- College of Electronic Science and Technology, National University of Defense Technology, Changsha, China
The global navigation satellite system is a high-precision radio navigation system based on artificial satellites. Among the factors affecting the positioning accuracy of the navigation satellite system, the multipath signal is the most significant and challenging to eliminate. Furthermore, the multipath environment of the receiver is also becoming more complex, and it seriously threatens the measurement accuracy and stability of the receiver. The multipath mitigation technology is also continuously improved and developed in practical application. This paper firstly introduces the concept and characteristics of multipath signals, and summarizes the influence of multipath signals on navigation satellite systems from two aspects of code tracking loop and carrier tracking loop. Furthermore, the existing multipath mitigation technology is summarized in four stages: signal system design, antenna design, baseband signal processing, and navigation data processing. Finally, according to the summary of the existing technology and the new demand, the future development direction of multipath suppression technology is prospected.
1 Introduction
Global Navigation Satellite System (GNSS) is an all-round, all-weather, all-time and high-precision radio navigation and timing system based on artificial satellites. The main functions of GNSS include positioning, navigation, and timing, it has been widely used in many fields. Such as, it can be used to confirm waypoints, routes, and tracks in geographic data collection [1]. Compared with traditional measurement methods, satellite navigation has higher accuracy, easier operation, and smaller instrument size [2] in high-precision surveying. It can also achieve high-precision and high-stability time synchronization functions [3]. In-vehicle navigation systems combine GNSS with automotive electronic technology to provide drivers with real-time road guidance [4, 5]. In modern aviation and navigation systems, GNSS has also played a huge role in surveying, navigation, automatic dependent surveillance, terrain awareness early warning systems [6, 7].
The GNSS generally consists of three independent parts: the space constellation, the ground monitoring, and the user equipment. The ground station realizes satellite orbit determination by receiving satellite signals. The satellite receives the orbit information transmitted by the ground station and then completes the rebroadcast. The user receiving equipment realizes its precise positioning by receiving satellite signals. The influence of multipath signal is mainly aimed at the user receiver, so the working principle of the receiver will be briefly introduced below. As shown in Figure 1, GNSS receiver generally includes the following three functional modules: 1) Antenna and front-end processor; 2) Baseband signal processor; 3) Navigation data processor.
In general, the signal processing flow in the GNSS receiver is as follow [8]:
1) The antenna receives the signal from the free space. The front-end processor converts the carrier frequency from the radio frequency (RF) to the intermediate frequency (IF) through down-conversion. The analog intermediate frequency signal is also digitally sampled and input to the baseband signal processing module;
2) The baseband signal processor realizes the demodulation of the received signal by calculating the correlation value between the Pseudo-Random Binary Sequence (Pseudo-code), carrier reference signal generated internally, and the digital sampling signal output by the RF Front-End of the receiver. Then the calculated correlation samples are accumulated and sent to the ranging processor in order to realize the tracking of the code phase and carrier phase;
3) When the carrier phase is tracked and locked, the navigation data processor demodulates the navigation message. The receiver can calculate the position and velocity of the satellite and the ambiguity (such as ionospheric delay, etc.) in the propagation time of the satellite signal. Then, according to the estimated signal parameters in the tracking loop and navigation information, measurement information such as GNSS pseudo-ranges can be calculated to achieve positioning.
There are many factors that can affect the positioning accuracy of the GNSS, including satellite and receiver clock errors, satellite orbit errors, ionospheric and tropospheric propagation delays, Earth rotation, relativistic effects and receiver noise, radio frequency interference and multipath, etc. Among them, the multipath signal is a significant error source, which is difficult to eliminate. Many error sources can be eliminated by differential technology. Such as ionospheric delay. The ionospheric error refers to the time delay of the GNSS signal caused by the influence of the ionosphere. It is difficult to calculate directly through mathematical formula. As for dual-frequency receiver, if the measurement noise is not considered, the pseudo-distance obtained by the two frequency measurements of the receiver is only different in the ionospheric delay part, so the dual-frequency receiver can reduce the measurement error caused by the ionosphere through differential technology. However, since the multipath signal error changes with the receiver environment in real-time, it cannot be effectively eliminated by differential technology.
There are also many effective suppression algorithms for interference signals. Many mature technologies, including onboard anti-jamming technology [9], auxiliary anti-jamming technology [10, 11], and receiver anti-jamming technology [12–14], are currently under further development.
The multipath signal is the interference signal formed by the line of sight (LOS) signal reflected (scattered) one or more times by other objects. Due to the actual complex environment of the receiver, solar panels on the satellite, tall buildings, and trees on the ground, etc., may become the source of the multipath signal for receivers. So the signal received by the antenna of the user equipment is a composite signal of the LOS signal and multiple reflected (scattered) signals. Due to the influence of the multipath signal, the amplitude, code phase, and carrier phase of the LOS signal may be distorted to a certain extent. The receiver’s acquisition and tracking of the LOS signal will also be affected, resulting in measurement errors. In severe cases, the deformed autocorrelation function and the superimposed wave signal with drastic changes in power will cause the receiver to lose lock [15, 16].
The research on multipath signals in GNSS can be traced back to the early 1970s [17]. After the Global Positioning System (GPS) was officially opened and put into use, NovAtel has successively proposed a series of multipath mitigation technologies [18, 19], which are also widely used in GPS systems. However, due to the complex characteristics of multipath signals. It is difficult to eliminate the errors caused by multipath signals entirely. To this day, multipath mitigation is still the most important research content in reducing measurement errors in GNSS systems.
2 The characteristics of multipath signal
2.1 Mathematical model of multipath signal
In general, multipath signals are mainly formed by the reflection of objects on the ground and around the antenna, the reflection of satellites, and the scattering of the atmospheric propagation medium. Figure 2 shows a simplified scenario of multipath signal generation.
Since the multipath signals originate from the reflection (scattering) of the LOS signal, there are the following essential characteristics [20]:
1) Compared with the LOS signal, the multipath signals travel a longer path during propagation, so their arrival time is always later than the LOS signal;
2) When reflected by the passive reflector, the signal always has an inevitable energy loss, and the longer propagation path will also make the energy loss of the multipath signal greater than that of the LOS signal. So when the LOS signal is not blocked (This paper only considers this situation), the signal power of the multipath signal is lower than the LOS signal;
3) The wavelength of the satellite navigation signal is generally at the decimeter level, and the multipath delay is much larger than its carrier wavelength. The phase change of the reflected wave caused by the multipath can be regarded as a random number with a value of [0, 2π].
Due to the complex environment where the receiver antenna is located, it is not easy to describe the multipath signal with a general mathematical model. Although there are some models that established on the multipath characteristics in specific scenarios such as forests, cities, and suburbs [21–25]. By analyzing the modeling methods of the references, these models are too complex to popularize. The reasons are: 1) These methods require long-term measurement in a particular area; 2) The characteristics of multipath signals will change slowly with time; 3) The receivers are used in many scenarios, and each scenario’s reflective surface features and quantity are very different. According to the above characteristics, the multipath signal is described in terms of attenuation, propagation delay, and carrier phase difference. In general, we can express the reception of the direct signal r(t) as [26]:
where A is the LOS signal amplitude, P(t) = R(t)D(t),
Then the reception of the ith multipath signal
where αi is attenuation coefficient of the ith multipath signal, and αi < 1, δi is the propagation delay of ith reflected signal, φi is the total phase change generated by the ith reflected signal at each reflector surface.
Assuming the GNSS receiver can receive M reflection signal, the received signal can be expressed as [26]:
2.2 Impact of multipath signal on global navigation satellite system
The signal transmitted by GNSS can be divided into three levels: carrier, pseudo-code, and data code. The data code is first modulated to pseudo-code, then modulated on a sinusoidal carrier through the digital baseband modulation method. Finally, the modulated signal is transmitted by the antenna. For precise positioning, the GNSS receiver generates two basic range measurements, pseudo-range and carrier phase, for each satellite. The multipath signals influence GNSS mainly through these two basic measurements.
2.2.1 Impact on the code tracking loop
The aim of the code tracking loop is to copy a pseudo-code whose phase is consistent with the pseudo-code phase of the received signal, and to strip the pseudo-code from the GNSS signal by correlation operation. Delay-Locked loop (DLL) is a form of code tracking loop, the principle of which is shown in Figure 3.
In the pseudo-range measurement, the code phase is directly measured by the receiver, which is obtained by the pseudo-code generator and pseudo-code correlator on the internal code tracking loop of the receiver. The transmission time of the signal can be obtained through the code phase, and the pseudo-range value can be calculated according to the reception time of the receiver and the transmission time. The traditional code signal tracking loop in the receiver adopts a DLL (As shown in Figure 3), it calculates the correlation value between the early-prompt-late three-way local pseudo-code signal and the received signal. By adjusting the duplicate pseudo-code phase, the code tracking loop makes the correlation value output by the early correlator and the late correlator equal. Finally, a non-coherent tracking loop is used to track the pseudo-code. The multipath signal will distort the correlation function. Therefore, the measurement accuracy of the GNSS receiver will be affected, and in severe cases, the receiver may lose lock.
As shown in Figure 4, in the absence of the influence of multipath signals, the auto-correlation function of the pseudo-code of the receiver is an isosceles triangle [27]. When multipath signals are received, the peak of the auto-correlation function may be shifted, resulting in the center of the early correlators and late correlator sampling point deviates from the arrival time of the LOS signal. The code phase measurement error results from this. The actual offset is related to the delay, amplitude, and phase parameters of the multipath signals relative to the LOS signal, this is also the reason for the measurement error. Generally speaking, the error caused by the multipath signal to the code phase is in the order of meters [28], which can significantly affect the positioning solution result.
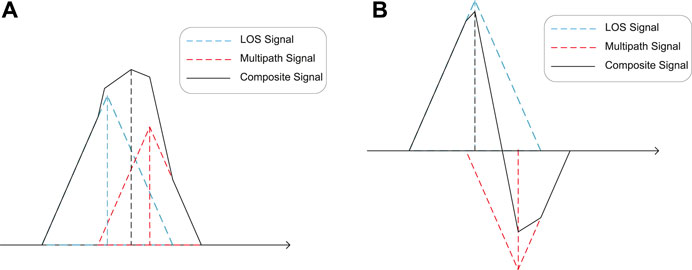
FIGURE 4. The auto-correlation function of receiver pseudo-code with a single multipath signal. (A) In-Phase multipath signal. (B) Reversed-Phase multipath signal.
2.2.2 Impact on carrier tracking loop
The purpose of carrier tracking loop is to copy the same carrier signal as the received satellite carrier signal, so that the received signal can be easily separated from the carrier. Phase-Locked Loop (PLL) is a form of carrier tracking loop, the principle of which is shown in Figure 5. By constantly adjusting the phase of the output signal, the phase of the output signal and the input signal is kept consistent at all times.
In the carrier phase measurement, the carrier phase of the receiver and the satellite are directly measured at the same time. The distance from the satellite to the receiver can be calculated using the phase difference between the two and the carrier wavelength. Nevertheless, it cannot directly output the positioning result because of the weekly ambiguity. In practical applications, the code phase is used to obtain a rough pseudo-range value, and the carrier phase provides a basis for higher-precision pseudo-range measurement.
As shown in Figure 6, when there is a multipath signal, the GNSS receiver directly receives the composite signal of the LOS signal and the multipath signal, and the composite signal has a phase change relative to the LOS signal θe. Then when the composite signal is correlated with the copied pseudo-code inside the receiver, the measurement error (i.e., phase change) θe of the composite signal relative to the LOS signal is:
where R(τ) represents the pseudo-code auto-correlation function. In general, the error caused by multipath to the carrier phase is in the order of centimeters, much smaller than the error caused by the code phase [28]. The error caused by multipath to the carrier phase will directly affect the positioning accuracy and the solution of the integer ambiguity. Finally, it will affect the actual performance of the whole receiver [29, 30].
If the difference between
It can be seen that when φI is in 0° ∼ 180°, the superposition result of the LOS signal and the multipath signal enhances the power of the received signal. When varphiI is in 180° ∼ 360°, the superposition result reduces the power of the received signal. Since the measurement error θe of the multipath signal is a random variable, the amplitude and phase of the composite signal (received signal) are also unstable. It even causes the receiver to lose lock in severe cases.
2.3 Summary
In this section, taking the multipath signal generated by the reflection of the ground and objects around the antenna as an example, a simplified mathematical model of multipath signal reception is established by the attenuation, propagation delay, and carrier phase difference parameters of multipath signals. Secondly, according to the model, the possible influence of multipath signals on the code tracking loop and carrier tracking loop is analyzed, which proves the importance of multipath suppression technology research.
3 Multipath mitigation technology
Existing multipath mitigation technology can be divided into the following four stages:
3.1 The design of new signal system
This stage focuses on designing in order to improve the ability of GNSS signal in multipath mitigation. It is an improvement before GNSS signal transmission. By designing the new signal system, there are two ways to achieve multipath mitigation: one is to improve the modulation mode of the signal; the other is to choose a more appropriate pseudo-code type and carrier frequency. They will be introduced in detail below:
3.1.1 Signal modulation for multipath mitigation
The signal needs to be modulated and transformed into a suitable signal before it can be transmitted in the channel. This section provides ideas for designing new multipath suppression methods by comparing the generation principles and multipath mitigation performance of the classical and new signal systems.
3.1.1.1 Classical signal system
Traditional satellite navigation systems such as the C/A signal of GPS and the B1I signal of BDS both use Binary Phase Shift Keying (BPSK) or Quadrature Phase Shift Keying (QPSK) modulation. In the actual processing, the QPSK signal can be regarded as two BPSK signals to be processed separately. This section will mainly analyze the BPSK signal.
As shown in Figure 7, in the navigation system, first of all, the data code d(t) needs to be directly modulated to the pseudo-code c(t) to obtain the spread spectrum signal s(t). Finally, the digital modulation method is used to modulate s(t) on the high-frequency carrier with the center frequency wc. The traditional digital modulation methods are BPSK and QPSK modulation, the specific implementation principle can be found in the book [31].
Then the time-domain expression of the BPSK signal in the navigation system is:
where P represents the signal power, and φ0 represents the initial carrier phase.
As mentioned in Section 2.2, for BPSK modulated signals, when performing pseudo-range measurement of the receiver, the local correlation operation is performed on the pseudo-code modulated signal, so the auto-correlation and cross-correlation characteristics of the pseudo-code are very important [32], the calculation of its auto-correlation function is as follows,
The auto-correlation function of the pseudo-code only has a single main peak at the zero point, and there is no apparent secondary peak. Secondly, it has been fully applied in the early navigation satellite system due to the simple implementation of BPSK modulation, constant modulus, and high-frequency band utilization.
3.1.1.2 New signal system
In 2001, Jhon W. Betz proposed to use binary offset carrier modulation to replace the BPSK modulation that is widely used in GNSS [33], which became the beginning of a new signal system for GNSS. The
BOC modulation is a new modulation method proposed to meet the signal spectrum separation, which not only enables the sharing of frequency bands between different satellite navigation systems but also has better-ranging performance and multipath mitigation performance than BPSK modulation. The modulation process [35] is shown in Figure 8. Different from the classical BPSK signal, the BOC modulation is obtained by re-modulating the sine or cosine sign function after pseudo-code modulation.
The time-domain expression is as follows:
The frequency-domain expression is as shown below [36]:
It can be seen from the Eq. 9 that the power spectrum of the BOC signal can be regarded as the result of moving the power spectrum of the traditional BPSK signal up and down, respectively. According to the analysis and simulation of the power spectral density and auto-correlation function of the BOC signal [37], taking BOC(1,1) and BOC(6,1) modulated signals as examples, their auto-correlation function and power spectrum characteristics are shown in Figures 9, 10, respectively.
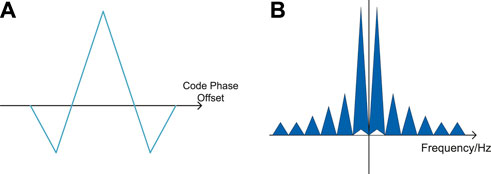
FIGURE 9. Auto-correlation function and power spectrum of BOC(1, 1) signal. (A) Auto-correlation function. (B) Power spectrum.
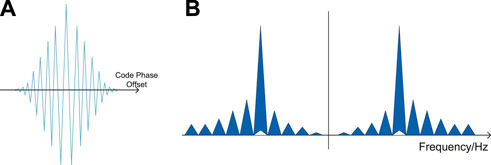
FIGURE 10. Auto-correlation function and power spectrum of BOC(6, 1) signal. (A) Auto-correlation function. (B) Power spectrum.
It can be seen from the figure that the power spectrum of the BOC signal is far away from the center frequency of the original BPSK, which realizes the spectrum sharing of the signal. Secondly, the narrower main peak of the auto-correlation function also enables the BOC signal to have higher precision code tracking performance and better multipath mitigation performance [38]. However, higher requirements are placed on the receiver acquisition and tracking due to the increase in the number of peaks of the auto-correlation function. The new signal obtained by mixing different modulation orders with a specific power ratio has a narrower main peak of auto-correlation and stronger multipath mitigation ability. It is also the idea of designing new modulation signals such as TMBOC, QMBOC, and CBOC.
BOC modulation has a series of advantages such as simple implementation, the narrow main peak, high positioning accuracy, strong anti-interference ability, large effective signal bandwidth, strong multipath mitigation performance, and strong code tracking performance [39]. However, during the synchronization of the BOC signal, it is effortless to lock on the wrong correlation edge peak, resulting in errors in the pseudo-range measurement, which is generally referred to as tracking ambiguity [40]. At the same time, when the modulation order becomes larger, the frequency spectrum at both ends of the IF is too far apart, and time delays of different magnitude will be generated during the receiving process of the receiver, thereby affecting the measurement and positioning of the receiver.
3.1.2 Pseudo-code and carrier selection for multipath mitigation
Generally speaking, the pseudo-code with a higher code rate has a more robust multipath mitigation performance for the long delay, which is also why the BOC signal has better multipath mitigation performance by being added with sub-carrier square wave modulation after the pseudo-code modulation. Secondly, selecting pseudo-code series with good auto-correlation and cross-correlation characteristics and the period as long as possible is also significant for multipath mitigation [41].
The carrier frequency determines the electromagnetic signal’s reflection (scattering) characteristics, So choosing an appropriate carrier frequency can significantly improve the multipath mitigation capability of the system. For example, Signals of different frequencies also have different degrees of diffuse reflection when they are reflected, the diffuse reflection will further weaken the multipath effect [42, 43].
The core idea of realizing multipath mitigation through the signal design of the new signal system is to increase the code rate of the signal to make the auto-correlation function as narrow as possible. It also makes this method effective against pseudo-code and carrier phase multipath. However, the disadvantage is that the multipath mitigation capability is limited, and it is difficult to directly modify the signal system of GNSS, which also dramatically limits the use of such methods. For example, BOC modulation was not initially designed to achieve multipath mitigation but to realize the isolation of military and civilian codes and the sharing of spectrum.
3.2 The anti-multipath antenna
The function of the receiver antenna is to receive the signal broadcast by the satellite and recover the original signal by removing the clutter and interference signal as far as possible. There are many mature to suppress interference signal [44, 45] and deception signal suppression [46] method, also has obtained a good effect, the following will introduce the primary antenna of the multipath signal suppression measures.
3.2.1 Polarized antenna
The polarized antenna is the most common, simple, and effective anti-multipath antenna. The LOS signal is a right-hand circularly polarized wave, and the signal after the odd number of reflections is a left-hand circularly polarized wave. According to the right-hand, circularly polarized antenna can reject the left-hand circularly polarized signal; the signal received by the right-hand circularly polarized antenna only contains the LOS signal and the even number of reflected signals. It makes the polarized antenna effectively realize the multipath mitigation effect [47]. Figure 11 shows a typical circularly polarized antenna.
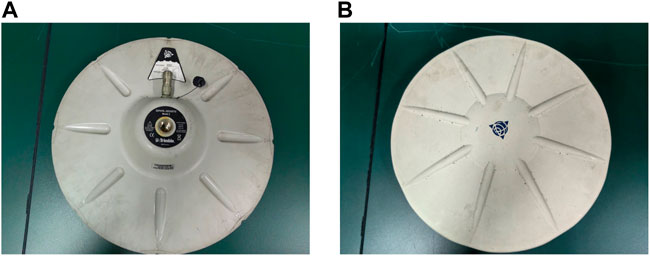
FIGURE 11. A typical circularly polarized antenna. (A) The reverse side of the antenna. (B) The front side of the antenna.
The dual polarized antenna provides another degree of freedom to distinguish LOS signals and composite signals based on a small amount of increase in the complexity of the receiver. It makes dual polarized antenna [48] in many areas has made the application. On this basis, the dual polarized antenna is generalized to multipath suppression, such as literature [49] based on maximum likelihood estimation to design a kind of double polarization multipath suppression algorithm—this algorithm in alleviating the short delay multipath better than single polarized antenna. Literature [50] adds dual-polarization technology to baseband signal processing and achieves a better multipath suppression effect. Although the dual-polarization technique has a better multipath suppression effect, it still faces some problems, such as the need for higher quality polarized antennas, more output channels, correlators, and computational loads.
3.2.2 Choke ring antenna
In GNSS, the more mature technology is the choke ring antenna. The design idea of the choke ring antenna is to reduce the antenna’s low elevation angle and backward gain by designing the antenna pattern [51]. So that the gain of the receiving antenna in the direction where the multipath signal arrives is small, and the number of reflected signals can be reduced. Effectively suppresses multipath signals from below ground level. Figure 12 shows a typical 3D choke ring antenna.
There have been many choke designs [52, 53] for reducing the cross-polarized component (multipath signal). However, under low elevation conditions, both the cross-polarized signal and the right-hand circularly polarized signal are suppressed, i.e., The LOS signal will also be weakened. In order to solve this problem, literature [54] uses the polarizer characteristics of omnidirectional antennas to design an anti-multipath antenna that can provide the maximum right-polarized and the minimum cross-polarization gain, which reduces the influence of the choke ring antenna to the LOS signal. The three-dimensional choke ring antenna proposed in [55] can alleviate the problem of insufficient antenna gain under low elevation angle conditions while preserving the stable phase center, amplitude, phase, and other properties of the signal. At present, the choke ring antennas have been widely used in GNSS of various countries.
Secondly, under the radiation pattern requirements, there are many kinds of structures for the anti-multipath antenna to achieve the miniaturization of the antenna and reduce the cost of the antenna. Such as Circular Patch Antenna [56], Four-L Probe Coupling Patch Antenna [57], Compact Dual-band Substrate Integrated Choke Ring Antenna [58], Annular Slot the Loaded Ground Plane Antenna [59], and the Integrated Multipath-Limiting Antenna [60] designed for local area enhancement system.
3.2.3 Other multipath mitigation measures
In addition to polarized antennas and choke ring antennas, there are many antenna technologies that can achieve multipath mitigation. For example, the spatial and temporal information of the signal can be extracted by the antenna array to estimate and suppress the multipath [61, 62]. Alternatively, the antenna array can be used to adjust the antenna direction by estimating the direction of the incoming wave to reduce the receive gain for multipath signals [63]. However, such algorithms are more complex, costly, and less versatile.
For large antennas with high-performance requirements, the multipath mitigation can also be considered from the location and erection of the antenna, such as: erecting the antenna in a high or empty place, far away from high-rise buildings, mooring, a large area of vegetation, and other environments easy to generate signal reflection, laying absorbing materials on the ground around the antenna.
Furthermore, adaptive beamforming has been widely used in multipath mitigation of antennas. Such as, The adaptive beamforming method [64] is proposed based on a Fractional Order Bidirectional Least Mean Square (FOBLMS), which achieves an excellent multipath mitigation effect. Since the anti-multipath antenna can directly attenuate the power of the multipath signal, it has the same effect on pseudo-code phase multipath and carrier phase multipath [65, 66]. In particular, there are some specific carrier phase multipath mitigation methods [67]. Secondly, using the characteristics of the receiving antenna can reduce the reception of multipath signals to a certain extent. However, the characteristic of anti-multipath antennas is that they can only suppress multipath signals at a specific angle. The rest of the multipath signals will enter the following signal processing stage. Therefore, it is necessary to process the multipath signals entering the receiver from other angles to reduce the impact of multipath signals on receiver measurement accuracy.
3.3 Multipath mitigation in baseband signal processing
The GNSS antenna receives the composite signal of the LOS signal and the multipath signal, so it is also the composite signal that is directly matched with the local pseudo-code and carrier during baseband signal processing, which will cause the receiver to generate measurement errors. Comparing the simulation analysis results of BPSK and BOC modulated signals under the two technologies of narrow correlation and high-resolution correlator [68], it can be found that the multipath mitigation effect will be of significant difference when the multipath mitigation technology is applied to different signal systems. Therefore, this section will analyze and compare their performance under different signal systems while summarizing various multipath mitigation techniques. This stage focuses on signal processing in order to eliminate multipath signals from received signals. It is an improvement after GNSS signal reception.
There are usually two ways to achieve multipath mitigation in baseband signal processing. One is to reduce the sensitivity of multipath signals by improving the correlator or discriminator of the receiver loop; the other is to use a statistical signal processing method based on measurement data to estimate signal parameters (LOS or multipath signals). These multipath mitigation techniques will be described in detail below.
3.3.1 Multipath mitigation technique based on improving correlator/discriminator
3.3.1.1 Narrow correlation technique
The correlator spacing used in the receiver code tracking loop of the traditional correlator is generally one chip, and the multipath mitigation ability is weak, which is difficult to meet the needs of users for high-precision positioning [69]. According to Section 2.2.1, the receiver obtains the measured code phase by equalizing the correlation results output by the early correlator and the late correlator. If the correlation interval of the correlator is smaller, the early-late tracking point will be closer to the peak value of the correlation function. At the peak value of the correlation function, the influence of the multipath signals on the phase measurement is the least [70], and the narrow correlation technology is designed based on this idea [71]. By reducing the correlation interval, the multipath error can be reduced to a maximum of one-tenth of that of a conventional correlator. However, the cost of reducing the correlation interval is wider pre-correlation bandwidth, higher sampling rate, and higher digital signal processing rate [71]. Secondly, when the correlator interval is reduced, the operating range of the code ring discriminator will be reduced. As a result, the dynamic performance of the code tracking loop is further reduced.
Due to the narrowing of the main peak range of the correlation peak of the BOC signal, the linear pulling range of the traditional narrow correlation technique is minimal when applied to the high-order BOC signal [72]. Secondly, the BOC signal suffers from tracking ambiguity caused by side peaks in the auto-correlation function. In using narrow correlation techniques, a wrong locking point in the code tracking loop discriminator will likely lead to missed or false detections. Therefore, the multipath mitigation performance of the traditional narrow correlation technology in the BPSK signal system is better than that of the BOC signal.
3.3.1.2 Code correlation reference waveforms technology
Young Lee first proposed Code Correlation Reference Waveforms (CCRW) technology. A better code ring discriminator can be obtained by designing correlators and particular code reference waveforms to sharpen cross-correlation functions so that we can obtain better multipath mitigation performance [73]. Compared with the structure of the traditional receiver, the receiver no longer generates early and late local pseudo-code signals. However, it generates a series of reference waveforms in the form of gate wave combination to correlate with the received signals, thereby constructing the desired discrimination error curve to achieve better multipath mitigation performance [74]. In the gate wave design, the desired discriminant error curve is generally constructed first; then the local reference waveform is constructed according to the discriminant error curve [75]. The typical local reference waveforms are W1, W2, W3, and W4. According to the different reference waveform generation methods, they are divided into two types: generation at chip flip (W1) and generation at chip-by-chip (W2, W3, W4) [75], the structure of each waveform is shown in Figure 13.
CCRW technology has the advantages of simple structure, relatively low hardware resource consumption, and real-time solid performance [76]. However, the performance of the CCRW algorithm is affected by the non-ideal characteristics of the RF channel, filter bandwidth, and anti-interference algorithm. The local reference waveform design needs to consider these non-ideal factors. Just like the narrow correlation technique, the tracking ambiguity may also exist if the W2, W3, and W4 reference waveforms of CCRW are directly applied to BOC signals. Therefore, the multipath mitigation ability of the general CCRW technique in the BPSK signal system is better than the BOC signal system.
In order to solve the ambiguity problem of CCRW in the BOC signal, the literature [77] introduced a series of bipolar reference waveforms based on the W2 code-related reference waveform structure to solve the tracking ambiguity problem in BOC (1,1). At the same time, the multipath mitigation capability is also improved.
3.3.1.3 Double DELTA correlator
Double DELTA Correlator uses two pairs of correlators to compute and form correlation curves locally. Based on this idea, many multipath mitigation techniques have been derived, including Strobe Technique, Pulse Aperture Correlator (PAC) Technique, and High-Resolution Correlator (HRC) Technique.
Strobe Technology [78] is the most representative of the Double DELTA correlator technology, put forward in 1996 by Astech company. The multipath mitigation is achieved by setting two groups of correlators with unequal correlation intervals and modifying the phase detection function. The correlator interval of the broad correlation group is twice that of the narrow correlation group. Because the Strobe correlator multipath mitigation for short delay multipath signal is sensitive [79, 80], and can suppress the majority of long time delay multipath signals. It has been widely used in GNSS. After analysis [81], When the Strobe correlator suppresses the error caused by the multipath signals, the multipath suppression performance under the two signal systems of BPSK and BOC is better than that of the narrow correlation technology [72]. However, since it requires multiple correlator calculations, the implementation is relatively complicated. Furthermore, the problem of tracking ambiguity still exists in Strobe technology, so the traditional Strobe technology is more suitable for BPSK modulation.
In order to solve the problem of tracking ambiguity in BOC signal, literature [82] proposed a narrow correlation-assisted Double-DELTA tracking method, which can detect the false-lock problem of Double-DELTA, making it suitable for BOC signal. The improved method enhances the robustness and compatibility of the tracking loop and reduces hardware resource consumption and algorithm complexity.
In addition, the double DELTA Correlator includes PAC technology and HRC technology. The PAC technology uses two early correlators, two late correlators, and one immediate correlator to compensate for the distorted correlation peak jointly. The phase detector function is a linear function of two pairs of early, and late narrow correlators [19].
HRC technology achieves multipath mitigation by changing the local reference waveform signal [83], which is also a CCRW technology. Its local reference waveform is shown in W1 of Figure 11, and multiple correlators are also used to achieve better multipath mitigation. However, the disadvantage of these techniques is that the implementation is complicated due to the need for multiple correlators.
In order to solve the problem of high complexity, literature [84] proposed a time-division multiplexing Strobe scheme for TMBOC modulation. It generates two time-multiplexing strobes to track the BOC(1,1) and BOC(6,1) components, simplifying the hardware structure and improving the tracking performance in thermal noise and multipath. Literature [85] adds a specific compensation factor to the traditional Strobe phase detector formula, uses the TK operator as the detection module, and constructs a new multipath suppression strategy so that the receiver has good performance for short-delay in-phase multipath signals.
3.3.1.4 Other multipath mitigation techniques
In addition, many multipath mitigation techniques are based on baseband signal processing. Such as the Multipath Elimination Technique (MET) [86], by setting two groups of narrow correlators on both sides of the correlation peak, the slopes on both sides of the correlation peak are obtained to reflect the code phase tracking error caused by multipath. It is worth noting that MET is also called Early Late Slope (ELS) in some literature. Finally, by adding a compensation factor h to the phase detector, the loop can be tracked at the correct position to achieve the purpose of multipath mitigation. Figure 14 shows a schematic diagram of the principle of MET. According to the geometric relationship, the slopes on both sides a1, a2 can be calculated, and then we get the difference between the early and late correlation values
Since early GNSS generally adopts BPSK modulation, the current multipath mitigation technology for BPSK signals is relatively mature. However, these multipath mitigation techniques generally have the problem of tracking ambiguity under BOC signals, especially for high-order BOC signals. There are many side peaks in the correlation peak, and the amplitude difference from the main peak is slight, and the code tracking loop is very easy. Falsely locked to the side peak, the tracking ambiguity is the most severe [87]. The multi-peak auto-correlation function can usually be solved by Dual-BPSK tracking technology. On this basis, literature [88] proposed a multipath mitigation method based on an offset correlator for BOC signals, which can effectively suppress multipath signals under the premise of low complexity.
Table 1 summarizes the characteristics of these technologies, ranking them according to the complexity of the implementation structure (from simple to complex): CCRW
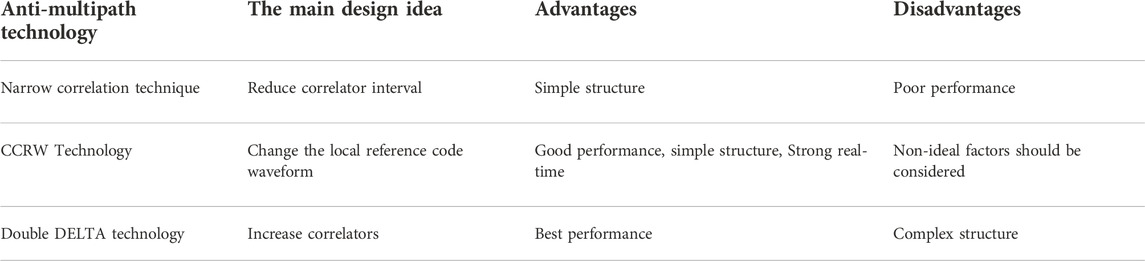
TABLE 1. Characteristics of improved multipath mitigation techniques based on correlator/discriminator.
Moreover, the literature [89] has developed a neural network-based DLL for multipath mitigation in a GPS receiver. That manipulates equally spaced samples of autocorrelation functions and is trained using a statistical distribution model of multipath time delay and power attenuation. It provides a new idea for the integration development of artificial intelligence algorithms and multipath mitigation technology.
3.3.2 Multipath mitigation technique based on signal parameter estimation
3.3.2.1 Multipath estimation delay locked loop technology
Multipath Estimation Delay Locked Loop (MEDLL) [18, 90] was proposed by NovAtel in 1995. It uses the structure of multiple correlators to sample a series of correlation values and obtain the correlation peak envelope and then uses the maximum likelihood estimation criterion to obtain the estimation and direct access of the parameters of the LOS signal. The correlator output of the signal, and finally realize the feedback of the tracking loop by using the phase detector and loop filter in the traditional tracking loop. Based on this basic idea, multipath suppression techniques have been derived. Such as Multipath Mitigation Technology (MMT) [91] and Vision Correlator [92, 93] Technology, the advantages of these methods is excellent multipath mitigation performance, and the maximum theoretical performance limit can be reached. However, better performance requires higher sampling frequency and more correlators, which greatly increases the system complexity and computational cost. Secondly, these estimation methods need long-term integration to obtain a good signal-to-noise ratio. Therefore, the application of these techniques is limited to static conditions.
3.3.2.2 Coupled amplitude delay locking loop technology
Coupled Amplitude Delay Locking Loop (CADLL) [94] is developed based on MEDLL technology. CADLL uses multiple parallel tracking units to separate the LOS signal from the multipath signal through the feedback loop. Then parallel tracking units track the code phase, carrier phase, and amplitude of the received signal. When the multipath signal is estimated, CADLL subtracts the multipath component from the input composite Signal to eliminate the influence of the multipath on the LOS signal [95]. Compared with MEDLL technology, CADLL technology requires a shorter integration time and lower system complexity; it also has some advantages, such as better noise performance and can track dynamic multipath signals. However, in some cases where the carrier-to-noise power ratio is very weak, the performance of CADLL will deteriorate sharply [94].
3.3.2.3 Other multipath mitigation techniques
In addition, there are many multipath mitigation methods based on parameter estimation. For example, literature [96] proposed a multipath signal delay estimation algorithm based on correlation model. The algorithm takes the correlation output of the received signal and the local C/A code as the model. It can accurately estimate the delay of LOS and multipath signals when the signal delay is unknown. Literature [97] proposed a multipath mitigation technology based on fast orthogonal search. It can achieve a higher code tracking effect in urban, canyon, and other multipath signal-intensive environments. The multipath mitigation performance was significantly better than the traditional multipath mitigation algorithm.
In general, the performance of multipath mitigation technology based on signal parameter estimation is better than traditional methods, and it can suppress the carrier phase multipath. However, there are few practical applications due to its large amount of calculation and poor real-time performance. The performance of the BPSK or BOC Signal System often depends on the correlator and phase detector used in the loop.
3.4 Multipath mitigation for navigation data processing
Due to the complex environment of the receiver and the different characteristics of the corresponding multipath signal, the pseudo-range measurement noise of the receiver has different statistical characteristics. The multipath mitigation in navigation data processing usually adopts the data post-processing method to process the Multipath errors in pseudo-range or carrier phase observations.
3.4.1 Multipath mitigation based on statistical signal processing
Since multipath signals have a specific temporal correlation, smoothing pseudo-range measurements over multiple epochs generally cannot reduce multipath errors in pseudo-range measurements. However, smoothing pseudo-ranges can achieve multipath mitigation using carrier phase measurements with less multipath error. Literature [98] proposed a method to correct the multipath error in differential phase observation using the signal-to-noise ratio information. This method can eliminate most low-frequency multipath signals and remove the high-frequency multipath signals through smoothing or Kalman [99].
3.4.2 Multipath mitigation based on empirical model
For some fixed scenarios, such as detection stations or fixed large-scale antennas, Since the environment in which multipath signals are generated is unchanged, certain regularities will appear over time. Based on this, a multipath error model is established by analyzing a large amount of multipath error data. This model, such as sidereal filtering technology [100] and wavelet analysis technology [101, 102], Empirical Mode Decomposition technique [103] et al, can be used to correct the multipath error in future measurements. Although the multipath mitigation effect of this method is good, it requires prior knowledge and data; it is only suitable for some specific models. Since it is a post-processing method, it is generally only suitable for monitoring stations and other situations where the surrounding environment does not change much.
In the latest research, adaptive literature Kalman filter has been applied to multipath mitigation and achieved excellent effect [104]. As improvements, literature [105] uses Unscented Kalman Filter (UKF) and the combination of wavelet transform and particle filter for multipath suppression.
These methods usually do not need to improve the internal signal processing unit of the receiver but can be implemented by software processing of the output data of the receiver. It also determines the characteristics of their effective mitigation of pseudo-code phase multipath and carrier phase multipath simultaneously [106, 107]. Secondly, its performance depends largely on the redundancy of measurement, the level of measurement noise, and a long observation. Therefore, an appropriate processing method for the measurement data can be selected according to the difference in the statistical characteristics of the measurement noise under different multipath signal receiving conditions to suppress the multipath error.
3.5 Summary
This section summarizes some classic multipath mitigation technologies in GNSS, as shown in Table 2. The research mainly focuses on the four stages of signal system design, antenna design, baseband signal processing, and navigation data post-processing. They apply to different application scenarios.
As shown in Table 2, the existing multipath mitigation methods are mainly reflected in the following four stages:
1) Reduce the main peak width of the auto-correlation function through the new signal system design so as to reduce the influence of the multipath signal on the satellite LOS signal, thereby reducing the measurement error;
2) According to the characteristics of the inconsistency between the incident direction of the multipath signal and the LOS signal, design an antenna that can reduce the reception of the multipath signals, and through the reasonable site selection and erection of the antenna, the generation of multipath signals can be reduced from the source;
3) During baseband signal processing, by designing correlators and phase detectors with different characteristics or using parameter estimation methods to reduce the influence of multipath signals on measurement accuracy;
4) When processing navigation data (Information Processing), the statistical characteristics of multipath errors are used to reduce the influence of multipath signals.
4 Future development direction of anti-multipath technology
This section will point out the possible development directions of future multipath mitigation technology according to the four stages summarized in the previous section.
4.1 Signal design for new system of low orbit satellite
The carrier frequency largely determines the propagation, reflection, and refraction characteristics of the corresponding electromagnetic wave signal [42]. However, the carrier frequency that the current navigation satellite system can select is minimal. Furthermore, the International Telecommunication Union also uniformly allocated the spectrum resources. So choosing an appropriate carrier frequency can improve the multipath mitigation performance, but this is usually difficult to exploit.
In general, choosing a pseudo-code series with a higher code rate, good auto-correlation characteristics, cross-correlation characteristics, and a period as long as possible can help reduce multipath errors [108]. A new pseudo-code series needs to be designed with the development of low-orbit satellite systems. Due to the sharp increase in the number of low-orbit satellites, a longer pseudo-code cycle is required to distinguish more satellites using code division multiple access; a large signal bandwidth is required to meet the communication needs, which are all in line with the requirements for multipath mitigation. It indirectly realizes the multipath mitigation. Further, it can also be considered to realize multipath mitigation from the design of the signal modulation method of the low-orbit satellite. Therefore, the new LEO satellite signal system design is a huge opportunity and challenge for multipath mitigation technology.
4.2 Miniaturization design of anti-multipath antenna
Although the multipath mitigation effect of anti-multipath antennas in GNSS is imposing, most of the anti-multipath antennas have the disadvantages of considerable size and heavy weight. With the further development of the GNSS, the receiver has a higher and higher demand for antenna miniaturization, and traditional anti-multipath antennas are usually challenging to take into account the two requirements of size and performance at the same time. Many researchers have done related work to solve this problem, such as miniaturizing the corrugated structure by implementing multiple folds or slits inside the corrugation and then improving the choke ring to obtain a miniaturized antenna [109]. Even so, this is still a direction that requires much research. Therefore, how to design a miniaturized and low-cost anti-multipath antenna without (less) reducing the multipath mitigation performance is a primary development direction of the anti-multipath antenna in the future.
4.3 Correlator improves/improves the efficiency of multipath mitigation algorithms
BOC signals have many advantages and have been widely used in GNSS, but applying traditional multipath mitigation techniques directly to the BOC signals will have ambiguity problems. Therefore, how to better realize multipath mitigation of BOC signals is an important research direction in the future.
In general, the method based on signal parameter estimation has better multipath mitigation performance than the traditional correlator, but due to its large amount of calculation, poor real-time performance, and high-performance requirements of the receiver. It dramatically limits its practical application scenarios. Currently, many researches focus on using new technologies in the field of signal processing to improve the computational efficiency of algorithms [110], which achieved good results. Therefore, there are two directions for further development of this type of technology: one is to reduce computing requirements through algorithm design; the other is to apply new technologies in the computer or signal processing field to improve computing efficiency.
4.4 Multipath mitigation algorithm that can adapt to highly dynamic environment
These methods generally use the statistical characteristics of multipath errors to reduce the influence of multipath signals, the disadvantage is that they require a long time of observation data to estimate the parameters of the statistical model of measurement noise. However, in some practical environments, the parameter changes of multipath signals have high dynamic characteristics and no noticeable change rules. The corresponding statistical characteristics of measurement noise will also change with time and environment [111, 112], limiting such methods in practical use. Therefore, designing a multipath mitigation algorithm with better performance or high adaptability to dynamic environment is of great significance.
4.5 Application of other new technology
Artificial intelligence is a new technical science that studies and develops theories, methods, technologies, and application systems for simulating, extending, and expanding human intelligence. In recent years, many great achievements have been made in artificial intelligence, which has accelerated the integration of artificial intelligence technology and technology in various fields. At present, artificial intelligence has achieved many applications in medicine, financial trade, education and other fields [113–115]. If artificial intelligence technology can be applied to multipath cancellation technology, it may further reduce the impact of multipath signals on receivers. Through the investigation, it can be found that: some researchers have applied artificial intelligence technology to the field of multipath signal prediction [116–118] and achieved outstanding results, and neural networks can also be used to simulate the effects of multipath signals on GNSS correlation output [119, 120], which also provides a new idea for future multipath mitigation techniques. Besides, a deep learning-aided spatial discriminator has also been proposed to compensate for the limitations of traditional beamforming methods, enabling multipath mitigation during signal reception [121].
In addition, the neural network can also be used in the design of low-orbit satellite pseudocodes to achieve better cross-correlation and auto-correlation performance. It can be used in the design of multipath suppression antennas to reduce the reception of multipath signals. It is used in the baseband signal processing algorithm to solve the problem that the complexity and performance of the current algorithm cannot be taken into account. It is used in navigation information processing to obtain higher-precision positioning results.
In practical application scenarios, combining the multipath technologies of each stage can obtain better multipath mitigation performance. For example, for a high-precision receiver, the signal system can choose BOC modulation, choose a choke ring antenna and consider its location and assumptions, and use CCRW technology in baseband signal processing. However, it should be noted that for signals with different modulation methods, the effects of each multipath mitigation technology in baseband signal processing stage are not consistent. Therefore, it is also an important research content to select appropriate multipath mitigation technology in each stage of signal processing. In this way, each stage has exerted its effect on multipath mitigation. It is significant for the GNSS receiver to reduce the influence of multipath signals and improve the measurement accuracy.
5 Conclusion
This paper established a simplified multipath signal model using the multipath signal’s attenuation, propagation delay, and carrier phase difference parameters. Then the influence of multipath signals on GNSS’s code tracking loop and carrier tracking loop was analyzed. The influence of the multipath signal on the code tracking loop is mainly reflected in the correlation function, and the influence on the carrier tracking loop is reflected in the carrier phase measurement. The multipath signal affects the two loops and reduces the measurement accuracy. In severe cases, it can cause the receiver to lose the lock.
From the perspective of signal system design, this paper introduces the principle and method of signal modulation and pseudo-code/carrier selection to achieve multipath mitigation. It points out that the core idea of multipath mitigation through the new system signal design is to improve the signal’s code rate, which provides design ideas for the future new satellite system signals in multipath mitigation. From the perspective of antenna design, this paper introduces the polarized antennas and choke antennas that can achieve multipath mitigation and some multipath mitigation measures. This dramatically reduces the number of multipath signals entering the GNSS receiver. However, the disadvantage is that due to hardware limitations, only the multipath signal at a specific angle can be suppressed, and some multipath signals will still enter the GNSS receiver. From the perspective of baseband signal processing, this paper introduces the classic multipath mitigation techniques based on correlator/discriminator improvements and multipath mitigation techniques based on signal parameter estimation. It is pointed out that the best anti-multipath performance is the dual DELTA technology, and the lowest complexity is the CCRW technology. Then the performance of each algorithm under different modulation methods is compared, and the tracking ambiguity problem that exists when the multipath mitigation technology is applied to BOC modulation is analyzed. From the perspective of navigation data post-processing, this paper introduces the classic multipath suppression method based on statistical signal processing and the multipath suppression method based on an empirical model. This approach does not require improving the receiver’s internal signal processing unit. However, the disadvantage is that performance depends heavily on the redundancy of the measurement and the level of measurement noise and requires long observations.
Finally, according to the existing multipath mitigation technology of these four links and the characteristics and development trends of each technology, the prospect of their future development direction is made. It is pointed out that the multipath resistance method of multiple links at the same time is of great significance to the GNSS receiver to improve the measurement accuracy.
Author contributions
Formal analysis, ZXu, ZXi, JS, and SN; Investigation, ZXu, ZL, ZXi, JS, and SN; Writing original draft preparation, ZXu, ZL, and SN; All authors have read and agreed to the published version of the manuscript.
Funding
This research was funded by the National Natural Science Foundation of China (No.62003354).
Acknowledgments
The authors would like to thank the editors and reviewers for their efforts to help the publication of this paper.
Conflict of interest
The authors declare that the research was conducted in the absence of any commercial or financial relationships that could be construed as a potential conflict of interest.
Publisher’s note
All claims expressed in this article are solely those of the authors and do not necessarily represent those of their affiliated organizations, or those of the publisher, the editors and the reviewers. Any product that may be evaluated in this article, or claim that may be made by its manufacturer, is not guaranteed or endorsed by the publisher.
References
1. Fossen TI. Line-of-sight path-following control utilizing an extended Kalman filter for estimation of speed and course over ground from GNSS positions. J Mar Sci Technol (2022) 27:806–13. doi:10.1007/s00773-022-00872-y
2. Jurišica L, Duchoň F, Kaštan D, Babinec A. High precision GNSS guidance for field mobile robots. Int J Adv Robotic Syst (2012) 9:169. doi:10.5772/52554
3. Guo W, Song W, Niu X, Lou Y, Gu S, Zhang S, et al. Foundation and performance evaluation of real-time GNSS high-precision one-way timing system. GPS Solut (2019) 23:23. doi:10.1007/s10291-018-0811-1
4. Elsheikh M, Noureldin A, Korenberg M. Integration of GNSS precise point positioning and reduced inertial sensor system for lane-level car navigation. IEEE Trans Intell Transport Syst (2022) 23:2246–61. doi:10.1109/TITS.2020.3040955
5. Tanil C, Khanafseh S, Joerger M, Kujur B, Kruger B, de Groot L, Pervan B. Optimal INS/GNSS coupling for autonomous car positioning integrity. In: Proceedings of the 32nd International Technical Meeting of the Satellite Division of The Institute of Navigation (ION GNSS+ 2019) (2019). p. 3123–40. doi:10.33012/2019.17013
6. Hegarty CJ, Chatre E. Evolution of the global navigation SatelliteSystem (GNSS). Proc IEEE (2008) 96:1902–17. doi:10.1109/JPROC.2008.2006090
7. Specht C, Dabrowski PS, Pawelski J, Specht M, Szot T. Comparative analysis of positioning accuracy of GNSS receivers of Samsung Galaxy smartphones in marine dynamic measurements. Adv Space Res (2019) 63:3018–28. doi:10.1016/j.asr.2018.05.019
8. Cheng C, Tourneret JY. An EM-based multipath interference mitigation in GNSS receivers. Signal Process. (2019) 162:141–52. doi:10.1016/j.sigpro.2019.04.018
9. Dutta P, Halder T, Banerjee S, Basak A, Nanda S, Chakravarty D. Analysis of jamming and anti jamming techniques for Galileo GNSS. Mater Today Proc (2022) 58:489–95. doi:10.1016/j.matpr.2022.03.009
10. Kuusniemi H, Bhuiyan MZH, Strom M, Soderholm S, Jokitalo T, Chen L, Chen R. Utilizing pulsed pseudolites and high-sensitivity GNSS for ubiquitous outdoor/indoor satellite navigation. In: 2012 International Conference on Indoor Positioning and Indoor Navigation (IPIN) (2012). p. 1–7. doi:10.1109/IPIN.2012.6418911
11. Liu Y, Li S, Fu Q, Liu Z, Zhou Q. Analysis of kalman filter innovation-based GNSS spoofing detection method for INS/GNSS integrated navigation system. IEEE Sensors J (2019) 19:5167–78. doi:10.1109/JSEN.2019.2902178
12. Borio D, O'Driscoll C, Fortuny J. GNSS jammers: Effects and countermeasures. In: 2012 6th ESA Workshop on Satellite Navigation Technologies (Navitec 2012) & European Workshop on GNSS Signals and Signal Processing (2012). p. 1–7. doi:10.1109/NAVITEC.2012.6423048
13. Lu Z, Nie J, Chen F, Chen H, Ou G. Adaptive time taps of STAP under channel mismatch for GNSS antenna arrays. IEEE Trans Instrum Meas (2017) 66:2813–24. doi:10.1109/TIM.2017.2728420
14. Lu Z, Chen H, Chen F, Nie J, Ou G. Blind adaptive channel mismatch equalisation method for GNSS antenna arrays. IET Radar, Sonar & Navigation (2018) 12:383–9. doi:10.1049/iet-rsn.2017.0416
15. Kos T, Markezic I, Pokrajcic J. Effects of multipath reception on GPS positioning performance. In: Proceedings ELMAR-2010 (2010). p. 399–402.
16. Peng Xie P, Petovello MG. Measuring GNSS multipath distributions in urban canyon environments. IEEE Trans Instrum Meas (2015) 64:366–77. doi:10.1109/TIM.2014.2342452
17. Spilker JJ, Axelrad P, Parkinson BW, Enge P. Global Positioning System: Theory and Applications, Volume I. Reston, Virginia, USA: American Institute of Aeronautics and Astronautics (1996).
18. Townsend BR, Fenton PC, Van Dierendonck KJ, Van Nee DR. Performance evaluation of the multipath estimating delay lock loop. Navigation (1995) 42:502–14. doi:10.1002/j.2161-4296.1995.tb01903.x
19. Yu L, Liu W, Langley R. Robust adaptive beamforming for multi-path environment based on domain weighted PCA. In: 2007 15th International Conference on Digital Signal Processing. Cardiff, UK: IEEE (2007). p. 91–4. doi:10.1109/ICDSP.2007.4288526
20. Braasch MS. Performance comparison of multipath mitigating receiver architectures. In: 2001 IEEE Aerospace Conference Proceedings (Cat. No. 01TH8542). Big Sky, MT, USA: IEEE (2001). p. 3–1309. vol. 3. doi:10.1109/AERO.2001.931361
21. Lehner A, Steingaß A. Characteristics of the land mobile navigation channel for pedestrian applications. In: Proceedings of the GNSS 2003, European Navigation Conference (2003).
22. Lehner A, Steingaß A. The Influence of Multipath on the positioning error. In: European Navigation Conference (ENC GNSS) (2003).
23. Lopez AR. LAAS/GBAS ground reference antenna with enhanced mitigation of ground multipath. In: Proceedings of the 2008 National Technical Meeting of the Institute of Navigation (2008). p. 389–93.
24. Steingass A, Lehner A. Land mobile satellite navigation-characteristics of the multipath channel. In: Proceedings of the 16th International Technical Meeting of the Satellite Division of the Institute of Navigation (ION GPS/GNSS 2003) (2003). p. 1016–22.
26. Parkinson BW, Spilker JJ. Progress in astronautics and aeronautics: Global positioning system: Theory and applications, vol. 164. Reston, Virginia, USA: Aiaa (1996).
27. Bhuiyan MZH. Analysis of multipath mitigation techniques for satellite-based positioning applications. Ph.D. thesis (2011).
28. Serrano L, Kim D, Langley RB. Carrier-phase multipath calibration in GPS-RTK machine-guidance applications. In: 2008 IEEE/ION Position, Location and Navigation Symposium. Monterey, CA, USA: IEEE (2008). p. 479–88. doi:10.1109/plans.2008.4570023
29. Lau L. Wavelet packets based denoising method for measurement domain repeat-time multipath filtering in GPS static high-precision positioning. GPS Solut (2017) 21:461–74. doi:10.1007/s10291-016-0533-1
30. Vahid AM, Huangfu C, Zhao Y. Wavelet thresholding method based on hypotheses testing and noise estimation for multipath mitigation in GPS receivers. In: 2015 International Conference on Computer Science and Intelligent Communication. Amsterdam, Netherlands: Atlantis Press (2015). p. 341–5. doi:10.2991/csic-15.2015.82
31. Oppenheim AV, Willsky AS, Nawab SH, Hernández GM. Signals & systems. New York City: Pearson Educación (1997).
32. Tian Y, Liu F, Zeng T, Zhang L. Analysis of cross correlation on code tracking and mitigation techniques using advanced correlator. In: Proceedings of the 2010 International Technical Meeting of the Institute of Navigation - Itm 2010. Washington: Inst Navigation (2010). p. 909–15.
33. Betz JW. Binary offset carrier modulations for radionavigation. Navigation (2001) 48:227–46. doi:10.1002/j.2161-4296.2001.tb00247.x
34. Lestarquit L, Artaud G, Issler JL. AltBOC for dummies or everything you always wanted to know about AltBOC. In: Proceedings of the 21st International Technical Meeting of the Satellite Division of The Institute of Navigation (ION GNSS 2008) (2008). p. 961–70.
35. Ke T, Hu X, Liu Y, Ran Y. The evaluation methods of sampling rate performance in GNSS receiver. In: International Conference on Space Information Technology 2009 (SPIE) (2010). p. 688–93. vol. 7651. doi:10.1117/12.855386
36. Benedetto F, Giunta G, Lohan ES, Renfors M. A fast unambiguous acquisition algorithm for BOC-modulated signals. IEEE Trans Veh Technol (2013) 62:1350–5. doi:10.1109/TVT.2012.2228681
37. Sun X, Liu Z, Ji Y, Yan S, Liang W. Sub cross-correlation shift cancellation technique for unambiguous tracking of BOC (kn, n) signals. In: 2020 8th International Conference on Digital Home (ICDH). Piscataway, NJ, USA: IEEE (2020). p. 239–47. doi:10.1109/ICDH51081.2020.00048
38. Yang C, Soloviev A, Vadlamani A, Ha JC. Long coherent combining and integration for BOC signal acquisition under strong interference. In: Proceedings of the 33rd International Technical Meeting of the Satellite Division of the Institute of Navigation (ION GNSS+ 2020) (2020). p. 2758–78. doi:10.33012/2020.17593
39. Nunes FD, Sousa FM, Leitao JM. Gating functions for multipath mitigation in GNSS BOC signals. IEEE Trans Aerosp Electron Syst (2007) 43:951–64. doi:10.1109/taes.2007.4383585
40. Yao Z, Gao Y, Gao M, Lu M. Generalized theory of BOC signal unambiguous tracking with two-dimensional loops. IEEE Trans Aerosp Electron Syst (2017) 53:3056–69. doi:10.1109/taes.2017.2726878
41. Tan S, Zhou B, Guo S, Liu Z. Studies of Compass navigation signals design. Scientia Sinica (Physica, Mechanica & Astronomica) (2010) 40:514–9.
42. Shen Zhang S, Bingyu Li B. The influence of scattering on multipath channel characteristic of tunnel. In: 6th International SYmposium on Antennas, Propagation and EM Theory, 2003. Proceedings. 2003 (2003). p. 576–8. doi:10.1109/ISAPE.2003.1276756
43. Aubry A, De Maio A, Foglia G, Orlando D. Diffuse multipath exploitation for adaptive radar detection. IEEE Trans Signal Process (2015) 63:1268–81. doi:10.1109/TSP.2014.2388439
44. Lu Z, Song J, Huang L, Ren C, Xiao Z, Li B. Distortionless 1/2 overlap windowing in frequency domain anti-jamming of satellite navigation receivers. Remote Sensing (2022) 14:1801. doi:10.3390/rs14081801
45. Song J, Lu Z, Xiao Z, Li B, Sun G. Optimal order of time-domain adaptive filter for anti-jamming navigation receiver. Remote Sensing (2022) 14:48. doi:10.3390/rs14010048
46. Ni S, Ren B, Chen F, Lu Z, Wang J, Ma P, et al. GNSS spoofing suppression based on multi-satellite and multi-channel array processing. Front Phys (2022) 10. doi:10.3389/fphy.2022.905918
47. Granger R, Simpson S. An analysis of multipath mitigation techniques suitable for geodetic antennas. In: Proceedings of the 21st International Technical Meeting of the Satellite Division of The Institute of Navigation (ION GNSS 2008) (2008). p. 2755–65.
48. Cardellach E, Tomas S, Oliveras S, Padulles R, Rius A, de la Torre-Juarez M, et al. Sensitivity of PAZ LEO polarimetric GNSS radio-occultation experiment to precipitation events. IEEE Trans Geosci Remote Sensing (2015) 53:190–206. doi:10.1109/TGRS.2014.2320309
49. Xie L, Cui X, Zhao S, Lu M. Mitigating multipath bias using a dual-polarization antenna: Theoretical performance, algorithm design, and simulation. Sensors (2017) 17:359. doi:10.3390/s17020359
50. Chu IW, Juang JC. Adaptive multipath mitigation in urban GNSS positioning. In: 27th International Technical Meeting of the Satellite Division of the Institute of Navigation, ION GNSS 2014; Tampa, Florida (2014). p. 2713–9.
51. Tranquilla JM, Carr J, Al-Rizzo HM. Analysis of a choke ring groundplane for multipath control in global positioning system (GPS) applications. IEEE Trans Antennas Propagat (1994) 42:905–11. doi:10.1109/8.299591
52. Allahgholi Pour Z, Shafai L. A ring choke excited Compact dual-mode circular waveguide feed for offset reflector antennas. IEEE Trans Antennas Propagat (2012) 60:3011–5. doi:10.1109/TAP.2012.2194662
53. Khosravi F, Moghadas H, Mousavi P. A GNSS antenna with a polarization selective surface for the mitigation of low-angle multipath interference. IEEE Trans Antennas Propagat (2015) 63:5287–95. doi:10.1109/tap.2015.2491323
54. Gafarov ER, Stankovsky AV, Salomatov YP. A GNSS dipole antenna with a meander-line polarizer for the reduction of multipath interference. In: 2017 Radiation and Scattering of Electromagnetic Waves (RSEMW). Divnomorskoe: IEEE (2017). p. 311–3. doi:10.1109/RSEMW.2017.8103659
55. Kunysz W. A three dimensional choke ring ground plane antenna. In: Proceedings of the 16th International Technical Meeting of the Satellite Division of the Institute of Navigation (ION GPS/GNSS 2003) (2003). p. 1883–8.
56. Boccia L, Amendola G, Di Massa G, Giulicchi L. Shorted annular patch antennas for multipath rejection in GPS-based attitude determination systems. Microw Opt Technol Lett (2001) 28:47–51. doi:10.1002/1098-2760(20010105)28:1<47::aid-mop14>3.0.co;2-3
57. EnCheng W, Zhuopeng W, Zhang C. A wideband antenna for global navigation satellite system with reduced multipath effect. Antennas Wirel Propag Lett (2013) 12:124–7. doi:10.1109/LAWP.2013.2243695
58. Taghdisi E, Ghaffarian S, Mirzavand R, Mousavi P. Compact substrate integrated choke ring ground structure for high-precision GNSS applications. In: 2020 IEEE International Symposium on Antennas and Propagation and North American Radio Science Meeting. Montreal, QC, Canada: IEEE (2020). p. 1705–6. doi:10.1109/ieeeconf35879.2020.9330348
59. Yang P, Yan F, Zhou L, Gao M, Yang F. A small low-multipath GNSS antenna using annular slot loaded ground plane. In: 2016 IEEE 5th Asia-Pacific Conference on Antennas and Propagation (APCAP). Kaohsiung, Taiwan: IEEE (2016). p. 349–50. doi:10.1109/APCAP.2016.7843237
60. Thornberg DB, Thornberg DS, Dibenedetto MF, Braasch MS, Van Graas F, Bartone C. LAAS integrated multipath-limiting antenna. Navigation (2003) 50:117–30. doi:10.1002/j.2161-4296.2003.tb00323.x
61. Ray J, Cannon M, Fenton P. GPS code and carrier multipath mitigation using a multiantenna system. IEEE Trans Aerosp Electron Syst (2001) 37:183–95. doi:10.1109/7.913677
62. Ray JK, Cannon ME, Fenton PC. Mitigation of static carrier-phase multipath effects using multiple closely spaced antennas. Navigation (1999) 46:193–201. doi:10.1002/j.2161-4296.1999.tb02406.x
63. Lu Z, Nie J, Wan Y, Ou G. Optimal reference element for interference suppression in GNSS antenna arrays under channel mismatch. IET Radar, Sonar & Navigation (2017) 11:1161–9. doi:10.1049/iet-rsn.2016.0582
64. Siridhara AL, Ratnam DV. Mitigation of multipath effects based on a robust fractional order bidirectional least mean square (FOBLMS) beamforming algorithm for GPS receivers. Wireless Pers Commun (2020) 112:743–61. doi:10.1007/s11277-020-07071-1
65. Huang L, Lu Z, Xiao Z, Ren C, Song J, Li B. Suppression of jammer multipath in GNSS antenna array receiver. Remote Sensing (2022) 14:350. doi:10.3390/rs14020350
66. Ma C, Tang X, Liu Y, Xiao Z, Sun G. A method of carrier phase multipath mitigation based on punctual code correlation reference waveform. In: China Satellite Navigation Conference (CSNC) 2018 Proceedings. Singapore: Springer (2018). p. 477–87. doi:10.1007/978-981-13-0029-5_42
67. Lau L, Cross P. Investigations into phase multipath mitigation techniques for high precision positioning in difficult environments. J Navigation (2007) 60:457–82. doi:10.1017/S0373463307004341
68. Jovanovic A, Tawk Y, Botteron C, Farine PA. Multipath mitigation techniques for CBOC, TMBOC and AltBOC signals using advanced correlators architectures. In: 2010 Ieee-Ion Position Location and Navigation Symposium Plans. New York: IEEE (2010). p. 580–9. doi:10.1109/plans.2010.5507231
69. Braasch M, van Dierendonck A. GPS receiver architectures and measurements. Proc IEEE (1999) 87:48–64. doi:10.1109/5.736341
70. Phelts RE, Enge P. The case for narrowband receivers. In: Proceedings of the 2000 National Technical Meeting of The Institute of Navigation (2000). p. 511–20.
71. Van Dierendonck A, Fenton P, Ford T. Theory and performance of narrow correlator spacing in a GPS receiver. Navigation (1992) 39:265–83. doi:10.1002/j.2161-4296.1992.tb02276.x
72. Xuefen Z, Xiyuan C, Xin C. Comparison between strobe correlator and narrow correlator on MBOC DLL tracking loop. In: 2011 IEEE International Instrumentation and Measurement Technology Conference (2011). p. 1–4. doi:10.1109/IMTC.2011.5944083
73. Betz JW, Fine PB. Effect of narrow correlator spacing on code tracking accuracy. In: Proceedings of the 2000 National Technical Meeting of The Institute of Navigation (2000). p. 716–23.
74. Wu J, Tang X, Li Z, Li C, Wang F. Cascaded interference and multipath suppression method using array antenna for GNSS receiver. IEEE Access (2019) 7:69274–82. doi:10.1109/ACCESS.2019.2918775
75. Liu Z, Pang J, Liu Y, Wang F. Double strobe technique for unambiguous tracking of TMBOC modulated signal in GPS. IEEE Signal Process Lett (2015) 22:2204–8. doi:10.1109/LSP.2015.2470240
76. Zhang K, Wang F. Joint code multipath mitigation for composite binary offset carrier modulated signals. Electron Lett (2011) 47:1374–5. doi:10.1049/el.2011.2911
77. Liu Z, Huang Y, Tang X, Wang F. Unambiguous S-curve shaping for multipath mitigation for BOC(1,1) modulated signals in GNSS. IEICE Trans Commun (2015) E98.B:1924–30. doi:10.1587/transcom.E98.B.1924
78. Garin L, van Diggelen F, Rousseau JM. Strobe & edge correlator multipath mitigation for code. In: Proceedings of the 9th International Technical Meeting of the Satellite Division of the Institute of Navigation (ION GPS 1996) (1996). p. 657–64.
79. Lee YC. Compatibility of the new military GPS signals with non-aviation receivers. In: ION 58th Annual Meeting and CIGTF 21st Guidance Test Symposium (2002).
80. Veitsel VA, Zhdanov AV, Zhodzishsky MI. The mitigation of multipath errors by strobe correlators in GPS/GLONASS receivers. GPS Solutions (1998) 2:38–45. doi:10.1007/PL00000035
81. Eissfeller MI. Comparison of multipath mitigation techniques with consideration of future signal structures. In: 16th International Technical Meeting of the Satellite Division of the Institute of Navigation (ION GPS/GNSS 2003) (2003).
82. Lin X, Zhang L, Ruan H, Liu F. Robustness and accuracy analysis of a compatible narrow correlation assisted double delta algorithm. In: J Sun, W Jiao, H Wu, and M Lu, editors. China satellite navigation conference (csnc) 2014 proceedings, vol I, Vol. 303. New York: Springer (2014). p. 661–71. doi:10.1007/978-3-642-54737-9_57
83. So H, Kim G, Lee T, Jeon S, Kee C. Modified high-resolution correlator technique for short-delayed multipath mitigation. J Navigation (2009) 62:523–42. doi:10.1017/S037346330900530X
84. Liu Z, Pang J, Liu Y, Wang F. Double strobe technique for unambiguous tracking of TMBOC modulated signal in GPS. IEEE Signal Process Lett (2015) 22:2204–8. doi:10.1109/LSP.2015.2470240
85. Chen M, Sun G. A strategy of multipath mitigation based on modified double-delta phase discriminator and TK. In: B Xu, editor. Proceedings of 2018 Ieee 3rd Advanced Information Technology, Electronic and Automation Control Conference (Iaeac 2018). New York: IEEE (2018). p. 321–5.
86.[Dataset] Van Nee RD. Apparatus for estimating a line of sight signal propagation time using a reduced-multipath correlation function (1997).
87. Yao Z, Lu M, Feng Z. Unambiguous sine-phased binary offset carrier modulated signal acquisition technique. IEEE Trans Wireless Commun (2010) 9:577–80. doi:10.1109/TWC.2010.02.091066
88. Wang C, Cui X, Wen J, Lu M, Wang J. An offset correlator based multipath mitigation method for dual BPSK tracking technique of wideband BOC signals. In: Proceedings of the 32nd International Technical Meeting of the Satellite Division of the Institute of Navigation (Ion Gnss+ 2019). Washington: Inst Navigation (2019). p. 3498–505. doi:10.33012/2019.16931
89. Orabi M, Khalife J, Abdallah AA, Kassas ZM, Saab SS. A machine learning approach for GPS code phase estimation in multipath environments. In: 2020 IEEE/ION Position, Location and Navigation Symposium (PLANS) (2020). p. 1224–9. doi:10.1109/PLANS46316.2020.9110155
90. Jia Q, Wu R, Wang W, Lu D, Wang L, Li J. Multipath interference mitigation in GNSS via WRELAX. GPS Solut (2017) 21:487–98. doi:10.1007/s10291-016-0538-9
91. Weill LR. Multipath mitigation using modernized GPS signals: How good can it get? In: Proceedings of the 15th International Technical Meeting of the Satellite Division of The Institute of Navigation (ION GPS 2002) (2002). p. 493–505.
92. Fenton P, Jones J. The theory and performance of NovAtel Inc.’s vision correlator. In: Proceedings of the 18th International Technical Meeting of the Satellite Division of The Institute of Navigation (2005).
93. Rae A, Basir O. Reducing multipath effects in vehicle localization by fusing GPS with machine vision. In: 2009 12th International Conference on Information Fusion. Seattle, WA, USA: IEEE (2009). p. 2099–106.
94. Chen X, Dovis F, Peng S, Morton Y. Comparative studies of GPS multipath mitigation methods performance. IEEE Trans Aerosp Electron Syst (2013) 49:1555–68. doi:10.1109/TAES.2013.6558004
95. Fang X, Chen X, Wang Y, Cai L, Ying R, Liu P. Multipath channel characteristics and multipath life-time model research for different GNSS orbit satellites. In: Proceedings of the 28th International Technical Meeting of the Satellite Division of the Institute of Navigation (ION GNSS+ 2015) (2015). p. 2957–65.
96. Li J, Wang W, Lu D, Wang L, Wu R. A correlate-based GPS multipath time delay estimation algorithm. In: J Sun, W Jiao, H Wu, and M Lu, editors. China satellite navigation conference (csnc) 2014 proceedings, vol I, Vol. 303. New York: Springer (2014). p. 53–62. doi:10.1007/978-3-642-54737-9_6
97. Tamazin M, Noureldin A, Korenberg MJ, Kamel AM. A new high-resolution GPS multipath mitigation technique using fast orthogonal search. J Navigation (2016) 69:794–814. doi:10.1017/S0373463315001022
98. Axelrad P, Comp CJ, Macdoran PF. SNR-based multipath error correction for GPS differential phase. IEEE Trans Aerosp Electron Syst (1996) 32:650–60. doi:10.1109/7.489508
99. Zheng J, Yan D, Yan M, Li Y, Zhao Y. An unscented kalman filter online identification approach for a nonlinear ship motion model using a self-navigation test. Machines (2022) 10:312. doi:10.3390/machines10050312
100. Choi K, Bilich A, Larson KM, Axelrad P. Modified sidereal filtering: Implications for high-rate GPS positioning. Geophys Res Lett (2004) 31. doi:10.1029/2004GL021621
101. Satirapod C, Rizos C. Multipath mitigation by wavelet analysis for gps base station applications. Surv Rev (2005) 38:2–10. doi:10.1179/sre.2005.38.295.2
102. Souza E, Monico G, Polezel W, Pagamisse A. An effective wavelet method to detect and mitigate low-frequency multipath effects. VI Hotine-marussi Symp Theor Comput Geodesy (2008) 132:179–84. doi:10.1007/978-3-540-74584-6_28
103. Dai W, Huang D, Cai C. Multipath mitigation via component analysis methods for GPS dynamic deformation monitoring. GPS Solut (2014) 18:417–28. doi:10.1007/s10291-013-0341-9
104. Kim Y, Kim J, Yu S, Kee C, Park B. Multipath mitigation using GPS/INS integrated navigation with adaptive kalman filtering. In: Proceedings of the 2016 International Technical Meeting of the Institute of Navigation (2016). p. 893–901. doi:10.33012/2016.13471
105. Zheng NS, Cai LS, Bian HF, Lin C. Hybrid particle filtering algorithm for GPS multipath mitigation. Trans Nonferrous Met Soc China (2014) 24:1554–61. doi:10.1016/S1003-6326(14)63225-8
106. Groves PD, Jiang Z, Skelton B, Cross PA, Lau L, Adane Y, et al. Novel multipath mitigation methods using a dual-polarization antenna. In: Proceedings of the 23rd International Technical Meeting of the Satellite Division of the Institute of Navigation (ION GNSS 2010) (2010). p. 140–51.
107. Liu H, Li X, Ge L, Rizos C, Wang F. Variable length LMS adaptive filter for carrier phase multipath mitigation. GPS Solut (2011) 15:29–38. doi:10.1007/s10291-010-0165-9
108. Niu M, Zhan X, Liu L, Su X. A class of SLCE-based spreading codes for GNSS use. IEEE Trans Aerosp Electron Syst (2013) 49:698–702. doi:10.1109/TAES.2013.6404137
109. Taghdisi E, Ghaffarian MS, Mirzavand R. Low-profile substrate integrated choke rings for GNSS multipath mitigation. IEEE Trans Antennas Propagat (2022) 70:1706–18. doi:10.1109/TAP.2021.3118809
110. Fernandez J, Pulido JA, Wis M, Closas P, Fernandez-Prades C, Zanier F, et al. Artemisa: New gnss receiver processing techniques for positioning and multipath mitigation. In: 6th Esa Workshop on Satellite Navigation Technologies (Navitec 2012) and European Workshop on Gnss Signals and Signal Processing. New York: IEEE (2012). doi:10.1109/navitec.2012.6423092
111. Zhao Y, Xue X, Zhang T. Receiver-channel based adaptive blind equalization approach for GPS dynamic multipath mitigation. Chin J Aeronautics (2013) 26:378–84. doi:10.1016/j.cja.2013.02.015
112. Farnham T. Indoor localisation of IoT devices by dynamic radio environment mapping. In: 2019 IEEE 5th World Forum on Internet of Things (WF-IoT) (2019). p. 340–5. doi:10.1109/WF-IoT.2019.8767296
113. Hamet P, Tremblay J. Artificial intelligence in medicine. Metabolism (2017) 69:S36–S40. doi:10.1016/j.metabol.2017.01.011
114. Hwang GJ, Tu YF. Roles and research trends of artificial intelligence in mathematics education: A bibliometric mapping analysis and systematic review. Mathematics (2021) 9:584. doi:10.3390/math9060584
115. Mhlanga D. Industry 4.0 in finance: The impact of artificial intelligence (AI) on digital financial inclusion. Int J Financial Stud (2020) 8:45. doi:10.3390/ijfs8030045
116. Blais A, Couellan N, Munin E. A novel image representation of GNSS correlation for deep learning multipath detection. Array (2022) 14:100167. doi:10.1016/j.array.2022.100167
117.[Dataset] Gonzalez T, Blais A, Couëllan N, Ruiz C. Distributional loss for convolutional neural network regression and application to GNSS multi-path estimation (2022). doi:10.48550/arXiv.2206.01473
118. Klimenko MY, Veitsel AV. Evaluation of neural network-based multipath mitigation approach for the GNSS receivers. In: 2021 Systems of Signal Synchronization, Generating and Processing in Telecommunications (SYNCHROINFO) (2021). p. 1–5. doi:10.1109/SYNCHROINFO51390.2021.9488410
119. Li H, Borhani-Darian P, Wu P, Closas P. Deep learning of GNSS signal correlation. In: Proceedings of the 33rd International Technical Meeting of the Satellite Division of The Institute of Navigation (ION GNSS+ 2020) (2020). p. 2836–47. doi:10.33012/2020.17598
120. Li H, Borhani-Darian P, Wu P, Closas P. Deep neural network correlators for GNSS multipath mitigation. IEEE Trans Aerosp Electron Syst (2022) 2022:1–23. doi:10.1109/TAES.2022.3197098
Keywords: global navigation satellite system, multipath mitigation, new signal system, anti-multipath antenna, baseband signal processing, navigation data processing
Citation: Xue Z, Lu Z, Xiao Z, Song J and Ni S (2022) Overview of multipath mitigation technology in global navigation satellite system. Front. Phys. 10:1071539. doi: 10.3389/fphy.2022.1071539
Received: 16 October 2022; Accepted: 28 November 2022;
Published: 09 December 2022.
Edited by:
Olga V. Khabarova, Institute of Terrestrial Magnetism Ionosphere and Radio Wave Propagation (RAS), RussiaCopyright © 2022 Xue, Lu, Xiao, Song and Ni. This is an open-access article distributed under the terms of the Creative Commons Attribution License (CC BY). The use, distribution or reproduction in other forums is permitted, provided the original author(s) and the copyright owner(s) are credited and that the original publication in this journal is cited, in accordance with accepted academic practice. No use, distribution or reproduction is permitted which does not comply with these terms.
*Correspondence: Zukun Lu, bHV6dWt1bkBudWR0LmVkdS5jbg==; Shaojie Ni, bmlzaGFvamllMTIzQDEyNi5jb20=