- 1School of Information and Communication Engineering, North University of China, Taiyuan, China
- 2Department of Physics, Korea Advanced Institute of Science and Technology, Daejeon, South Korea
- 3School of Semiconductors and Physics, North University of China, Taiyuan, China
To optimize the output of SBS sub-nanosecond pulse compression, two kinds of compact double-cell structures are carried out and compared experimentally. The beam parameters of the compact double-cell structure are calculated theoretically, which provides the selection guidance of structural parameters such as lens focal length and SBS cell size. The dependence of lens parameters and medium parameters on SBS output parameters are experimentally studied. Results show that SBS pulse compression enters the saturation region at a low injection energy with a long focal length lens or a large gain coefficient medium. For compact double-cell setup with one lens, it is easy to obtain narrow pulses for the medium FC40 with a short phonon lifetime. While in setup with two lenses, it is easy to obtain SBS output with a high energy efficiency and narrow pulse width for HT110 medium with a large gain coefficient. The pulse width compression ratio is up to 16 times after optimization. These experimental results can provide references for the experimental design of SBS pulse compression.
1 Introduction
A single-longitudinal mode sub-nanosecond pulse laser with good beam quality has important applications in the fields of fast ignition lasing radiation [1], space debris detection [2, 3], medical laser cosmetology [4], Doppler wind radar [5], and high spatial resolution in LIDAR Thomson scattering diagnostics [6], etc. Stimulated Brillouin scattering (SBS) has proved to be an effective technical means to compress long nanosecond pulses to sub-nanosecond pulses and improve the beam quality of the laser system [7–9]. The compact double-cell structure based on liquid media, with the advantages of a high pulse compression ratio, no high pressure, a small absorption coefficient, and a high laser damage threshold compared with gas and solid media, are commonly and widely used in high-energy sub-nanosecond pulse laser systems [10–14]. SBS pulse compressors with a compact double-cell structure have received considerable attention by employing the medium of perfluorocarbon-compounds (PFCs) liquids and perfluoropolyether (PFPE) liquids.
Many previous theoretical and experimental studies have been conducted to obtain a high-reflected energy efficiency and narrow-pulse width beam laser by optimizing the structure parameters and medium parameters. Feng et al. stated that the interaction length between the Stokes and pump pulses is the key parameter in achieving sub-phonon lifetime pulse compression and experiment was conducted by employing an energy-scalable generator-amplifier setup [12]. Xu et al. used a combination of theoretical modeling and experiments to identify and optimize all critical parameters, including optical configuration, interaction length, intensity matching, choice of gain medium and thermal stability in an energy-scalable generator-amplifier setup [15]. Yoshida et al. achieved a high compression ratio by SBS consisting of two long cells [11]. Guo et al. designed a single-cell experiment to study the energy reflectivity property for the SBS medium of PFCs and PFPE in high peak power laser [16], and Park et al. also measured the SBS characteristics in several liquids [17]. Hasi et al. stated the characteristics of four PFCs media for two-stage SBS pulse compression structure [18]. Therefore, the optimization of the structure parameters and medium parameters for compact double-cell structures is required to study systematically to obtain good SBS compression with a high energy reflectivity.
In this study, to obtain sub-nanosecond pulses with a high reflected energy efficiency, the SBS pulse compression for two types of compact double-cell structure setups are experimentally studied and compared. The focal depth and focal spot size in the compact double-cell structure are calculated, which provides theoretical guidance for structural design such as lens focal length and medium cell size. The experimental setup is described in Section 2. In Section 3, calculation of beam parameters in compact double-cell structure is presented. In Section 4, the experimental results and discussion are presented, and Section 5 concludes the paper. This work has important reference value for the design of SBS experimental structure in high-energy laser systems.
2 Experimental setup
The experimental setup employed in this research is shown in Figure 1. The pumping source is p-polarized light originating from an injection seeded single-longitudinal-mode laser at a fundamental wavelength of 1,064 nm. The pump beam has a pulse width of 10 ns operating at a repetition rate of 10 Hz. The laser propagates from left to right. A half-wave plate (HWP1) and a polarization beam splitter (PBS1) were used to control the input beam energy. Two setups are employed in this research. The input beam is introduced into the SBS amplifier cell through a quarter-wave plate (QWP) and a pair of lenses L1 and L2 with focal lengths of 30 cm and −20 cm, respectively in setup A. The pump beam size is reduced from 8 mm to 5.2 mm using a beam reducer. The laser is focused into the generator cell through a focusing lens L3 with focal lengths of 20 cm or 50 cm, respectively. In setup B, the input beam is introduced into the SBS amplifier cell through a QWP and a lens L4 with focal length of 200 cm and focused into the generator cell through a focusing lens L5 with focal length of 50 cm or 75 cm, respectively.
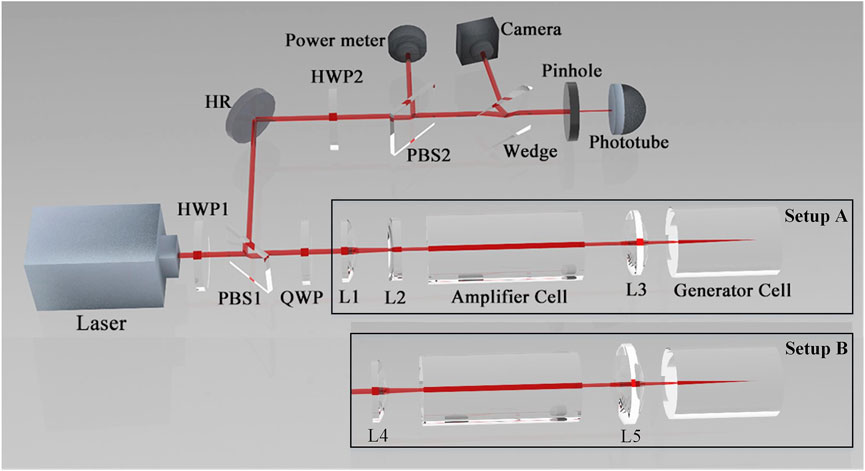
FIGURE 1. Schematic of experimental setups for compact double-cell SBS compressor. QWP, quarter wave plate; HWP1∼2, half wave plate; L1∼5, lens. In setup A, the input beam is introduced into the SBS amplifier cell through a QWP and a pair of lenses L1 and L2 and focused into the generator cell through a lens L3. In setup B, the input beam is introduced into the SBS amplifier cell through a QWP and a lens L4 and focused into the generator cell through a focusing lens L5.
In the process of SBS pulse compression, the phonon lifetime and the gain coefficient are two significant parameters affecting the compressed pulse width. The influence weight between the two parameters, the phonon lifetime and the gain coefficient, is necessary to obtain a good SBS compression [7]. Based on this, we choose two commonly used media of perfluoropolyether liquid HT110 and heavy fluorocarbon liquid FC40 as the objectives due to the large difference between their phonon lifetimes and gain coefficients, which are made by Solvay Company and 3M Company, respectively. Phonon lifetimes of the medium of HT110 and FC40 are .6 ns and .2 ns, respectively. SBS gain coefficients of the medium of HT110 and FC40 are 4.7 cm/GW and 1.8 cm/GW, respectively [7].
3 Calculation of beam parameters in compact double-cell structure
To avoid damaging the lens and window mirror of SBS cell in the design of compact double-cell structure, it is necessary to select the lens and SBS cell with appropriate parameters. Taking setup B as an example, the focal depths and focal spot sizes within the generator cell are theoretically calculated. It is generally believed that the generation position of the Stokes beam is at the focal point within the generator cell. The focal depth indicates the interaction length between the pump beam and the Stokes beam, which affects the compressed pulse width of SBS. It is easy to cause optical breakdown and permanent damage to the optical window mirror of SBS cell if the beam size of the focus spot in the generator cell is too small. Therefore, it is important to select the generator cell with the appropriate physical length.
In the compact double-cell structure, the contour maps of the focal depth and focal spot radius in the generator cell are shown in Figure 2. Physical parameters used for the simulation are as follows. The pump beam quality of M2 is 2. The radius of the pump beam spot is 4 mm, and the distance from the waist position of the pump beam to the lens of L4 is 50 cm. The physical length of the amplifier cell is 100 cm, and the distance between the lens L5 and the window mirror of generator cell is 5 cm. The refractive index of SBS liquid medium is 1.28. The optical wavelength is 1,064 nm.
According to ABCD matrix theory, the variations of focal depth and focal spot radius with the focal lengths of lens L4 and L5 can be calculated as shown in Figure 2 f4 and f5 represent the focal lengths of lens L4 and L5, respectively. It can be seen from Figure 2A that, within the range of focal length f4 of lens L4 less than 200 cm, the focal depth within the generator cell is jointly affected by the focal lengths of lens L4 and L5. When focal length of lens L4 is greater than 200 cm, the focal depth of the generator cell is more sensitive to the focal length of lens L5. In Figure 2B, the focal spot size varies greatly with the focal length of lens L5, and it is not sensitive to the focal length of lens L4. When the focal length of lens L5 is fixed, the focal depth and the focal spot size gradually become stable with the increase of the focal length of lens L4. According to the theoretical calculation, a generator cell with a physical length of 80 cm is required when the focal length f5 of the lens L5 is 50 cm, and a generator cell with a physical length of 20 cm is required when the focal length f5 of lens L5 is 15/20 cm.
4 Experimental results and discussion
To optimize the output parameters of SBS pulse compression and obtain the optimization rules, the experiments for setup A and setup B are respectively studied. A comparison of the output parameters under different focal lengths of lens L3 and SBS media is shown in Figure 3 using setup A. The experimental results show that the energy extraction efficiency improves and the compressed pulse width becomes narrow with an increase of the input energy. In this experiment, the focal length of lens L3 is 20 cm or 50 cm, respectively. Figure 3A shows the reflected energy efficiency under different focal lengths of lens L3 and media. The average energy efficiencies are 74.8%, 68.5%, and 77.1% for the cases of L3 lens of 50 cm with FC40 medium, 50 cm with HT110, and 20 cm with HT110, respectively, at the pump energy of 70 mJ. Comparing the cases of lens L3 with focal lengths of 20 cm and 50 cm in HT110 medium, the reflected energy efficiency of the shorter focusing lens of 20 cm is obviously greater than that of the focusing lens of 50 cm. This is because as the focal length of lens L3 increases, the focal spot size in the generator cell also increases, which causes the decrease of the pump power density at the generation position of the Stokes seed beam under the same pumping conditions, thus leading to the reduction of the energy reflectivity. For the setup A with a focal length of 50 cm and HT110 medium, the reflected energy efficiency enters the saturation region when the injected energy is 30 mJ, which is not conducive to further improvement of the reflected energy. The corresponding compressed pulse width using HT110 is shown in Figure 3B, with the increase of injected energy, the SBS compressed pulse width gradually narrows and finally enters the saturation region. The minimum pulse widths are 649 ps ± 14 ps at the pump energy of 50 mJ and 654 ps ± 9 ps at 70 mJ for the lens focal lengths of 50 cm and 20 cm, respectively. With the focal length of L3 lens increasing, the compressed pulse width becomes narrower as the injection energy and enters the saturation region at the lower pump energy. The longer focal length of the focusing lens, the lager the focal depth within the generator cell, which indicates that the longer interaction length between the pump beam and Stokes beam is easier to obtain the narrow compressed pulse width. According to the experimental results, the pump power density and interaction length both affect the energy efficiency and compressed pulse width. Setup A with short focusing lenses is easy to obtain a high reflection energy, while with long focusing lenses is easy to obtain narrow compressed pulse width.
With the focusing lens L3 of 50 cm, compared with the medium of FC40 and HT110 in Figure 3A, the energy efficiency obtained by the two media is the same at the pump energy of 40 mJ. When the injected energy is less than 40 mJ, the reflected energy efficiency with HT110 is higher than that of FC40 medium. When the injected energy is greater than 40 mJ, the reflected energy used FC40 medium is greater than that of HT110. The experimental results show that it is easy to obtain a high energy efficiency in the case of a medium with a high gain coefficient. So the energy efficiency with HT110 medium is higher than that of FC40 at a low injection pump power. With the increase of injection pump power, the energy efficiency for HT110 with a high gain coefficient enters the gain saturation region earlier than the case for FC40 with a low gain coefficient, then the energy efficiency of FC40 is higher than that of the medium HT110. The relationship between the compressed pulse width and the injected energy for different media is shown in Figure 3B. At low injection energy, the compressed pulse width using HT110 medium is slightly narrower than that of FC40, this is because the medium with a high gain coefficient can effectively amplify the front part of the Stokes pulse. With the increase of injection energy, the compressed pulse width using FC40 medium is narrower than that of HT110, which is because during the amplification process of the Stokes pulse, the front part and back part are both amplified resulting in the broadening of the compresses pulse. Under the injection energy of 70 mJ, the average pulse widths measured in FC40 and HT110 media are 620 ps ± 12 ps and 660 ps ± 26 ps, respectively. For compact double-cell setup A, it is easy to obtain narrow pulses for the medium FC40 with a short phonon lifetime.
In setup B, the focal length of lens L4 is 200 cm, and it is 50 cm or 75 cm for the lens L5, respectively. The SBS output parameters under different lenses and media employing setup B are shown in Figure 4. Figure 4A illustrates the reflected energy efficiency under different lens focal lengths and media. The average energy efficiencies are 71.3%, 75.5%, and 68.3% for the cases of lens group of 200–50 cm with FC40 medium, 200–50 cm with HT110, and 200–75 cm with HT110, respectively, at the pump energy of 70 mJ. Comparing the cases of lens L5 with focal lengths of 50 cm and 75 cm in HT110 medium, the reflected energy efficiency with a short focusing lens of 50 cm is larger than that of the focusing lens of 75 cm. For the case with lens focal length of 75 cm, the reflected energy efficiency enters the saturation region when the injected energy is 30 mJ, which is not conducive to further improvement of the reflected energy. The corresponding compressed pulse widths are shown in Figure 4B. The minimum pulse widths are 626 ps ± 27 ps ns at the pump energy of 45 mJ and 619 ps ± 16 ps at 60 mJ for the lens focal lengths of 50 cm and 75 cm, respectively, and the pulse width compression ratio is up to 16 times. With the increase of injected energy, the SBS compressed pulse width gradually narrows and finally enters the gain saturation region. In the case of 75 cm, the pulse width become widening when the injected energy exceeds 30 mJ.
With the focusing lens L5 of 50 cm, compared with the medium of FC40 and HT110 in Figure 4A, the reflected energy used HT110 medium is much higher than that of FC40 medium at the same pump energy. The relationship between the compressed pulse width and the injected energy under different media is shown in Figure 4B. Under the injection energy of 45 mJ, the average pulse widths measured in FC40 and HT110 media are 653 ps ± 25 ps and 626 ps ± 27 ps, respectively. The compressed pulse width of HT110 medium is slightly narrower than that of FC40. This is because the medium with a high gain coefficient can effectively amplify the front part of Stokes pulse. Therefore, in compact double-cell setup B, it is easy to obtain a high energy efficiency and narrow pulse width for the medium HT110 with a large gain coefficient.
5 Conclusion
In this study, experiments in the compact double-cell structure are conducted to optimize the output parameters of SBS pulse compression. Firstly, the focal depth and focal spot size in the compact double-cell structure are calculated, which provides theoretical guidance for the structural parameter design such as lens focal length and SBS cell size. Then, the influence of the lens and medium parameters on output parameters of SBS pulse compression is experimentally studied for setup A and setup B, respectively. For setup A, it is easy to obtain a high reflection energy efficiency with a short focal length lens, while easy to obtain a narrow compressed pulse width with a long focal length lens. As the focal length of focusing lens increases, the injection energy at which the compressed pulse width enters the saturation region becomes small, and the compressed pulse width is narrower. The experimental results show that it is easy to obtain a high energy efficiency in the case of a medium with a large gain coefficient. With the increase of injection pump power, the energy efficiency for HT110 with a high gain coefficient enters the gain saturation region earlier than the case for FC40 with a low gain coefficient. For compact double-cell setup A, it is easy to obtain narrow pulses for the medium FC40 with a short phonon lifetime. However, in compact double-cell setup B, it is easy to obtain a high energy efficiency and narrow pulse width for the medium HT110 with a large gain coefficient. The pulse width compression ratio is up to 16 times after optimization. These experimental results can provide output optimization guidance for the experimental design of SBS pulse compression in high-energy laser systems.
Data availability statement
The original contributions presented in the study are included in the article/supplementary material, further inquiries can be directed to the corresponding author.
Author contributions
HW conducted the experiment and wrote the manuscript, SS did the simulation, and GW checked the writing.
Funding
National Natural Science Foundation of China (62105303); Fundamental Research program of Shanxi Province (20210302124026); Shanxi Scholarship Council of China (2020-102); Scientific and Technological Innovation Programs in Shanxi (2020L0265); 2021 China-Korea Young Scientist Exchange Program.
Conflict of interest
The authors declare that the research was conducted in the absence of any commercial or financial relationships that could be construed as a potential conflict of interest.
Publisher’s note
All claims expressed in this article are solely those of the authors and do not necessarily represent those of their affiliated organizations, or those of the publisher, the editors and the reviewers. Any product that may be evaluated in this article, or claim that may be made by its manufacturer, is not guaranteed or endorsed by the publisher.
References
1. Zhang F, Cai HB, Zhou WM, Dai ZS, Shan LQ, Xu H, et al. Enhanced energy coupling for indirect-drive fast-ignition fusion targets. Nat Phys (2020) 16(7):810–4. doi:10.1038/s41567-020-0878-9
2. Quinn MN, Jukna V, Ebisuzaki T, Dicaire I, Soulard R, Summerer L, et al. Space-based application of the CAN laser to LIDAR and orbital debris remediation. Eur Phys Journal-Special Top (2015) 224(13):2645–55. doi:10.1140/epjst/e2015-02577-5
3. Li HW, Zhao B, Jin LW, Wang DM, Gao W. Flat gain over arbitrary orbital angular momentum modes in Brillouin amplification. Photon Res (2019) 7(7):748–53. doi:10.1364/Prj.7.000748
4. Baumler W, Weiss KT. Laser assisted tattoo removal - state of the art and new developments. Photochem Photobiol Sci (2019) 18(2):349–58. doi:10.1039/c8pp00416a
5. Zhang X, Dai J, He T, Zhao C, Huang J, Li X, et al. Overview of the Chinese lidar satellite development. In: LIDAR imaging detection and target recognition 2017. Washington, United States: International Society for Optics and Photonics (2017). doi:10.1117/12.2296275
6. Gowers CW, Brown BW, Fajemirokun H, Nielsen P, Nizienko Y, Schunke B. Recent developments in LIDAR Thomson scattering measurements on JET (invited). Rev Scientific Instr (1995) 66(1):471–5. doi:10.1063/1.1146321
7. Wang H, Cha S, Kong HJ, Wang Y, Lu Z. Minimizing cross sectional pulse width difference between central and edge parts of SBS compressed beam. Opt Express (2019) 27(2):1646–59. doi:10.1364/OE.27.001646
8. Cao C, Wang Y, Bai Z, Li Y, Yu Y, Lu Z. Developments of picosecond lasers based on stimulated Brillouin scattering pulse compression. Front Phys (2021) 9:524. doi:10.3389/fphy.2021.747272
9. Kang Z, Fan Z, Huang Y, Zhang H, Ge W, Li M, et al. High-repetition-rate, high-pulse-energy, and high-beam-quality laser system using an ultraclean closed-type SBS-PCM. Opt Express (2018) 26(6):6560–71. doi:10.1364/OE.26.006560
10. Bai ZX, Yuan H, Liu ZH, Xu PB, Gao QL, Williams RJ, et al. Stimulated Brillouin scattering materials, experimental design and applications: A review. Opt Mater (2018) 75:626–45. doi:10.1016/j.optmat.2017.10.035
11. Yoshida H, Hatae T, Fujita H, Nakatsuka M, Kitamura S. A high-energy 160-ps pulse generation by stimulated Brillouin scattering from heavy fluorocarbon liquid at 1064 nm wavelength. Opt Express (2009) 17(16):13654–62. doi:10.1364/oe.17.013654
12. Feng C, Xu X, Diels JC. High-energy sub-phonon lifetime pulse compression by stimulated Brillouin scattering in liquids. Opt Express (2017) 25(11):12421–34. doi:10.1364/OE.25.012421
13. Shin JS, Kong HJ. Phase fluctuation of self-phase-controlled stimulated Brillouin scattering waves via K8 glass. Opt Commun (2012) 285(13-14):2977–9. doi:10.1016/j.optcom.2012.02.055
14. Zhang A, Qiao S, Sheng L, Huang L, Liu Z, Ju J, et al. Study on external cavity diode laser with a wide mode-hopping free tuning range. Front Phys (2022) 10:1093179. doi:10.3389/fphy.2022.1093179
15. Xu X, Feng C, Diels JC. Optimizing sub-ns pulse compression for high energy application. Opt Express (2014) 22(11):13904–15. doi:10.1364/OE.22.013904
16. Guo X, Hasi W, Zhong Z, Jin C, Lin D, He W, et al. Research on the SBS mediums used in high peak power laser system and their selection principle. Laser Part Beams (2012) 30(4):525–30. doi:10.1017/S0263034612000390
17. Park H, Lim C, Yoshida H, Nakatsuka M. Measurement of stimulated Brillouin scattering characteristics in heavy fluorocarbon liquids and perfluoropolyether liquids. Jpn J Appl Phys Part 1-Regular Pap Brief Commun Rev Pap (2006) 45(6A):5073–5. doi:10.1143/Jjap.45.5073
Keywords: high-energy laser, stimulated Brillouin scattering, pulse compression, sub-nanosecond pulse, beam parameter
Citation: Wang H, Shan S and Wang G (2023) Experimental optimization of compact double-cell stimulated Brillouin scattering pulse compressor. Front. Phys. 10:1115557. doi: 10.3389/fphy.2022.1115557
Received: 04 December 2022; Accepted: 20 December 2022;
Published: 08 March 2023.
Edited by:
Shuo Liu, Hebei University of Technology, ChinaReviewed by:
Liwen Sheng, Ceyear Technologies Co., Ltd, ChinaHui Yang, Southwest Jiaotong University, China
Copyright © 2023 Wang, Shan and Wang. This is an open-access article distributed under the terms of the Creative Commons Attribution License (CC BY). The use, distribution or reproduction in other forums is permitted, provided the original author(s) and the copyright owner(s) are credited and that the original publication in this journal is cited, in accordance with accepted academic practice. No use, distribution or reproduction is permitted which does not comply with these terms.
*Correspondence: Hongli Wang, wanghl@nuc.edu.cn