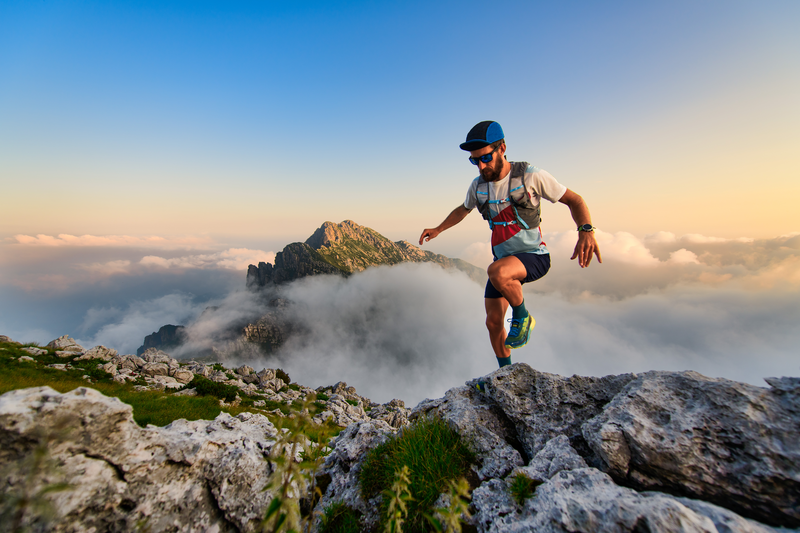
95% of researchers rate our articles as excellent or good
Learn more about the work of our research integrity team to safeguard the quality of each article we publish.
Find out more
EDITORIAL article
Front. Phys. , 17 November 2022
Sec. Soft Matter Physics
Volume 10 - 2022 | https://doi.org/10.3389/fphy.2022.1079979
This article is part of the Research Topic Multiscale Soft Tissue Biomechanics and Cell Mechanobiology: Towards Coupling Extracellular Biophysical Cues and Cellular Function View all 6 articles
Editorial on the Research Topic
Multiscale Soft Tissue Biomechanics and Cell Mechanobiology: Towards Coupling Extracellular Biophysical Cues and Cellular Function
Soft matter biomechanics and cell mechanobiology are rapidly developing interdisciplinary academic fields integrating biophysics, biology, and engineering sciences. These fields aim to uncover the behavior of living soft materials for emerging needs in basic and translational research. Soft matter biomechanics apply mechanical principles to understand the behavior of viscoelastic biological systems such as cells, extracellular matrix (ECM), and tissues. Mechanobiology focuses on the influences of the structural microenvironment and physical forces on molecules, cells and tissues. Specifically, cell mechanobiology studies the basic mechanisms of how cells generate mechanical forces and respond to extracellular mechanical stimuli. The forces and mechano-chemical properties can regulate a broad range of cell functions such as proliferation, polarization, morphogenesis, motility, differentiation, and death [1,2]. ECM structure was shown to be another factor impacting cellular behavior [3,4]. Understanding the underlying mechanisms of cell-ECM interplay is important because of its regulatory role in normal tissue development and homeostasis[5,6]. Cell-ECM interactions are of particular importance in disease development, as the deregulation of matrix mechanical and structural responses can be associated with cancer metastasis, cardiovascular disorders, tissue aging, and fibrosis [7–11].
The ECM is a complex macromolecular network that forms a microenvironment for cells and provides them with structural and mechanical support. It is composed of glycosaminoglycans, proteoglycans, glycoproteins, and fibrous proteins such as collagen, elastin, fibronectin, and laminin [12,13]. There is also a variety of other molecules in the ECM, such as cytokines, chemokines, growth factors, matrix degradation enzymes, and inhibitors [14,15]. All these molecules are crucially important for defining cellular phenotype and functionality. The cell-ECM interaction is mediated via various types of receptors, the majority of which comprise integrins, discoidin domain receptors, and syndecans [16–18].
This Research Topic collects five valuable papers covering four original in vitro and in vivo studies and a minireview.
Vasquez-Hidalgo et al. study cellular force generation in response to chemical and mechanical cues using a biophysical model. A better understanding of the mechanisms of traction force generation between cells and substrates is needed since these forces are higher for metastatic cancer cells and, hence, could be considered as promising biomarkers. The authors show that several components influence traction force generation: the number of active myosin complexes, substrate stiffness, the kinetics of bonds between cells and substrates, and mechanical reinforcement at the adhesion sites. It is concluded that the impulse, magnitude, and duration of a force-generating event are the key limiting factors in traction stress.
Asadishekari et al. fabricate porous tunable ECM scaffolds comprised of two of the most abundant components of the ECM: collagen and fibronectin proteins (fn). The authors show how cell adhesive and invasive properties can be controlled by thermally switching the fn conformations assessed using Förster Resonance Energy Transfer techniques. It is demonstrated that tuning architecture and mechanics of these scaffolds can direct cell functionality and matrix deposition. The system can potentially be used as a 3D platform mimicking physiological and tumorous conditions.
Le Cerf et al. study an even more simplified model of the ECM consisting only of collagen. They use atomic force microscopy to obtain information about the nanomechanical properties of their model tissue and complement those with Fourier-transform infrared (FTIR) spectroscopy of the matrix. The authors show a bi-modal distribution for the Young’s model of collagen and discuss possible underlining structural mechanisms. They report that nano-mechanical evaluations are more sensitive to the effects of cross-linking on collagen properties compared to FTIR spectroscopy. Further studies are required to establish a relationship between the chemical and physical properties of the collagen matrix.
Tong et al. review the progress made in the manufacturing of microfluidic scaffold materials. Three-dimensional bioprinting methods hold great promise for organ biofabrication and regenerative medicine. The authors identify several promising developments, in particular towards scaffolds with addressable plumbing (i.e., with valves). They conclude that even though progress has been made, materials with the desired characteristics (biocompatible, biodegradable, flexible, photo-crosslinkable, transparent) have not been designed yet.
He et al. study renal fibrosis, the final manifestation of chronic kidney disease characterized by an excessive accumulation and deposition of ECM components. Renal ECM, a complex network of collagens, elastin, and several glycoproteins and proteoglycans, provides structural and mechanical support to renal cells and regulates the differentiation of neighboring cells in renal aging and fibrosis. It is a dynamic structure undergoing remodeling, and increases its stiffness during fibrosis. The authors constructed kidney ECM gels of several stiffnesses mimicking ECMs of healthy and diseased animals. By combining in vitro measurements of ECM stiffening by renal fibroblasts with in vivo studies in rats, they conclude that the increase in stiffness is mutually causal with the activation of the Yes-associated protein (YAP) pathway. Polydatin is shown to regulate this YAP-related mechano-transduction pathway and hence is a potential therapeutic strategy.
The collected papers highlight how soft matter biophysics research, by applying advanced experimental and modeling approaches, contributes to improving human health. Recent progress in imaging techniques permits an unprecedented level of structural details that together with the application of biophysical and biochemical functional assays can provide quantitative information about how cellular behavior is affected by the biophysical properties of the extracellular environment such as matrix viscoelasticity, its structural characteristics and stability. Such studies can shed light on the coupling of biology, chemistry, and mechanics (the “triple-point”) in describing cellular behavior. We anticipate further rapid progress in this field.
All authors listed have made a substantial, direct, and intellectual contribution to the work and approved it for publication.
The authors declare that the research was conducted in the absence of any commercial or financial relationships that could be construed as a potential conflict of interest.
All claims expressed in this article are solely those of the authors and do not necessarily represent those of their affiliated organizations, or those of the publisher, the editors and the reviewers. Any product that may be evaluated in this article, or claim that may be made by its manufacturer, is not guaranteed or endorsed by the publisher.
1. Jansen KA, Donato DM, Balcioglu HE, Schmidt T, Danen EHJ, Koenderink GH. A guide to mechanobiology: Where biology and physics meet. Biochim Biophys Acta - Mol Cel Res (2015) 1853:3043–52. doi:10.1016/j.bbamcr.2015.05.007
2. Marastoni S, Ligresti G, Lorenzon E, Colombatti A, Mongiat M. Extracellular matrix: A matter of life and death. Connect Tissue Res (2008) 49:203–6. doi:10.1080/03008200802143190
3. Miron-Mendoza M, Seemann J, Grinnell F. The differential regulation of cell motile activity through matrix stiffness and porosity in three dimensional collagen matrices. Biomaterials (2010) 31:6425–35. doi:10.1016/j.biomaterials.2010.04.064
4. Paul CD, Mistriotis P, Konstantopoulos K. Cancer cell motility: Lessons from migration in confined spaces. Nat Rev Cancer (2017) 17:131–40. doi:10.1038/nrc.2016.123
5. Hansen CE, Qiu Y, McCarty OJT, Lam WA. Platelet mechanotransduction. Annu Rev Biomed Eng (2018) 20:253–75. doi:10.1146/annurev-bioeng-062117-121215
6. Kolahi KS, Mofrad MRK. Mechanotransduction: A major regulator of homeostasis and development. WIREs Mech Dis (2010) 2:625–39. doi:10.1002/wsbm.79
7. Niu L, Cheng B, Huang G, Nan K, Han S, Ren H, et al. A positive mechanobiological feedback loop controls bistable switching of cardiac fibroblast phenotype. Cell Discov (2022) 8:84–16. doi:10.1038/s41421-022-00427-w
8. Phillip JM, Aifuwa I, Walston J, Wirtz D. The mechanobiology of aging. Annu Rev Biomed Eng (2015) 17:113–41. doi:10.1146/annurev-bioeng-071114-040829
9. Schroer AK, Merryman WD. Mechanobiology of myofibroblast adhesion in fibrotic cardiac disease. J Cel Sci (2015) 128:1865–75. doi:10.1242/jcs.162891
10. Takahashi K, Kakimoto Y, Toda K, Naruse K. Mechanobiology in cardiac physiology and diseases. J Cel Mol Med (2013) 17:225–32. doi:10.1111/jcmm.12027
11. Yu W, Sharma S, Rao E, Rowat AC, Gimzewski JK, Han D, et al. Cancer cell mechanobiology: A new frontier for cancer research. J Natl Cancer Cent (2022) 2:10–7. doi:10.1016/j.jncc.2021.11.007
12. Frantz C, Stewart KM, Weaver VM. The extracellular matrix at a glance. J Cel Sci (2010) 123:4195–200. doi:10.1242/jcs.023820
13. Schaefer L, Schaefer RM. Proteoglycans: From structural compounds to signaling molecules. Cell Tissue Res (2009) 339:237–46. doi:10.1007/s00441-009-0821-y
14. Hynes RO. The extracellular matrix: Not just pretty fibrils. Science (2009) 326:1216–9. doi:10.1126/science.1176009
15. Sainio A, Järveläinen H. Extracellular matrix-cell interactions: Focus on therapeutic applications. Cell Signal (2020) 66:109487. doi:10.1016/j.cellsig.2019.109487
16. Harburger DS, Calderwood DA. Integrin signalling at a glance. J Cel Sci (2009) 122:159–63. doi:10.1242/jcs.018093
17. Leitinger B, Hohenester E. Mammalian collagen receptors. Matrix Biol (2007) 26:146–55. doi:10.1016/j.matbio.2006.10.007
Keywords: mechanobiology, extracellular matrix (ECM), soft tissue biomechanics, microfluidics, biofabrication, renal fibrosis, traction force, matrix deposition
Citation: Kim OV, Li X and Baljon ARC (2022) Editorial: Multiscale soft tissue biomechanics and cell mechanobiology: Towards coupling extracellular biophysical cues and cellular function. Front. Phys. 10:1079979. doi: 10.3389/fphy.2022.1079979
Received: 25 October 2022; Accepted: 03 November 2022;
Published: 17 November 2022.
Edited and reviewed by:
Jasper Van Der Gucht, Wageningen University and Research, NetherlandsCopyright © 2022 Kim, Li and Baljon. This is an open-access article distributed under the terms of the Creative Commons Attribution License (CC BY). The use, distribution or reproduction in other forums is permitted, provided the original author(s) and the copyright owner(s) are credited and that the original publication in this journal is cited, in accordance with accepted academic practice. No use, distribution or reproduction is permitted which does not comply with these terms.
*Correspondence: Oleg V. Kim, b2xlZ2tpbUBwZW5ubWVkaWNpbmUudXBlbm4uZWR1JiN4MDIwMGE7, b2xlZ2tpbUB2dC5lZHUmI3gwMjAwYTs=
Disclaimer: All claims expressed in this article are solely those of the authors and do not necessarily represent those of their affiliated organizations, or those of the publisher, the editors and the reviewers. Any product that may be evaluated in this article or claim that may be made by its manufacturer is not guaranteed or endorsed by the publisher.
Research integrity at Frontiers
Learn more about the work of our research integrity team to safeguard the quality of each article we publish.