samples. Biomed Opt Express (2018) 9:2266. doi:10.1364/BOE.9.002266
15. Zang Z, Li Z, Lu X, Liang J, Wang J, Cui H-L, et al. Terahertz Spectroscopy for Quantification of Free Water and Bound Water in Leaf. Comput Electron Agric (2021) 191:106515. doi:10.1016/j.compag.2021.106515
16. Woodward RM, Cole BE, Wallace VP, Pye RJ, Arnone DD, Linfield EH, et al. Terahertz Pulse Imaging in Reflection Geometry of Human Skin Cancer and Skin Tissue. Phys Med Biol (2002) 47:3853–63. doi:10.1088/0031-9155/47/21/325
17. Zhang W, Brown ER, Rahman M, Norton ML. Observation of Terahertz Absorption Signatures in Microliter DNA Solutions. Appl Phys Lett (2013) 102:023701. doi:10.1063/1.4775696
18. He Y, Ung BS-Y, Parrott EPJ, Ahuja AT, Pickwell-MacPherson E. Freeze-thaw Hysteresis Effects in Terahertz Imaging of Biomedical Tissues. Biomed Opt Express (2016) 7:4711. doi:10.1364/BOE.7.004711
19. Gavdush AA, Chernomyrdin NV, Komandin GA, Dolganova IN, Nikitin PV, Musina GR, et al. Terahertz Dielectric Spectroscopy of Human Brain Gliomas and Intact Tissues Ex Vivo: Double-Debye and Double-Overdamped-Oscillator Models of Dielectric Response. Biomed Opt Express (2021) 12:69–83. doi:10.1364/BOE.411025
20. Yuefang Hua Y, Hongjian Zhang H. Qualitative and Quantitative Detection of Pesticides with Terahertz Time-Domain Spectroscopy. IEEE Trans Microwave Theor Techn. (2010) 58:2064–70. doi:10.1109/TMTT.2010.2050184
21. Qin B, Li Z, Luo Z, Li Y, Zhang H. Terahertz Time-Domain Spectroscopy Combined with PCA-CFSFDP Applied for Pesticide Detection. Opt Quant Electron (2017) 49:244. doi:10.1007/s11082-017-1080-x
22. Afsharinejad A, Davy A, Naftaly M. Variability of Terahertz Transmission Measured in Live Plant Leaves. IEEE Geosci Remote Sensing Lett (2017) 14:636–8. doi:10.1109/LGRS.2017.2667225
23. Ma Q, Teng Y, Li C, Jiang L. Simultaneous Quantitative Determination of Low-Concentration Ternary Pesticide Mixtures in Wheat Flour Based on Terahertz Spectroscopy and BPNN. Food Chem (2022) 377:132030. doi:10.1016/j.foodchem.2021.132030
24. Chen Y, Liu H, Fitch MJ, Osiander R, Spicer JB, Shur M, et al. THz Diffuse Reflectance Spectra of Selected Explosives and Related Compounds. In: RJ Hwu, DM Woolard, and MJ Rosker, editors. Terahertz For Military And Security Applications III. Defense and Security. Orlando, FL, USA. Bellingham: SPIE (2005). p. 19–24. doi:10.1117/12.602959
25. Tzydynzhapov G, Gusikhin P, Muravev V, Dremin A, Nefyodov Y, Kukushkin I. New Real-Time Sub-terahertz Security Body Scanner. J Infrared Milli Terahz Waves (2020) 41:632–41. doi:10.1007/s10762-020-00683-5
26. Abraham E, Younus A, Delagnes JC, Mounaix P. Non-invasive Investigation of Art Paintings by Terahertz Imaging. Appl Phys A (2010) 100:585–90. doi:10.1007/s00339-010-5642-z
27. Tu W, Zhong S, Incecik A, Fu X. Defect Feature Extraction of marine Protective Coatings by Terahertz Pulsed Imaging. Ocean Eng (2018) 155:382–91. doi:10.1016/j.oceaneng.2018.01.033
28. Krügener K, Ornik J, Schneider LM, Jäckel A, Koch-Dandolo CL, Castro-Camus E, et al. Terahertz Inspection of Buildings and Architectural Art. Appl Sci (2020) 10:5166. doi:10.3390/app10155166
29. Zhang Y, Ren G, Zhou X, Zhou L, Ta L, Su X. Research on Red and green Porcelain Sherd of Bayi Kiln Using Terahertz Time-Domain Spectroscopy and Imaging. Jpn J Appl Phys (2021) 60:122010. doi:10.35848/1347-4065/ac38fc
30. Chen H, Ma S-H, Yan W-X, Wu X-M, Wang X-Z. The Diagnosis of Human Liver Cancer by Using THz Fiber-Scanning Near-Field Imaging. Chin Phys. Lett. (2013) 30:030702. doi:10.1088/0256-307X/30/3/030702
31. Grüner G, Dahl C. Millimeter and Submillimeter Wave Spectroscopy of Solids. Berlin, Heidelberg: Springer (1998).
32. Dressel M, Drichko N, Gorshunov B, Pimenov A. THz Spectroscopy of Superconductors. IEEE J Select Top Quan Electron. (2008) 14:399–406. doi:10.1109/JSTQE.2007.910764
33. Kiwa T, Tonouchi M, Yamashita M, Kawase K. Laser Terahertz-Emission Microscope for Inspecting Electrical Faults in Integrated Circuits. Opt Lett (2003) 28:2058. doi:10.1364/OL.28.002058
34. Auston DH, Smith PR. Generation and Detection of Millimeter Waves by Picosecond Photoconductivity. Appl Phys Lett (1983) 43:631–3. doi:10.1063/1.94468
35. Grischkowsky D, Keiding S, van Exter M, Fattinger C. Far-infrared Time-Domain Spectroscopy with Terahertz Beams of Dielectrics and Semiconductors. J Opt Soc Am B (1990) 7:2006. doi:10.1364/JOSAB.7.002006
36. Leahy-Hoppa MR, Miragliotta J, Osiander R, Burnett J, Dikmelik Y, McEnnis C, et al. Ultrafast Laser-Based Spectroscopy and Sensing: Applications in LIBS, CARS, and THz Spectroscopy. Sensors (2010) 10:4342–72. doi:10.3390/s100504342
37. Cao C, Zhang Z, Zhao X, Zhang H, Zhang T, Yu Y. Review of Terahertz Time Domain and Frequency Domain Spectroscopy. Spectrosc Spectr Anal (2018) 38:2688. doi:10.3964/j.issn.1000-0593(2018)09-2688-12
38. Yu L, Hao L, Meiqiong T, Jiaoqi H, Wei L, Jinying D, et al. The Medical Application of Terahertz Technology in Non-invasive Detection of Cells and Tissues: Opportunities and Challenges. RSC Adv (2019) 9:9354–63. doi:10.1039/C8RA10605C
39. Wang Q, Xie L, Ying Y. Overview of Imaging Methods Based on Terahertz Time-Domain Spectroscopy. Appl Spectrosc Rev (2022) 57:249–64. doi:10.1080/05704928.2021.1875480
40. Fischer BM, Walther M, Jepsen PU. Far-infrared Vibrational Modes of DNA Components Studied by Terahertz Time-Domain Spectroscopy. Phys Med Biol (2002) 47:3807–14. doi:10.1088/0031-9155/47/21/319
41. Globus T, Woolard D, Crowe TW, Khromova T, Gelmont B, Hesler J. Terahertz Fourier Transform Characterization of Biological Materials in a Liquid Phase. J Phys D: Appl Phys (2006) 39:3405–13. doi:10.1088/0022-3727/39/15/028
42. Tsurkan MV, Balbekin NS, Sobakinskaya EA, Panin AN, Vaks VL. Terahertz Spectroscopy of DNA. Opt Spectrosc (2013) 114:894–8. doi:10.1134/S0030400X13060222
43. Tang M, Huang Q, Wei D, Zhao G, Chang T, Kou K, et al. Terahertz Spectroscopy of Oligonucleotides in Aqueous Solutions. J Biomed Opt (2015) 20:095009. doi:10.1117/1.JBO.20.9.095009
44. Kutteruf MR, Brown CM, Iwaki LK, Campbell MB, Korter TM, Heilweil EJ. Terahertz Spectroscopy of Short-Chain Polypeptides. Chem Phys Lett (2003) 375:337–43. doi:10.1016/S0009-2614(03)00856-X
45. Yi W, Yu J, Xu Y, Wang F, Yu Q, Sun H, et al. Broadband Terahertz Spectroscopy of Amino Acids. Instrumentation Sci Technol (2017) 45:423–39. doi:10.1080/10739149.2016.1270961
46. Bian Y, Zhang X, Zhu Z, Wu X, Li X, Yang B. Investigation of the Correlations between Amino Acids, Amino Acid Mixtures and Dipeptides by Terahertz Spectroscopy. J Infrared Milli Terahz Waves (2021) 42:64–75. doi:10.1007/s10762-020-00757-4
47. Xie L, Yao Y, Ying Y. The Application of Terahertz Spectroscopy to Protein Detection: A Review. Appl Spectrosc Rev (2014) 49:448–61. doi:10.1080/05704928.2013.847845
48. Wei X, Li S, Zhu S, Zheng W, Xie Y, Zhou S, et al. Terahertz Spectroscopy Combined with Data Dimensionality Reduction Algorithms for Quantitative Analysis of Protein Content in Soybeans. Spectrochimica Acta A: Mol Biomol Spectrosc (2021) 253:119571. doi:10.1016/j.saa.2021.119571
49. Klokkou NT, Rowe DJ, Bowden BM, Sessions NP, West JJ, Wilkinson JS, et al. Structured Surface Wetting of a PTFE Flow-Cell for Terahertz Spectroscopy of Proteins. Sensors Actuators B: Chem (2022) 352:131003. doi:10.1016/j.snb.2021.131003
50. Cheon H, Yang H-j., Lee S-H, Kim YA, Son J-H. Terahertz Molecular Resonance of Cancer DNA. Sci Rep (2016) 6:37103. doi:10.1038/srep37103
51. Cheon H, Paik JH, Choi M, Yang H-J, Son J-H. Detection and Manipulation of Methylation in Blood Cancer DNA Using Terahertz Radiation. Sci Rep (2019) 9:6413. doi:10.1038/s41598-019-42855-x
52. Cheon H, Yang H-J, Choi M, Son J-H. Effective Demethylation of Melanoma Cells Using Terahertz Radiation. Biomed Opt Express (2019) 10:4931. doi:10.1364/BOE.10.004931
53. Wallace VP, Fitzgerald AJ, Pickwell E, Pye RJ, Taday PF, Flanagan N, et al. Terahertz Pulsed Spectroscopy of Human Basal Cell Carcinoma. Appl Spectrosc (2006) 60:1127–33. doi:10.1366/000370206778664635
54. Ashworth PC, Pickwell-MacPherson E, Provenzano E, Pinder SE, Purushotham AD, Pepper M, et al. Terahertz Pulsed Spectroscopy of Freshly Excised Human Breast Cancer. Opt Express (2009) 17:12444. doi:10.1364/OE.17.012444
55. Hou D, Li X, Cai J, Ma Y, Kang X, Huang P, et al. Terahertz Spectroscopic Investigation of Human Gastric normal and Tumor Tissues. Phys Med Biol (2014) 59:5423–40. doi:10.1088/0031-9155/59/18/5423
56. Meng K, Chen T-n., Chen T, Zhu L-g., Liu Q, Li Z, et al. Terahertz Pulsed Spectroscopy of Paraffin-Embedded Brain Glioma. J Biomed Opt (2014) 19:077001. doi:10.1117/1.JBO.19.7.077001
57. Gavdush AA, Chernomyrdin NV, Malakhov KM, Beshplav S-IT, Dolganova IN, Kosyrkova AV, et al. Terahertz Spectroscopy of Gelatin-Embedded Human Brain Gliomas of Different Grades: a Road toward Intraoperative THz Diagnosis. J Biomed Opt (2019) 24:1. doi:10.1117/1.JBO.24.2.027001
58. Wahaia F, Valusis G, Bernardo LM, Almeida A, Moreira JA, Lopes PC, et al. Detection of colon Cancer by Terahertz Techniques. J Mol Struct (2011) 1006:77–82. doi:10.1016/j.molstruc.2011.05.049
59. Eadie LH, Reid CB, Fitzgerald AJ, Wallace VP. Optimizing Multi-Dimensional Terahertz Imaging Analysis for colon Cancer Diagnosis. Expert Syst Appl (2013) 40:2043–50. doi:10.1016/j.eswa.2012.10.019
60. Sim YC, Park JY, Ahn K-M, Park C, Son J-H. Terahertz Imaging of Excised Oral Cancer at Frozen Temperature. Biomed Opt Express (2013) 4:1413. doi:10.1364/BOE.4.001413
61. Zaytsev KI, Kudrin KG, Karasik VE, Reshetov IV, Yurchenko SO. In Vivo terahertz Spectroscopy of Pigmentary Skin Nevi: Pilot Study of Non-invasive Early Diagnosis of Dysplasia. Appl Phys Lett (2015) 106:053702. doi:10.1063/1.4907350
62. Zhang CH, Zhao GF, Jin BB, Hou YY, Jia HH, Chen J, et al. Terahertz Imaging on Subcutaneous Tissues and Liver Inflamed by Liver Cancer Cells. Terahertz Sci Technol (2012) 5:114–23. doi:10.11906/TST.114-123.2012.09.10
63. Bowman TC, El-Shenawee M, Campbell LK. Terahertz Imaging of Excised Breast Tumor Tissue on Paraffin Sections. IEEE Trans Antennas Propagat (2015) 63:2088–97. doi:10.1109/TAP.2015.2406893
64. Chen H, Ma S, Wu X, Yang W, Zhao T. Diagnose Human Colonic Tissues by Terahertz Near-Field Imaging. J Biomed Opt (2015) 20:036017. doi:10.1117/1.JBO.20.3.036017
65. Wahaia F, Kasalynas I, Venckevicius R, Seliuta D, Valusis G, Urbanowicz A, et al. Terahertz Absorption and Reflection Imaging of Carcinoma-Affected colon Tissues Embedded in Paraffin. J Mol Struct (2016) 1107:214–9. doi:10.1016/j.molstruc.2015.11.048
66. Ji YB, Park CH, Kim H, Kim S-H, Lee GM, Noh SK, et al. Feasibility of Terahertz Reflectometry for Discrimination of Human Early Gastric Cancers. Biomed Opt Express (2015) 6:1398. doi:10.1364/BOE.6.001398
67. Joseph CS, Patel R, Neel VA, Giles RH, Yaroslavsky AN. Imaging of Ex Vivononmelanoma Skin Cancers in the Optical and Terahertz Spectral Regions Optical and Terahertz Skin Cancers Imaging. J Biophoton (2014) 7:295–303. doi:10.1002/jbio.201200111
68. Qi N, Zhang Z, Xiang Y, Yang Y, Liang X, Harrington Pd. B. Terahertz Time-Domain Spectroscopy Combined with Support Vector Machines and Partial Least Squares-Discriminant Analysis Applied for the Diagnosis of Cervical Carcinoma. Anal Methods (2015) 7:2333–8. doi:10.1039/C4AY02665A
69. Liu W, Zhang R, Ling Y, Tang H, She R, Wei G, et al. Automatic Recognition of Breast Invasive Ductal Carcinoma Based on Terahertz Spectroscopy with Wavelet Packet Transform and Machine Learning. Biomed Opt Express (2020) 11:971. doi:10.1364/BOE.381623
70. Liu H, Vohra N, Bailey K, El-Shenawee M, Nelson AH. Deep Learning Classification of Breast Cancer Tissue from Terahertz Imaging through Wavelet Synchro-Squeezed Transformation and Transfer Learning. J Infrared Milli Terahz Waves (2022) 43:48–70. doi:10.1007/s10762-021-00839-x
71. Crawley DA, Longbottom C, Cole BE, Ciesla CM, Arnone D, Wallace VP, et al. Terahertz Pulse Imaging: A Pilot Study of Potential Applications in Dentistry. Caries Res (2003) 37:352–9. doi:10.1159/000072167
72. Crawley D, Longbottom C, Wallace VP, Cole B, Arnone D, Pepper M. Three-dimensional Terahertz Pulse Imaging of Dental Tissue. J Biomed Opt (2003) 8:303. doi:10.1117/1.1559059
73. Pickwell E, Wallace VP, Cole BE, Ali S, Longbottom C, Lynch RJM, et al. A Comparison of Terahertz Pulsed Imaging with Transmission Microradiography for Depth Measurement of Enamel Demineralisation In Vitro. Caries Res (2007) 41:49–55. doi:10.1159/000096105
74. Churchley D, Lynch RJM, Lippert F, O’Bryan Eder JS, Alton J, Gonzalez-Cabezas C. Terahertz Pulsed Imaging Study to Assess Remineralization of Artificial Caries Lesions. J Biomed Opt (2011) 16:026001. doi:10.1117/1.3540277
75. Sy S, Huang S, Wang Y-XJ, Yu J, Ahuja AT, Zhang Y-t., et al. Terahertz Spectroscopy of Liver Cirrhosis: Investigating the Origin of Contrast. Phys Med Biol (2010) 55:7587–96. doi:10.1088/0031-9155/55/24/013
76. Lykina AA, Anfertev VA, Domracheva EG, Chernyaeva MB, Kononova YA, Toropova YG, et al. Terahertz High-Resolution Spectroscopy of thermal Decomposition Gas Products of Diabetic and Non-diabetic Blood Plasma and Kidney Tissue Pellets. J Biomed Opt (2021) 26:26. doi:10.1117/1.JBO.26.4.043008
77. Lykina AA, Nazarov MM, Konnikova MR, Mustafin IA, Vaks VL, Anfertev VA, et al. Terahertz Spectroscopy of Diabetic and Non-diabetic Human Blood Plasma Pellets. J Biomed Opt (2021) 26:043006. doi:10.1117/1.JBO.26.4.043006
78. Ke L, Wu QYS, Zhang N, Yang Z, Teo EPW, Mehta JS, et al. Terahertz Spectroscopy Analysis of Human Corneal Sublayers. J Biomed Opt (2021) 26:043011. doi:10.1117/1.JBO.26.4.043011
79. Yuefang Hua Y, Hongjian Zhang H, Hongliang Zhou H. Quantitative Determination of Cyfluthrin in N-Hexane by Terahertz Time-Domain Spectroscopy with Chemometrics Methods. IEEE Trans Instrum Meas (2010) 59:1414–23. doi:10.1109/TIM.2010.2041020
80. Wang Q, Ma Y-h. Qualitative and Quantitative Identification of Nitrofen in Terahertz Region. Chemometrics Intell Lab Syst (2013) 127:43–8. doi:10.1016/j.chemolab.2013.05.011
81. Ma Y, Wang Q, Li L. PLS Model Investigation of Thiabendazole Based on THz Spectrum. J Quantitative Spectrosc Radiative Transfer (2013) 117:7–14. doi:10.1016/j.jqsrt.2012.12.003
82. Maeng I, Baek SH, Kim HY, Ok G-S, Choi S-W, Chun HS. Feasibility of Using Terahertz Spectroscopy to Detect Seven Different Pesticides in Wheat Flour. J Food Prot (2014) 77:2081–7. doi:10.4315/0362-028X.JFP-14-138
83. Baek SH, Kang JH, Hwang YH, Ok KM, Kwak K, Chun HS. Detection of Methomyl, a Carbamate Insecticide, in Food Matrices Using Terahertz Time-Domain Spectroscopy. J Infrared Milli Terahz Waves (2016) 37:486–97. doi:10.1007/s10762-015-0234-9
84. Zhang H, Li Z. Terahertz Spectroscopy Applied to Quantitative Determination of Harmful Additives in Medicinal Herbs. Optik (2018) 156:834–40. doi:10.1016/j.ijleo.2017.12.050
85. Liu W, Zhao P, Wu C, Liu C, Yang J, Zheng L. Rapid Determination of Aflatoxin B1 Concentration in Soybean Oil Using Terahertz Spectroscopy with Chemometric Methods. Food Chem (2019) 293:213–9. doi:10.1016/j.foodchem.2019.04.081
86. Kou T, Ye J, Wang J, Peng Y, Wang Z, Shi C, et al. Terahertz Spectroscopy for Accurate Identification of Panax Quinquefolium Basing on Nonconjugated 24(R)-Pseudoginsenoside F11. Plant Phenomics (2021) 2021:1–8. doi:10.34133/2021/6793457
87. Yang S, Li C, Mei Y, Liu W, Liu R, Chen W, et al. Discrimination of Corn Variety Using Terahertz Spectroscopy Combined with Chemometrics Methods. Spectrochimica Acta Part A: Mol Biomol Spectrosc (2021) 252:119475. doi:10.1016/j.saa.2021.119475
88. Liu W, Liu C, Chen F, Yang J, Zheng L. Discrimination of Transgenic Soybean Seeds by Terahertz Spectroscopy. Sci Rep (2016) 6:35799. doi:10.1038/srep35799
89. Dworak V, Augustin S, Gebbers R. Application of Terahertz Radiation to Soil Measurements: Initial Results. Sensors (2011) 11:9973–88. doi:10.3390/s111009973
90. Li B, Wang M-h., Cao W, Zhang Z-w. Research on Heavy Metal Ions Detection in Soil with Terahertz Time-Domain Spectroscopy. In: X-C Zhang, J Yao, C Zhang, and Z Wang, editors. International Symposium on Photoelectronic Detection and Imaging 2011: Terahertz Wave Technologies and Applications. Beijing, ChinaBellingham: SPIE (2011). p. 81951V. doi:10.1117/12.902302
91. Song MJ., Li JS. Detection of POPs in Soil by Using Terahertz Time-Domain Spectroscopy. In: J Yao, XC Zhang, D Yan, and J Liu, editors. Photonics And Optoelectronics Meetings (POEM) 2011: Laser And Terahertz Science And Technology. Photonics and Optoelectronics Meetings 2011; 2012 Mar 14. Wuhan, China. Bellingham: SPIE (2012). p. 833017. doi:10.1117/12.919970
92. Pan S, Qin B, Bi L, Zheng J, Yang R, Yang X, et al. An Unsupervised Learning Method for the Detection of Genetically Modified Crops Based on Terahertz Spectral Data Analysis. Security Commun Networks (2021) 2021:1–7. doi:10.1155/2021/5516253
93. Liu J, Fan L, Liu Y, Mao L, Kan J. Application of Terahertz Spectroscopy and Chemometrics for Discrimination of Transgenic Camellia Oil. Spectrochimica Acta Part A: Mol Biomol Spectrosc (2019) 206:165–9. doi:10.1016/j.saa.2018.08.005
94. Chen T, Li Z, Yin X, Hu F, Hu C. Discrimination of Genetically Modified Sugar Beets Based on Terahertz Spectroscopy. Spectrochimica Acta Part A: Mol Biomol Spectrosc (2016) 153:586–90. doi:10.1016/j.saa.2015.09.028
95. Campbell MB, Heilweil EJ. Noninvasive Detection of Weapons of Mass Destruction Using Terahertz Radiation. In: RJ Hwu, and DL Woolard, editors. Terahertz For Military And Security Applications. AeroSense 2003; 2003 Jul 29. Orlando, FL, USA. Bellingham: SPIE (2003). p. 5070. doi:10.1117/12.504297
96. Chen Y, Liu H, Deng Y, Veksler DB, Shur MS, Zhang X-C, et al. Spectroscopic Characterization of Explosives in the Far-Infrared Region. In: RJ Hwu, and DL Woolard, editors. Terahertz For Military And Security Applications II. Defense and Security. Orlando, FL, USA. Bellingham: SPIE (2004). p. 5411. doi:10.1117/12.540945
97. Chen Y, Liu H, Zhang X-C. Experimental and Density Functional Theory Study on Thz Spectra of 4-Nt and 2, 6-Dnt. Int J Hi Spe Ele Syst (2007) 17:283–91. doi:10.1142/S0129156407004503
98. Chen Y, Liu H, Deng Y, Schauki D, Fitch MJ, Osiander R, et al. THz Spectroscopic Investigation of 2,4-dinitrotoluene. Chem Phys Lett (2004) 400:357–61. doi:10.1016/j.cplett.2004.10.117
99. Liu H-B, Chen Y, Bastiaans GJ, Zhang X-C. Detection and Identification of Explosive RDX by THz Diffuse Reflection Spectroscopy. Opt Express (2006) 14:415. doi:10.1364/OPEX.14.000415
100. Chen J, Chen Y, Zhao H, Bastiaans GJ, Zhang X-C. Absorption Coefficients of Selected Explosives and Related Compounds in the Range of 0.1-2.8 THz. Opt Express (2007) 15:12060. doi:10.1364/OE.15.012060
101. Chen Y, Ma Y, Lu Z, Qiu L, He J. Terahertz Spectroscopic Uncertainty Analysis for Explosive Mixture Components Determination Using Multi-Objective Micro-genetic Algorithm. Adv Eng Softw (2011) 42:649–59. doi:10.1016/j.advengsoft.2011.04.011
102. Trofimov VA, Varentsova SA. Efficiency of Using the Spectral Dynamics Analysis for Pulsed THz Spectroscopy of Both Explosive and Other Materials. In: SS Bishop, and JC Isaacs, editors. Detection And Sensing Of Mines, Explosive Objects, and Obscured Targets XX. SPIE Defense + Security. Baltimore, MD, USA. Bellingham: SPIE (2015). p. 945409. doi:10.1117/12.2178001
103. Trofimov VA, Varentsova SA. A Possible Way for the Detection and Identification of Dangerous Substances in Ternary Mixtures Using THz Pulsed Spectroscopy. Sensors (2019) 19:2365. doi:10.3390/s19102365
104. Davies AG, Burnett AD, Fan W, Linfield EH, Cunningham JE. Terahertz Spectroscopy of Explosives and Drugs. Mater Today (2008) 11:18–26. doi:10.1016/S1369-7021(08)70016-6
105. Burnett AD, Fan W, Upadhya PC, Cunningham JE, Hargreaves MD, Munshi T, et al. Broadband Terahertz Time-Domain Spectroscopy of Drugs-Of-Abuse and the Use of Principal Component Analysis. Analyst (2009) 134:1658. doi:10.1039/b817839a
106. Federici JF, Schulkin B, Huang F, Gary D, Barat R, Oliveira F, et al. THz Imaging and Sensing for Security Applications-Explosives, Weapons and Drugs. Semicond Sci Technol (2005) 20:S266–S280. doi:10.1088/0268-1242/20/7/018
107. Shi X, Chen J. Development of a Standoff Terahertz Imaging System for Concealed Weapon Detection. Microw Opt Technol Lett (2019) 61:1116–20. doi:10.1002/mop.31688
108. Liang D, Pan J, Yu Y, Zhou H. Concealed Object Segmentation in Terahertz Imaging via Adversarial Learning. Optik (2019) 185:1104–14. doi:10.1016/j.ijleo.2019.04.034
109. Alexander NE, Alderman B, Allona F, Frijlink P, Gonzalo R, Hägelen M, et al. TeraSCREEN: Multi-Frequency Multi-Mode Terahertz Screening for Border Checks. In: DA Wikner, and AR Luukanen, editors. Passive and Active Millimeter-Wave Imaging XVII. SPIE Defense + Security. Baltimore, MD, USA. Bellingham: SPIE (2014). p. 907802. doi:10.1117/12.2049926
110. Jackson JB, Mourou M, Whitaker JF, Duling IN, Williamson SL, Menu M, et al. Terahertz Imaging for Non-destructive Evaluation of Mural Paintings. Opt Commun (2008) 281:527–32. doi:10.1016/j.optcom.2007.10.049
111. Koch Dandolo CL, Cosentino A, Jepsen PU. Inspection of Panel Paintings beneath Gilded Finishes Using Terahertz Time-Domain Imaging. Stud Conservation (2015) 60:S159–S166. doi:10.1179/0039363015Z.000000000220
112. Li C, Shi N, Li G, Zhang Z, Gu A, Lei Y, et al. Study of the Traditional Chinese Pigments by Terahertz Time-Domain and Fourier-Transform Infrared Spectroscopy. In: Y Wang, X Li, B Lee, and X Tan, editors. AOPC 2017: Optical Storage And Display Technology. Applied Optics and Photonics China (AOPC2017). Beijing, China. Bellingham: SPIE (2017). p. 1045902. doi:10.1117/12.2281602
113. Kleist EM, Koch Dandolo CL, Guillet J-P, Mounaix P, Korter TM. Terahertz Spectroscopy and Quantum Mechanical Simulations of Crystalline Copper-Containing Historical Pigments. J Phys Chem A (2019) 123:1225–32. doi:10.1021/acs.jpca.8b11676
114. Squires AD, Lewis RA. Terahertz Analysis of Phthalocyanine Pigments. J Infrared Milli Terahz Waves (2019) 40:738–51. doi:10.1007/s10762-019-00599-9
115. Lee JE, Lee H, Kim J, Jung TS, Kim JH, Kim J, et al. Terahertz Spectroscopic Analysis of the Vermilion Pigment in Free-Standing and Polyethylene-Mixed Forms. ACS Omega (2021) 6:13802–6. doi:10.1021/acsomega.1c01336
116. Zhan H, Wu S, Bao R, Ge L, Zhao K. Qualitative Identification of Crude Oils from Different Oil fields Using Terahertz Time-Domain Spectroscopy. Fuel (2015) 143:189–93. doi:10.1016/j.fuel.2014.11.047
117. Li Y, Miao X, Zhan H, Wang W, Bao R, Leng W, et al. Evaluating Oil Potential in Shale Formations Using Terahertz Time-Domain Spectroscopy. J Energ Resour Technol (2018) 140:034501. doi:10.1115/1.4038664
118. Patil MR, Ganorkar SB, Patil AS, Shirkhedkar AA. Terahertz Spectroscopy: Encoding the Discovery, Instrumentation, and Applications toward Pharmaceutical Prospectives. Crit Rev Anal Chem (2022) 52:343–55. doi:10.1080/10408347.2020.1802219
119. Zhang L, Zhang M, Mujumdar AS. Terahertz Spectroscopy: A Powerful Technique for Food Drying Research. Food Rev Int (2021). doi:10.1080/87559129.2021.1936004 ahead of print
120. Cosentino A. Terahertz and Cultural Heritage Science: Examination of Art and Archaeology. Technologies (2016) 4:6. doi:10.3390/technologies4010006
121. Yin M, Tang S, Tong M. The Application of Terahertz Spectroscopy to Liquid Petrochemicals Detection: A Review. Appl Spectrosc Rev (2016) 51:379–96. doi:10.1080/05704928.2016.1141291
122. Caloz C, Itoh T. Electromagnetic Metamaterials: Transmission Line Theory and Microwave Applications. Hoboken, New Jersey: John Wiley & Sons (2005).
123. Cui T. Electromagnetic Metamaterials—From Effective media to Field Programmable Systems. Sci Sin Informationis (2020) 50:1427. doi:10.1360/SSI-2020-0123
124. Schurig D, Mock JJ, Smith DR. Electric-field-coupled Resonators for Negative Permittivity Metamaterials. Appl Phys Lett (2006) 88:041109. doi:10.1063/1.2166681
125. Xu X, Fu Q, Gu H, Guo Y, Zhou H, Zhang J, et al. Polyaniline Crystalline Nanostructures Dependent Negative Permittivity Metamaterials. Polymer (2020) 188:122129. doi:10.1016/j.polymer.2019.122129
126. Zhao Q, Kang L, Du B, Li B, Zhou J, Tang H, et al. Electrically Tunable Negative Permeability Metamaterials Based on Nematic Liquid Crystals. Appl Phys Lett (2007) 90:011112. doi:10.1063/1.2430485
127. Marqués R, Medina F, Rafii-El-Idrissi R. Role of Bianisotropy in Negative Permeability and Left-Handed Metamaterials. Phys Rev B (2002) 65:144440. doi:10.1103/PhysRevB.65.144440
128. Padilla WJ, Basov DN, Smith DR. Negative Refractive index Metamaterials. Mater Today (2006) 9:28–35. doi:10.1016/S1369-7021(06)71573-5
129. Valentine J, Zhang S, Zentgraf T, Ulin-Avila E, Genov DA, Bartal G, et al. Three-dimensional Optical Metamaterial with a Negative Refractive index. Nature (2008) 455:376–9. doi:10.1038/nature07247
130. Moitra P, Yang Y, Anderson Z, Kravchenko , Briggs DP, Valentine J. Realization of an All-Dielectric Zero-index Optical Metamaterial. Nat Photon (2013) 7:791–5. doi:10.1038/nphoton.2013.214
131. Ding F, Cui Y, Ge X, Jin Y, He S. Ultra-broadband Microwave Metamaterial Absorber. Appl Phys Lett (2012) 100:103506. doi:10.1063/1.3692178
132. Liu Y, Huang X, Yang H, Hua L, Lei Y. Zigzag Reflective Multifunctional Metamaterial Absorber and Polarization Rotator with Horizontal Strip Structure. Phys Scr (2020) 95:085510. doi:10.1088/1402-4896/aba475
133. Nie P, Zhu D, Cui Z, Qu F, Lin L, Wang Y. Sensitive Detection of Chlorpyrifos Pesticide Using an All-Dielectric Broadband Terahertz Metamaterial Absorber. Sensors Actuators B: Chem (2020) 307:127642. doi:10.1016/j.snb.2019.127642
134. Zhang X, Forrest SR. Theory of the Perfect Lens. Phys Rev B (2011) 84:045427. doi:10.1103/PhysRevB.84.045427
135. Liu Y, Yang H, Huang X, Yu Z, Li S, Yang Y. A Metamaterial Polarization Converter with Half Reflection and Half Transmission Simultaneously. Phys Lett A (2021) 389:127101. doi:10.1016/j.physleta.2020.127101
136. Yen TJ, Padilla WJ, Fang N, Vier DC, Smith DR, Pendry JB, et al. Terahertz Magnetic Response from Artificial Materials. Science (2004) 303:1494–6. doi:10.1126/science.1094025
137. Bui TS, Dao TD, Dang LH, Vu LD, Ohi A, Nabatame T, et al. Metamaterial-enhanced Vibrational Absorption Spectroscopy for the Detection of Protein Molecules. Sci Rep (2016) 6:32123. doi:10.1038/srep32123
138. Wang S, Xia L, Mao H, Jiang X, Yan S, Wang H, et al. Terahertz Biosensing Based on a Polarization-Insensitive Metamaterial. IEEE Photon Technol Lett (2016) 1. doi:10.1109/LPT.2016.2522473
139. Tang M, Zhang M, Yan S, Xia L, Yang Z, Du C, et al. Detection of DNA Oligonucleotides with Base Mutations by Terahertz Spectroscopy and Microstructures. PLOS ONE (2018) 13:e0191515. doi:10.1371/journal.pone.0191515
140. Geng Z, Zhang X, Fan Z, Lv X, Chen H. A Route to Terahertz Metamaterial Biosensor Integrated with Microfluidics for Liver Cancer Biomarker Testing in Early Stage. Sci Rep (2017) 7:16378. doi:10.1038/s41598-017-16762-y
141. Yan X, Yang M, Zhang Z, Liang L, Wei D, Wang M, et al. The Terahertz Electromagnetically Induced Transparency-like Metamaterials for Sensitive Biosensors in the Detection of Cancer Cells. Biosens Bioelectron (2019) 126:485–92. doi:10.1016/j.bios.2018.11.014
142. Karmakar S, Kumar D, Varshney RK, Chowdhury DR. Strong Terahertz Matter Interaction Induced Ultrasensitive Sensing in Fano Cavity Based Stacked Metamaterials. J Phys D: Appl Phys (2020) 53:415101. doi:10.1088/1361-6463/ab94e3
143. Ahmadivand A, Gerislioglu B, Ramezani Z, Kaushik A, Manickam P, Ghoreishi SA. Functionalized Terahertz Plasmonic Metasensors: Femtomolar-Level Detection of SARS-CoV-2 Spike Proteins. Biosens Bioelectron (2021) 177:112971. doi:10.1016/j.bios.2021.112971
144. Cui N, Guan M, Xu M, Fang W, Zhang Y, Zhao C, et al. Design and Application of Terahertz Metamaterial Sensor Based on DSRRs in Clinical Quantitative Detection of Carcinoembryonic Antigen. Opt Express (2020) 28:16834. doi:10.1364/OE.393397
145. Cui N, Guan M, Xu M, Zhao C, Shao H, Zhang Y, et al. High Electric Field-Enhanced Terahertz Metamaterials with Bowtie Triangle Rings: Modeling, Mechanism, and Carbohydrate Antigen 125 Detection. J Phys Chem C (2021) 125:19374–81. doi:10.1021/acs.jpcc.1c05483
146. Qin B, Li Z, Hu F, Hu C, Chen T, Zhang H, et al. Highly Sensitive Detection of Carbendazim by Using Terahertz Time-Domain Spectroscopy Combined with Metamaterial. IEEE Trans Thz Sci Technol (2018) 8:149–54. doi:10.1109/TTHZ.2017.2787458
147. Qin J, Xie L, Ying Y. A High-Sensitivity Terahertz Spectroscopy Technology for Tetracycline Hydrochloride Detection Using Metamaterials. Food Chem (2016) 211:300–5. doi:10.1016/j.foodchem.2016.05.059
148. Xu W, Xie L, Zhu J, Wang W, Ye Z, Ma Y, et al. Terahertz Sensing of Chlorpyrifos-Methyl Using Metamaterials. Food Chem (2017) 218:330–4. doi:10.1016/j.foodchem.2016.09.032
149. Liu J. High-sensitivity Detection Method for Organochlorine Pesticide Residues Based on Loop-Shaped Absorber. Mater Chem Phys (2020) 242:122542. doi:10.1016/j.matchemphys.2019.122542
Keywords: terahertz spectroscopy, substance detection, biological application, agriculture application, safety inspection, metamaterial
Citation: Fu X, Liu Y, Chen Q, Fu Y and Cui TJ (2022) Applications of Terahertz Spectroscopy in the Detection and Recognition of Substances. Front. Phys. 10:869537. doi: 10.3389/fphy.2022.869537
Received: 04 February 2022; Accepted: 22 April 2022;
Published: 12 May 2022.
Edited by:
Yuping Yang, Minzu University of China, ChinaReviewed by:
Dibakar Roy Chowdhury, Mahindra École Centrale College of Engineering, IndiaCopyright © 2022 Fu, Liu, Chen, Fu and Cui. This is an open-access article distributed under the terms of the Creative Commons Attribution License (CC BY). The use, distribution or reproduction in other forums is permitted, provided the original author(s) and the copyright owner(s) are credited and that the original publication in this journal is cited, in accordance with accepted academic practice. No use, distribution or reproduction is permitted which does not comply with these terms.
*Correspondence: Tie Jun Cui, dGpjdWlAc2V1LmVkdS5jbg==
Disclaimer: All claims expressed in this article are solely those of the authors and do not necessarily represent those of their affiliated organizations, or those of the publisher, the editors and the reviewers. Any product that may be evaluated in this article or claim that may be made by its manufacturer is not guaranteed or endorsed by the publisher.
Research integrity at Frontiers
Learn more about the work of our research integrity team to safeguard the quality of each article we publish.