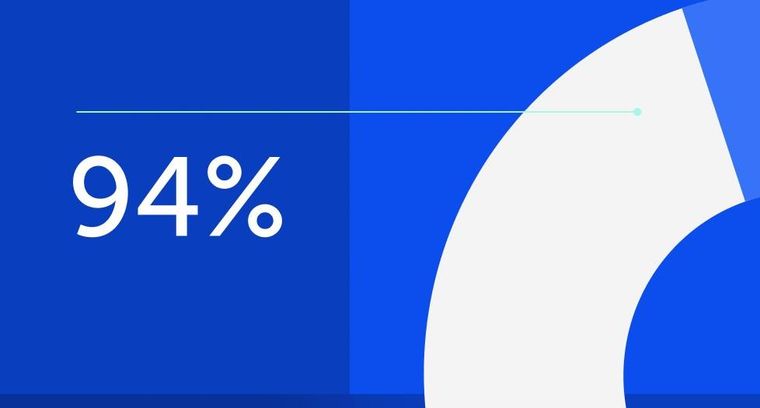
94% of researchers rate our articles as excellent or good
Learn more about the work of our research integrity team to safeguard the quality of each article we publish.
Find out more
BRIEF RESEARCH REPORT article
Front. Phys., 28 June 2022
Sec. Low-Temperature Plasma Physics
Volume 10 - 2022 | https://doi.org/10.3389/fphy.2022.833704
Propylene carbonate (PC) has the advantages of great permittivity, high breakdown strength, and great environmental adaptability. However, the applications of propylene carbonate in pulsed power systems have been restricted by the significant polarity effect (differences between the positive breakdown strength and the negative breakdown strength). In this article, the breakdown characteristics of PC by nano-modification under microsecond pulse have been systematically studied. The results showed that the positive breakdown strength is about twice that of the negative breakdown strength of pure PC. After nano-modification, the negative breakdown strength increases significantly, while the positive breakdown strength decreases. The energy band theory of nanofluids was established.
High power, high repetition frequency, and compactness are important development directions of the pulsed power technology [1–3]. The liquid medium, due to its easy forming capability, great restorative capacity, and high breakdown strength, is widely used in the pulse-forming line as an energy storage medium. The liquid medium mainly includes oil medium and water medium [4–6].
The advantage of a water medium is the high dielectric constant and high energy storage density [7, 8]. However, the water medium requires a deionization system to hold the resistivity, which is against the compactness of the pulsed power system [9]. For oil medium, the resistivity and breakdown strength are relatively high; however, the poor degradation ability limits its wide application [10–12].
Propylene carbonate, with its great permittivity, high breakdown strength, and eco-friendly features, has a great potential in improving the compactness of pulsed power systems, while the strong polarity effect restricts further growth [13, 14]. The polarity effect refers to the difference between positive and negative breakdown strengths. With the development of nanotechnology, researchers try to modify the breakdown characteristic of the liquid medium [15, 16]. In 1998, Segal reported that the breakdown strength of transformer oil increased by more than 50% after nano-modification [17]. It can be seen that nano-modification has a great application prospect for improving the breakdown strength of the liquid medium.
In this article, we used nano-modification to improve the polar effect of propylene carbonate. First, we set up a microsecond pulse breakdown platform composed of a pulse generator and a breakdown test. Second, we prepared TiO2 propylene carbonate nanofluids. Third, the positive and negative breakdown strengths of nanofluids were measured, respectively, and findings showed that the polarity effect was improved effectively after nano-modification. Finally, the liquid medium energy band model was built.
The pulse generator (ARC-01) shown in Figure 1A can output 380 kV under a single pulse. The apparatus was completely satisfactory for the breakdown test. Figure 1B shows the diagram of the breakdown test under microsecond pulses. When the main thyristor was turned on, the secondary capacitor will be boosted by the Tesla transformer. When the voltage of the test cell reaches the breakdown threshold of the liquid medium, the liquid breaks down, and the test cell is turned on. Figure 1C shows the test cell (20 × 20 × 20 cm) with embedded needle-plate electrodes. The diameter of the needle tip was about 0.23 mm, and the diameter of the plate was 40 mm. The distance between the needle and plate was 5 mm, which can be controlled within 0.1 mm by using a high-speed camera (HSFC).
FIGURE 1. Liquid medium microsecond pulse breakdown test platform. (A) Pulse generator; (B) breakdown test circuit diagram; (C) breakdown test piece.
The preparation method of nanofluids comprises the following steps: first, nanoparticles were placed in a vacuum dryer for 24 h. Second, nanoparticles were dispersed into pure PC by using planetary and vertical mixers. Third, the mixture was poured into an ultrasonic pulverizer for 0.5 h. Finally, the nanofluids were obtained by 24 h ball milling. The nanofluids prepared by this method can remain stable for more than 1 week.
At standard conditions (25°C, 0.1 MPa), the positive (the needle electrode was set to ground) and negative (the plate electrode was set to ground) breakdown voltage and current of pure PC and nanofluids were tested. High-voltage probes (Pintech, 1000:1, 220 MHz) were used to measure the voltage, and Pearson coils (Model 6585, 1 V/A, 200 MHz) were used to measure current, respectively.
The negative breakdown waveforms of the pure propylene carbonate and nanofluids are shown in Figure 2. The breakdown waveforms can be divided into two parts: 1) pre-breakdown (the process of voltage and current changes slowly) and 2) breakdown (the process of voltage and current changes rapidly). It can be found that the negative breakdown voltage of pure PC was 33 kV, while the negative breakdown voltage of nanofluids was 45 kV. During the pre-breakdown, the current of pure PC changed significantly. When reaching 2.5 A, the pure PC breakdown started. However, for nano-fluids, the voltage and current changed significantly. Once reaching 5 A, the nanofluid breakdown started. The pre-breakdown time of nanofluids was shorter than that of pure PC.
The positive breakdown waveforms of the pure propylene carbonate and nanofluids are shown in Figure 3. It can be found that the positive breakdown voltage of pure PC (76 kV) was about twice the negative polarity breakdown voltage of pure PC (33 kV), and the pre-breakdown time was about 40 ns. After nano-modification, the positive breakdown voltage of propylene carbonate nanofluids dropped to 51 kV, and the pre-breakdown time extended to about 80 ns.
For polar liquid medium, such as deionized water and propylene carbonate, the Helmholtz double layer shown in Figure 4 at the interface between the electrode and liquid medium was formed. When the electrode is in contact with the liquid medium, the electron cloud will adsorb on the liquid molecules on the electrode, forming the inner Helmholtz layer (IHL) and the outer Helmholtz layer (OHL). The outward layer is the Gouy–Chapman space charge layer which depends on the net space charge density [18].
FIGURE 4. Helmholtz double layer at the interface. (A) Electron cloud, (B) inner Helmholtz layer, (C) outer Helmholtz layer, and (D) space charge layer.
The potential distribution under different applied voltages is shown in Figure 5. Without an applied electric field, electrons will be injected into the electrode by the liquid medium so that the negative charge will accumulate on one side of the electrode in the Helmholtz double layer, resulting in the potential inside the liquid medium being higher. However, with the applied electric field, the positive charge will accumulate on the liquid medium near the cathode, enhancing the electric field, while the negative charge will accumulate on the liquid medium near the cathode, weakening the electric field. Finally, the electric field of the Helmholtz double layer at the cathode will increase with the increase in the applied voltage, and the electric field of the double layer at the anode will decrease with the increase in the applied voltage. So the negative breakdown strength is lower than the positive breakdown strength.
Figure 6 shows the electronic state distribution at the interface between the cathode and the liquid. It can be found that the energy band of the liquid medium at the interface tilts under the applied electric field. The electron near the Fermi level Ef will tunnel, and the hole is generated. Then, the hole will be filled by other electrons with energy release. The energy will most likely be absorbed by free electrons in the non-radiative form, resulting in the free electrons crossing the barrier. In nanofluids, the nanoparticles will introduce a large number of traps in PC. Traps will lift the surrounding potential barrier and effectively inhibit the electrons from entering the liquid, resulting in the increase in the negative polarity breakdown strength.
FIGURE 6. Electronic state distribution at the interface between the cathode and the liquid. (A) Propylene carbonate/electrode interface. (B) Nanofluids/electrode interface.
Figure 7 shows the electronic state distribution at the interface between the anode and the liquid. It can be found that the energy band of the liquid medium at the interface tilts under the applied electric field. The ions will gather near the anode, enhancing the electric field on the anode surface. Similarly, electrons will tunnel. Then, the electron enters the Fermi level and releases energy. The energy will be transferred to electrons distributed below the Fermi level with the generation of holes. Finally, holes will be filled by electrons of the liquid medium in the tunneling effect. Thus, many holes are generated in the liquid and move away from the anode under the electric field. In nanofluids, nanoparticles will significantly increase the hole density near the anode. The hole will accelerate into the PC under the electric field, resulting in a decrease in the positive breakdown strength.
FIGURE 7. Electronic state distribution at the interface between the anode and the liquid. (A) Propylene carbonate/electrode interface. (B) Nanofluids/electrode interface.
In this study, we measured positive and negative breakdown strengths in pure propylene carbonate and nanofluids. Based on the results, the conclusions are drawn as follows:
1. Under the same experimental conditions, the positive breakdown strength is twice the negative breakdown strength of propylene carbonate.
2. After nano-modification, the positive breakdown strength is almost equal to the negative breakdown strength. The polarity effect of propylene carbonate is improved.
3. Nanoparticles can capture electrons effectively, resulting in the increase in the negative breakdown strength of propylene carbonate. Also, nanoparticles can increase the hole density near the anode, resulting in a decrease in the positive breakdown strength of propylene carbonate.
The raw data supporting the conclusion of this article will be made available by the authors, without undue reservation.
ZZ: Conceptualization. DL: Preparation, creation, and presentation of the published work. YH: Investigation.
The authors declare that the research was conducted in the absence of any commercial or financial relationships that could be construed as a potential conflict of interest.
All claims expressed in this article are solely those of the authors and do not necessarily represent those of their affiliated organizations, or those of the publisher, the editors, and the reviewers. Any product that may be evaluated in this article, or claim that may be made by its manufacturer, is not guaranteed or endorsed by the publisher.
We thank the National Natural Science Foundation of China (Grant Nos. 51677190) and the Hunan Outstanding Youth Fund Project (Grant Nos. 2017JJ1005) for supporting this research.
1. Schamiloglu E, Barker RJ, Gundersen M, Neuber AA. Modern Pulsed Power: Charlie Martin and beyond. Proc IEEE (2004) 92(7):1014–20. doi:10.1109/jproc.2004.829058
3. Gundersen MA. Compact High Power Repetitive Pulsed Power Instrumentation[J]. Compact High Power Repetitive Pulsed Power Instrumentation (2004).
4. Butcher M, Neuber AA, Cevallos MD, Dickens JC, Krompholz H. Conduction and Breakdown Mechanismsin Transformer Oil. IEEE Trans Plasma Sci (2006) 34(2):467–75. doi:10.1109/tps.2006.872487
5. Stygar WA, Wagoner TC, Ives HC, Wallace ZR, Anaya V, Corley JP. Water-dielectric-breakdown Relation for the Design of Large-Area Multimegavolt Pulsed-Power Systems[J]. Phys Rev Spec Top - Acc Beams (2006) 9(7). doi:10.1103/physrevstab.9.070401
6. Gerasimov AI. Water as an Insulator in Pulsed Facilities (Review). Instrum Exp Tech (2005) 48(2):141–67. doi:10.1007/s10786-005-0029-7
7. Zhang Z, Zhang J, Yang J. Experimental Study on High Electrical Breakdown of Water Dielectric[J]. Plasma Sci Tech (2005) 7(6):3161–5.
8. Zhang Z, Zhang J, Yang J. Influence of Low Speed Rolling Movement on High Electrical Breakdown for Water Dielectric with Microsecond Charging[J]. Plasma Sci Tech (2006) 8(2):195–7.
9. Joshi RP, Thagard SM. Streamer-Like Electrical Discharges in Water: Part I. Fundamental Mechanisms. Plasma Chem Plasma Process (2013) 33(1):1–15. doi:10.1007/s11090-012-9425-5
10. Yusnida MY, Kamarol M, Ahmad MA. Breakdown voltage characteristic of MO and RBDPO mixture for power transformer insulation. IEEE Colloquium on Humanities, Science and Engineering (CHUSER). IEEE (2013) 64(4):1756–9.
11. Schoenbach K, Kolb J, Xiao S, Katsuki S, Joshi R. Electrical Breakdown of Water in Microgaps[J]. Plasma Sourc Sci Tech (2008) 17(2):24010.
12. Xiao S, Kolb J, Kono S, Katsuki S, Joshi RP, Laroussi M, et al. High Power Water Switches: Postbreakdown Phenomena and Dielectric Recovery. IEEE Trans Dielect Electr Insul (2004) 11(4):604–12. doi:10.1109/tdei.2004.1324350
13. Bolund BF, Berglund M, Bernhoff H. Dielectric Study of Water/methanol Mixtures for Use in Pulsed-Power Water Capacitors. J Appl Phys (2003) 93(5):2895–9. doi:10.1063/1.1544644
14. Shu Xiao X, Kolb JF, Malik MA, Xinpei L, Laroussi M, Joshi RP, et al. Electrical Breakdown and Dielectric Recovery of Propylene Carbonate. IEEE Trans Plasma Sci (2006) 34(5):1653–61. doi:10.1109/tps.2006.876512
15. Wang Z, Zhang Z-c., Zhang J-d. Polarity Effects of Propylene Carbonate on Breakdown Strength in Microsecond Range. Chin J Chem Phys (2015) 28(5):657–60. doi:10.1063/1674-0068/28/cjcp1504080
16. Abdul-Malek Z. Effects of Plasma Treated Alumina Nanoparticles on Breakdown Strength, Partial Discharge Resistance, and Thermophysical Properties of Mineral Oil-Based Nanofluids[J]. Materials (2021) 14.
17. Chiesa M, Das SK. Experimental Investigation of the Dielectric and Cooling Performance of Colloidal Suspensions in Insulating Media[J]. Colloids Surf A: Physicochemical Eng Aspects (2009) 335(1-3):88–97. doi:10.1016/j.colsurfa.2008.10.044
18. Segal V, Hjortsberg A, Rabinovich A, Nattrass D, Raj K. AC (60 Hz) and Impulsebreakdown Strength of a Colloidal Fluid Based on Transformer Oil and Magnetite Nanoparticles[C]. In Conference Record of the 1998 IEEE International Symposium on Electrical Insulation (Cat. No.98CH36239). (1998) 7-10 June 1998. Arlington, VA, USA
Keywords: nanoliquid, pulsed power, streamer, polarity, propylene carbonate
Citation: Zicheng Z, Diangeng L and Yanpan H (2022) Breakdown Characteristic Research on Propylene Carbonate After Nano-Modification. Front. Phys. 10:833704. doi: 10.3389/fphy.2022.833704
Received: 12 December 2021; Accepted: 10 May 2022;
Published: 28 June 2022.
Edited by:
Antonio D’Angola, University of Basilicata, ItalyReviewed by:
Zhi Fang, Nanjing Tech University, ChinaCopyright © 2022 Zicheng, Diangeng and Yanpan. This is an open-access article distributed under the terms of the Creative Commons Attribution License (CC BY). The use, distribution or reproduction in other forums is permitted, provided the original author(s) and the copyright owner(s) are credited and that the original publication in this journal is cited, in accordance with accepted academic practice. No use, distribution or reproduction is permitted which does not comply with these terms.
*Correspondence: Li Diangeng, ZGlhbmdlbmdsaUAxNjMuY29t
Disclaimer: All claims expressed in this article are solely those of the authors and do not necessarily represent those of their affiliated organizations, or those of the publisher, the editors and the reviewers. Any product that may be evaluated in this article or claim that may be made by its manufacturer is not guaranteed or endorsed by the publisher.
Research integrity at Frontiers
Learn more about the work of our research integrity team to safeguard the quality of each article we publish.